ABSTRACT
CD39 and CD73 are key enzymes in the adenosine (ADO) pathway. ADO modulates pathophysiological responses of immune cells, including B cells. It has recently emerged that a subpopulation of ADO-producing CD39+CD73+ B cells has regulatory properties. Here, we define the CD39high subset of these cells as the major contributor to the regulatory network operated by human B lymphocytes. Peripheral blood B cells were sorted into CD39neg, CD39inter and CD39high subsets. The phenotype, proliferation and IL-10 secretion by these B cells were studied by flow cytometry. 5′-AMP and ADO levels were measured by mass spectrometry. Agonists or antagonists of A1R, A2AR and A3R were used to study ADO-receptor signaling in B cells. Inhibition of effector T-cell (Teff) activation/proliferation by B cells was assessed in co-cultures. Cytokine production was measured by Luminex. Upon in vitro activation and culture of B cells, the subset of CD39high B cells increased in frequency (p < 0.001). CD39high B cells upregulated CD73 expression, proliferated (approximately 40% of CD39high B cells were Ki-67+ and secreted fold-2 higher IL-10 and ADO levels than CD39neg or CD39inter B cells. CD39high B cells co-cultured with autologous Teff suppressed T-cell activation/proliferation and secreted elevated levels of IL-6 and IL-10. The A1R and A2AR agonists promoted expansion and functions of CD39high B cells. CD39 ectonucleotidase is upregulated in a subset of in vitro-activated B cells which utilize ADO and IL-10 to suppress Teff functions. Proliferation and functions of these CD39high B cells are regulated by A1R- and A2AR-mediated autocrine signaling.
Introduction
ADO is a purine nucleoside which plays an important role in various pathophysiological processes.Citation1 ADO and its receptors (ADOR) are responsible for transmission of signals critical to a variety of biological systems and pathways, including the immune system.Citation2,3 The ADO pathway is a prominent component of inflammatory microenvironments, where an excess of exogenous (e)ATP is hydrolyzed to ADO in two successive steps by the purinergic enzymes, ectonucleoside triphosphate diphosphohydrolase (NTPDase1/CD39) and ecto-5′-nucleotidase (CD73).Citation4 The two ectoenzymes are expressed by a variety of tissue cells and by various cell types of the hematopoietic system.Citation5 Once released from cells into the extracellular space, ADO engages the P1 ADO receptors, A1, A2A, A2B and A3 on target cells. Immune cells express ADOR which contribute to the regulation of immune responses.Citation5 T lymphocytes appear to mainly utilize A2AR, whereby ADO interacting with the receptor transmits immunosuppressive signals, leading to activation of adenylyl cyclase (AC), upregulation of cAMP levels and inhibition of Teff functions.Citation6,7 In contrast, peripheral regulatory T cells (pTreg) or myeloid-derived suppressor cells (MDSC) are activated by ADO signaling via A2AR and their immunosuppressive functions are enhanced.Citation8,9 The role of A1R or A3R in ADO-mediated immune regulation is not entirely clear, although 5′-AMP was reported to be an A1R agonist with affinity equal to or better than that of ADO.Citation10 In cancer, ADO is viewed as a pro-tumor factor which suppresses antitumor functions of Teff and promotes functions of suppressor cells (Treg and MDSC).Citation11,12
Generally, B cells are viewed as promoters of T-cell activation and proliferation. However, recent studies identified a small subset of CD19+CD25+ circulating B cells that can suppress functions of other lymphocytes.Citation13 This B-cell subset (“Breg”) directly suppresses proliferation of CD4+ T cells by producing IL-10 or TGF-β or acting indirectly, promotes expansion and functions of Treg.Citation13 Our recent study shows that upon in vitro activation, human CD19+ B cells inhibit Teff proliferation; in contrast, resting B cells promote Teff proliferation.Citation14 T-cell suppression by activated B cells is associated with upregulation of CD39 on the B-cell surface.Citation14 Activated B cells in the presence of eATP upregulate CD39 but downregulate CD73 expression and mainly produce 5′-AMP but little ADO.Citation14 Nevertheless, these B cells inhibit T-cell proliferation and cytokine production by a mechanism presumably driven by 5′-AMP signaling.Citation14 As 5′-AMP was reported to be an A1R agonist,Citation10 we surmise that functions of A1R+ T cells are inhibited not only by ADO via A2AR but also by B cell-derived 5′-AMP signaling via the A1R. The molecular mechanisms involved in the ATP-driven suppression of T-cell functions by B cells remain poorly understood, and the identity of Breg responsible for this effect and their characteristics remain unclear. In fact, the precise Breg phenotype has been elusive and seems to be species dependent and modulated by environmental or contextual cellular interactions.Citation15 Variously described as CD19+CD38highCD24high or CD19+CD25high B cells, Breg in humans regulate functions of Th1 helper cells by producing immunosuppressive IL-10 or degranulation of perforin/granzyme molecules, respectively.Citation16,17
The antibody-independent regulation of T-cell functions by B cells is of great interest, largely because of the potential involvement of Breg in inflammation, autoimmune diseases and cancer.Citation18,19 Functional impairments of CD19+CD38highCD24high Breg in systemic lupus erythematosus (SLE) patientsCitation17 and an increased frequency of CD19+CD25high Breg during clinical manifestations of multiple sclerosisCitation16 illustrate their role in autoimmune diseases. Contributions of Breg to cancer progression are poorly understood. On the other hand, the use of ADO by Treg for suppression of antitumor-reactive T cells in the tumor microenvironment represents a potentially important immunoregulatory mechanism operating in cancer.Citation20 Given the current data emphasizing the critical role of the B-cell presence in the immune signature of human tumors for outcome and responses to therapy,Citation21 the mechanisms B cells use to mediate suppression of antitumor responses are of primary interest. The major objective of this study was to further evaluate the phenotypic characteristics and functional roles of human activated CD39+ B cells producing 5′-AMP and ADO in regulating T lymphocyte responses.
Results
In vitro-activated B cells upregulate CD39 surface expression levels
Resting and activated B cells were tested for CD39 and CD73 surface expression levels. Although there was no significant difference between the frequency of CD20+CD39+ and CD20+CD73+ cells (), mean expression levels (MFI) of CD39 were significantly higher in in vitro-activated B cells (12 ± 1.2 and 5.6 ± 0.34 with p < 0.001) than resting B cells (). The MFI for CD73 tended to be higher in activated B cells (). Also, the frequency of CD20+ CD39high B cells was significantly increased upon B-cell activation relative to that in resting B cells (5.6 ± 0.1 and 1.4 ± 0.1,respectively) with the p < 0.0001) ().
Figure 1. CD39 and CD73 expression in resting and activated human B cells. CD20+ B cells were tested by flow cytometry immediately after isolation from the peripheral blood or after 4 d of activation in the presence of IL-4 and CD40L. (A) Representative histograms illustrating upregulation of CD39 expression levels in activated B cells. Isotype control (left), resting B cells (center) and activated B cells (right). (B) Percentages of CD39+ B cells in resting and activated populations were comparable (NSD). CD39+ B cells accounted for > 90% of all cells. (C) Expression levels (Mean Fluorescence Intensity, MFI) of CD39 in resting and activated B-cells **p < 0.001. (D) Percentages of CD39high B cells in resting and activated populations. ***p < 0.0001. (E) Percentages of CD73+ B cells in resting and activated populations (NSD). (F) MFI of CD73 in resting and activated B cells. The data are mean values ± S.E.M. of five independent experiments, as determined by Student’s t-test.)
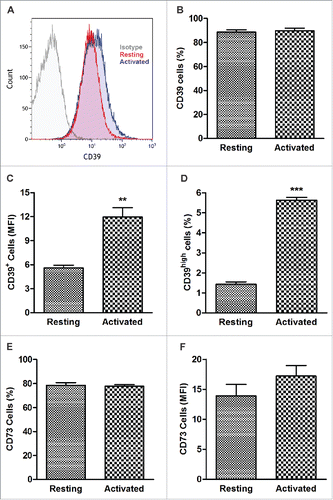
CD39high B cells have a regulatory phenotype
Upon sorting cultured B cells into the CD39neg, CD39inter and CD39high subsets, expression of IL-10 was tested by flow cytometry (). IL-10 production was previously described as a characteristic attribute of Breg.Citation22 In comparison to resting B cells, activated B cells upregulated IL-10 expression, and the fold increase was significantly higher in the CD39high subset than in the other two subsets (). Resting B cells used as controls in these experiments expressed no IL-10 (data not shown). In addition, sorted B cells (CD39neg, CD39inter and CD39high) were tested for surface expression of CD24, CD25, CD38 by flow cytometry (). B cells that were CD39high also expressed higher levels of CD25 () and somewhat higher levels of CD24 () than B cells in the other two subsets. CD38high, another marker reported to characterize Breg, was only upregulated in CD39high B cells (). In aggregate, these results indicate that the CD39high B-cell subset has the phenotype previously described for BregCitation13,17 and that high levels of CD39 and IL-10 expression in this B-cell subset are consistent with its regulatory potential.
Figure 2. Co-Expression of IL-10, CD39, CD24, CD25 and CD38 in the three sorted subsets of CD39+ B cells. Activated B cells were sorted for CD39 expression levels and analyzed by flow cytometry for co-expression of IL-10 (A), CD25 (B), CD24 (C) and CD38 (D–F). In (B) and (C), CD39neg cells are green, CD39inter cells are blue and CD39high cells are red. In (D–E), dot plots show CD38 expression levels in CD39neg B cells (D), CD39inter B cells (E) and CD39high B cells (F). The data are representative of three independent experiments (B–F). In (A), the data are mean values ± SEM of three independent experiments as determined by one-way ANOVA followed by post-hoc comparisons (Tukey). The same statistical analysis was used for the data shown in .
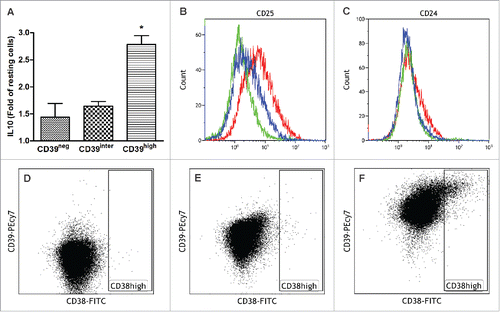
Figure 3. Expression of CD39 and CD73 on the surface of CD39neg, CD39inter and CD39high B cells and 5′AMP or adenosine production. Activated B cells were sorted relative to CD39 expression and analyzed by flow cytometry for surface expression of CD39 (A) or CD73 (B). In (A), CD39high B cells had higher (p < 0.001) mean expression levels of CD 39 than CD39inter B cells. In (B), significantly higher percentages (*p < 0.05) of CD39inter and CD39high B cells than CD39neg B cells co-expressed CD73. The results are presented as mean values ± S.E.M. of three independent experiments. In (C) and (D), the subsets of B cells (25,000 cells/well) were incubated with 20 µM ATP and the production 5′AMP and adenosine were analyzed by mass spectrometry. (C) 5′AMP production after B cell incubation in the presence of ATP with or without CD39-specific blocking Ab (or isotype control) is higher in CD39high B cells (ap < 0.001) than in CD39inter B cells (b). (D) Adenosine production after B-cell incubation in presence of ATP with or without CD39-specific blocking Ab (or isotype control) was also higher (cp < 0.05) in CD39high B cells than in CD39inter B cells (d). The results are presented as mean values ± S.E.M. of three independent experiments. CD39neg B cells produced minimal levels of 5′-AMP or ADO. ND stands for Not Detectable.
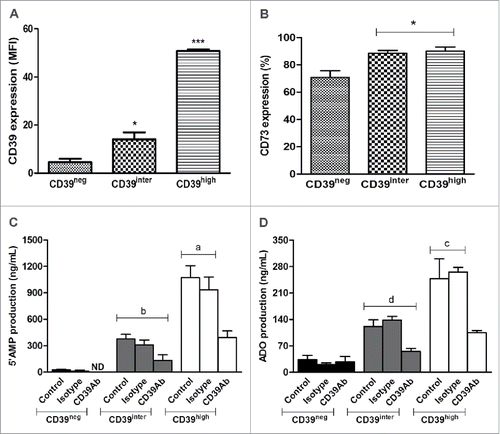
5′-AMP and ADO production by CD39inter and CD39high B cells
Sorted CD39neg, CD39inter and CD39high B cells were tested for levels (MFI) of CD39 expression and co-expression of CD73 (). As expected, an increase in CD39 expression was significantly higher (p < 0.001) in CD39high than CD39inter B cells. The frequency of sorted CD39inter and CD39high B cells co-expressing CD73 was equally high (approaching 90%), while significantly fewer (70%; p < 0.05) of CD39neg B cells were CD73 (). Next, the sorted B-cell subsets were pre-incubated in the presence of exogenously added ATP, and assessed for the production of 5′-AMP or ADO by mass spectrometry. To confirm the role of CD39 ectoenzyme in the pathway, anti-CD39 blocking Ab (or isotype control Ab) was added to B cells 2 h before incubation of B cells with ATP. As expected, CD39high B cells hydrolyzed significantly more ATP and ADP to 5′AMP than B cells in the CD39inter subset (p < 0.001, ). As the production of 5′AMP was increased in CD39high cells and CD73 expression was also high in these B cells, they produced significantly more ADO than CD39inter B cells (p < 0.05, ). In the presence of blocking anti-CD39 Ab, production of 5′AMP and ADO by CD39high B cells was significantly reduced (p < 0.001 and p < 0.05, ). CD39neg B cells produced minimal levels of 5′-AMP or ADO.
Proliferation of CD39high B cells
In vitro-activated B cells were sorted into the three subsets after 4 d in culture and assessed for Ki-67 expression by flow cytometry. As shown in , Ki-67 was only positive in CD39high B cells. The frequency of Ki-67+ B cells was minimal in the other two B-cell subsets. The quantitative analysis of gated CD39neg and CD39inter subsets showed very low frequency of Ki-67+ cells (1.85 ± 0.45% and 3.83 ± 0.65%, respectively) vs. 40.0 ± 7.4% of Ki-67+ cells in the CD39high B cell subset (p < 0.001). The data indicated that CD39high B cells were the only vigorously proliferating population in 4-d cultures containing CD40L and IL-4 (). In all proliferation experiments, isotype control Abs were used as well as resting B cells, and the results were negative for staining with Ki-67 Abs (data not shown).
Figure 4. The proliferation rate of CD39neg, CD39inter and CD39high B cells. The cells were in vitro-activated, and Ki-67 expression was measured in the three B-cell subsets. (A, B and C) Shown are representative histograms of Ki67 expression in CD39neg, CD39inter and CD39high B cells. (D) Percentages of CD39neg, CD39inter and CD39high B cells that expressed Ki67 upon activation. **CD39high B cells had significantly higher percentages of Ki67+ cells than the other two subsets (*p < 0.001). (E) Proliferation of CD39high B cells and (F) percent of CD39high B cells after modulation of adenosine receptors A1R, A2AR and A3R. In vitro-activated B cells were treated with the following pharmacologic agents: 6, 2-chloro-N6-cyclopentyladenosine (CCPA, A1R agonist); CGS 21680 hydrochloride (A2AR agonist); and 2-Cl-IB-MECA (A3R agonist) and/or ADO receptor (ADOR) antagonists, PSB-36 (A1R antagonist), ZM241385 (A2R antagonist) and PSB-10 (A3R antagonist). ADO agonists and antagonists (all used at 1 µM) were added to in vitro-activated proliferating B cells for 4 d, and Ki-67 expression was measured by flow cytometry. *Significantly different from the control group (p < 0.001).
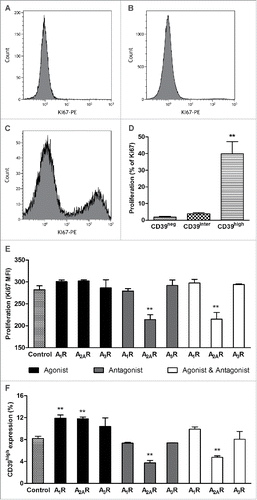
Cytokine profiles of CD39neg, CD39inter and CD39high B cells
In vitro-activated B-cells were sorted into CD39neg, CD39inter or CD39high subsets and the cells were incubated in presence of IL-4 and CD40L for 24 h. Supernatant was collected for cytokine measurements. The data in show that CD39high B cells spontaneously produced more IL-6 (p < 0.0001), TNF-α (p < 0.001) and GM-CSF (p < 0.001) than CD39neg or CD39inter B cells. The cytokine data are consistent with the strong proliferative potential of CD39high B cells.
Table 1. CD39high cells secrete more cytokines during B cell proliferation than CD39neg or CD39inter cells.Footnote
Proliferation of CD39high B cells is not inhibited by autocrine ADO or 5′-AMP
Because CD39high B cells produced high levels of 5′-AMP, ADO and IL-10, all of which are considered to be immunoinhibitory, it was important to determine whether these factors interfered with functions of the producer B cells. To this end, in vitro-activated CD39high B cells were cultured in the presence of 20 µM ATP ± the ecto-5′-nucleotidase (CD73) inhibitor (AMPCP, 10 µM) for 4 d Proliferation (MFI for Ki-67 expression) and CD39 expression levels were measured in these B cells. Neither AMPCP nor ATP nor AMPCP+ATP reduced proliferation or CD39 expression levels of CD39high B cells, suggesting that 5′AMP and ADO produced by these cells mediated no autocrine inhibitory effects (data not shown). There remained a possibility that 5′-AMP produced in excess by CD39high B cells and reported to be able to signal via the A1RCitation10 had stimulatory autocrine effects on proliferation of these cells. In a series of experiments with pharmacologic agonists and antagonists of A1R, A2AR and A3R (but not of A2BR which is not expressed in B cellsCitation14) we showed that only A2AR antagonist, ZM241385, significantly (p < 0.001) decreased both proliferation and CD39 expression in CD39high B cells (). The A1R agonist, CCPA, and A2AR agonist, CSG21680, increased proliferation of CD39high B cells, albeit not significantly, and their CD39 expression levels at p < 0.001. ZM241385 reversed stimulatory effects of CSG21680 on proliferation and CD39 expression levels in CD39high B cells (). Together, these data suggest that the A2AR as well as A1R might mediate autocrine stimulatory effects of ADO or 5′-AMP, respectively, in ADO-producing CD39high B cells, implicating autocrine signaling in their proliferation and survival.
CD39high B-cells suppress functions of autologous T effector cells
To test the immunosuppressive capability of in vitro-activated B cells, we isolated CD39neg, CD39inter or CD39high B cells by sorting and co-incubated them with autologous CD4+CD39neg Teff cells at the ratio of 1:1 in media containing CD40L and IL-4 (B-cell activation) as well as IL-2 and anti-CD3/CD28 Abs (T-cell activation) for 24 h and 72 h. Changes in the activation status of Teff co-incubated with CD39neg, CD39inter or CD39high B cells for 24 h were measured by monitoring CD69 expression levels in Teff. As shown in , CD39high B cells significantly suppressed CD69 expression levels (MFI) in Teff, while CD39neg and CD39inter B cells did not (p < 0.05). In addition, proliferation (frequency of Ki-67+ T cells) was monitored in 72 h co-cultures. In comparison to non-activated (resting) Teff, activated Teff proliferated well in culture as determined by the frequency of Ki-67+ T cells (). Upon co-incubation with CD39high B cells, the percentage of Ki-67+ B cells was significantly reduced (p < 0.05, ). Neither CD39neg nor CD39inter B cells exerted significant anti-proliferative effects on Teff ().
Figure 5. Suppression of activation and proliferation of T-effector cells (CD4+CD39neg) after co-culture with CD39neg, CD39inter and CD39high B cells. The sorted subsets of B cells were co-cultured with T effector cells for 24 h or 72 h and CD69 (A–C) or Ki-67 expression (D–F) levels were measured, respectively. (A, B) Representative histograms illustrate CD69 expression on activated T effector cells cultured alone (A) or after co-culture with CD39high B cells (B). (D, E) Representative histograms illustrate proliferation of activated T-effector cultured alone (D) or after co-culture with CD39high B cells (E). (C and F) Suppression of CD69 expression on activated T effector cells (C) or of proliferation (Ki67 expression) of responder T cells (F) after co-culture with CD39neg, CD39inter and CD39high B cells. Control in (F) denotes proliferation of T effector cells alone. * indicates significant difference (p < 0.05) from the control. In (C), * indicates significant suppression in CD69 expression induced by CD39high cells relative to CD39inter B cells (p < 0.01). CD39neg B cells served as control. The data are presented as mean values ± SEM of three independent experiments.
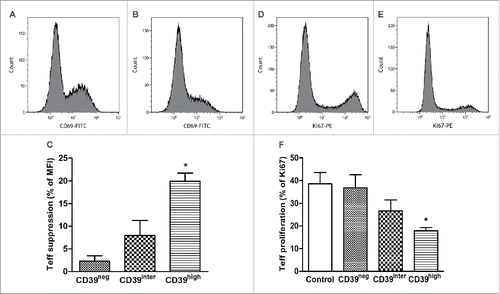
Cytokine production in co-cultures of CD39high B cells with autologous Teff
Teff (CD4+CD39neg) were co-cultured with CD39neg, CD39inter or CD39high B-cells in the presence of T-cell and B-cell activators as described above. The supernatants were collected after 24 h and analyzed for inflammatory and anti-inflammatory cytokines by Luminex. As shown in , a significant (p < 0.05) increase in IL-6 was seen in supernatants of all co-cultures and the highest IL-6 levels were detected in co-cultures of Teff with CD39high B cells (p < 0.0001). Also, IL-10 levels were significantly elevated in co-cultures of Teff with CD39high B cells relative to levels in the supernatants of Teff cultured alone (p < 0.05). No significant differences were seen in IL-1β, GM-CSF and TNF-α levels in co-cultures of in vitro-activated B cells with Teff relative to levels measured in supernatants of Teff cultured alone. Thus, CD39high B cells inducing changes in IL-6 and IL-10 production skewed the cytokine production toward the immunosuppressive profile in these co-cultures.
Table 2. Co-culture between Teff and CD39 B cells subsets.Footnotea
Discussion
The view of B cells and their role in mediating immune responses has drastically changed in the past decade.Citation23 Classically, B cells have been recognized as major effector cells of humoral immunity mediating antibody-dependent reactivity such as opsonization, complement fixation, cytotoxicity or neutralization. More recently, antibody-independent activities of B cells have attracted attention. Emerging evidence indicates that B cells not only differentiate to antibody-producing plasma cells but also produce a broad array of cytokines and chemokines, polarize behavior of other immune cells and participate in the regulation of innate as well as adaptive arms of the immune system.Citation24 Immunoregulatory functions of B cells are being intensively investigated because of the potential impact of suppressive mechanisms these cells exert on immune responses in health and disease. The subset of regulatory B cells (Breg) remains relatively poorly characterized, and human Breg, in parallel with human Treg, seem to be phenotypically and functionally diverse.Citation18 This diversity and plasticity of regulatory lymphocyte populations, likely reflecting adaptation to the environmental context, complicate studies of mechanisms responsible for their regulatory functions.
It is well known that B cells utilize the ADO pathway. Co-expression of active ectonucleotidases, CD39 and CD73, in resting circulating B cells suggests they can hydrolyze eATP, yielding 5′-AMP and ADO.Citation14 We have recently reported that in vitro activation of human B cells with CD40L and IL-4 leads to significant upregulation of surface CD39 expression levels and of its enzymatic activity.Citation14 While CD73 surface expression was not measurably increased, ADO production by these activated B cells also increased and translated into upregulated immunosuppression of Teff functions.Citation14 It now appears that only a subset of in vitro-activated B cells which acquire especially high co-expression of CD39 and CD73 on the cell surface meet the phenotypic and functional criteria for Breg. These CD39high B cells are also CD24+, CD25high and CD38high. They are a proliferating population of B cells that produce IL-6 (a B-cell stimulatory factor). The high levels of 5′-AMP and ADO and moderate levels of IL-10 they produce are responsible, at least in part, for suppression of autologous CD4+ T cell proliferation we observed in co-cultures.
While a large proportion of in vitro-activated B cells are CD39+, less than 10% have the CD39high phenotype in 4-d cultures (). A large majority remains CD39low or CD39inter, although their conversion into CD39high B cells is clearly a possibility. Further, as CD39high B cells proliferate better than CD39inter B cells, their content might gradually increase, depending on culture conditions. Proliferation of this subset of CD39high B cells is favored because of the cytokines they produce, including IL-6, GM-CSF and TNF-α (). GM-CSF and IL-6 have been previously described to promote B-cell differentiation to the regulatory phenotype as also reported for IL-10.Citation25,26 Furthermore, the CD40L and IL-4 stimulation has been shown to promote transcription of the TNF-α gene and to increase B-cell growth.Citation27
It is reasonable to expect that as the proportion of these CD39high B cells increases, so do levels of 5′-AMP and ADO they produce. Indeed, ADO produced by CD39high B cells is likely to be responsible for suppression of activation and proliferation of CD4+ Teff in co-cultures of these lymphocyte populations (). Binding of Breg-derived ADO to A2AR on CD4+ Teff increases cAMP levels resulting in a loss of effector function, a mechanism that is also utilized by Treg as shown by us and others.Citation28 We suspected that 5′-AMP, especially when produced in excess by in vitro-activated B cells, might also contribute to suppression of Teff functions, signaling via the A1R receptor. However, neither Teff nor Treg proliferation in response to anti-CD3/CD28 Ab plus IL-2 stimulation was inhibited by exogenous 5′-AMP tested at a wide dose range in our hands (data not shown). In this study, suppressive effects of CD39high B cells on CD4+ Teff proliferation were shown to be mediated by A2AR, confirming that in human T cells, the A2AR functions as the main inhibitory receptor.Citation29
The A2AR is the most extensively studied ADOR in immune cells, and its involvement in ADO-mediated suppression of T lymphocytes has been well documented.Citation30,31 Less is known about ADO signaling via A2AR in B cells. We have previously shown that in vitro-activated B cells express A1R, A2AR and A3R but not A2BR.Citation14 Therefore, autocrine effects of 5′-AMP and ADO produced by these B cells deserve attention. Here, we considered the possibility that CD39/CD73-mediated hydrolysis of eATP to 5′-AMP and ADO might also promote expansion of CD39high Breg. Surprisingly, we observed that ZM241385, an A2AR antagonist, significantly decreased CD39 expression levels and suppressed proliferation of CD39high B cells, suggesting that ADO exerts growth-stimulatory rather than inhibitory autocrine effects in these cells. Similar effects of ADO were reported to occur in murine Treg,Citation32 supporting the above advanced scenario. Furthermore, CGS21680, an A2AR agonist, mildly increased activation and proliferation of CD39high B cells. Also, as shown in , ZM241385 (an A2AR antagonist) reversed the increases in the frequency of CD39high B cells induced by CGS21680 (an A2AR agonist). Thus, in CD39high Breg, the A2AR functions as a stimulatory receptor. This finding is in line with the emerging data which indicate that inhibitory and stimulatory signals are handled differently in regulatory lymphocytes such as Treg vs. Teff lymphocytes.Citation9 For example, usually inhibitory signaling via PD-1 is reported altered to stimulatory signaling in Treg found in environments rich in suppressive factors such as tumor microenvironments.Citation33,34 Our data suggest that the same mechanisms of “reverse” signaling apply to Breg.
Using pharmacologic inhibitors, we observed that in CD39high Breg, the A1R might also be involved in autocrine signaling. It has been recently reported that 5′-AMP can bind with a high affinity to A1R on HEK293 cells,Citation10 raising the question whether this nucleotide could deliver immunomodulatory signals to B cells. We observed that the treatment of in vitro-activated B cells with eATP plus AMPCP (a CD73 inhibitor) in order to prevent 5′-AMP utilization and increase its concentration in culture, did not have adverse effects on CD39high B-cell proliferation. In fact, in the presence of CCPA, an A1R agonist, the frequency of CD39high B cells was significantly increased (). These experiments suggested that either autocrine 5′-AMP or ADO signaling via A1R in the in vitro-activated B cells might lead to upregulation of CD39 expression and to expansion of CD39high B cells. Thus, in vitro-activated CD39high Breg, which produce elevated levels of 5′-AMP and ADO, might utilize the A1R for the autocrine upregulation of their proliferation. If so, then our in vitro experiments suggest that A1R, in addition to A2AR, functions as a stimulatory receptor in Breg. These results further strengthen the notion that activated regulatory T and B cells use “reverse signaling,” so that A1R and A2AR have the same rather than opposite biological effects.
The picture of a human CD39high Breg that emerges from this study presents an enzymatically active suppressor cell which vigorously produces 5′-AMP and ADO and uses the adenosinergic pathway to mediate suppression of Teff. At the same time, expression of ADOR enables Breg not only to survive in the immunosuppressive milieu but to use 5′-AMP and ADO to promote its functions, including expression of CD39 and proliferation. We have reported earlier that A3R also participates in autocrine ADO signaling in B cells: it is responsible for suppression of ADO generation in activated B cells, presumably downregulating their immunoinhibitory effects.Citation14 The balance between the various ADOR-mediated signals may be maintained by the ratio of 5′-AMP and ADO levels these cells produce as well as the levels of ATP available for enzymatic hydrolysis. This balance may be entirely dependent on the microenvironment in which Breg operate. Clearly, our in vitro experiments are intrinsically limited because they cannot mimic the TME. Nevertheless, they offer a potential view of how functions of regulatory immune cells are shaped by the environment.
Materials and methods
PBMC and cell separation
For a large-scale B-cell separation, buffy coats collected from normal donors were purchased from the Central Blood Bank of Pittsburgh. For smaller-scale experiments, venous blood was obtained from normal volunteers all of whom signed an informed consent approved by the University of Pittsburgh IRB (IRB # 991206).
CD19+ B cells were separated from PBMC using anti-CD19 Ab-coated magnetic beads (Miltenyi Biotec). Teff (CD4+CD39neg) were separated from PBMC by negative selection of CD4+ T cells followed by positive selection of CD39+ cells, using the Miltenyi reagents as previously described.Citation14 CD4+CD39neg cells were also collected. All separation procedures were performed according to the manufacturers’ instructions. B cells isolated from PBMC of healthy donors were routinely phenotyped by flow cytometry and found to have a purity of >90 %. After isolation from PBMC, CD19+ B cells were cultured in the presence of CD40L and IL-4 for 4 d as described below and sorted into CD39neg, CD39inter or CD39high cells using a Beckman Coulter cell sorter.
B-cell culture and sorting of B-cell subsets
Freshly separated CD19+ B cells were seeded in wells of six-well plates and cultured in RPMI containing 10% (v/v) fetal bovine serum (FBS), 4 mM L-glutamine, 100 U/mL penicillin and 100 mg/mL streptomycin (all from GIBCO). B cells were cultured in the presence of human CD40L (2 µg/mL; Cell Signaling) and IL-4 (200 IU/mL; Miltenyi Biotec) for 4 d at 37°C in the atmosphere of 5% CO2 in air. These cultured B cells are referred to as “in vitro-activated B cells.” After harvesting, in vitro-activated B cells were phenotyped for expression of CD39 and sorted into CD39neg, CD39inter and CD39high subsets. The sorted B cells were examined for expression of surface or intracytoplasmic markers and functional attributes as described below.
Surface staining for flow cytometry
Cultured unsorted and in vitro-activated sorted B cells (CD39neg, CD39inter and CD39high) were suspended in the staining buffer (PBS + 1% FBS + 0.09% sodium azide) and stained with labeled monoclonal antibodies (mAbs) specific for surface antigens: CD20-APC770 (Miltenyi Biotec), CD24-EF450 (eBioscience), CD25-PC5 (Beckman Coulter), CD38-FITC (eBioscience), CD39-PC7 (eBioscience), CD73-PE (BioLegend), and respective isotype-specific Abs. Briefly, B cells were incubated with mAbs for 25 min in the dark, washed twice in the PBS buffer and then analyzed by flow cytometry using a Gallios 10-color cytometer and a Kaluza Flow Cytometry Software (Beckman Coulter).
IL-10 secretion by B cells
In vitro-activated B cells were harvested by centrifugation (400rpm for 6 min), washed in cold buffer tested for IL-10 secretion using the kit purchased from Miltenyi Biotec. Cells were re-suspended in 80 µL of cold buffer plus 20 µL of the IL-10 capture reagent as recommended by the manufacturer. After 5 min of incubation on ice, 2 mL of warm medium was added and the cells were incubated for 45 min at 37°C. Following washing with cold buffer, anti-IL10-PE mAbs (or isotype control Abs), anti-CD39-PC7 and anti-CD20-APC770 Abs were added to the cells and incubated for 20 min in the dark. After washing, the cells were phenotyped by flow cytometry, acquiring one-million cells per sample. The frequency of IL-10 secreting B cells in the different B-cell subsets (CD39neg, CD39inter and CD39high) was measured as recommended by the kit manufacturer, using resting B cells as a reference. The data were analyzed by Kaluza Flow Cytometry Software→ (Beckman Coulter).
Proliferation assays
Proliferation of either B cells or Teff was measured in Ki-67-based assays using the “one-step protocol for intracellular (nuclear) proteins” (eBioscience). Briefly, sorted B cells were cultured alone in the presence of CD40L or IL-4 or were co-incubated with autologous Teff (see below) for 72 h. Teff were activated with anti-CD3/CD28 Abs on beads (2.25:10/beads:cells, Miltenyi Biotec) and IL-2 (225 IU/mL). After culture, cells were stained for surface proteins, washed and permeabilized using the Fixation/Permeabilization buffer supplied by eBioscience for 40 min at 4°C in the dark. Next, the cells were incubated with anti-Ki67 Abs (eBioscience) or the isotype control Ab mixed at the 1:1 ratio with the permeabilization buffer for 30 min at room temperature (RT) in the dark. Cells were washed twice and immediately acquired for flow cytometry. Autologous resting B cells or resting T cells were used as negative controls. In some experiments, in vitro-activated B cells were incubated in the presence of 20 µM ATP or ATP plus AMPCP (CD73 inhibitor, 10 µM). Alternatively, ADO agonists and antagonists (all used at 1 µM, as previously describedCitation14) were added to in vitro-activated proliferating B cells for 4 d, and Ki-67 expression was measured by flow cytometry.
Co-incubation assays
Various subsets of in vitro-activated B cells (CD39neg, CD39inter and CD39high) were co-cultured with autologous Teff (CD4+CD39neg) at the 1:1 ratio in the presence of anti-CD3/CD28 Abs and IL-2 (at the concentrations specified above) for 24 h. Autologous T cells were maintained in culture prior to co-incubation assays. At the end of T cell–B cell co-cultures, cells were washed and incubated with mAbs specific for CD69-FITC, CD39-PC7, CD20-APC770 and CD4-EF450 or the relevant isotype controls for 25 min in the dark, as described above. Suppression of Teff proliferation by in vitro-activated B cells was evaluated relative to proliferation of activated Teff alone using Kaluza→ Flow Cytometry Software (Beckman Coulter).
Cytokine measurements
Cytokine production by the sorted B-cell subsets, CD39neg, CD39inter and CD39high alone or after their co-culture with autologous CD4+CD39neg T cells at the 1:1 ratio for 24 h was measured using Luminex. Supernatants were collected and the cytokine content was measured using a 10-plex panel, according to the manufacturer’s instructions (Life Technologies/Invitrogen).
Agonists and antagonists of ADORs
The following ADOR antagonists were purchased from Tocris Bioscience: PSB-36, an A1R antagonist and PSB-10, an A3R antagonist. Also, ZM241385, an A2AR antagonist, was purchased from Sigma-Aldrich. The following ADOR agonists were purchased from Tocris Bioscience: 2-chloro-N6-cyclopentyladenosine, an A1R agonist; CGS21680 hydrochloride, an A2AR agonist; and 2-Cl-IB-MECA, an A3R agonist.
Mass spectrometry
Sorted in vitro-activated B cells (CD39neg, CD39inter and CD39high subsets) were isolated from PBMC as described above. For detection of 5′-AMP and ADO production, 25,000 B cells were incubated in 200 µL PBS in 96-well plates in the presence and absence of anti-CD39 blocking Ab (generously provided by Orega, France) or IgG1 isotype control for 2 h. Then the three subsets of in vitro-activated CD39+ B cells were incubated with 20 µM ATP for 45 min. Control wells contained B cells alone or ATP alone. All experiments were performed in duplicate. Cells and supernatants were collected, centrifuged twice and boiled for 5 min to inactivate ADO-degrading enzymes, and stored at −80°C for subsequent analyses. Purines were measured using liquid chromatography-tandem mass spectrometry by selected reaction monitoring with 13C10-ADO as the internal standard. Samples were injected into an Acuity ultra-performance liquid chromatographic system (Waters) and were separated on a C18 column (Waters UPLC BEH C18; 1.7 micron; 2.1 × 100 mm) using the following elution conditions: mobile phase A, 1% acetic acid in H2O; mobile phase B, methanol; flow rate, 0.3 mL/min; elution gradient (A/B) was 99.5%/0.5% (0 to 2 minutes), 98%/2% (2 to 3 min), 85%/15% (3 to 4 min), and 99.5%/0.5% (4 to 5 min). Purine levels were analyzed with a TSQ Quantum-Ultra triple quadrupole mass spectrometry equipped with a heated electrospray ionization source. The mass spectrometer was operated in the positive-ion mode and the following mass-to-charge transitions were monitored: 348→136 for 5′-AMP; 268→136 for ADO.
Statistical analysis
The data were analyzed by one-way analysis of variance (ANOVA) followed by a post-hoc test for multiple comparisons (Tukey test) using GraphPad Prism software. The data are expressed as the mean ± S.E.M. Differences were considered significant at p < 0.05.
Disclosure of Potential Conflicts of Interest
No potential conflicts of interest were disclosed.
Funding
The study was in part supported by NIH grants R0-1 CA 1168628 to TLW and NIH grants NS087978, HL109002, DK091190, HL069846, DK068575 and DK079307 to EKJ. F.F. was supported by a fellowship from PDSE/CAPES (Brazil). This project used the expert assistance of the UPCI Flow Cytometry Facility and was supported in part by award P30 CA047904.
References
- Antonioli L, Blandizzi C, Pacher P, Hasko G. Immunity, inflammation and cancer: a leading role for adenosine. Nat Rev Cancer 2013; 13:842-57; PMID:24226193; http://dx.doi.org/10.1038/nrc3613
- Yegutkin GG. Enzymes involved in metabolism of extracellular nucleotides and nucleosides: functional implications and measurement of activities. Crit Rev Biochem Mol Biol 2014; 49:473-97; PMID:25418535; http://dx.doi.org/10.3109/10409238.2014.953627
- Beavis PA, Stagg J, Darcy PK, Smyth MJ. CD73: a potent suppressor of antitumor immune responses. Trends Immunol 2012; 33:231-7; PMID:22487321; http://dx.doi.org/10.1016/j.it.2012.02.009
- Linden J, Cekic C. Regulation of lymphocyte function by adenosine. Arterioscler Thromb Vasc Biol 2012; 32:2097-103; PMID:22772752; http://dx.doi.org/10.1161/ATVBAHA.111.226837
- Burnstock G, Boeynaems JM. Purinergic signalling and immune cells. Purinergic Signal 2014; 10:529-64; PMID:25352330; http://dx.doi.org/10.1007/s11302-014-9427-2
- Sitkovsky MV. T regulatory cells: hypoxia-adenosinergic suppression and re-direction of the immune response. Trends Immunol 2009; 30:102-8; PMID:19201652; http://dx.doi.org/10.1016/j.it.2008.12.002
- Bopp T, Becker C, Klein M, Klein-Hessling S, Palmetshofer A, Serfling E, Heib V, Becker M, Kubach J, Schmitt S et al. Cyclic adenosine monophosphate is a key component of regulatory T cell-mediated suppression. J Exp Med 2007; 204:1303-10; PMID:17502663; http://dx.doi.org/10.1084/jem.20062129
- Cekic C, Day YJ, Sag D, Linden J. Myeloid expression of adenosine A2A receptor suppresses T and NK cell responses in the solid tumor microenvironment. Cancer Res 2014; 74:7250-9; PMID:25377469; http://dx.doi.org/10.1158/0008-5472.CAN-13-3583
- Ohta A, Sitkovsky M. Extracellular adenosine-mediated modulation of regulatory T cells. Front Immunol 2014; 5:304; PMID:25071765; http://dx.doi.org/10.3389/fimmu.2014.00304
- Rittiner JE, Korboukh I, Hull-Ryde EA, Jin J, Janzen WP, Frye SV, Zylka MJ. AMP is an adenosine A1 receptor agonist. J Biol Chem 2012; 287:5301-9; PMID:22215671; http://dx.doi.org/10.1074/jbc.M111.291666
- Mandapathil M, Szczepanski MJ, Szajnik M, Ren J, Lenzner DE, Jackson EK, Gorelik E, Lang S, Johnson JT, Whiteside TL. Increased ectonucleotidase expression and activity in regulatory T cells of patients with head and neck cancer. Clin Cancer Res 2009; 15:6348-57; PMID:19825957; http://dx.doi.org/10.1158/1078-0432.CCR-09-1143
- Whiteside TL, Mandapathil M, Schuler P. The role of the adenosinergic pathway in immunosuppression mediated by human regulatory T cells (Treg). Curr Med Chem 2011; 18:5217-23; PMID:22087822; http://dx.doi.org/10.2174/092986711798184334
- Kessel A, Haj T, Peri R, Snir A, Melamed D, Sabo E, Toubi E. Human CD19(+)CD25(high) B regulatory cells suppress proliferation of CD4(+) T cells and enhance Foxp3 and CTLA-4 expression in T-regulatory cells. Autoimmun Rev 2012; 11:670-7; PMID:22155204; http://dx.doi.org/10.1016/j.autrev.2011.11.018
- Saze Z, Schuler PJ, Hong CS, Cheng D, Jackson EK, Whiteside TL. Adenosine production by human B cells and B cell-mediated suppression of activated T cells. Blood 2013; 122:9-18; PMID:23678003; http://dx.doi.org/10.1182/blood-2013-02-482406
- Zhang X. Regulatory functions of innate-like B cells. Cell Mol Immunol 2013; 10:113-21; PMID:23396472; http://dx.doi.org/10.1038/cmi.2012.63
- de Andres C, Tejera-Alhambra M, Alonso B, Valor L, Teijeiro R, Ramos-Medina R, Mateos D, Faure F, Sanchez-Ramon S. New regulatory CD19(+)CD25(+) B-cell subset in clinically isolated syndrome and multiple sclerosis relapse. Changes after glucocorticoids. J Neuroimmunol 2014; 270:37-44; PMID:24662004; http://dx.doi.org/10.1016/j.jneuroim.2014.02.003
- Blair PA, Norena LY, Flores-Borja F, Rawlings DJ, Isenberg DA, Ehrenstein MR, Mauri C. CD19(+)CD24(hi)CD38(hi) B cells exhibit regulatory capacity in healthy individuals but are functionally impaired in systemic Lupus Erythematosus patients. Immunity 2010; 32:129-40; PMID:20079667; http://dx.doi.org/10.1016/j.immuni.2009.11.009
- Rosser EC, Mauri C. Regulatory B cells: Origin, phenotype, and function. Immunity 2015; 42:607-12; PMID:25902480; http://dx.doi.org/10.1016/j.immuni.2015.04.005
- Yang M, Rui K, Wang S, Lu L. Regulatory B cells in autoimmune diseases. Cell Mol Immunol 2013; 10:122-32; PMID:23292280; http://dx.doi.org/10.1038/cmi.2012.60
- Kumar V. Adenosine as an endogenous immunoregulator in cancer pathogenesis: where to go? Purinergic Signal 2013; 9:145-65; PMID:23271562; http://dx.doi.org/10.1007/s11302-012-9349-9
- Shao Y, Lo CM, Ling CC, Liu XB, Ng KT, Chu AC, Ma YY, Li CX, Fan ST, Man K. Regulatory B cells accelerate hepatocellular carcinoma progression via CD40/CD154 signaling pathway. Cancer Lett 2014; 355:264-72; PMID:25301451; http://dx.doi.org/10.1016/j.canlet.2014.09.026
- Stanic B, van de Veen W, Wirz OF, Ruckert B, Morita H, Sollner S, Akdis CA, Akdis M. IL-10-overexpressing B cells regulate innate and adaptive immune responses. J Allergy Clin Immunol 2015; 135:771-80.e8; PMID:25240783; http://dx.doi.org/10.1016/j.jaci.2014.07.041
- Rincon-Arevalo H, Sanchez-Parra CC, Castano D, Yassin L, Vasquez G. Regulatory B cells and mechanisms. Int Rev Immunol 2015; PMID:25793964; http://dx.doi.org/10.3109/08830185.2015.1015719
- Fillatreau S. Regulatory plasma cells. Curr Opin Pharmacol 2015; 23:1-5; PMID:25978519; http://dx.doi.org/10.1016/j.coph.2015.04.006
- Rosser EC, Oleinika K, Tonon S, Doyle R, Bosma A, Carter NA, Harris KA, Jones SA, Klein N, Mauri C. Regulatory B cells are induced by gut microbiota-driven interleukin-1beta and interleukin-6 production. Nat Med 2014; 20:1334-9; PMID:25326801; http://dx.doi.org/10.1038/nm.3680
- Deng J, Galipeau J. Reprogramming of B cells into regulatory cells with engineered fusokines. Infect Disord Drug Targets 2012; 12:248-54; PMID:22394176; http://dx.doi.org/10.2174/187152612800564392
- Boussiotis VA, Nadler LM, Strominger JL, Goldfeld AE. Tumor necrosis factor alpha is an autocrine growth factor for normal human B cells. Proc Natl Acad Sci U S A 1994; 91:7007-11; PMID:7518925; http://dx.doi.org/10.1073/pnas.91.15.7007
- Mandapathil M, Hilldorfer B, Szczepanski MJ, Czystowska M, Szajnik M, Ren J, Lang S, Jackson EK, Gorelik E, Whiteside TL. Generation and accumulation of immunosuppressive adenosine by human CD4+CD25highFOXP3+ regulatory T cells. J Biol Chem 2010; 285:7176-86; PMID:19858205; http://dx.doi.org/10.1074/jbc.M109.047423
- Huang S, Apasov S, Koshiba M, Sitkovsky M. Role of A2a extracellular adenosine receptor-mediated signaling in adenosine-mediated inhibition of T-cell activation and expansion. Blood 1997; 90:1600-10; PMID:9269779
- Sitkovsky M, Lukashev D, Deaglio S, Dwyer K, Robson SC, Ohta A. Adenosine A2A receptor antagonists: blockade of adenosinergic effects and T regulatory cells. Br J Pharmacol 2008; 153(Suppl 1):S457-64; PMID:18311159; http://dx.doi.org/10.1038/bjb.2008.23
- Muller-Haegele S, Muller L, Whiteside TL. Immunoregulatory activity of adenosine and its role in human cancer progression. Expert Rev Clin Immunol 2014; 10:897-914; PMID:24871693; http://dx.doi.org/10.1586/1744666X.2014.915739
- Ohta A, Kini R, Subramanian M, Madasu M, Sitkovsky M. The development and immunosuppressive functions of CD4(+) CD25(+) FoxP3(+) regulatory T cells are under influence of the adenosine-A2A adenosine receptor pathway. Front Immunol 2012; 3:190; PMID:22783261; http://dx.doi.org/10.3389/fimmu.2012.00190
- Zhou Q, Munger ME, Highfill SL, Tolar J, Weigel BJ, Riddle M, Sharpe AH, Vallera DA, Azuma M, Levine BL et al. Program death-1 signaling and regulatory T cells collaborate to resist the function of adoptively transferred cytotoxic T lymphocytes in advanced acute myeloid leukemia. Blood 2010; 116:2484-93; PMID:20570856; http://dx.doi.org/10.1182/blood-2010-03-275446
- Francisco LM, Salinas VH, Brown KE, Vanguri VK, Freeman GJ, Kuchroo VK, Sharpe AH. PD-L1 regulates the development, maintenance, and function of indued regulatory T cells. J Exp Med 2009; 206:3015-29