ABSTRACT
Agonistic tumor necrosis factor (TNF)-related apoptosis-inducing ligand (TRAIL)-receptor-specific antibodies are attractive antitumor therapeutics. Recently, our group has generated several human monoclonal antibodies (mAbs) to TRAIL-receptor-1 (TRAIL-R1) (TR1-IgGs) using ISAAC technology. However, these TR1-IgGs did not demonstrate ideal apoptosis-inducing capacity in the absence of additional antibodies. To overcome this limitation, we class-switched the TR1-IgGs to TRAIL-R1 IgM antibodies (TR1-IgMs); TR1-IgMs might possess high valency and facilitate the crosslinking of the cell surface receptors. We showed that the TR1-IgMs bound TRAIL-R1, activated the caspase signal, and induced strong apoptosis (100-fold higher compared with the IgG form in one case) in human tumor cell lines without any additional crosslinking in vitro. We further demonstrated that these TR1-IgMs dramatically inhibited tumor growth in a xenograft model through the caspase activation cascade. These data suggest that TR1-IgMs may become potential immunotherapeutic agents for cancer therapy.
Abbreviations
DISC | = | death-inducing signaling complex |
EC50 | = | 50% effective concentration |
ELISA | = | enzyme-linked immunosorbent assay |
FITC | = | fluorescein isothiocyanate |
IgG | = | immunoglobulin G |
IgM | = | immunoglobulin M |
mAbs | = | monoclonal antibodies |
PE | = | phycoerythrin |
SCID | = | severe combined immunodeficient |
TRAIL | = | tumor necrosis factor-related apoptosis-inducing ligand |
TRAIL-R | = | TRAIL receptors |
TR1-IgG | = | TRAIL-R1-specific IgG |
TR1-IgM | = | TRAIL-R1-specific IgM |
Introduction
Apoptosis is a cellular process in which the organism regulates the number of cells in normal tissue compartments and eliminates unnecessary or damaged cells.Citation1 There are two major signaling pathways that trigger apoptosis in mammalian cells: the intrinsic and extrinsic pathways.Citation2 The TRAIL, a member of the TNF superfamily of cytokines, is a trimeric protein that is capable of activating both the extrinsic and intrinsic pathways for cellular apoptosis.Citation3,4 By binding to TRAIL-R1 and -R2, TRAIL induces the formation of the death-inducing signaling complex (DISC), which consists of a Fas-associated death domain and procaspase-8 and -10.Citation5,6 The activated caspase-8 then triggers the apoptotic pathway by activating caspase-3, -6, and -7, which leads to cell death.Citation6-8 In addition to TRAIL-R1 and -R2, there are other receptors, such as TRAIL-R3, -R4, and osteoprotegerin (OPG), although these receptors cannot induce cellular apoptosis because they lack functional death domains.Citation9-12
It is worth noting that TRAIL-induced apoptosis only occurs in human cancer cell lines, whereas it rarely occurs in normal cells.Citation13-17 Accordingly, TRAIL-R1 and -R2 have been widely studied as potential therapeutic targets of cancer in the past, and TRAIL variants and mAbs of TRAIL-R1 and -R2 have been developed as possible reagents for cancer treatment. However, the hepatotoxicity and short half-life of TRAIL variants have hampered their application.Citation18 Therefore, TRAIL-R1/2 antibodies are expected to be more attractive for cancer therapy.
TRAIL-R1/2 can independently self-assemble into trimers without any other ligands.Citation19 Their further assembly with ligands is a prerequisite for activating apoptosis.Citation20 Previous studies using xenograft tumor-bearing mice that were immune-deficient and lacked endogenous IgGs demonstrated that the anti-TRAIL-R1 or -R2 antibodies showed ideal antitumor effects by crosslinking the Fcγ receptors (FcγRs) on immune cells.Citation21-25 However, a series of clinical trials based on these effective antitumor antibodies have failedCitation26,27 because the crosslinking with FcγR might be impaired in the presence of competitive endogenous IgGs.Citation28
Regarding other crosslinking, the use of secondary antibodies is not suitable for clinical treatments in patients because these antibodies are usually prepared from animals and may induce side effects due to their antigenicity.Citation29 Therefore, agonistic TRAIL-R1/2-specific mAbs that induce cancer cell apoptosis in the absence of crosslinking reagents are desired for cancer therapy. In this context, Pukac et al. developed a fully human agonistic TRAIL-R1 mAb, HGS-ETR1, which achieved significant apoptosis of tumor cells in vitro and in vivo, without additional crosslinking.Citation22 However, HGS-ETR1 did not show antitumor effects in clinical trials.Citation30-32
We have also developed a series of human TRAIL-R1-specific IgGs (TR1-IgGs) that induce apoptosis using the ISAAC technologyCitation33 and human immunoglobulin γ and κ loci chimeric TransChromo (TC) mice.Citation34 However, these mAbs could not induce apoptosis in the absence of crosslinking antibodies.Citation35 Antibodies of the IgM class play an important role in primary immune responses.Citation36,37 The predominant structure of IgM in serum is a pentameric form.Citation38 Recently, Kunert’s group constructed a class-switched HIV-specific IgM by combining the variable region of HIV-IgG with the constant region of IgM.Citation39,40 Considering this finding, we hypothesized that the conversion of TR1-IgG to pentameric IgM might facilitate the cell surface receptor crosslinking due to the high valency of IgM. In this study, we generated TRAIL-R1-IgM antibodies (TR1-IgMs) by combining the variable region of TR1-IgGsCitation35 with the constant region of IgM. The resulting TR1-IgMs induced strong apoptosis in not only various human cancer cell lines but also xenograft tumor-bearing mice. These TR1-IgMs antibodies may become an expectable immunotherapeutic agent for cancer therapy.
Results
TR1-IgMs bind specifically to human TRAIL-R1
To produce the TR1-IgMs, we constructed the TR1-IgM cDNA by substituting the constant region of five clones (401, 404, 419, 422, and 438) of TR1-IgG with that of IgM.Citation35 We then co-transfected the cells with the heavy- and light-chain cDNAs together with or without the joining (J) chain cDNA. An anti-influenza IgM was also produced as a control using the same method. The purified TR1-IgMs and the control-IgM were confirmed by western blotting (). Previous reports stated that the J chain existed only in pentameric IgMs, whereas the hexameric and pentameric forms of IgM were produced without the J chain.Citation41 Consistently, western blot analysis revealed that without J chain, the TR1-IgMs (TR1-IgM(J-)s) and control-IgM(J-) were present in both the pentameric and hexameric forms, whereas the TR1-IgM(J+)s and control-IgM(J+) with the J chain only formed pentamers (). The TR1-IgM(J+)s and control-IgM(J+) were adopted in the subsequent studies because the pentameric form of IgM is predominant in human serum.Citation38
Figure 1. Construction and evaluation of the TR1-IgMs. (A) Western blot analysis of the recombinant purified TR1-IgMs. Ten nanograms of each of the biotinylated-TR1-IgM(+/−)s (TR1-IgM(J+/−)-401, 404, 419, 422, and 438) and control-IgM(+/−) was immunoblotted with horseradish peroxidase-conjugated streptavidin. (B) Reactivity of the TR1-IgM(J+)s with the recombinant human TRAIL-R family. The binding of the TR1-IgM(J+)s to human TRAIL-R1-Fc, TRAIL-R2-Fc, TRAIL-R3-Fc, TRAIL-R4-Fc, or OPG was examined by ELISA. Control-IgM(J+) was used as a control. The data are shown as the mean ± SD of triplicate cultures. (C) The binding of the TR1-IgM(J+)s to the Colo205 cell line was analyzed by flow cytometry. The cells were stained with 5 μg/mL of biotinylated-TR1-IgM(J+)s and control-IgM(J+) as indicated, followed by R-PE-conjugated streptavidin, and analyzed with flow cytometry. X-axis, fluorescence intensity of PE; Y-axis, relative cell number. Representative data of three independent experiments in which similar results were obtained are shown.
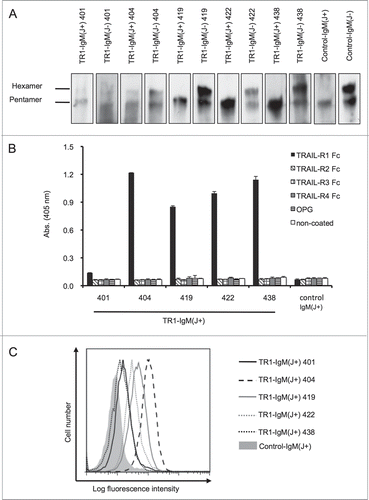
To clarify whether the TR1-IgM(J+)s bind to recombinant human TRAIL receptor (TRAIL-R), we performed an enzyme-linked immunosorbent assay (ELISA) using chimeric recombinant human TRAIL-R-Fc proteins. All five TR1-IgM(J+)s speci-fically bound to TRAIL-R1-Fc, but not to TRAIL-R2-Fc, TRAIL-R3-Fc, TRAIL-R4-Fc, or OPG (). The control-IgM did not bind to any of the proteins. The binding of TR1-IgM(J+) 401 to rhTRAIL was significantly weak. This weak binding may be due to its previously demonstrated low affinity.Citation35 In agreement with a previous study,Citation35 flow cytometry analysis also revealed that the TR1-IgM(J+)s bound to the surfaces of tumor cells that expressed TRAIL-R1 ( and Fig. S1). Together, these results indicated that our five clones of TR1-IgMs specifically bound to TRAIL-R1.
TR1-IgMs induce cell death by activating caspase signaling in human tumor cell lines
Next, we investigated whether the TR1-IgM(J+)s could induce cell death in cancer cell lines without additional crosslinking. Treatment of the TRAIL-sensitive Colo205 cell lineCitation42 with various concentrations of TR1-IgM(J+)s resulted in a significant and dose-dependent reduction of cell viability at 24 h, whereas the groups treated with control-IgM(J+), control-IgG, or TR1-IgGs did not show cytotoxic activity, except that the TR1-IgG 438-treated group exhibited a weak induction of cell death (). The 50% effective concentration (EC50) of TR1-IgM(J+)s for the Colo205 cell line ranged from 0.5 to 100 ng/mL ( and Fig. S2). We compared the cytotoxic activity of TR1-IgG in the presence of the secondary crosslinking antibody with that of TR1-IgM (Fig. S3). TR1-IgG 422 with the secondary crosslinking antibody induced apoptosis in Colo205 cell line with EC50 of approximately 1,000 ng/mL, whereas that of TR-IgM 422 was 0.5 ng/mL, showing the remarkably higher cytotoxic activity of TR1-IgM compared to TR-1 IgG even in the presence of crosslinking antibody. TR1-IgM(J+) effectively induced apoptosis in the TRAIL-sensitive DU145 cell lineCitation43 but not in the TRAIL-resistant A549Citation44 and MCF7Citation45 cell lines (Fig. S4). The current results demonstrated that the TR1-IgM(J+)s effectively induced apoptosis in the TRAIL-sensitive cell lines.
Figure 2. TR1-IgMs induce tumor cell killing by apoptotic signaling. (A) Cell viability assay. Colo205 cells were incubated with the indicated antibodies for 24 h, and cell viability was measured. (B) Measurement of activated caspases. The cells were incubated with the indicated concentrations of TR1-IgM(J+)s or control-IgM(J+). Activated caspase-8, and -3/7 were measured after 3 and 6 h of culture, respectively. (C) Caspase-dependent cell death in Colo205 cells. The cells were incubated with or without the indicated concentrations of z-VAD-fmk for 4 h. Then, the cells were cultured with TR1-IgMs or control-IgM for 24 h. After cell culture, the cell viability was determined. The data are shown as the mean ± SD of triplicate cultures. *p < 0.05 by Student’s t-test.
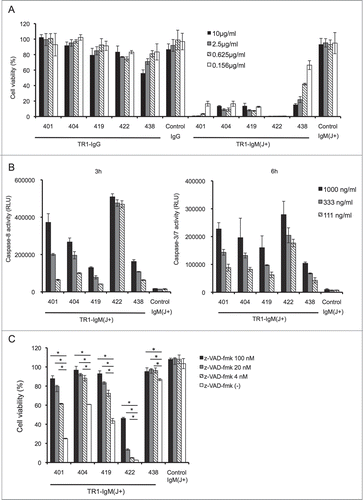
Table 1. EC50 values of the TR1-IgM(J+)s
It has been reported that TRAIL-induced apoptosis in human cancer cells is primarily triggered by the activation of caspase-8 and -3, the major caspases that are responsible for apoptosis.Citation6 To verify whether TR1-IgMs induce cell death via the caspase activation pathway, we incubated Colo205 cells with TR1-IgM(J+)s, and the caspase activation was investigated. As shown in , caspase-8 and capase-3/7 were clearly activated in a dose-dependent fashion after 3 and 6 h of culture, respectively. The control-IgM(J+) did not induce caspase-8 or caspase-3 activity in Colo205 cells. Furthermore, the presence of a pan-caspase inhibitor, z-VAD-fmk, which has been reported to block caspase-mediated apoptosis,Citation46 prevented the TR1-IgM(J+)s-induced reduction of cell viability corresponding to TRAIL-induced apoptosis (). These results showed that TR1-IgM(J+)s induced cell death in TRAIL-R1-expressing cell lines in a caspase-dependent fashion.
In vivo antitumor effect of the TR1-IgM antibodies without a crosslinking reagent
To assess the antitumor activity of TR1-IgM(J+)s in vivo, we evaluated the capacity of the most effective TR1-IgM(J+) 422 antibody () in xenograft models using Colo205 tumor-transplanted SCID mice. The tumors were pre-established to a volume of approximately 100 mm3, and different doses of TR1-IgM(J+) 422 (1, 3.3, and 10 mg/kg body weight) and control-IgM(J+) (10 mg/kg body weight) were then intravenously (i.v.) administered to the mice three times per week. As shown in and 3B, treatment with TR1-IgM(J+) 422 resulted in an initial reduction in the tumor size and the retardation of tumor growth in a dose-dependent manner. The control-IgM(J+) treatment did not inhibit tumor growth. Histological analysis of the tumor sections demonstrated that TR1-IgM(J+) 422 induced cell death within 2 d after treatment (). To clarify whether the cell death was due to the caspase-dependent apoptosis, we analyzed caspase-3 activation in the tumors that had been treated with the TR1-IgM(J+) 422 antibody. We found that antibody administration clearly induced caspase-3 activation in the pre-developed Colo205 tumor cells 4 h after administration (). These data demonstrate that the TR1-IgM(J+) 422 antibody penetrated the tumor in vivo to induce immediate caspase-dependent apoptosis and cell death, exhibiting a potent antitumor effect.
Figure 3. Antitumor efficacy of TR1-IgMs on Colo205 cell-derived mouse xenograft tumors in vivo. (A) The tumor growth curves of each group of tumor-bearing mice. SCID mice (10 per group) bearing Colo205 xenograft tumors were i.v. injected with TR1-IgM(J+) 422 or control-IgM(J+) on the indicated days (arrows). Each time point represents the mean value (±S .E.M.) of the tumor sizes within the treated group on the day of measurement. (B) Representative photographs of the Colo205 tumor-bearing mice in each group on day 0 or day 20 after treatment. The magnified photo shows that the tumors (within dotted lines) in the TR1-IgM(J+) 422 (10mg/kg)-treated mice were notably reduced compared with the tumors in the control-IgM(J+) (10mg/kg)-treated mice. (C) Representative photomicrographs of hematoxylin and eosin staining of the tumor tissues from each group of mice at 2 d post-treatment. Expanded views of the region marked with black boxes are shown (right). Original magnification, ×100 (left), ×400 (right). (D) Caspase-3 activation following the injection of TR1-IgM(J+) 422. SCID mice that had pre-established Colo205 tumor xenografts were injected with a single dose (10 mg/kg body weight) of TR1-IgM(J+) 422 (solid line) or control-IgM (shaded peak). The tumors were then excised 4 h after injection, and activated caspase-3 induction was analyzed by flow cytometry. X-axis; fluorescence intensity of fluorescein isothiocyanate (FITC), Y-axis; relative cell number.
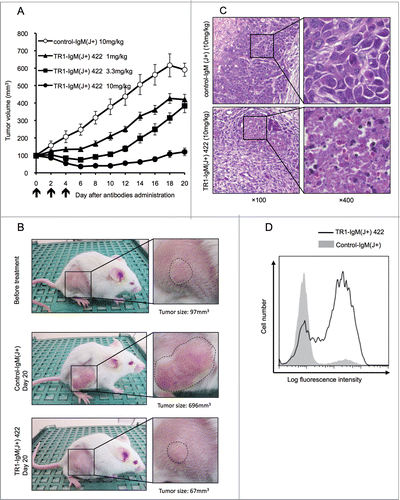
Discussion
Previously, we used TC mice to develop fully human TR1-IgGs with tumoricidal activity,Citation35 but these mAbs required additional crosslinking antibodies to induce apoptosis in the target cells.Citation35 Additional antibodies may induce serious side effects due to their antigenicityCitation29 and the formation of immune complexes with blood IgGs. To overcome this limitation, we converted the TR1-IgGs to TR1-IgMs because secreted IgMs have 10 antigen-binding sites and have a superior capacity to crosslink molecules compared with IgGs.Citation47 The resulting TR1-IgMs are expected to induce apoptosis in vitro and in vivo in the absence of additional crosslinking and, thus, would be more suitable for cancer therapy.
High functionality is one of the critical parameters for antibodies to be used as potent and efficacious therapeutics.Citation48 In the present study, we demonstrated that one of the TR1-IgMs, TR1-IgM(J+) 422, showed high antitumor activity on the Colo205 cell line in vitro, with an EC50 of approximately 0.5 ng/mL. This result was approximately 200-fold higher than that of HGS-ETR1, an agonistic anti-TR1 antibody used in clinical trials.Citation22 Therefore, TR1-IgM(J+) 422 could be an efficient antitumor agent in cancer therapy.
One of drawbacks of IgM-form antibodies as therapeutic antibodies is that they are difficult to produce compared with IgG-form antibodies. In this context, Tchoudakova et al developed a PER.C6® cell line that could produce large amounts of IgMs, which promotes the scale-up of IgM production for clinical use.Citation49 The second drawback of IgM-form antibodies is that their half-life in the blood is shorter than that of IgG.Citation50 In fact, in the xenograft model, the administration of a high dose (10 mg/kg) of TR1-IgM(J+) 422 elicited significant tumor regression during the first 8 d in all of the mice. However, beginning day 9, the tumors slowly began to re-grow. With these drawbacks, the protocol for TR1-IgM production and administration should be further optimized.
It has been reported that combination treatments with anti-TRAIL-R1 or R2 antibodies and different chemotherapeutics resulted in enhanced cell killing in vitro compared to antibodies or chemotherapeutics alone.Citation22-25,28,51,52 The enhanced cell death may be because the chemotherapeutics can upregulate TRAIL-R1/2Citation53,54 and proapoptotic proteinCitation55 and decrease antiapoptotic protein expression levels.Citation53 In addition, Tuthill et al. found that an anti-TRAIL-R2 antibody and recombinant TRAIL can synergize to kill cancer cells.Citation56 In our previous study, the combination of TR1-IgGs and recombinant TRAIL significantly induced cell death compared with TR1-IgG or recombinant TRAIL alone.Citation35 Therefore, the combination of TR1-IgMs with TRAIL or chemotherapeutics should be examined to determine whether they enhance the antitumor activity in non-sensitive cell lines.
Regarding the correlation between tumoricidal activity and TR1-IgM affinity, we prepared five clones of TR1-IgMs in this study ( and Fig. S2). Among them, TR1-IgM(J+) 422 showed the highest antitumor activity (EC50 = 0.5 ng/mL) and moderate affinity (1.4 × 10−8 M).Citation35 By contrast, TR1-IgM(J+) 404 showed approximately 10-fold higher affinity (1.5 × 10−9 M)Citation35 compared with TR1-IgM(J+) 422, but the EC50 of TR1-IgM(J+) 404 was approximately 20-fold lower (9 ng/mL) than that of TR1-IgM(J+) 422. These results suggest that the antitumor effect was not correlated with the IgM affinity. We previously analyzed the relative epitopes of the TR1-IgG antibodiesCitation57 and showed that the TR1-404 antibody and TRAIL competitively bound to TR1, but the TR1-422 antibody did not. Thus, the antitumor activity may depend on the epitope that is recognized by the individual antibodies. Taken together, we believe that TR1-IgMs may greatly contribute to the promotion of apoptosis for the clinical treatment of cancer.
Materials and methods
Production and purification of the antibodies
We cloned the J chain cDNA (GenBank accession number: NM_144646) and inserted it into a pcDNA3.4 expression vector (Life Technologies, Carlsbad, CA). We also amplified the antibody cDNAs encoding the VH of TR1-IgGsCitation35 or an anti-influenza IgG (control antibody)Citation33 and cloned them together with cDNA encoding the entire immunoglobulin constant region for IgM (GenBank accession number: X57086) into a pcDNA3.4 expression vector, respectively. We co-transfected Expi293FTM cells (Life Technologies, Carlsbad, CA) with the TR1-IgMs or control-IgM heavy chain and light chain vectors with or without the J chain expression vectors. We then collected the culture supernatants and purified the antibodies using HiTrap IgM Purification HP Columns (GE Healthcare, Marlborough, MA), according to the manufacturer’s instructions. We then conjugated biotin to the IgMs using the biotin Labeling Kit-NH2 (Dojindo, Kumamoto, Japan), according to the manufacturer’s instructions.
SDS-PAGE and western blotting analysis of the recombinant IgMs
We resolved the biotinylated IgMs on polyacrylamide-agarose composite gels under non-reducing conditions, as previously described.Citation58 Briefly, the gel was prepared using 3% acrylamide-N,N"diallyltartardiamide (29/1), 0.5% agarose, 0.1 M phosphate buffer (pH 7.0), 0.1% SDS, and 15% glycerol. The sample buffer contained 0.02 M phosphate buffer (pH 7.0), 30% glycerol, 1% SDS, and 0.1% bromophenol blue. The electrophoresis buffer contained 0.1 M phosphate buffer (pH 7.0) and 0.1% SDS. After electrophoresis, we transferred the proteins from the gel onto an Immobilon-P nylon membrane (Millipore, Bedford, MA). We treated the membrane with 0.04% skim milk and probed it with the antibodies. Visualization was performed using an ECL detection system (GE Healthcare, Marlborough, MA).
ELISA
We coated 96-well MaxiSorp plates (Nunc, Neptune, NJ) with 50 μL per well of either 1 μg/mL recombinant TRAIL-R1-Fc, TRAIL-R2-Fc, TRAIL-R3-Fc, TRAIL-R4-Fc, or OPG (R&D Systems, Inc., Minneapolis, MN) in PBS and subsequently blocked the plates with 3% bovine serum albumin (BSA) in PBS. After washing, we added 1 μg/mL of the antibodies to the plates and incubated them at room temperature (r.t.) for 1 h. We detected the antibodies that bound to the antigens using IgM-specific antibodies conjugated to alkaline phosphatase (Sigma, St. Louis, MO) and p-nitrophenylphosphate (Sigma, St. Louis, MO). We measured the optical absorbance at 405 nm with an ELISA reader (BMG LABTECH, Offenburg, Germany), according to the manufacturer’s instructions.
Flow cytometry analysis
Colo205, MCF7, DU145, and A549 cell lines were incubated with 5 μg/mL of biotinylated-IgM(J+)s for 20 min at r.t. The cells were then washed and stained with R-phycoerythrin (PE)-conjugated streptavidin for 20 min at r.t. After washing, the cells were analyzed with a FACSCanto flow cytometer (Becton Dickinson Biosciences, San Jose, CA). FlowJo 8.4.7 software (Tree Star, Inc.., Ashland, OR) was used for data analysis.
Cell viability assays
Colo205, DU145, A549, and MCF7 cell lines were cultured in 96-well white plates (Nunc, Neptune, NJ) (1×104 cells per well) and incubated with the indicated concentrations of antibodies at 37°C and 5% CO2 for 24 h. Cell viability was determined by CellTiter-Glo™ Luminescent Cell Viability Assay (Promega, San Luis Obispo, CA), according to the manufacturer’s instructions.
Caspase activity assays
The Colo205 cell line was cultured in 96-well white plates (1×104 cells per well) and incubated with the indicated concentration of antibodies at 37°C and 5% CO2 for 3 and 6 h to analyze the caspase-8 and caspase-3/7 activities, respectively. The caspase-8 and caspase-3/7 activities were measured using Caspase-Glo™ 8 Assays (Promega, San Luis Obispo, CA) and Caspase-Glo™ 3/7 Assays (Promega, San Luis Obispo, CA), respectively, according to the manufacturer’s instructions. The potency of caspase activation was compared with the levels in non-treated cells.
For the caspase inhibition experiments, Colo205 cells were cultured in 96-well white plates and incubated with or without the indicated concentrations of the pan-caspase inhibitor z-VAD-fmk (R&D Systems, Inc.., Minneapolis, MN) at 37°C and 5% CO2 for 4 h and then cultured with the indicated concentration of antibodies for 24 h in 5% CO2. Cell viability was determined as described above.
In vivo tumor models
The experiments using mice were approved by the Committee on Animal Experiments at the University of Toyama. Seven-week-old female severe combined immunodeficient (SCID) mice (C.B-17/IcrHsd-Prkdcscid; Sankyo Lab Service, Tokyo, Japan) were used. Colo205 cells (1×107) were subcutaneously (s.c.) inoculated in the lower right flank of the mouse. Treatment was initiated when the tumor volume reached ∼100 mm3, and 10 mice per group were used for each treatment group. TR1-IgM(J+) or control-IgM(J+) was administered to the animals i.v. via the tail vein in a dose/weight-matched fashion on the indicated days. The tumors were measured in the two largest dimensions, and the tumor volumes in mm3 were calculated using the following formula: tumor volume = length × width2 × 1/2. The experiments were concluded three weeks after the initial dosing.
Histological analysis of the xenograft tumors
The mice bearing the Colo205 cell-derived xenograft tumors were i.v.-treated with three doses of TR1-IgM(J+) 422 or control-IgM(J+). 2d after the antibody treatment, the mice were sacrificed, and the tumors were excised. The tumor tissues were placed into formalin for overnight fixation at r.t. The tissues were processed into paraffin blocks, and 4-μm-thick sections were stained with hematoxylin and eosin (Wako, Osaka, Japan).
Cleaved caspase-3 activity in xenograft tumors
The mice bearing the Colo205 cell-derived xenograft tumors were i.v. injected with a single dose of TR1-IgM(J+) or control-IgM(J+). 4h after treatment, the mice were sacrificed, and the tumors were excised. Single-cell suspensions were prepared using the Tumor Dissociation Kit, human (Miltenyi Biotec, Bergisch Gladbach, Germany), and the gentleMACS™Dissociator (Miltenyi Biotec, Bergisch Gladbach, Germany) according to the standard protocol provided with the kit. After fixation and permeabilization with IntraPrep™ (Beckman Coulter, Miami, FL), the single-cell suspensions were incubated with a rabbit anti-cleaved caspase-3 antibody (Cell Signaling Technology, Beverly, MA) for 20 min at r.t. The cells were then washed and stained with R-PE-conjugated streptavidin for 20 min at r.t. After washing, the cells were analyzed using a FACSCanto flow cytometer (BD Biosciences, Bedford, MA).
Disclosure of potential conflicts of interest
The ISAAC method is covered by patents that have been exclusively licensed to Valneva (Nantes, France).
Author contributions
X.P. and T.O. performed and analyzed the experiments. All authors designed the experiments. X.P., T.O., H.K., and A.M. wrote the manuscript.
KONI_A_1131380_s02.pptx
Download MS Power Point (216.4 KB)Acknowledgments
We thank Sanae Hirota for technical assistance and Kaoru Hata for secretarial work. We are very grateful to Masakiyo Sasahara and his colleagues, Department of Pathology, University of Toyama, for their generous helping of preparation and analysis of specimen.
Funding
This research was supported by grants from the Hokuriku Innovation Cluster for Health Science; the Platform for Drug Discovery, Informatics, and Structural Life Science from the Ministry of Education, Culture, Sports, Science and Technology, Japan.
References
- Steller H. Mechanisms and genes of cellular suicide. Science 1995; 267:1445-9; PMID:7878463; http://dx.doi.org/10.1126/science.7878-463
- Cryns V, Yuan J. Proteases to die for. Genes Dev 1998; 12:1551-70; PMID:9620844; http://dx.doi.org/10.1101/gad.12.11.1551
- Wiley SR, Schooley K, Smolak PJ, Din WS, Huang CP, Nicholl JK, Sutherland GR, Smith TD, Rauch C, Smith CA et al. Identification and characterization of a new member of the TNF family that induces apoptosis. Immunity 1995; 3:673-82; PMID:8777713; http://dx.doi.org/10.1016/1074-7613(95)90057-8
- Pitti RM, Marsters SA, Ruppert S, Donahue CJ, Moore A, Ashkenazi A. Induction of apoptosis by Apo-2 ligand, a new member of the tumor necrosis factor cytokine family. J Biol Chem 1996; 271:12687-90; PMID:8663110; http://dx.doi.org/10.1074/jbc.271.22.12687
- Kischkel FC, Hellbardt S, Behrmann I, Germer M, Pawlita M, Krammer PH, Peter ME. Cytotoxicity-dependent APO-1 (Fas/CD95)-associated proteins form a death-inducing signaling complex (DISC) with the receptor. EMBO J 1995; 14:5579-88; PMID:8521815
- Wallach D, Varfolomeev EE, Malinin NL, Goltsev YV, Kovalenko AV, Boldin MP. Tumor necrosis factor receptor and Fas signaling mechanisms. Annu Rev Immunol 1999; 17:331-67; PMID:10358762; http://dx.doi.org/10.1146/annurev.immunol.17.1.331
- Siegel RM, Muppidi JR, Sarker M, Lobito A, Jen M, Martin D, Straus SE, Lenardo MJ. SPOTS: signaling protein oligomeric transduction structures are early mediators of death receptor-induced apoptosis at the plasma membrane. J Cell Biol 2004; 167:735-44; PMID:15557123; http://dx.doi.org/10.1083/jcb.200406101
- Wilson NS, Dixit V, Ashkenazi A. Death receptor signal transducers: nodes of coordination in immune signaling networks. Nat Immunol 2009; 10:348-55; PMID:19295631; http://dx.doi.org/10.1038/ni.1714
- Degli-Esposti MA, Dougall WC, Smolak PJ, Waugh JY, Smith CA, Goodwin RG. The novel receptor TRAIL-R4 induces NF-kappaB and protects against TRAIL-mediated apoptosis, yet retains an incomplete death domain. Immunity 1997; 7:813-20; PMID:9430226; http://dx.doi.org/10.1016/S1074-7613(00)80399-4
- Degli-Esposti MA, Smolak PJ, Walczak H, Waugh J, Huang CP, DuBose RF, Goodwin RG, Smith CA. Cloning and characterization of TRAIL-R3, a novel member of the emerging TRAIL receptor family. J Exp Med 1997; 186:1165-70; PMID:9314565; http://dx.doi.org/10.1084/jem.186.7.1165
- Marsters SA, Sheridan JP, Pitti RM, Huang A, Skubatch M, Baldwin D, Yuan J, Gurney A, Goddard AD, Godowski P et al. A novel receptor for Apo2L/TRAIL contains a truncated death domain. Curr Biol 1997; 7:1003-6; PMID:9382840; http://dx.doi.org/10.1016/S0960-9822(06)00422-2
- Simonet WS, Lacey DL, Dunstan CR, Kelley M, Chang MS, Luthy R, Nguyen HQ, Wooden S, Bennett L, Boone T et al. Osteoprotegerin: a novel secreted protein involved in the regulation of bone density. Cell 1997; 89:309-19; PMID:9108485; http://dx.doi.org/10.1016/S0092-8674(00)80209-3
- Walczak H, Degli-Esposti MA, Johnson RS, Smolak PJ, Waugh JY, Boiani N, Timour MS, Gerhart MJ, Schooley KA, Smith CA et al. TRAIL-R2: a novel apoptosis-mediating receptor for TRAIL. EMBO J 1997; 16:5386-97; PMID:9311998; http://dx.doi.org/10.1093/emboj/16.17.5386
- Pan G, O'Rourke K, Chinnaiyan AM, Gentz R, Ebner R, Ni J, Dixit VM. The receptor for the cytotoxic ligand TRAIL. Science 1997; 276:111-3; PMID:9082980; http://dx.doi.org/10.1126/science.276.5309.111
- Walczak H, Miller RE, Ariail K, Gliniak B, Griffith TS, Kubin M, Chin W, Jones J, Woodward A, Le T et al. Tumoricidal activity of tumor necrosis factor-related apoptosis-inducing ligand in vivo. Nat Med 1999; 5:157-63; PMID:9930862; http://dx.doi.org/10.1038/5517
- Ashkenazi A. Targeting death and decoy receptors of the tumour-necrosis factor superfamily. Nat Rev Cancer 2002; 2:420-30; PMID:12189384; http://dx.doi.org/10.1038/nrc821
- Koschny R, Walczak H, Ganten TM. The promise of TRAIL–potential and risks of a novel anticancer therapy. J Mol Med (Berl) 2007; 85:923-35; PMID:17437073; http://dx.doi.org/10.1007/s00109-007-0194-1
- Kelley SK, Harris LA, Xie D, Deforge L, Totpal K, Bussiere J, Fox JA. Preclinical studies to predict the disposition of Apo2L/tumor necrosis factor-related apoptosis-inducing ligand in humans: characterization of in vivo efficacy, pharmacokinetics, and safety. J Pharmacol Exp Ther 2001; 299:31-8; PMID:11561060
- Chan FK, Chun HJ, Zheng L, Siegel RM, Bui KL, Lenardo MJ. A domain in TNF receptors that mediates ligand-independent receptor assembly and signaling. Science 2000; 288:2351-4; PMID:10875917; http://dx.doi.org/10.1126/science.288.5475.2351
- Hymowitz SG, O'Connell MP, Ultsch MH, Hurst A, Totpal K, Ashkenazi A, de Vos AM, Kelley RF. A unique zinc-binding site revealed by a high-resolution X-ray structure of homotrimeric Apo2L/TRAIL. Biochemistry 2000; 39:633-40; PMID:10651627; http://dx.doi.org/10.1021/bi992242l
- Kelley RF, Totpal K, Lindstrom SH, Mathieu M, Billeci K, Deforge L, Pai R, Hymowitz SG, Ashkenazi A. Receptor-selective mutants of apoptosis-inducing ligand 2/tumor necrosis factor-related apoptosis-inducing ligand reveal a greater contribution of death receptor (DR) 5 than DR4 to apoptosis signaling. J Biol Chem 2005; 280:2205-12; PMID:15520016; http://dx.doi.org/10.1074/jbc.M410660200
- Pukac L, Kanakaraj P, Humphreys R, Alderson R, Bloom M, Sung C, Riccobene T, Johnson R, Fiscella M, Mahoney A et al. HGS-ETR1, a fully human TRAIL-receptor 1 monoclonal antibody, induces cell death in multiple tumour types in vitro and in vivo. Br J Cancer 2005; 92:1430-41; PMID:15846298; http://dx.doi.org/10.1038/sj.bjc.6602487
- Li J, Knee DA, Wang YZ, Zhang QX, Johnson JA, Cheng J, He H, Miller C, Li ZF, Kowal C et al. LBY135, a novel anti-DR5 agonistic antibody induces tumor cell-specific cytotoxic activity in human colon tumor cell lines and xenografts. Drug Dev Res 2008; 69:69-82; http://dx.doi.org/10.1002/ddr.20229
- Yada A, Yazawa M, Ishida S, Yoshida H, Ichikawa K, Kurakata S, Fujiwara K. A novel humanized anti-human death receptor 5 antibody CS-1008 induces apoptosis in tumor cells without toxicity in hepatocytes. Ann Oncol 2008; 19:1060-7; PMID:18304965; http://dx.doi.org/10.1093/annonc/mdn015
- Qiu Y, Zhang Z, Shi J, Liu S, Liu Y, Zheng D. A novel anti-DR5 chimeric antibody and epirubicin synergistically suppress tumor growth. IUBMB Life 2012; 64:757-65; PMID:22815059; http://dx.doi.org/10.1002/iub.1064
- Soria JC, Mark Z, Zatloukal P, Szima B, Albert I, Juhasz E, Pujol JL, Kozielski J, Baker N, Smethurst D et al. Randomized phase II study of dulanermin in combination with paclitaxel, carboplatin, and bevacizumab in advanced non-small-cell lung cancer. J Clin Oncol 2011; 29:4442-51; PMID:22010015; http://dx.doi.org/10.1200/JCO.2011.37.2623
- Trarbach T, Moehler M, Heinemann V, Kohne CH, Przyborek M, Schulz C, Sneller V, Gallant G, Kanzler S. Phase II trial of mapatumumab, a fully human agonistic monoclonal antibody that targets and activates the tumour necrosis factor apoptosis-inducing ligand receptor-1 (TRAIL-R1), in patients with refractory colorectal cancer. Br J Cancer 2010; 102:506-12; PMID:20068564; http://dx.doi.org/10.1038/sj.bjc.6605507
- Gieffers C, Kluge M, Merz C, Sykora J, Thiemann M, Schaal R, Fischer C, Branschadel M, Abhari BA, Hohenberger P et al. APG350 induces superior clustering of TRAIL receptors and shows therapeutic antitumor efficacy independent of cross-linking via Fcgamma receptors. Mol Cancer Ther 2013; 12:2735-47; PMID:24101228; http://dx.doi.org/10.1158/1535-7163.MCT-13-0323
- Hwang WY, Foote J. Immunogenicity of engineered antibodies. Methods 2005; 36:3-10; PMID:15848070; http://dx.doi.org/10.1016/j.ymeth.2005.01.001
- Mom CH, Verweij J, Oldenhuis CNAM, Gietema JA, Fox NL, Miceli R, Eskens FALM, Loos WJ, de Vries EGE, Sleijfer S. Mapatumumab, a Fully Human Agonistic Monoclonal Antibody That Targets TRAIL-R1, in Combination with Gemcitabine and Cisplatin: a Phase I Study. Clin Cancer Res 2009; 15:5584-90; PMID:19690193; http://dx.doi.org/10.1158/1078-0432.CCR-09-0996
- Greco FA, Bonomi P, Crawford J, Kelly K, Oh Y, Halpern W, Lo L, Gallant G, Klein J. Phase 2 study of mapatumumab, a fully human agonistic monoclonal antibody which targets and activates the TRAIL receptor-1, in patients with advanced non-small cell lung cancer. Lung Cancer 2008; 61:82-90; PMID:18255187; http://dx.doi.org/10.1016/j.lungcan.2007.12.011
- von Pawel J, Harvey JH, Spigel DR, Dediu M, Reck M, Cebotaru CL, Humphreys RC, Gribbin MJ, Fox NL, Camidge DR. Phase II trial of mapatumumab, a fully human agonist monoclonal antibody to tumor necrosis factor-related apoptosis-inducing ligand receptor 1 (TRAIL-R1), in combination with paclitaxel and carboplatin in patients with advanced non-small-cell lung cancer. Clin Lung Cancer 2014; 15:188-96 e2; PMID:24560012; http://dx.doi.org/10.1016/j.cllc.2013.12.005
- Jin A, Ozawa T, Tajiri K, Obata T, Kondo S, Kinoshita K, Kadowaki S, Takahashi K, Sugiyama T, Kishi H et al. A rapid and efficient single-cell manipulation method for screening antigen-specific antibody-secreting cells from human peripheral blood. Nat Med 2009; 15:1088-92; PMID:19684583; http://dx.doi.org/10.1038/nm.1966
- Ishida I, Tomizuka K, Yoshida H, Tahara T, Takahashi N, Ohguma A, Tanaka S, Umehashi M, Maeda H, Nozaki C et al. Production of human monoclonal and polyclonal antibodies in TransChromo animals. Cloning Stem Cells 2002; 4:91-102; PMID:12006160; http://dx.doi.org/10.1089/153623002753632084
- Jin A, Ozawa T, Tajiri K, Lin Z, Obata T, Ishida I, Kishi H, Muraguchi A. Generation of TRAIL-receptor 1-specific human monoclonal Ab by a combination of immunospot array assay on a chip and human Ab-producing mice. Eur J Immunol 2010; 40:3591-3; PMID:21072874; http://dx.doi.org/10.1002/eji.201040551
- Baumgarth N. Secreted IgM versus BLyS in germinal center formation. Nat Immunol 2000; 1:179; PMID:10973267; http://dx.doi.org/10.1038/79708
- Vollmers HP, Brandlein S. Natural antibodies and cancer. N Biotechnol 2009; 25:294-8; PMID:19442595; http://dx.doi.org/10.1016/j.nbt.2009.03.016
- Eskeland T, Christensen TB. IgM molecules with and without J chain in serum and after purification, studied by ultracentrifugation, electrophoresis, and electron microscopy. Scandinavian J Immunol 1975; 4:217-28; PMID:807966; http://dx.doi.org/10.1111/j.1365-3083.1975.tb02620.x
- Kunert R, Wolbank S, Stiegler G, Weik R, Katinger H. Characterization of molecular features, antigen-binding, and in vitro properties of IgG and IgM variants of 4E10, an anti-HIV type 1 neutralizing monoclonal antibody. AIDS Res Hum Retroviruses 2004; 20:755-62; PMID:15307922; http://dx.doi.org/10.1089/088-9222041524571
- Wolbank S, Kunert R, Stiegler G, Katinger H. Characterization of human class-switched polymeric (immunoglobulin M [IgM] and IgA) anti-human immunodeficiency virus type 1 antibodies 2F5 and 2G12. J Virol 2003; 77:4095-103; PMID:12634368; http://dx.doi.org/10.1128/JVI.77.7.4095-4103.2003
- Wiersma EJ, Collins C, Fazel S, Shulman MJ. Structural and functional analysis of J chain-deficient IgM. J Immunol 1998; 160:5979-89 ; PMID:9637512
- Strater J, Walczak H, Pukrop T, Von Muller L, Hasel C, Kornmann M, Mertens T, Moller P. TRAIL and its receptors in the colonic epithelium: a putative role in the defense of viral infections. Gastroenterology 2002; 122:659-66; PMID:11874999; http://dx.doi.org/10.1053/gast.2002.31889
- Chaudhary KS, Abel PD, Stamp GW, Lalani E. Differential expression of cell death regulators in response to thapsigargin and adriamycin in Bcl-2 transfected DU145 prostatic cancer cells. J Pathol 2001; 193:522-9; PMID:11276013; http://dx.doi.org/10.1002/1096-9896(2000)9999:9999%3c::AID-PATH821%3e3.0.CO;2-Y
- Shankar S, Srivastava RK. Enhancement of therapeutic potential of TRAIL by cancer chemotherapy and irradiation: mechanisms and clinical implications. Drug Resist Updat 2004; 7:139-56; PMID:15158769; http://dx.doi.org/10.1016/j.drup.2004.03.002
- Sanlioglu AD, Dirice E, Aydin C, Erin N, Koksoy S, Sanlioglu S. Surface TRAIL decoy receptor-4 expression is correlated with TRAIL resistance in MCF7 breast cancer cells. BMC cancer 2005; 5:54; PMID:15916713; http://dx.doi.org/10.1186/1471-2407-5-54
- Herbeuval JP, Lambert C, Sabido O, Cottier M, Fournel P, Dy M, Genin C. Macrophages from cancer patients: analysis of TRAIL, TRAIL receptors, and colon tumor cell apoptosis. J Natl Cancer Inst 2003; 95:611-21; PMID:12697854; http://dx.doi.org/10.1093/jnci/95.8.611
- Petrusic V, Zivkovic I, Stojanovic M, Stojicevic I, Marinkovic E, Inic-Kanada A, Dimitijevic L. Antigenic specificity and expression of a natural idiotope on human pentameric and hexameric IgM polymers. Immunol Res 2011; 51:97-107; PMID:21786026; http://dx.doi.org/10.1007/s12026-011-8236-8
- Sliwkowski MX, Mellman I. Antibody therapeutics in cancer. Science 2013; 341:1192-8; PMID:24031011; http://dx.doi.org/10.1126/science.1241145
- Tchoudakova A, Hensel F, Murillo A, Eng B, Foley M, Smith L, Schoenen F, Hildebrand A, Kelter AR, Ilag LL et al. High level expression of functional human IgMs in human PER.C6 cells. MAbs 2009; 1:163-71; PMID:20061826; http://dx.doi.org/10.4161/mabs.1.2.7945
- Lobo ED, Hansen RJ, Balthasar JP. Antibody pharmacokinetics and pharmacodynamics. J Pharmaceutical Sci 2004; 93:2645-68; PMID:15389672; http://dx.doi.org/10.1002/jps.20178
- Adams C, Totpal K, Lawrence D, Marsters S, Pitti R, Yee S, Ross S, Deforge L, Koeppen H, Sagolla M et al. Structural and functional analysis of the interaction between the agonistic monoclonal antibody Apomab and the proapoptotic receptor DR5. Cell Death Differ 2008; 15:751-61; PMID:18219321; http://dx.doi.org/10.1038/sj.cdd.4402306
- Kaplan-Lefko PJ, Graves JD, Zoog SJ, Pan Y, Wall J, Branstetter DG, Moriguchi J, Coxon A, Huard JN, Xu R et al. Conatumumab, a fully human agonist antibody to death receptor 5, induces apoptosis via caspase activation in multiple tumor types. Cancer Biol Therapy 2010; 9:618-31; PMID:20150762; http://dx.doi.org/10.4161/cbt.9.8.11264
- Shankar S, Chen X, Srivastava RK. Effects of sequential treatments with chemotherapeutic drugs followed by TRAIL on prostate cancer in vitro and in vivo. Prostate 2005; 62:165-86; PMID:15389801; http://dx.doi.org/10.1002/pros.20126
- Clayer M, Bouralexis S, Evdokiou A, Hay S, Atkins GJ, Findlay DM. Enhanced apoptosis of soft tissue sarcoma cells with chemotherapy: A potential new approach using TRAIL. J Orthop Surg 2001; 9:19-22; PMID:12118126
- LeBlanc H, Lawrence D, Varfolomeev E, Totpal K, Morlan J, Schow P, Fong S, Schwall R, Sinicropi D, Ashkenazi A. Tumor-cell resistance to death receptor–induced apoptosis through mutational inactivation of the proapoptotic Bcl-2 homolog Bax. Nat Med 2002; 8:274-81; PMID:11875499; http://dx.doi.org/10.1038/nm0302-274
- Tuthill MH, Montinaro A, Zinngrebe J, Prieske K, Draber P, Prieske S, Newsom-Davis T, von Karstedt S, Graves J, Walczak H. TRAIL-R2-specific antibodies and recombinant TRAIL can synergise to kill cancer cells. Oncogene 2015; 34:2138-44; PMID:24909167; http://dx.doi.org/10.1038/onc.2014.156
- Lin ZH, Jin A, Ozawa T, Tajiri K, Obata T, Ishida I, Jin F, Kishi H, Muraguchi A. Post-translational modification of TRAIL receptor type 1 on various tumor cells and the susceptibility of tumors to TRAIL-induced apoptosis. Biochem Biophys Res Commun 2010; 395:251-7; PMID:20363213; http://dx.doi.org/10.1016/j.bbrc.2010.03.175
- Sorensen V, Rasmussen IB, Norderhaug L, Natvig I, Michaelsen TE, Sandlie I. Effect of the IgM and IgA secretory tailpieces on polymerization and secretion of IgM and IgG. J Immunol 1996; 156:2858-65; PMID:8609405