ABSTRACT
Background: Radiotherapy (RT) is a mainstay for the treatment of lung cancer, but the effective dose is often limited by the development of radiation-induced pneumonitis and pulmonary fibrosis. Transforming growth factor β (TGFβ) and platelet-derived growth factor (PDGF) play crucial roles in the development of these diseases, but the effects of dual growth factor inhibition on pulmonary fibrosis development remain unclear. Methods: C57BL/6 mice were treated with 20 Gy to the thorax to induce pulmonary fibrosis. PDGF receptor inhibitors SU9518 and SU14816 (imatinib) and TGFβ receptor inhibitor galunisertib were applied individually or in combinations after RT. Lung density and septal fibrosis were measured by high-resolution CT and MRI. Lung histology and gene expression analyses were performed and Osteopontin levels were studied. Results: Treatment with SU9518, SU14816 or galunisertib individually attenuated radiation-induced pulmonary inflammation and fibrosis and decreased radiological and histological signs of lung damage. Combining PDGF and TGFβ inhibitors showed to be feasible and safe in a mouse model, and dual inhibition significantly attenuated radiation-induced lung damage and extended mouse survival compared to blockage of either pathway alone. Gene expression analysis of irradiated lung tissue showed upregulation of PDGF and TGFβ-dependent signaling components by thoracic irradiation, and upregulation patterns show crosstalk between downstream mediators of the PDGF and TGFβ pathways. Conclusion: Combined small-molecule inhibition of PDGF and TGFβ signaling is a safe and effective treatment for radiation-induced pulmonary inflammation and fibrosis in mice and may offer a novel approach for treatment of fibrotic lung diseases in humans. Translational statement: RT is an effective treatment modality for cancer with limitations due to acute and chronic toxicities, where TGFβ and PDGF play a key role. Here, we show that a combined inhibition of TGFβ and PDGF signaling is more effective in attenuating radiation-induced lung damage compared to blocking either pathway alone. We used the TGFβ-receptor I inhibitor galunisertib, an effective anticancer compound in preclinical models and the PDGFR inhibitors imatinib and SU9518, a sunitinib analog. Our signaling data suggest that the reduction of TGFβ and PDGF signaling and the attenuation of SPP1 (Osteopontin) expression may be responsible for the observed benefits. With the clinical availability of similar compounds currently in phase-I/II trials as cancer therapeutics or already approved for certain cancers or idiopathic lung fibrosis (IPF), our study suggests that the combined application of small molecule inhibitors of TGFβ and PDGF signaling may offer a promising approach to treat radiation-associated toxicity in RT of lung cancer.
Introduction
RT is one of the mainstays in the treatment of lung cancer. However, delivery of high doses to the tumor is often hampered by the risk of inducing radiation-induced lung injury (RILI). Lung damage due to thoracic radiation comprises acute responses like inflammation and pneumonitis as well as chronic effects such as pulmonary fibrosis.Citation1-4 Despite the increasing use of highly conformal RT techniques, up to 15% of patients develop pneumonitis and consecutively pulmonary fibrosis after chest irradiation.Citation5,6 The exact molecular mechanisms leading to the development of radiation-induced pulmonary fibrosis have not yet been fully identified, but may involve the production of release of pro-inflammatory and proliferative signaling molecules by the affected tissue, resulting in fibroblast replication, invasion of inflammatory cells and deposition of excess extracellular matrix.Citation7,8 Clinical signs of progressive pulmonary fibrosis include increasing dyspnea, deteriorating lung function and accumulation of interstitial fluid, eventually leading to respiratory failure. While steroids and other forms of anti-inflammatory therapy have been established to control acute pulmonary inflammation, no medical therapy for pulmonary fibrosis has been approved for routine clinical use despite the apparent need for an effective treatment.Citation9
Several studies using animal models of radiation-induced pulmonary fibrosis have presented evidence for the involvement of specific growth factors in the pathogenesis of this disease. The PDGF pathway has come into focus as it may be involved in the downstream signaling of various known fibrogenic mediators such as TGFβ, tumor necrosis factor α (TNFα) and interleukin 1.Citation2,10,11 The PDGF family contains a group of disulfide-bonded homodimers or heterodimers with four potential subunits (PDGF-A, PDGF-B, PDGF-C, PDGF-D) that exert their effects on PDGF receptor (PDGFR) tyrosine kinases α and β.Citation12 Inhibition of PDGF-PDGFR signaling in irradiated lung tissue has shown significant improvement of survival and pulmonary fibrosis in animal studies.Citation2,13
Recent evidence also suggests an involvement of TGFβ in the development of pulmonary fibrosis, and inhibition of the TGFβ receptor (TGFβR) has shown promising results regarding the attenuation of this disease.Citation14-17 The three distinct TGFβ receptor types identified to date (TGFβR I, TGFβR II, TGFβR III) of which TGFβR I and TGFβR II have a dimeric serine/threonine kinases that are activated by the various proteins of the TGFβ superfamily and may be useful targets for an anti-fibrotic treatment in RILI.Citation18
In this study, we investigated the effects of inhibition of the PDGF and TGFβ pathways on the development of radiation-induced pulmonary fibrosis. We used the oral TGFβR I inhibitor galunisertib (LY2157299 monohydrate),Citation19 alone or in combination with small-molecule PDGF inhibitors SU9518 and SU14816 (imatinib) in a murine model of RILI and pulmonary fibrosis. Post-irradiation survival and weight loss were assessed for 6 mo, and non-invasive radiological lung imaging by CT and MRI was performed after a single thoracic RT fraction of 20 Gy. Additionally, histology and gene expression patterns of irradiated lung tissue were analyzed to assess a potential benefit of dual pathway inhibition for the attenuation of RILI and pulmonary fibrosis.
Materials and methods
Animal model and treatment protocol
Female fibrosis prone C57BL/6N mice were ordered from Charles River Laboratories (Sulzfeld, Germany). For thoracic RT, animals were anesthetized and treated with a single fraction of 20 Gy using a linear accelerator at a dose rate of 3 Gy/min. All extra-thoracic organs were excluded from the radiation field.
Consecutive drug treatment was administered orally. The selective TGFβRI inhibitor galunisertib (LY2157299 monohydrate, plasma half life < 24 hCitation20) was kindly provided by Lilly (Indianapolis, USA) and was given twice daily per oral gavage for a duration of 4 weeks. The PDGF receptor inhibitor SU9518 and the imatinib analog SU14816 (plasma half lives of ∼76 h) were kindly supplied by SUGEN (San Francisco, USA) and were given twice weekly per oral gavage until the end of the observation period in order to achieve and maintain a clinically relevant dose as determined by previous studies.Citation2,14 Drug treatment started 2 weeks after thoracic irradiation when acute inflammation had subsided as described earlier. Animals were followed as described below until 24 weeks after irradiation, and lung imaging as well as body weight analysis was performed regularly during this time course as indicated in . A total of 170 mice in 12 groups were used. There were six unirradiated control groups (untreated, LY, IM, SU, LY + IM, LY + SU; n = 8–10 mice each) and six irradiated groups (RT, RT + LY, RT + IM, RT + SU, RT + LY + IM, RT + LY + SU, n = 18–20 mice each). In all studies, animal care and use were done in accordance with federal and local laws, policies, regulations, and standards in effect at the time of their conduct, e.g., Animal Welfare Act and Regulations. All study protocols were approved in advance by the respective institutional and governmental animal protection authorities.
Figure 1. Combined PDGF and TGFβ signaling inhibition improves mouse survival and attenuates weight loss. (A) Schematic depiction of the mouse treatment algorithm up to week 24. (B) Kaplan–Meier curve showing the survival probability of mice treated with radiotherapy (RT) either alone or in combination with galunisertib (LY2157299 monohydrateor LY), imatinib (IM) or SU9518 (SU). Statistical analysis performed by log-rank test. (C) Changes in animal body weight after irradiation and treatment with galunisertib, imatinib or SU9518. Graphs depict mean values +/− SD. Statistical analysis performed by paired Student's t-test; *p < 0.05.
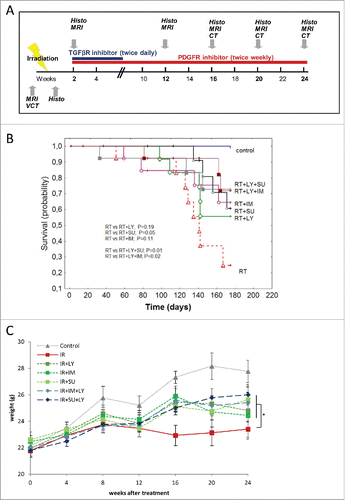
High resolution CT of mouse lungs
Two mice of each treatment group were randomly selected for lung density imaging using high resolution CT (HR-CT). HR-CT images were taken at weeks 16, 20 and 24 after irradiation on a Siemens Somatom Plus four multi-slice CT scanner (Erlangen, Germany) using an inter-slice distance of 0.5 mm.
Lung density was expressed in Hounsfield units (HU). Slices at the level of the tracheal bifurcation and maximum cardiac diameter were chosen for analysis, and four regions of interest (ROIs) were defined in each selected slice: right and left anterior, right and left posterior, resulting in eight individual density values per mouse lung. The total arithmetic mean ± standard deviation (SD) was calculated and used as a quantitative parameter for lung density and fibrosis. For all imaging examinations, mice were anesthetized with inhalative isoflurane.
Magnetic resonance imaging of mouse lungs
Magnetic resonance imaging (MRI) scans of mouse lungs were conducted using the same two randomly selected mice from each group that were also used for CT scanning. Images were taken 1 week before and 2, 12, 16, 20 and 24 weeks after irradiation using a Siemens Magnetom Symphony Syngo MR 2004A scanner (1.5 Tesla). T2-weighted images were used to gather further information on increased lung density as they have the potential differentiate between pulmonary fibrosis and edema. Given the high breathing frequency of mice, no gating or motion correction has been performed resulting in specific motion artifacts, resulting in slightly limited image quality.
Lung histology
For histological analysis, two mice were euthanized after 2 d as well as 2, 12, 16, 20 and 24 weeks after thoracic RT. Lung tissue was fixed by intratracheal instillation of 4% formalin in PBS and then embedded in paraffin and sectioned at 5 µm, before staining with hematoxylin-eosin (H&E), Masson's trichrome and Sirius red. Inflammatory cells were counted in 10 randomly chosen slides from each sample, and septal thickness was analyzed morphometrically. Osteopontin was detected using a specific monoclonal antibody (Abcam, ab69498). Visualization was carried out using biotinylated secondary antibodies followed by an avidin-biotin peroxidase complex (Vectastain, PK6100) and 3, 3′-diaminobenzidine as a chromogen (Vectastain, PK6100). Images were captured using a Nikon Eclipse E600 microscope equipped with a Nikon digital sight DS-U1 (Nikon, Chiyoda, Tokyo, Japan).
Gene expression profiles of lung tissue
Pulmonary gene expression was analyzed at 24 weeks after irradiation to assess the effects of RT and PDGF or TGFβ pathway inhibition on mouse lungs. RNA was extracted from treated animals' lung tissue; it was labeled using an Agilent One Color RNA Spike-In kit (Agilent Technologies, Wilmington, USA) and consecutively purified with a NucleoSpinR RNA L kit (Macherey-Nagel, Düren, Germany). Gene expression profiles were then acquired with a whole human genome microarray 4 × 44 k (#G4112F, Agilent Technologies). Hybridization signals were detected on an Agilent Microarray Scanner and data extracted using Agilent feature extraction software (Agilent, Version 9.1). Expression data were analyzed using SUMO software (SUMO — Statistical Utility for Micro array and Omics data; http://angiogenesis.dkfz.de/oncoexpress/software/sumo) using ANOVA with Bonferroni correction. Genes were considered regulated (normalized vs. untreated controls) if substantially (>2-fold) and significantly (p < 0.05) regulated. The gene array data have been submitted to the GEO repository and the accession number is GSE65011.
Statistical analysis
Mouse survival curves were calculated using the Kaplan–Meier method, and log-rank tests were used to assess statistical differences between treatment groups. All quantitative values are given as mean values ± SD. Two-tailed t-tests were used for parametric comparisons between two groups, and the Kruskall–Wallis test for ordinal data (Statistica 6.0). p < 0.05 was considered statistically significant.
Results
PDGF and TGFβ inhibition improve survival after thoracic irradiation
Mice were treated with a single radiation fraction of 20 Gy to the thorax, resulting in a significant reduction of survival compared to untreated animals (p < 0.01, log-rank test) (). Addition of galunisertib or SU14816 to the treatment schedule led to a small but non-significant increase in mouse overall survival compared to irradiation alone (p = 0.19 for galunisertib, p = 0.11 for SU14816, log-rank test). PDGF inhibition using SU9518 significantly attenuated the observed reduction in survival especially during the early period up to 16 weeks after radiation treatment (p < 0.05, log-rank test). Combined inhibition of PDGF and TGFβ signaling through a combination of galunisertib + SU14816 as well as galunisertib + SU9518 after RT significantly improved mouse survival rates in comparison to sole IR treatment (p < 0.05 for both combinations, log-rank test).
The adverse effects of thoracic irradiation in mice were also reflected in increased weight loss after treatment (). While untreated mice had 6 g of weight gain on average, irradiated animals gained less than 2 g of body weight during the 24 weeks follow-up period. Weight loss was attenuated when either PDGF or TGFβ signaling was inhibited after RT (p < 0.05, Student's t-test). In contrast to the effects seen for mouse survival, a combined inhibition of both pathways did not lead to an additional improvement in body weight compared to single-agent inhibition of either pathway (p < 0.05).
HRCT measurement of lung density after thoracic irradiation
High resolution computed tomography (HRCT) examinations were carried out at 16, 20 and 24 weeks after irradiation to assess morphologic changes in lung density caused by thoracic IR treatment. After 16 weeks, typical radiological features of lung fibrosis were observed in irradiated mice, including irregular septal thickening, patchy reticular abnormalities with intralobular opacities and honeycombing (). At weeks 20 and 24, these radiological signs of fibrosis became even more prominent, suggesting a progressive course of fibrosis development after irradiation. Single-agent treatment with either PDGFR inhibitors SU14816 or SU9518 or TGFβR inhibitor galunisertib as well as combination treatments markedly reduced the radiological signs of fibrogenesis for all analyzed time points after IR treatment. Additionally, a quantitative assessment of lung density (quantified in HU) was carried out as a measure for pulmonary fibrosis (). While the RT only group showed strongly increased density values compared to the control group for all measured time points, both single-agent and combined inhibition of PDGF and TGFβ signaling markedly attenuated the increase in lung density induced by thoracic RT (p < 0.05 for all inhibitors, Student's t-test). However, the limited resolution of HRCT analysis did not allow a statistically significant distinction between the individual efficiencies of the different agents with regard to average lung density. Treatment with the inhibitors without irradiation did only transiently result in a slightly increased pulmonary density at week 20 (Fig. S1).
Figure 2. PDGF and TGFβ signaling inhibition reduce radiological signs of pulmonary fibrosis after irradiation. (A) Sample CT images of mouse thoraces at 16, 20 and 24 weeks after irradiation and treatment with galunisertib (LY), imatinib (IM) or SU9518 (SU). Black arrows indicate areas of increased lung density. (B) Quantification of mouse pulmonary density at three different time points after treatment with ionizing radiation and PDGF or TGFβ inhibitors. Average density was measured in Hounsfield units from four standardized regions of interest at the level of the tracheal bifurcation. Graphs depict mean values +/− standard deviation. Statistical analysis was performed by 2-tailed Student's t-test. *p < 0.05; ***p < 0.001. (C) T1-weighted FLASH MRI images of mouse thoraces taken at 16, 20 and 24 weeks after irradiation and treatment with galunisertib (LY), imatinib (IM) or SU9518 (SU).
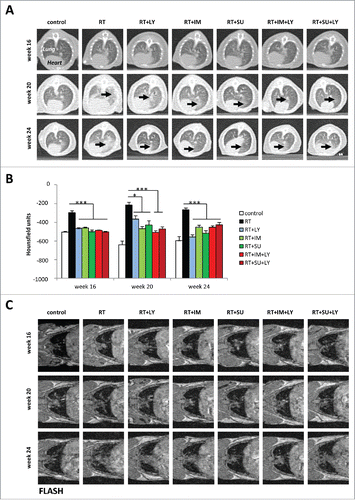
MRI measurement of lung density after thoracic irradiation
MRI was performed to further characterize the increased lung density observed after thoracic irradiation. In mice treated only with irradiation, T1-weighted MRI images revealed hyperintense signals that were first detected at week 12 and covered the whole lung parenchyma by week 24, suggesting increased tissue density due to pulmonary fibrosis (). Mice treated with either SU14816 or SU9518 or galunisertib after irradiation exhibited predominantly physiological lung tissue comparable to that of untreated controls; only discreet hyperintense signals were detected in peripheral lung sections towards the end of the observation period, indicating the onset of mild pulmonary fibrosis. Lungs from animals treated with a combination of PDGFR and TGFβR inhibitors showed no signs of pathological alterations in tissue architecture during the complete observation period. T2-weighted images did not reveal any pulmonary edema or pleural effusion as a reaction of irradiated lungs to IR treatment at any observation point (Fig. S2).
PDGF and TGFβ inhibition attenuate radiation-induced septal fibrosis development
Pulmonary septal thickness was analyzed in irradiated mouse lungs to quantify the morphological and functional changes during the course of radiation-induced fibrogenesis and to assess potential beneficial effects of PDGF and TGFβ inhibition.
Septal thickness began to increase at 12 weeks after RT and was shown to further increase during the observation period (). At 24 weeks after thoracic irradiation, septal thickness in the RT group was severely elevated, corresponding to a strong increase in interstitial collagen fiber deposition as assessed by Sirius red staining (, lower panel). Treatment of mice with PDGF inhibitors SU14816 or SU9518 or TGFβ inhibitor galunisertib after irradiation markedly reduced pulmonary septal thickness at all tested time points (, p < 0.001, Student's t-test). The combination of PDGF and TGFβ signaling inhibition resulted in an incremental, significant attenuation of pulmonary fibrosis compared to single-agent treatment. In such conditions, septal diameters were similar to untreated control at weeks 20 and 24 (p < 0.001, Student's t-test).
Figure 3. Combined PDGF and TGFβ signaling inhibition reduce radiation-induced thickening of pulmonary septa and invasion of inflammatory cells. (A) Sample images of treated mouse lungs stained with hematoxylin-eosin, Masson's trichrome and Sirius red. (B) Average thickness of mouse pulmonary septa after irradiation and treatment with galunisertib (LY), imatinib (IM) or SU9518 (SU). (C) Quantification of leukocyte infiltration of irradiated mouse lungs. Graphs depict mean values +/− SD. Statistical analysis was performed by Student's t-test; ***p < 0.001.
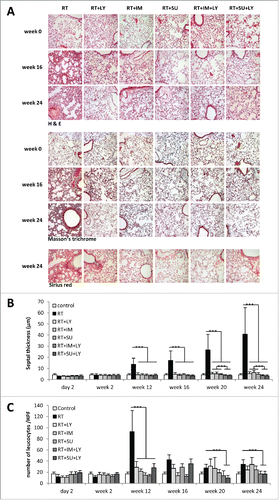
In addition, leukocyte infiltration was quantified to assess the inflammatory component of radiation-induced pulmonary fibrosis development (). At 12 weeks after RT, the observed onset of septal fibrosis development was accompanied by a peak in leukocyte infiltration. Between weeks 16 and 24, leukocyte counts were decreasing, but without reaching baseline levels. Treatment of irradiated animals with SU14816, SU9518 or galunisertib resulted in a massive reduction of leukocyte infiltration at week 12 (p < 0.001 for all treatment groups, Student's t-test), and continued to show smaller effects on leukocyte counts during the extended observation period. At later time points between 20 and 24 weeks, combined PDGFR and TGFβR inhibition showed a significant decrease of leukocytes compared to irradiated tissue, while single agent treatment with either PDGFR or TGFβR inhibitors resulted in non-significant changes in leukocyte invasion compared to irradiated mice.
H&E staining of mouse lungs at week 12 after RT demonstrated numerous alveolar macrophages containing cellular debris as well as formations of fibroblast foci in the alveolar interstitium (). Up to week 24, the observed interstitial fibroblast foci expanded and coalesced into large, cell-dense areas. Masson's trichrome and Sirius red stainings revealed vast areas of fibrotic lesions by week 24 after thoracic RT. Treatment of irradiated mice with PDGF or TGFβ inhibitors resulted in a strong reduction of fibroblast invasion, collagen deposition and other histologically visible signs of tissue fibrosis, while these drugs did not have any effect on lung architecture in unirradiated animals (Fig. S3). The observed reduction in fibrotic changes was even more pronounced when PDGF and TGFβ signaling were simultaneously blocked.
PDGF and TGFβ inhibition modify radiation-induced expression of fibrogenic genes
Gene expression profiles were analyzed using mouse lung tissue harvested at week 24. Using a critical p value of 0.002 with Bonferroni correction for the 1 factor ANOVA a number of 225 significantly regulated genes were detected and clustered in a heatmap resulting in three major clusters (). Cluster a contains a large group of genes affected by the different combinations of drug treatment (PDGFR-inhibitors Imatinib or SU and the TGFBR inhibitor galunisertib) and not influenced by radiation. The small group of genes in cluster b were upregulated by radiation and normalized or inhibited by drug treatments including SPP1 (Osteopontin, see fig B and ) which may be associated with the antifibrotic drug effects. Cluster c contains genes which were mainly regulated by the combination of irradiation plus PDGFR-inhibition but were largely independent of TGFBR inhibition.
Figure 4. Gene expression profile of mouse lung tissue treated with ionizing irradiation and PDGF or TGFβ signaling inhibition. (A) Heat map showing the patterns of gene regulation after thoracic irradiation of mice and/or treatment with galunisertib (LY), imatinib (IM) or SU9518 (SU) and combinations. Three main clusters arise from a 1 factor ANOVA analysis: Cluster a groups genes which were mainly activated by the combinatory treatment of PDGFR-inhibitors (Imatinib or Su) with the TGFBR inhibitor galunisertib (LY). Irradiation had only a minor influence. Genes in cluster b were upregulated by radiation and normalized or inhibited by drug treatments which may be associated with their antifibrotic effects including SPP1 (Osteopontin, see B and ). Cluster C contains genes which are mainly activated by the combination of irradiation plus PDGFR-inhibition largely independent of TGFBR inhibition. (B) Relative mRNA expression of genes involved in PDGF and TGFβ signaling. Graphs depict mean values +/− SD. Statistical analysis was performed by Student's t-test; *p < 0.05, **p < 0.01, ***p < 0.001.
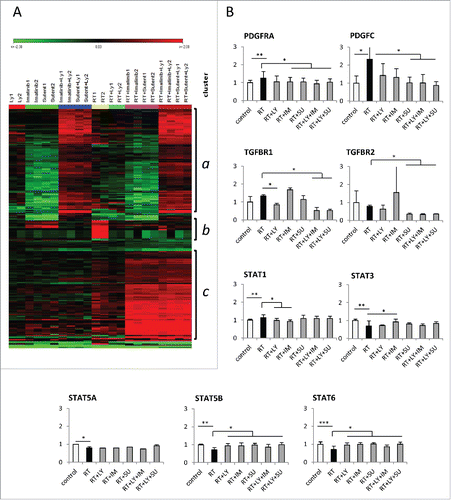
Figure 5. Radiation-induced Osteopontin expression is attenuated by PDGF or TGFβ signaling inhibition. (A) Relative mRNA expression of the SPP1 gene encoding the Osteopontin protein after thoracic irradiation and treatment with galunisertib (LY), imatinib (IM) or SU9518 (SU). Graphs depict mean values +/− SD. (week 24; Student's t-test; *p < 0.05 ). (B) Representative slides showing the time course of Osteopontin (OPN) staining of mouse lungs after radiation treatment and inhibition of PDGF and TGFβ signaling. (C) Quantification of Osteopontin staining in mouse lungs. For assessment of OPN expression, the intensity of cytoplasmic and cell membranous immunostaining was pathologically scored on anordinal scale of 0 (absence), 1+ (weak), 2+ (moderate), and 3+ (strong). Graphs depict mean values +/− standard deviation. (Kruskall–Wallis test; *p < 0.05 RT vs. RT+ monotherapy; **p < 0.05 RT+ monotherapy vs. RT+ dual therapy).

Detailed analyses of genes that could influence fibrogenesis showed that radiation with a single dose of 20 Gy resulted in a significantly increased expression of PDGFRA and PDGFC (p < 0.05, Student's t-test) (). STAT1 as a downstream target of the PDGF receptor was significantly upregulated in mice after radiation, while STAT3, STAT5A, STAT5B and STAT6 were downregulated upon thoracic RT (p < 0.05). Treatment with PDGFR inhibitors SU9518 or SU14816 resulted in decreased expression of PDGFRA, PDGFC and the PDGF-associated gene STAT1 as well as a significant increase in STAT5B and STAT6 levels compared with RT only lung tissue (p < 0.05). Blockade of the PDGF receptors also resulted in a reduced expression of TGFBR2 encoding the TGFβ receptor 2 (p < 0.05). TGFβ inhibition reduced the expression of TGFBR1 and significantly influenced the expression of the PDGF-dependent downstream regulators STAT1, STAT5B and STAT6 (p < 0.05). Combined inhibition of PDGF and TGFβ pathways in unirradiated tissue showed a significant upregulation of PDGFRA and PDGFD as well as TGFβ2 (Fig. S4). While certainly not all results of the genome-wide gene expression analysis can be explained with a high degree of certainty, here we speculate that the effective long term inhibition of PDGF and TGFβ signaling prompted a compensatory escape mechanism resulting in a transcriptional upregulation of the genes. This effect did obviously not occur when radiation was present. This may point to a more intricate crosstalk of PDGF, TGFβ and radiation than is currently known. In fact, upon lung irradiation, dual pathway blockade markedly reduced expression of PDGFRA, PDGFC, TGFBR1, TGFBR2 compared to tissue only treated with 20 Gy (p < 0.05). In contrast, STAT5B and STAT6 were found to be significantly upregulated by a combined treatment with PDGFR and TGFβR inhibitors (p < 0.05).
PDGF and TGFβ inhibition attenuate radiation-induced expression of Osteopontin
The chemokine Osteopontin is encoded by the SPP1 gene and has been suggested as a mediator for the invasion of profibrotic fibroblasts and hence as a biomarker for pulmonary fibrosis.Citation21-23
Irradiation of mouse lungs with 20 Gy induced a 3-fold increase in the expression of SPP1 compared to the untreated controls (). This increase was found fully abrogated when radiation treatment was followed by single-agent PDGF or TGFβ inhibition as well as combined blockade of both pathways. Immunohistochemical analysis of Osteopontin protein expression showed a 2-fold increase in staining intensity in mouse lung tissue at 12 weeks and a 3-fold increase at 24 weeks after irradiation (). Animals treated with SU14816, SU9518 or galunisertib after thoracic RT did not have an increase in Osteopontin protein expression at either time point after treatment. We also observed an additional benefit of combined inhibition of PDGF and TGFβ signaling compared to single-agent inhibition of either pathway individually (, p < 0.05).
Discussion
In this analysis, we examined the effects of dual inhibition of the PDGF and TGFβ pathways by small-molecule inhibitors to achieve an attenuation of radiation-induced lung damage and pulmonary fibrosis. Using two different PDGFR inhibitors, SU9518 and SU14816 (imatinib) and the specific TGFβR inhibitor, galunisertib, we found that dual-pathway blockade after thoracic irradiation was not only feasible and safe to treat irradiated animals, but also showed a significantly higher efficiency in reducing radiologic and pathologic signs of radiation-induced pulmonary fibrosis compared to single-agent treatment. These findings correlated well with the observed mouse health where mice treated with agents inhibiting both signaling pathways showed a significantly increased overall survival compared with animals treated with radiation alone or single inhibition of either pathway.
The role of PDGF and TGFβ signaling in the development of pulmonary fibrosis has been well established (reviewed inCitation24 andCitation25), and upregulation of components of the PDGF and TGFβ signaling cascades has been reported in lung tissues after thoracic RT.Citation2,26-28 Based on the rationale that both pathways play an important role in mediating pulmonary fibrosis, earlier publications have demonstrated improvements in the development of radiation-induced fibrosis by blocking either PDGF or TGFβ effects in animal models.Citation2,14
In the present study, PDGFR inhibition was achieved with either SU9518 or SU14816. SU9518 is a highly selective inhibitor of PDGF receptors α and β has been shown to block PDGFR kinase activity and PDGFR-induced cellular proliferation.Citation29,30 SU14816 (imatinib) was developed as an inhibitor of tyrosine kinase ABL and introduced into the clinic as an agent against chronic myelogenous leukemia; it has additional activity against c-Kit and PDGF receptors α and β; hence, the activities of SU9518 and SU14816 overlap regarding the inhibition of PDGF receptors.Citation31 Galunisertib is a highly selective inhibitor of TGFβR1 that was demonstrated to completely inhibit phosphorylation and activation of several of the receptor's downstream targets.Citation20
In recent years, in-depth analysis of profibrotic signaling has produced evidence for interconnections between the PDGF and TGFβ pathways: In studies examining bleomycin-induced lung damage both in vitro and in animal models, the profibrotic impact of TGFβ signaling was demonstrated to be regulated by the PDGF pathway and in turn mediated expression of PDGFCitation10,32,33; additionally, small molecule inhibitors of PDGF receptors were shown to also influence TGFβ-mediated profibrotic effects on lung fibroblasts.Citation13 The data demonstrate crosstalk between the PDGF and TGFβ signaling cascades and suggest the existence of a feedback loop between the two pathways. Supporting these notions of a connection between both pathways, our gene expression data revealed reduced levels of TGFβR2 by PDGF inhibition, and reversely, downregulation of the PDGF downstream target STAT1 by blockade of the TGFβ pathway. Notwithstanding this overlap and considering the various interdependent cascades within an intricate signaling network involved in many biological processes, the idea of blocking more than one pathway has become consequential for the treatment of various diseases including malignant diseases.Citation34-36 In our dataset, combined inhibition of PDGF and TGFβ signaling showed additional benefits regarding pulmonary fibrosis development and mouse survival when compared to inhibition of each pathway alone despite a hypothesized interaction between the two analyzed signaling cascades. As both the PDGF and TGFβ-mediated signal transductions employ redundant downstream mediators and may also involve several other “fibrogenic” signaling pathways, single-agent inhibition of either pathway likely does not achieve complete blockade of both cascades. Additionally, we observed that only upon dual pathway inhibition, PDGFRA and PDGFD as well as TGFB2 were found to be significantly upregulated in lung tissue. This increase in expression suggests a mechanism to compensate for the loss of TGFβ and PDGF signaling and hence implies an efficient blockade of both pathways as compared to single-agent inhibition.
Pulmonary fibrosis can be elicited by various detrimental agents or conditions, but the pathophysiological steps resulting in the development of the disease are similar irrespective of the nature of the initial trigger. IPF has the highest prevalence with between 65 and 81 cases per 100,000 people.Citation37 Due to the often fatal nature of this disease, there have been strong research efforts in recent years to identify viable treatment options. Pirfenidone is one of the two drugs that are currently approved for IPF in the clinic.Citation38 Intriguingly, pirfendidone has been shown to act as a partial TGFβ antagonist,Citation39 which may also strengthen the concept of TGFβ inhibitors in radiation-induced lung fibrosis. Similarly, the other drug recently clinically approved for IPF, nintedanib, is a PDGFR small molecule inhibitor itself.Citation40
With respect to the animal model, bleomycin-induced lung fibrosis models have been widely used to unravel the underlying mechanisms of pathogenesis.Citation10,32,41-43 However, instillation of bleomycin has been shown to highly accelerate fibrogenesis and results in fibrotic remodeling after only 2 weeks.Citation44 Therefore, despite common steps toward pulmonary fibrogenesis, the radiation-induced model may allow a more generalized transfer of findings to other forms of pulmonary fibrosis. Our model employing ionizing radiation led to a delayed course of fibrogenesis taking up to 24 weeks and may therefore resemble the development of pulmonary fibrosis in humans more closely.Citation2 Additionally, the stepwise thickening of lung septa and increase in pulmonary density as demonstrated here by CT and MR imaging as well as the continuous increase in collagen fiber deposition and fibroblast foci aggregation shown in histological samples of irradiated mouse lungs have been shown to also occur in the human disease.Citation45,46 Therefore, it is conceivable that the beneficial effects of combined PDFGR and TGFβR inhibition on radiation-induced pulmonary fibrosis may be transferrable to other forms of fibrotic disease of the lung.
The secreted glycoprotein, Osteopontin has been shown to mechanistically contribute to fibrogenesis and was therefore suggested as a useful biomarker for the diagnosis and progression of different forms of pulmonary fibrosis.Citation21-23,47 In our data set, radiation-induced pulmonary fibrosis was associated with highly increased expression of Osteopontin both on the mRNA and protein level, in line with findings published from patients with idiopathic pulmonary fibrosis.Citation48 One cannot exclude that Osteopontin levels were correlative and for example at least partially caused indirectly by hypoxia and HIF signaling. However, the significantly reduced levels of the biomarker Osteopontin by both PDGF and TGFβ-inhibiting drugs, which was enhanced by their combination, was associated with a strong reduction of fibrotic disease activity. This may support the notion for a more causative role for Osteopontin in radiation-induced lung fibrogenesis.
Our gene expression data () derived from lung samples at week 24 demonstrated the effects of monotherapies but also showed the complicated effects of combinatorial treatments. We found that radiation-upregulated genes such as SPP1 (Ostepontin) were downregulated by TGFβ and PDGF blockade. Thus, the expression data generated hypotheses for the potential beneficial antifibrotic drug effects, but also reflected the intricate signaling network that emerges long after the causative events had occurred. The expression data also reflected the shorter administration of the TGFβR inhibitor galunisertib (given for 4 weeks from week 2 to 6) compared with the PDGFR blockade which was given for 22 weeks throughout from week 2 until the end of observation at week 24. Likewise, the antifibrotic effects of galunisertib (administered for 4 weeks) could have been more prominent vs. PDGF inhibition (given for almost 6 mo). One reason for the 4 week only administration time was the shorter half life in plasma and the necessary BID oral gavage which makes it difficult to study long-term administration due to potential local side effects. Another reason was the avoidance of unwanted side effects by an overly long TGFβ inhibition.Citation49
Generally, the STAT proteins are thought to play an important role in fibrogenesis in most organs.Citation50,51 For example, IFNγ, a pleiotropic cytokine produced by T cells and NK cells, plays fundamental roles in innate and acquired immune responses, and transcriptional responses induced by IFNγ in most cells are mediated through the Jak-STAT pathway. Inhibitors of the Jak-STAT pathway are generally thought to inhibit fibrosis. However, the picture in the literature is conflicting, and the knowledge in the radiation context in the lungs is scarce. In general, STAT1 is thought to be a negative regulator of fibrosis, while the role of STAT2 is largely unknown.Citation52 STAT3 has been reported to have antifibrotic effects but the definitive role of STAT3 and the IL6-STAT3 pathway in lung fibrosis is unclear and the published results are conflicting.Citation53,54 The role of STAT4 in fibrosis is obscure with IL12 activation of STAT4 in immune cells inducing inflammation. STAT5 has been shown to have anti-fibrotic effects.Citation55 The role of STAT6 remains unclear in the literature. We found that radiation resulted in upregulated STAT1, but in downregulated the other STAT genes, while the PDGF and TGFβ inhibition counteracted the effects of radiation. Taken together, our data support the notion that the STATs are important regulators in radiation-induced lung fibrogenesis, but at the same time suggest a distinct time and context dependence.
Furthermore, despite the different timings of the treatments, the overlapping sections in the Venn diagrams (Fig. S5) showed that several genes were collectively regulated in the different groups. This may support a fibrosis associated network concept triggered by the interaction of radiation and drugs resulting in both gene expression and phenotypic consequences long after the initiating events.
Beyond their involvement in the development of pulmonary fibrosis, there is emerging evidence that an inhibition of PDGF and TGFβ signaling may also increase antitumor effects of RT.Citation30,56 This is interesting on a basic science level, because it links fibrogenesis with cancer, but may also be of translational interest, especially in the treatment of lung cancer, when signaling inhibition could act as both RT enhancer and reducer of side effects. One specific novelty of the presented study is the combined use of two agents to attenuate radiation-induced fibrosis and radiation-induced side effects in general. To our best knowledge, such a combinatorial strategy has not been reported. This aspect is interesting since in a therapeutic context for example with respect to cancer treatments, a combinatorial use of agents has been advocated in particular for targeted drugs.
The limitations of our study include the non-specificity of the PDGF signaling inhibitors SU9518 (sunitinib analog) and SU14816 (imatinib). One cannot completely exclude effects on other kinases because both drugs do have off target effects and inhibit other kinases; although with less potency and the significant overlap for PDGF only. Further limitations are the potential side effects in the clinical setting. Known potential side effects of PDGF-inhibiting drugs like Sunitinib (Sutent™) or Imatinib (Glivec™) use include vascular and homeostatic effects potentially leading to bleeding, swelling, blood pressure changes, rashes, fatigue, nausea and others. For TGFβ targeting clinical experience is more scarce, but all strategies are faced with the fact that TGFβ signaling is ubiquitously involved in many normal physiological functions including immune response. Long-term suppression of this pathway may lead to harmful off-target effects including for example effects on major vessels that might be enlarged by combined PDGF blockade. Other limits of the study include the use one high dose radiation rather than demonstrating a dose response including fractionation; the impossibility to completely understand the mechanisms of interaction of several drugs and radiation over 6 mo due to limits of animal numbers or limits of IHC staining for a complete inflammatome response. Moreover, although the antifibrotic effects and consecutive extension of the mouse life span were remarkable, the animals were not completely cured from the fibrotic lung disease, and it is likely that they still died prematurely.
However, despite these limitations we could show that the combination of TGFβ and PDGF blockade was an effective preclinical strategy to attenuate radiation-induced pneumonitis and lung fibrosis. With the clinical availability of such compounds our study suggests that this combination may offer a promising approach for clinical translation in lung cancer or other tumor entities.
Disclosure of potential conflicts of interest
Michael Lahn was an employee of Eli Lilly & Co. and holds stocks in the company.
KONI_A_1123366_supplemental_material.zip
Download Zip (1,017.4 KB)Acknowledgments
We acknowledge the technical assistance of M. Szymbara, and the scientific contributions of S. Bartling, H.-J. Gröne, A. Abdollahi, K. Lipson and J. Debus.
References
- Tsoutsou PG, Koukourakis MI. Radiation pneumonitis and fibrosis: mechanisms underlying its pathogenesis and implications for future research. Int J Radiat Oncol Biol Phys 2006; 66:1281-93; PMID:17126203; http://dx.doi.org/10.1016/j.ijrobp.2006.08.058
- Abdollahi A, Li M, Ping G, Plathow C, Domhan S, Kiessling F, Lee LB, McMahon G, Grone HJ, Lipson KE et al. Inhibition of platelet-derived growth factor signaling attenuates pulmonary fibrosis. J Exp Med 2005; 201:925-35; PMID:15781583; http://dx.doi.org/10.1084/jem.20041393
- Benveniste MF, Welsh J, Godoy MC, Betancourt SL, Mawlawi OR, Munden RF. New era of radiotherapy: an update in radiation-induced lung disease. Clin Radiol 2013; 68:e275-90; PMID:23473474; http://dx.doi.org/10.1016/j.crad.2013.01.013
- Nicolay NH, Lopez Perez R, Debus J, Huber PE. Mesenchymal stem cells - A new hope for radiotherapy-induced tissue damage? Cancer Lett 2015; 366:133-40; PMID:26166559; http://dx.doi.org/10.1016/j.canlet.2015.06.012
- Kong FM, Zhao L, Hayman JA. The role of radiation therapy in thoracic tumors. Hematol Oncol Clin North Am 2006; 20:363-400; PMID:16730299; http://dx.doi.org/10.1016/j.hoc.2006.01.021
- Roeder F, Friedrich J, Timke C, Kappes J, Huber P, Krempien R, Debus J, Bischof M. Correlation of patient-related factors and dose-volume histogram parameters with the onset of radiation pneumonitis in patients with small cell lung cancer. Strahlenther Onkol 2010; 186:149-56; PMID:20165822; http://dx.doi.org/10.1007/s00066-010-2018-4
- Yarnold J, Brotons MC. Pathogenetic mechanisms in radiation fibrosis. Radiother Oncol 2010; 97:149-61; PMID:20888056; http://dx.doi.org/10.1016/j.radonc.2010.09.002
- Rubin P, Johnston CJ, Williams JP, McDonald S, Finkelstein JN. A perpetual cascade of cytokines postirradiation leads to pulmonary fibrosis. Int J Radiat Oncol Biol Phys 1995; 33:99-109; PMID:7642437; http://dx.doi.org/10.1016/0360-3016(95)00095-G
- Williams JP, Johnston CJ, Finkelstein JN. Treatment for radiation-induced pulmonary late effects: spoiled for choice or looking in the wrong direction? Curr Drug Targets 2010; 11:1386-94; PMID:20583979; http://dx.doi.org/10.2174/1389450111009011386
- Andrianifahanana M, Wilkes MC, Gupta SK, Rahimi RA, Repellin CE, Edens M, Wittenberger J, Yin X, Maidl E, Becker J et al. ProfibroticTGFbeta responses require the cooperative action of PDGF and ErbB receptor tyrosine kinases. FASEB J 2013; 27(11):4444-54; PMID:23913859; http://dx.doi.org/10.1096/fj.12-224907
- Kolb M, Margetts PJ, Anthony DC, Pitossi F, Gauldie J. Transient expression of IL-1beta induces acute lung injury and chronic repair leading to pulmonary fibrosis. J Clin Invest 2001; 107:1529-36; PMID:11413160; http://dx.doi.org/10.1172/JCI12568
- Farooqi AA, Waseem S, Riaz AM, Dilawar BA, Mukhtar S, Minhaj S, Waseem MS, Daniel S, Malik BA, Nawaz A et al. PDGF: the nuts and bolts of signalling toolbox. Tumour Biol 2011; 32:1057-70; PMID:21769672; http://dx.doi.org/10.1007/s13277-011-0212-3
- Chaudhary NI, Roth GJ, Hilberg F, Muller-Quernheim J, Prasse A, Zissel G, Schnapp A, Park JE. Inhibition of PDGF, VEGF and FGF signalling attenuates fibrosis. Eur Respir J 2007; 29:976-85; PMID:17301095; http://dx.doi.org/10.1183/09031936.00152106
- Flechsig P, Dadrich M, Bickelhaupt S, Jenne J, Hauser K, Timke C, Peschke P, Hahn EW, Grone HJ, Yingling J et al. LY2109761 attenuates radiation-induced pulmonary murine fibrosis via reversal of TGF-β and BMP-associated proinflammatory and proangiogenic signals. Clin Cancer Res 2012; 18:3616-27; PMID:22547771; http://dx.doi.org/10.1158/1078-0432.CCR-11-2855
- Puthawala K, Hadjiangelis N, Jacoby SC, Bayongan E, Zhao Z, Yang Z, Devitt ML, Horan GS, Weinreb PH, Lukashev ME et al. Inhibition of integrin α(v)beta6, an activator of latent transforming growth factor-β, prevents radiation-induced lung fibrosis. Am J Respir Crit Care Med 2008; 177:82-90; PMID:17916808; http://dx.doi.org/10.1164/rccm.200706-806OC
- Cigna N, Farrokhi Moshai E, Brayer S, Marchal-Somme J, Wemeau-Stervinou L, Fabre A, Mal H, Leseche G, Dehoux M, Soler P et al. The hedgehog system machinery controls transforming growth factor-β-dependent myofibroblastic differentiation in humans: involvement in idiopathic pulmonary fibrosis. Am J Pathol 2012; 181:2126-37; PMID:23031257; http://dx.doi.org/10.1016/j.ajpath.2012.08.019
- Leask A, Abraham DJ. TGF-β signaling and the fibrotic response. FASEB J 2004; 18:816-27; PMID:15117886; http://dx.doi.org/10.1096/fj.03-1273rev
- Attisano L, Wrana JL. Signal transduction by the TGF-β superfamily. Science 2002; 296:1646-7; PMID:12040180; http://dx.doi.org/10.1126/science.1071809
- Rodon J, Carducci MA, Sepúlveda JM, Azaro A, Calvo E, Seoane J, Brana I, Sicart E, Gueorguieva I, Cleverly A et al. Integrated data review of the first-in-human dose (FHD) study evaluating safety, pharmacokinetics (PK), and pharmacodynamics (PD) of the oral transforming growth factor-β (TGF-ß) receptor I kinase inhibitor, LY2157299 monohydrate (LY). Annual Meeting of the American Society of Clinical Oncology (ASCO), Chicago 2013; Abstract Number 2016.
- Bueno L, de Alwis DP, Pitou C, Yingling J, Lahn M, Glatt S, Troconiz IF. Semi-mechanistic modelling of the tumour growth inhibitory effects of LY2157299, a new type I receptor TGF-β kinase antagonist, in mice. Eur J Cancer 2008; 44:142-50; PMID:18039567; http://dx.doi.org/10.1016/j.ejca.2007.10.008
- Tsukui T, Ueha S, Abe J, Hashimoto S, Shichino S, Shimaoka T, Shand FH, Arakawa Y, Oshima K, Hattori M et al. Qualitative rather than quantitative changes are hallmarks of fibroblasts in bleomycin-induced pulmonary fibrosis. Am J Pathol 2013; 183:758-73; PMID:23886891; http://dx.doi.org/10.1016/j.ajpath.2013.06.005
- Schneider DJ, Lindsay JC, Zhou Y, Molina JG, Blackburn MR. Adenosine and osteopontin contribute to the development of chronic obstructive pulmonary disease. FASEB J 2010; 24:70-80; PMID:19720619; http://dx.doi.org/10.1096/fj.09-140772
- Vij R, Noth I. Peripheral blood biomarkers in idiopathic pulmonary fibrosis. Transl Res 2012; 159:218-27; PMID:22424426; http://dx.doi.org/10.1016/j.trsl.2012.01.012
- Koli K, Myllarniemi M, Keski-Oja J, Kinnula VL. Transforming growth factor-β activation in the lung: focus on fibrosis and reactive oxygen species. Antioxid Redox Signal 2008; 10:333-42; PMID:17961070; http://dx.doi.org/10.1089/ars.2007.1914
- Ingram JL, Bonner JC. EGF and PDGF receptor tyrosine kinases as therapeutic targets for chronic lung diseases. Curr Mol Med 2006; 6:409-21; PMID:16900664; http://dx.doi.org/10.2174/156652406777435426
- Rube CE, Uthe D, Schmid KW, Richter KD, Wessel J, Schuck A, Willich N, Rube C. Dose-dependent induction of transforming growth factor β (TGF-β) in the lung tissue of fibrosis-prone mice after thoracic irradiation. Int J Radiat Oncol Biol Phys 2000; 47:1033-42; PMID:10863076; http://dx.doi.org/10.1016/S0360-3016(00)00482-X
- Yi ES, Bedoya A, Lee H, Chin E, Saunders W, Kim SJ, Danielpour D, Remick DG, Yin S, Ulich TR. Radiation-induced lung injury in vivo: expression of transforming growth factor-β precedes fibrosis. Inflammation 1996; 20:339-52; PMID:8872498; http://dx.doi.org/10.1007/BF01486737
- Thornton SC, Walsh BJ, Bennett S, Robbins JM, Foulcher E, Morgan GW, Penny R, Breit SN. Both in vitro and in vivo irradiation are associated with induction of macrophage-derived fibroblast growth factors. Clin Exp Immunol 1996; 103:67-73; PMID:8565289; http://dx.doi.org/10.1046/j.1365-2249.1996.898598.x
- Yamasaki Y, Miyoshi K, Oda N, Watanabe M, Miyake H, Chan J, Wang X, Sun L, Tang C, McMahon G et al. Weekly dosing with the platelet-derived growth factor receptor tyrosine kinase inhibitor SU9518 significantly inhibits arterial stenosis. Circ Res 2001; 88:630-6; PMID:11282898; http://dx.doi.org/10.1161/01.RES.88.6.630
- Li M, Ping G, Plathow C, Trinh T, Lipson KE, Hauser K, Krempien R, Debus J, Abdollahi A, Huber PE. Small molecule receptor tyrosine kinase inhibitor of platelet-derived growth factor signaling (SU9518) modifies radiation response in fibroblasts and endothelial cells. BMC Cancer 2006; 6:79; PMID:16556328; http://dx.doi.org/10.1186/1471-2407-6-79
- Waller CF. Imatinibmesylate. Recent Results Cancer Res 2010; 184:3-20; PMID:20072827; http://dx.doi.org/10.1007/978-3-642-54490-3_1
- Rhee CK, Lee SH, Yoon HK, Kim SC, Lee SY, Kwon SS, Kim YK, Kim KH, Kim TJ, Kim JW. Effect of nilotinib on bleomycin-induced acute lung injury and pulmonary fibrosis in mice. Respiration 2011; 82:273-87; PMID:21659722; http://dx.doi.org/10.1159/000327719
- Bonner JC, Badgett A, Lindroos PM, Osornio-Vargas AR. Transforming growth factor β 1 downregulates the platelet-derived growth factor α-receptor subtype on human lung fibroblasts in vitro. Am J Respir Cell Mol Biol 1995; 13:496-505; PMID:7546780; http://dx.doi.org/10.1165/ajrcmb.13.4.7546780
- Martelli AM, Chiarini F, Evangelisti C, Cappellini A, Buontempo F, Bressanin D, Fini M, McCubrey JA. Two hits are better than one: targeting both phosphatidylinositol 3-kinase and mammalian target of rapamycin as a therapeutic strategy for acute leukemia treatment. Oncotarget 2012; 3:371-94; PMID:22564882; http://dx.doi.org/10.18632/oncotarget.477
- Konecny GE. Emerging strategies for the dual inhibition of HER2-positive breast cancer. Current Opin Obstet Gynecol 2013; 25:55-65; PMID:23241641; http://dx.doi.org/10.1097/GCO.0b013e32835c5e90
- Socinski MA. Multitargeted receptor tyrosine kinase inhibition: an antiangiogenic strategy in non-small cell lung cancer. Cancer Treat Rev 2011; 37:611-7; PMID:21641723; http://dx.doi.org/10.1016/j.ctrv.2011.04.003
- Raghu G, Collard HR, Egan JJ, Martinez FJ, Behr J, Brown KK, Colby TV, Cordier JF, Flaherty KR, Lasky JA et al. An official ATS/ERS/JRS/ALAT statement: idiopathic pulmonary fibrosis: evidence-based guidelines for diagnosis and management. Am J Respir Crit Care Med 2011; 183:788-824; PMID:21471066; http://dx.doi.org/10.1164/rccm.2009-040GL
- King TE, Jr, Bradford WZ, Castro-Bernardini S, Fagan EA, Glaspole I, Glassberg MK, Gorina E, Hopkins PM, Kardatzke D, Lancaster L et al. A phase 3 trial of pirfenidone in patients with idiopathic pulmonary fibrosis. N Engl J Med 2014; 370:2083-92; PMID:24836312; http://dx.doi.org/10.1056/NEJMoa1402582
- Yang Y, Ye Y, Lin X, Wu K, Yu M. Inhibition of pirfenidone on TGF-beta2 induced proliferation, migration and epithlial-mesenchymal transition of human lens epithelial cells line SRA01/04. PloSOne 2013; 8:e56837; PMID:23437252; http://dx.doi.org/10.1371/journal.pone.0056837
- Richeldi L, Cottin V, Flaherty KR, Kolb M, Inoue Y, Raghu G, Taniguchi H, Hansell DM, Nicholson AG, Le Maulf F et al. Design of the INPULSIS trials: two phase 3 trials of nintedanib in patients with idiopathic pulmonary fibrosis. Respir Med 2014; 108:1023-30; PMID:24834811; http://dx.doi.org/10.1016/j.rmed.2014.04.011
- Sharplin J, Franko AJ. A quantitative histological study of strain-dependent differences in the effects of irradiation on mouse lung during the intermediate and late phases. Radiat Res 1989; 119:15-31; PMID:2756106; http://dx.doi.org/10.2307/3577364
- Gurujeyalakshmi G, Hollinger MA, Giri SN. Pirfenidone inhibits PDGF isoforms in bleomycin hamster model of lung fibrosis at the translationallevel. Am J Physiol 1999; 276:L311-8; PMID:9950894.
- Maeda A, Hiyama K, Yamakido H, Ishioka S, Yamakido M. Increased expression of platelet-derived growth factor A and insulin-like growth factor-I in BAL cells during the development of bleomycin-induced pulmonary fibrosis in mice. Chest 1996; 109:780-6; PMID:8617091; http://dx.doi.org/10.1378/chest.109.3.780
- Daniels CE, Wilkes MC, Edens M, Kottom TJ, Murphy SJ, Limper AH, Leof EB. Imatinibmesylate inhibits the profibrogenic activity of TGF-β and prevents bleomycin-mediated lung fibrosis. J Clin Invest 2004; 114:1308-16; PMID:15520863; http://dx.doi.org/10.1172/JCI200419603
- Kirk JM, Da Costa PE, Turner-Warwick M, Littleton RJ, Laurent GJ. Biochemical evidence for an increased and progressive deposition of collagen in lungs of patients with pulmonary fibrosis. Clin Sci 1986; 70:39-45; PMID:3943276; http://dx.doi.org/10.1042/cs0700039
- Yamashita M, Yamauchi K, Chiba R, Iwama N, Date F, Shibata N, Kumagai H, Risteli J, Sato S, Takahashi T et al. The definition of fibrogenic processes in fibroblastic foci of idiopathic pulmonary fibrosis based on morphometric quantification of extracellular matrices. Hum Pathol 2009; 40:1278-87; PMID:19386353; http://dx.doi.org/10.1016/j.humpath.2009.01.014
- Sabo-Attwood T, Ramos-Nino ME, Eugenia-Ariza M, Macpherson MB, Butnor KJ, Vacek PC, McGee SP, Clark JC, Steele C, Mossman BT. Osteopontin modulates inflammation, mucin production, and gene expression signatures after inhalation of asbestos in a murine model of fibrosis. Am J Pathol 2011; 178:1975-85; PMID:21514415; http://dx.doi.org/10.1016/j.ajpath.2011.01.048
- Pardo A, Gibson K, Cisneros J, Richards TJ, Yang Y, Becerril C, Yousem S, Herrera I, Ruiz V, Selman M et al. Up-regulation and profibrotic role of osteopontin in human idiopathic pulmonary fibrosis. PLoS Med 2005; 2:e251; PMID:16128620; http://dx.doi.org/10.1371/journal.pmed.0020251
- Connolly EC, Freimuth J, Akhurst RJ. Complexities of TGF-β targeted cancer therapy. Int J Biol Sci 2012; 8:964-78; PMID:22811618; http://dx.doi.org/10.7150/ijbs.4564
- Kong X, Horiguchi N, Mori M, Gao B. Cytokines and STATs in Liver Fibrosis. Front Physiol 2012; 3:69; PMID:22493582; http://dx.doi.org/10.3389/fphys.2012.00069
- Nazha A, Khoury JD, Rampal RK, Daver N. Fibrogenesis in Primary Myelofibrosis: Diagnostic, Clinical, and Therapeutic Implications. Oncologist 2015; 20:1154-60; PMID:26304912; http://dx.doi.org/10.1634/theoncologist.2015-0094
- Ryter SW, Choi AM, Kim HP. Profibrogenic phenotype in caveolin-1 deficiency via differential regulation of STAT-1/3 proteins. Biochem Cell Biol 2014; 92:370-8; PMID:25263949; http://dx.doi.org/10.1139/bcb-2014-0075
- Pedroza M, Le TT, Lewis K, Karmouty-Quintana H, To S, George AT, Blackburn MR, Tweardy DJ, Agarwal SK. STAT-3 contributes to pulmonary fibrosis through epithelial injury and fibroblast-myofibroblast differentiation. FASEB J 2015; 30(1):129-40; PMID:26324850
- Tang J, Liu CY, Lu MM, Zhang J, Mei WJ, Yang WJ, Xie YY, Huang L, Peng ZZ, Yuan QJ et al. Fluorofenidone protects against renal fibrosis by inhibiting STAT3 tyrosine phosphorylation. Mol Cell Biochem 2015; 407:77-87; PMID:26033204; http://dx.doi.org/10.1007/s11010-015-2456-5
- Wiezel D, Assadi MH, Landau D, Troib A, Kachko L, Rabkin R, Segev Y. Impaired renal growth hormone JAK/STAT5 signaling in chronic kidney disease. Nephrol Dial Transpl 2014; 29:791-9; PMID:24463190; http://dx.doi.org/10.1093/ndt/gfu003
- TimkeC, ZieherH, RothA, HauserK, LipsonKE, WeberKJ, DebusJ, AbdollahiA, HuberPE. Combination of vascular endothelial growth factor receptor/platelet-derived growth factor receptor inhibition markedly improves radiation tumor therapy. Clin Cancer Res 2008; 14:2210-9; PMID:18381963; http://dx.doi.org/10.1158/1078-0432.CCR-07-1893