ABSTRACT
Attenuated measles virus (MV) is currently being evaluated in clinical trials as an oncolytic therapeutic agent. Originally used for its lytic activity against tumor cells, it is now admitted that the effectiveness of MV also lies in its ability to initiate antitumor immune responses through the activation of dendritic cells (DCs). In this study, we investigated the capacity of oncolytic MV to convert human blood myeloid CD1c+ DCs and plasmacytoid DCs (pDCs) into cytotoxic effectors. We found that MV induces the expression of the cytotoxic protein TNF-related apoptosis-inducing ligand (TRAIL) on the surface of DCs. We demonstrate that the secretion of interferon-α (IFN-α) by DCs in response to MV is responsible for this TRAIL expression. Several types of PRRs (pattern recognition receptors) have been implicated in MV genome recognition, including RLRs (RIG-I-like receptors) and TLRs (Toll-like receptors). We showed that CD1c+ DCs secrete modest amounts of IFN-α and express TRAIL in an RLR-dependent manner upon exposure to MV. In pDCs, MV is recognized by RLRs and also by TLR7, leading to the secretion of high amounts of IFN-α and TRAIL expression. Finally, we showed that MV-stimulated DCs induce TRAIL-mediated cell death of Jurkat cells, confirming their acquisition of cytotoxic functions. Our results demonstrate that MV can activate cytotoxic myeloid CD1c+ DCs and pDCs, which may participate to the antitumor immune response.
Abbreviations
APC | = | antigen-presenting cells |
BMDM | = | bone marrow-derived macrophages |
DC | = | dendritic cells |
dsRNA | = | double-stranded RNA |
DR5 | = | death receptor 5 |
HIV | = | human immunodeficiency virus |
IFN | = | interferon |
IFNAR | = | IFN-α/β receptor |
IKK | = | IκB kinases |
IRF | = | IFN regulatory factor |
ISG | = | IFN-stimulated genes |
JAK | = | Janus kinases |
MAVS | = | mitochondrial antiviral signaling |
Mda5 | = | melanoma differentiation-associated protein |
Mo-DC | = | monocyte-derived DC |
MOI | = | multiplicity of infection |
MV | = | measles virus |
NF-κB | = | nuclear factor kappa-light-chain enhancer of activated B cells |
pDC | = | plasmacytoid DC |
PRR | = | pattern recognition receptors |
RIG-I | = | retinoic acid-inducible gene I |
RLR | = | RIG-I-like receptors |
shRNA | = | short-hairpin RNA |
ssRNA | = | single-stranded RNA |
TBK1 | = | TANK-binding kinase 1 |
TLR | = | toll-like receptors |
TRAF3 | = | tumor necrosis factor (TNF)-receptor-associated factor 3 |
TRAIL | = | TNF-related apoptosis-inducing ligand |
UV | = | ultraviolet |
Introduction
Among the viruses currently evaluated for their antitumor properties, attenuated strains of measles virus (MV) display a potent and spontaneous oncolytic activity against a wide variety of cancers.Citation1 Indeed, MV Schwarz and Edmonston live-attenuated vaccine strains preferentially infect and kill tumor cells, which often overexpress CD46, the major receptor for attenuated MV, and present defects in the antiviral innate immune response.Citation2,3 Phase I clinical trials using MV Edmonston were conducted against cutaneous T cell lymphoma,Citation4 ovarian cancer,Citation5,6 and disseminated multiple myeloma,Citation7 and encouraging clinical benefits were observed. Although the effectiveness of oncolytic viruses, including MV, was originally thought to be mainly based on their lytic activity against tumor cells, growing evidence now points out their ability to initiate systemic antitumor immune responses.Citation6,8 They are therefore considered as new immunotherapeutic agents against cancer.Citation9
Oncolytic MV can stimulate antitumor immune responses through its ability to stimulate dendritic cells (DCs).Citation10–12 DCs are antigen-presenting cells (APCs) that play a central role in the establishment of immune responses notably by inducing antigen-specific T cell responses. In humans, numerous DC subsets have been characterized, based on their phenotype, localization and function.Citation13 In the blood, three main DC subsets are found: the myeloid CD141+ DCs and CD1c+ DCs, and plasmacytoid dendritic cells (pDCs), which are specialized in rapid and massive secretion of type I IFNs in response to viral nucleic acids, in addition to displaying APC functions.Citation14 DCs are also able to exert cytotoxic activity after appropriate activation.Citation15 Indeed, the expression of the TRAIL (TNF-related apoptosis-inducing ligand) protein on the surface of DCs elicits the death of target cells that express TRAIL receptors, such as tumor cells and cells infected by viruses.Citation16,17 Mo-DCs (monocyte-derived DCs) infected with the wild-type strain of MV,Citation18,19 pDCs exposed to inactivated influenza virusCitation20 or pDCs from HIV (human immunodeficiency virus)-infected patientsCitation21 express TRAIL after secreting IFN-α and are responsible for the lysis of TRAIL-sensitive cells. In addition, exposure of pDCs in vitro to TLR7/8 and TLR9 agonists endows them with a tumoricidal activity that relies on their TRAIL expression.Citation20,22,23
Several types of PRRs (pattern recognition receptors) are found in DCs, such as the ubiquitously expressed RLRs (RIG-I-like receptors) or the TLRs (toll-like receptors) whose expression is more restricted. These receptors allow DCs to recognize invading pathogens.Citation24 Among the PRRs, the cytosolic RLRs RIG-I (retinoic acid-inducible gene I) and Mda5 (melanoma differentiation-associated protein 5) are able to recognize MV replication intermediates.Citation25,26 After activation of these RLRs, the signal transduces through MAVS (mitochondrial antiviral signaling) proteins, allowing the activation of TBK-1 (TANK-binding kinase 1) and different IKKs (IκB kinases, IKK-α, -β and -ϵ), responsible for the activation of the transcription factors IRF-3 and −7 (IFN regulatory factor) and NF-κB (nuclear factor kappa-light-chain enhancer of activated B cells).Citation27 These activated transcription factors drive the expression of genes encoding for type I IFNs, as well as other pro-inflammatory cytokines and antiviral proteins. Stimulation of pDCs can also occur via recognition of incoming viral ssRNA by TLR7 in the endosomal/lysosomal compartment.Citation12 After the detection of viral ssRNA by TLR7, the MyD88 pathway induces the activation of NF-κB and IRF-7 that leads to the secretion of large amounts of IFN-α.Citation24
We have previously shown that MV-infected tumor cells induce a strong IFN-α secretion by pDCs.Citation12 Furthermore, wild-type MV is able to generate cytotoxic Mo-DCs.Citation18 In this study, we addressed the in vitro ability of MV Schwarz to induce cytotoxic functions in human blood pDCs and CD1c+ DCs. We show that upon exposure to MV, pDCs and CD1c+ DCs secrete IFN-α and express TRAIL on their surface. We then analyzed the sensors involved in the recognition of MV by DCs. Our data suggest that in pDCs, both TLR7 and RLR pathways are activated and responsible for the secretion of massive amounts of IFN-α and the subsequent expression of TRAIL. On the other hand, in CD1c+ DCs, MV triggers only the RLR signaling pathway, resulting in the production of a modest amount of IFN-α that is sufficient to induce TRAIL expression. Using the pDC-like cell line Gen2.2, we confirmed the ability of RIG-I to sense MV dsRNA. We finally assessed the functional cytotoxic activity of these two subsets of DCs against TRAIL-sensitive Jurkat cells. Our results showed that MV-activated pDCs and CD1c+ DCs become efficient cytotoxic effectors that can cause lysis of TRAIL-sensitive cells.
Results
MV induces TRAIL expression and IFN-α secretion by pDCs and CD1c+ DCs
First, we exposed pDCs and CD1c+ DCs to the virus at a MOI (multiplicity of infection) of 10 and assessed expression of the maturation marker CD83, the expression of TRAIL and the secretion of IFN-α. pDCs expressed CD83 and TRAIL on their surface after exposure to MV and IL3, compared with pDCs cultured with the survival factor IL3 alone (). As previously reported,Citation12 MV also induced the secretion of large amounts of IFN-α by pDCs (). pDC activation can also be reached without IL3 when a higher MOI of MV is used (Fig. S1). Like pDCs, myeloid CD1c+ DCs expressed CD83 and TRAIL after exposure to MV, but they secreted around 250 times less IFN-α than pDCs (). The two subsets of DCs responded differently to the TLR7-specific agonist R837. R837-treated pDCs expressed CD83, TRAIL and secreted IFN-α, although to a lesser extent than in response to MV (6-fold difference) (). CD1c+ DCs exposed to R837 also expressed the maturation marker CD83, but only around 5% of cells expressed TRAIL. Moreover, they failed to secrete detectable amounts of IFN-α (). We also measured the expression of two other markers involved in the cytotoxic activity: granzyme B and perforin. Neither pDCs nor CD1c+ DCs secreted perforin in response to MV and R837 (data not shown). Granzyme B was secreted by pDCs exposed to IL3 or to MV in presence of IL3 (Fig. S2), but not by CD1c+ DCs (data not shown).
Figure 1. MV induces TRAIL expression and IFN-α secretion by pDCs and CD1c+ DCs. pDCs were cultured with IL3, IL3+MV or the TLR7 agonist R837 (A). CD1c+ DCs were cultured alone (−), with MV or R837 (B). The expression of surface markers by the indicated cells was determined by flow cytometry. IFN-α secretion was measured by ELISA. #, values are below the limit of detection of the kit (7 pg/mL). Results are expressed as the mean ± SEM of three independent experiments. *p < 0.05, one-way ANOVA (Kruskal–Wallis).
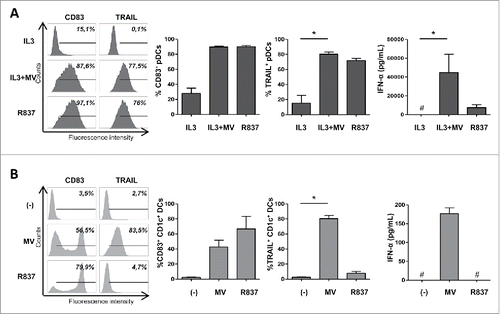
We have previously shown that oncolytic MV Schwarz induces immunogenic death of tumor cells, compared with UV-irradiation.Citation10,12 We thus analyzed whether pDCs and CD1c+ DCs expressed TRAIL in response to MV-infected tumor cells compared with UV-irradiated tumor cells. We observed TRAIL expression by pDCs and CD1c+ DCs only when they were co-cultured with MV-infected tumor cells (). We confirmed that TRAIL expression on DCs is not due to the tumor cells themselves because there is no IFN-α secretion neither TRAIL expression by DCs when they are exposed to non-infected tumor cells (Fig. S3). These results suggest that MV is able to trigger TRAIL expression on both subsets of DCs in the context of tumor cell infection.
Figure 2. MV-infected tumor cells induce TRAIL expression on pDCs and CD1c+ DCs. pDCs were cultured with IL3 or with MV-infected or UV-irradiated M18 melanoma cells (A). CD1c+ DCs were cultured alone (−) or with MV-infected or UV-irradiated M18 melanoma cells (B). The expression of TRAIL by the indicated cells was determined by flow cytometry. Results are expressed as the mean ± SEM of three independent experiments.
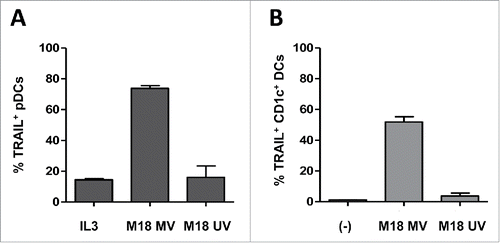
TRAIL expression depends on IFN-α secreted by DCs exposed to MV
The gene encoding TRAIL is an interferon-stimulated gene (ISG) whose expression is type I IFNs-dependent in DCs.Citation28 Thus, we investigated whether TRAIL expression is affected by the pretreatment of pDCs and CD1c+ DCs with Ruxolitinib, an inhibitor of the Janus kinases JAK1 and 2 implicated in the IFNAR (IFN-α/β Receptor) signaling pathway. IFN-α secretion and TRAIL expression by pDCs in response to MV or R837 was reduced after pretreatment with Ruxolitinib (). Similarly, Ruxolitinib inhibited the secretion of IFN-α and the expression of TRAIL on CD1c+ DCs exposed to MV (). Moreover, treatment with type I IFNs induced the expression of TRAIL by pDCs and CD1c+ DCs (), confirming that TRAIL is an ISG. Treatment of these two types of DCs with Ruxolitinib prior to type I IFNs exposure reduced TRAIL expression (). These results indicate that MV-mediated TRAIL expression on pDCs and CD1c+ DCs is dependent on type I IFNs signaling.
Figure 3. TRAIL expression depends on IFN-α secreted by DCs exposed to MV. pDCs (A) and CD1c+ DCs (B) were pretreated or not with Ruxolitinib (Rux) before exposure to IL3+MV or MV respectively, or before exposure to R837. The secretion of IFN-α was measured by ELISA. #, values are below the limit of detection of the kit (7 pg/mL). The expression of TRAIL by the indicated cells was determined by flow cytometry. (C) pDCs and CD1c+ DCs were pretreated or not with Rux before exposure to type I IFNs (rhIFN-α-2a and rhIFN-β-1a). TRAIL positive cells were quantified by flow cytometry. Results are expressed as the mean ± SEM of three independent experiments. *p < 0.05, Mann–Whitney test.
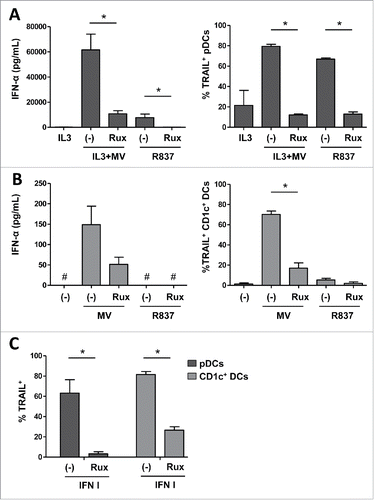
MV induces IFN-α and TRAIL expression following RLR activation in CD1c+ DCs and RLR/TLR7 activation in pDCs
To identify the sensor(s) responsible for MV recognition in pDCs and CD1c+ DCs, and subsequent IFN-α secretion and TRAIL expression, we used two inhibitors: IRS661, a specific inhibitor of TLR729, and MRT67307, an inhibitor of the kinases TBK1 and IKK-ϵ that transduce the signal from the RLRs RIG-I and Mda5.Citation30 Pretreatment with IRS661 of pDCs exposed to MV reduced the secretion of IFN-α by more than 20-folds (). However, the expression of TRAIL induced by MV was not modified by IRS661, possibly due to high residual amounts of IFN-α still produced in the presence of the drug. In contrast, IRS661 was able to completely prevent both R837-mediated IFN-α production and TRAIL expression, confirming the specificity of the inhibitor. These results suggest that MV is not detected only by TLR7 but at least by one other sensor in pDCs.
Figure 4. MV induces IFN-α and TRAIL expression following RLR activation in CD1c+ DCs and RLR/TLR7 activation in pDCs. (A) pDCs were pretreated or not with IRS661 (TLR7 inhibitor) or with MRT67307 (TBK1 and IKK-ϵ inhibitor) and then cultured with IL3, IL3+MV or R837. (B) CD1c+ DCs were pretreated or not with IRS661 or MRT67307 and then cultured alone (−) or with MV. (C) pDCs were cultured with IL3+MV or with IL3+UV-inactivated MV (MV UV*) and CD1c+ DCs were cultured alone (−), with MV or MV UV*. (D) pDCs and CD1c+ DCs were exposed to 5′-ppp-dsRNA LyoVec, a RIG-I agonist. (A–D) IFN-α secretion was measured by ELISA. #, values are below the limit of detection of the kit (7 pg/mL). The expression of TRAIL by the indicated cells was determined by flow cytometry. Results are expressed as the mean ± SEM of three independent experiments. (A, B) *p < 0.05, one-way ANOVA (Kruskal–Wallis). (C) *p < 0.05, Mann–Whitney test.
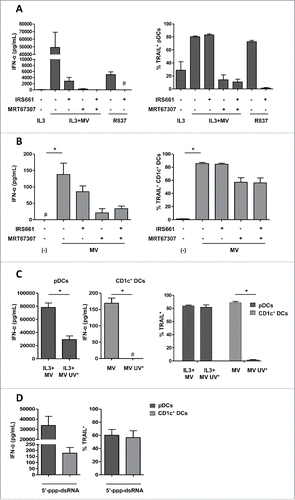
We therefore assessed the effect of the RLR pathway inhibitor MRT67307 in combination with the TLR7 inhibitor IRS661 on MV-mediated pDCs activation. The two inhibitors completely abolished the secretion of IFN-α and greatly diminished the expression of TRAIL by pDCs exposed to MV, suggesting that both signaling pathways are involved in sensing MV (). However, we noted that MRT67307 alone inhibited IFN-α secretion and TRAIL expression as efficiently as in combination with IRS661. We therefore wonder whether MRT67307 also interferes with TLR7 or type I IFN signaling pathways and performed additional experiments to test this hypothesis. Surprisingly, R837 and type I IFNs (IFN-α and IFN-β) failed to activate pDCs when they were pretreated with MRT67307, suggesting that the drug has off-target effects (Figs. S4A and B).
We performed similar experiments on CD1c+ DCs. We observed that the pretreatment with the TLR7 inhibitor IRS661 scarcely affected the secretion of IFN-α by CD1c+ DCs exposed to MV and did not affect TRAIL expression (). These results suggest that TLR7 does not play a major role in the recognition of MV in CD1c+ DCs. The RLRs inhibitor MRT67307 reduced by 6-folds the secretion of IFN-α and reduced by 25% the fraction of CD1c+ DCs that express TRAIL (). No synergy was observed between the two inhibitors IRS661 and MRT67307. However, MRT67307 also exhibited off-target effect on CD1c+ DCs by affecting type I IFN signaling (data not shown).
Even though the results obtained with cells treated with MRT67307 did not allow us to conclude about a possible implication of the RLR signaling in MV-mediated pDCs and CD1c+ DCs activation, they suggest that TLR7 is not the unique detector of MV in pDCs and is not implicated in CD1c+ DCs to induce IFN-α secretion and TRAIL expression.
To gather evidences that MV RNA can be detected by RLRs in DCs, we exposed them to UV-inactivated MV. UV irradiation impairs the replication of the virus and the formation of replication intermediates, thus inhibiting the recognition of replicative viral RNA by RLRs. By contrast, the UV-inactivated MV should be recognized by TLR7 since viral replication is not required for this pathway.Citation31,32 pDCs exposed to UV-inactivated MV secreted 2.5 times less IFN-α than pDCs exposed to MV (). This result indicates that viral replication contributes to IFN-α production, reinforcing the idea that RLRs participate in the detection of MV in pDCs. However, pDCs exposed to UV-inactivated virus express similar amounts of TRAIL compared with pDCs exposed to live virus (), possibly because the UV-inactivated MV was still able to induce the secretion of IFN-α by pDCs through TLR7. In contrast, UV-inactivated MV failed to induce IFN-α secretion and TRAIL expression by CD1c+ DCs (). This result confirms that MV replication is necessary in CD1c+ DCs to induce IFN-α secretion and TRAIL expression through the RLR pathway.
To demonstrate that the activation of cytosolic RLRs can lead to the stimulation of both pDCs and CD1c+ DCs, we treated DCs with the synthetic RIG-I agonist 5′-ppp-dsRNA LyoVec (5′-triphosphate-double-stranded RNA) and measured IFN-α secretion and TRAIL expression. We showed that the 5′-ppp-dsRNA induced the secretion of IFN-α by pDCs and CD1c+ DCs (). The production of IFN-α was associated with the expression of TRAIL on both DCs subsets.
Together, these results suggest that the secretion of IFN-α and the subsequent TRAIL expression depend on the recognition of MV by both TLR7 and the cytosolic RLRs in pDCs, whereas only the RLRs are involved in sensing MV in CD1c+ DCs.
MV induces IFN-α and TRAIL expression after RIG-I activation in Gen2.2 cells
To explore further the identity of the sensor(s) involved in the recognition of MV by pDCs, silencing experiments were conducted in Gen2.2 cells, which are reliable surrogate for pDCs.Citation20 These cells can be efficiently transduced using lentiviral particles.Citation33 Stable shRNA-silencing reduced the level of RIG-I and TLR7 expression by around 80% and 60%, respectively, in Gen2.2 cells when compared with shScrambled control cells (Fig. S5). As previously described,Citation34–36 Gen2.2 cells were less potent producers of type I IFNs than pDCs (). The amount of IFN-α and TRAIL expression in response to MV were reduced in the Gen2.2 cells stably expressing RIG-I shRNA (shRIG-I Gen2.2) compared with the cells expressing scrambled control shRNA (shScrambled Gen2.2) or TLR7 shRNA (shTLR7 Gen2.2) (). This result suggests that RIG-I is involved in the recognition of MV in Gen2.2 cells. Furthermore, Gen2.2 cells were not able to secrete IFN-α after exposure to the UV-inactivated MV (). It is possible that small amounts of IFN-α were secreted but fell under the detection limit of the assay. TRAIL expression was reduced upon exposure to UV-inactivated virus compared with live virus (), further confirming the role of RLRs in MV recognition in pDCs. No involvement of TLR7 in MV-mediated IFN-α production and TRAIL expression in Gen2.2 cells was found (), suggesting that in this model, RIG-I is the primary sensor for MV.
Figure 5. MV induces IFN-α and TRAIL expression after RIG-I activation in Gen2.2 cells. Gen2.2 cells established by transduction with lentiviruses expressing scrambled shRNA, shRNA against TLR7 (shTLR7) or RIG-I (shRIG-I) were cultured with IL3, IL3+MV or IL3+UV-inactivated MV (MV UV*). IFN-α secretion was measured by ELISA. #, values are below the limit of detection of the kit (7 pg/mL).The percentage of TRAIL positive Gen2.2 cells was determined by flow cytometry. Results are expressed as the mean ± SEM of three independent experiments. *p < 0.05, one-way ANOVA (Kruskal–Wallis).
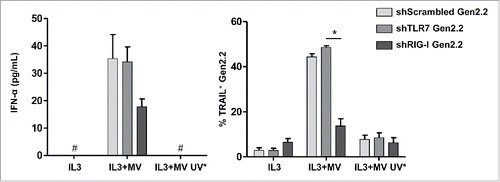
DCs activated with MV acquire a functional cytotoxic activity
To further characterize the cytotoxic activity of the TRAIL+ DCs, we performed a cytotoxic assay with Jurkat cells as target cells, as they express the TRAIL receptor DR5 (death receptor 5) and are sensitive to TRAIL-dependent cell death.Citation22
At an effector:target ratio of 20:1, we detected that 18.1% of Jurkat cells were specifically lyzed by pDCs exposed to MV (). This specific cell death was reduced when pDCs were pretreated with Ruxolitinib, but not with IRS661. Thus, the specific lysis of Jurkat cells by pDCs correlated with TRAIL expression (). After stimulation with the TLR7 agonist R837, pDCs were able to kill 21% of Jurkat cells. Moreover, pDCs acquired a functional cytotoxic activity against Jurkat cells after activation with exogenous type I IFNs, which was abolished by the specific inhibitor Ruxolitinib, or after activation with the RIG-I agonist 5′-ppp-dsRNA.
Figure 6. DCs activated with MV acquire a functional cytotoxic activity. (A) pDCs were pretreated or not with IRS661 or Rux and then cultured with IL3+MV, R837, type I IFNs (rhIFN-α-2a and rhIFN-β-1a) or 5′-ppp-dsRNA LyoVec. (B) CD1c+ DCs were pretreated or not with IRS661 or Rux and then cultured with MV, UV-inactivated MV, R837, type I IFNs (rhIFN-α-2a and rhIFN-β-1a) or 5′-ppp-dsRNA LyoVec. (A, B) DCs were then added to the target Jurkat cells at an effector:target ratio of 20:1 and the percentage of specific lysis of Jurkat cells was determined by the measure of 51Cr release in the supernatants. Results are expressed as mean ± SEM of at least three independent experiments and each condition was assessed in triplicates for each experiment. For the left panel: *p < 0.05, one-way ANOVA (Kruskal–Wallis); for the right panel: *p < 0.05, Mann–Whitney test.
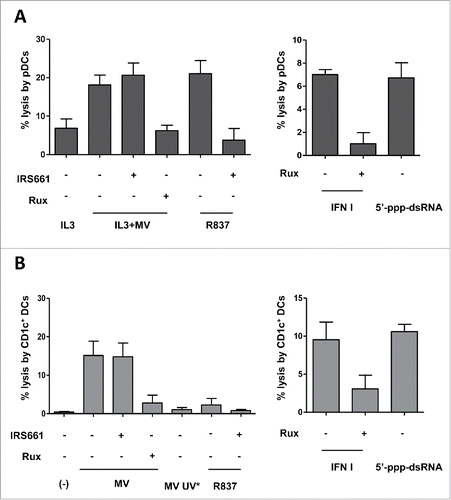
CD1c+ DCs were also able to kill Jurkat cells after exposure to MV (15% of specific lysis) (). As observed previously for TRAIL expression (), the lysis of Jurkat cells was not affected by the TLR7 inhibitor IRS661 (). As expected, the UV-inactivated MV, as well as R837, did not generate functional cytotoxic CD1c+ DCs (). As observed in pDCs (), both exogenous type I IFNs and 5′-ppp-dsRNA induced cytotoxic CD1c+ DCs (). Type I IFNs-mediated cytotoxicity was reduced when these DCs were pretreated with the inhibitor Ruxolitinib. This result was consistent with the expression profile of TRAIL in the presence of this inhibitor ().
Discussion
Several studies show that DCs can become cytotoxic after stimulation by viruses, especially RNA viruses, or by different TLRs agonists.Citation15 This phenomenon has been described more extensively for the pDC subset. In this study, we showed that oncolytic MV induces the expression of the pro-apoptotic protein TRAIL on human blood myeloid CD1c+ DCs and pDCs. We also determined that MV induces the secretion of large amounts of IFN-α by pDCs, whereas CD1c+ DCs secrete this cytokine in smaller quantities. We then demonstrated, using the JAK 1 and 2 signaling inhibitor Ruxolitinib, that the IFN-α secreted by both subsets of DCs in response to MV is involved in TRAIL expression. Finally, we showed that IFN-α secretion and subsequent TRAIL expression are triggered by the detection of MV by the cytosolic RLRs in CD1c+ DCs, whereas in pDCs both the RLRs and TLR7 signaling pathways are involved. Our results are consistent with previous works, showing that TRAIL expression on Mo-DCs and pDCs depends on IFN-α secretion induced by their stimulation.Citation19-22 However, one study revealed that TRAIL can also be rapidly expressed on pDCs after a TLR7 stimulation, following STAT1 phosphorylation and independently of their IFN-α secretion.Citation37
Several studies were conducted to identify which PRRs are involved in the detection of RNA viruses and which signaling pathways are subsequently activated in plasmacytoid and myeloid DCs. Experiments have been performed using DCs from mice deficient for different components of the RLR pathway such as RIG-I,Citation38 TBK-1 and IKK-ϵCitation39 or MAVS,Citation40 and from mice deficient for TLR7 or MyD88, which is involved in the TLR7 signaling pathway.Citation38 Altogether, these reports suggested that the RLR pathway is exploited by myeloid DCs to recognize and respond to RNA viruses, whereas pDCs respond exclusively through TLR7. This “TLR7 exclusive sensing” model in pDCs is now being reconsidered. Indeed, Kato et al. noted that IFN-α secretion by Newcastle disease virus-stimulated pDCs from MyD88-deficient mice is impaired, but not completely abrogated.Citation38 This suggests that other pathways, including the RIG-I-mediated one, may contribute to type I IFNs response to RNA viruses in pDCs. In addition, Hornung and collaborators demonstrated that negative-sense RNA viruses such as paramyxoviruses are able to induce a replication-dependent, TLR7-independent IFN-α secretion.Citation41 Moreover, our recent study highlighted the involvement of the RLR signaling in pDCs responding to the yellow fever live vaccine YF-17D, which is a positive-sense RNA virus.Citation36 We demonstrated that this virus is able to stimulate either the TLR7 or the RIG-I signaling pathway in pDCs, depending on how it is delivered to the cells. Here, we showed that the specific inhibition of TLR7 was not sufficient to abrogate the secretion of IFN-α and the expression of TRAIL in pDCs, suggesting that MV activates the RLR pathway in addition to TLR7 in these cells. Moreover, pDCs exposed to a UV-inactivated MV, whose replication is abrogated, secrete less IFN-α than those exposed to a live MV, indicating that replication intermediates are detected by RLRs in pDCs. UV-irradiation does not affect TLR7 activation, since viral replication is not required for the TLR7 recognition. UV-inactivated MV,Citation31 Sendai virus and influenza virusCitation32 are all able to induce IFN-α secretion albeit to a lesser extent than their replicative counterparts. The results obtained with Gen2.2 cells also confirmed that RIG-I is involved in the secretion of IFN-α and the expression of TRAIL in response to MV.
We did not expect to observe such an important reduction of IFN-α secretion and TRAIL expression on pDCs following pretreatment with MRT67307. This drug is supposed to inhibit specifically the RLR kinases TBK1 and IKK-ϵ. In our hand, the inhibitor also affects TLR7 signaling and type I IFN signaling through IFNAR. This is consistent with previous studies showing that the TLR7 agonist R837 induces the activation of TBK1 through its phosphorylation in bone marrow-derived macrophages (BMDMs)Citation42 and that the protein TRAF3 (TNF receptor-associated factor 3) links TLR adaptor proteins to the RLR kinases TBK1 and IKK-ϵ and is involved in the TLR7-dependent type I IFNs production in pDCsCitation43 and BMDMs.Citation44
Our results suggest that in CD1c+ DCs, the RLR pathway is solely responsible for the secretion of IFN-α and the expression of TRAIL in response to MV. There is a controversy concerning the expression of TLR7 by CD1c+ DCs: some reports showed that these DCs express it,Citation45–47 although at a much lower level than pDCs, whereas others studies showed an expression restricted to pDCs.Citation48,49 We showed here that R837 induces the expression of the maturation marker CD83 by CD1c+ DCs, suggesting that they express TLR7. However, this TLR7 agonist fails to trigger the production of IFN-α. This absence of IFN-α secretion in response to a TLR7 agonist in myeloid DCs may also be explained by the absence of constitutive expression of the transcription factor IRF-7, which is the main driver of IFN-α induction.Citation50 By contrast, IRF-7 is constitutively expressed at a high level in pDCs, allowing the production of high amounts of type I IFNs.Citation49,51,52 We confirmed the involvement of the RLR pathway in CD1c+ DCs in response to MV by using a UV-inactivated MV, whose replication is impaired and failed to induce the secretion of IFN-α and the expression of TRAIL.
In our study, we demonstrated that the expression of TRAIL, induced by MV on DCs, renders them functionally cytotoxic against Jurkat cells, which are known to be sensitive to TRAIL-mediated cell death.Citation22,23,53 Even though we measured granzyme B secretion by pDCs stimulated by MV, we have doubts concerning its cytotoxic effect in the absence of perforin. However, granzyme B alone could have antitumor functionsCitation54 and could also facilitate the cytotoxic lymphocytes transmigration by remodeling the extracellular matrix.Citation55 Contrary to the study that identified cytotoxic myeloid CD11c+ DCs, which express perforin and granzyme B after stimulation with a TLR7/8 ligand,Citation53 none of these proteins are expressed nor secreted by CD1c+ DCs exposed to MV in our hands. Furthermore, the level of TRAIL-mediated cytotoxicity of MV-stimulated pDCs and CD1c+ DCs is lower than the level obtained with CD8+ cytotoxic T lymphocytes and NK cells that use cytotoxic granules containing perforin and granzyme B56.
The activation of TRAIL-mediated cytotoxic functions in DCs exposed to MV reveals new potential therapeutic mechanisms in cancer treatment by oncolytic MV. In a mouse melanoma model, the topical application of Aldara® cream, containing the TLR7 ligand imiquimod, leads to the recruitment of pDCs at the tumor site and correlates with partial or complete regressions of tumors.Citation57 Recently, the mechanism of action was uncovered, explaining how pDCs convert into antitumor cytotoxic effectors through the expression of TRAIL and the pro-apoptotic protein granzyme B58. Similarly, treatment of basal cell carcinoma patients with Aldara® cream leads to partial or complete regressions of the lesions, associated with an inflammatory infiltrate containing cytotoxic pDCs and myeloid CD11c+ DCs.Citation53 Together, these reports suggest that the induction of cytotoxic DCs with a TLR7 agonist is favorable to obtain tumor regression. Our results suggest that it may be also achieved with oncolytic MV.
In addition to induce functional cytotoxic DCs, we and others have demonstrated that oncolytic MV is able to trigger the immunogenic death of tumor cells, which activates the maturation of DCs and their ability to cross-present tumor antigens.Citation6,10-12 Moreover, MV induces the secretion of IFN-α by DCs, especially by pDCs, which contributes to elicit antitumor immune responses and correlates with favorable disease outcomes.Citation59 Type I IFNs secreted in the tumor environment have also been reported to sensitize tumor cells to TRAIL-mediated lysis.Citation20 Thus, oncolytic MV represents a potent immunotherapeutic agent, which activates diverse antitumor mechanisms in different DC subsets that may participate to tumor cell eradication.
Materials and methods
Dendritic cell isolation
Platelet apheresis residues from healthy donors were provided by the Etablissement Français du Sang of Nantes (agreement NTS-2014–06). DCs were obtained from peripheral blood mononuclear cells (PBMCs), as previously described.Citation60 Briefly, DCs were enriched by counterflow centrifugation elutriation and then purified, using a human pan-DC pre-enrichment kit (StemCell Technologies, Inc.) as recommended by the manufacturer, which consists in an immunomagnetic negative isolation of DCs. Among the cells obtained, pDCs were stained with an APC-conjugated anti-BDCA-4 mAb (clone AD5–17F6, Miltenyi) and CD1c+ DCs with a FITC-conjugated anti-CD1c mAb (clone L161, eBiosciences) to sort them by flow cytometry (FACS Aria III, BD Biosciences). To improve the cell sorting, BV421-conjugated anti-CD3 (clone UCHT1), anti-CD19 (clone HIB19) and anti-CD14 (clone MϕP9) mAbs (BD Biosciences) were used to eliminate other immune populations.
Biological materials
DCs were cultured in RPMI-1640 medium supplemented with 2% human albumin, 100 U/mL penicillin, 100 µg/mL streptomycin and 2 mM L-glutamine (all reagents were from Gibco-Invitrogen) at 37°C in a 5% CO2 atmosphere for 16 h. 100,000 DCs were seeded in 96-well plates in a final volume of 200 µL. CD1c+ DCs were cultured alone or with the live-attenuated Schwarz strain of measles virus (MV) at an MOI (multiplicity of infection) of 10. pDCs were cultured with the survival factor IL3 alone (rhIL3, 20 ng/mL, CellGenix) or with IL3 and MV (MOI = 10). MV is produced and purified as previously described.Citation61 MV was inactivated by irradiation with UV-B (312 nm) during 10 min (Bio-Link, Vilber Lourmat). DCs were also cultivated with the TLR7 agonist R837 (1 µg/mL, Invivogen), exogenous type I IFNs (rhIFN-α-2a and rhIFN-β-1a, 100 ng/mL, ImmunoTools) or the RIG-I agonist 5′-ppp-dsRNA LyoVec (10 µg/mL, Invivogen). The cationic lipid transfection reagent LyoVec complexed with the RIG-I agonist facilitates its uptake. For the inhibition experiments of TLR7, TBK1 and IKK-ϵ, or JAK 1 and 2, DCs were pretreated for 30 min with a specific immunoregulatory DNA sequence IRS661 (1 µM, Eurofins), MRT67307 (8 µM, Sigma Aldrich) or Ruxolitinib (2.5 µM, Invivogen), respectively. After these pretreatments, the agonists were added to the cultures.
The pDC cell line Gen2.2 was obtained from Dr Joël Plumas and has been described elsewhere.Citation20 Gen2.2 cells were grown in RPMI medium supplemented with 10% fetal bovine serum (FBS), 1 mM sodium pyruvate and non-essential amino acids. The generation of the lentiviral vector particles-transduced shScrambled, shTLR7 and shRIG-I Gen2.2 cells was previously described.Citation36 One million Gen2.2 cells were seeded in 12-well plates in 2 mL complete medium. Cells were left uninfected, or were infected with the live or UV-inactivated MV at an MOI of 10 for 18 h, in the presence of IL3.
Flow cytometry
To determine the expression of CD83 and TRAIL on DCs, cells were stained with a PE-Cy7-conjugated anti-CD83 mAb (clone HB15e, BD Biosciences) and a PE-conjugated anti-TRAIL mAb (clone RIK-2, Biolegend). Results are presented as the percentage of DCs expressing the different markers compared with DCs stained with the corresponding isotypes. The analysis was performed on live cells that were Zombie NIR™ (Biolegend) negative, BDCA-4+ for the pDCs and CD1c+ for the CD1c+ DCs. Fluorescence was analyzed with a FACS Canto II (BD Biosciences) using the BD FACSDivaTM software.
Cytokine detection
IFN-α production was measured by ELISA (Mabtech) according to the manufacturer's instructions. Supernatants of pDCs and CD1c+ DCs were collected after 16 h. ELISA were performed directly without freezing supernatants.
Cytotoxicity assay
Jurkat cells were cultured in RPMI-1640 medium supplemented with 10% fetal calf serum, 100 U/mL penicillin, 100 µg/mL streptomycin and 2 mM L-glutamine (all reagents were from Gibco-Invitrogen) at 37°C in a 5% CO2 atmosphere. The cytotoxicity assay was performed in this medium. Target Jurkat cells were incubated for 1 h with Na251CrO4 at 37°C and then washed with culture medium. 1,000 51Cr-labeled target cells per well were seeded in 96-well plates and the effector cells (DCs) were added at an effector to target (E:T) ratio of 20:1, in triplicates. After 4 h of incubation at 37°C, 25 µL of supernatant were harvested and mixed with 100 µL of the scintillation liquid cocktail (OptiPhase Supermix, PerkinElmer). The 51Cr released in supernatants was measured using the Microβ® plate-based counter (PerkinElmer). The percentage of specific lysis was calculated as follows: [(ER − SR) / (MR − SR)] × 100, where ER, SR and MR correspond to experimental, spontaneous and maximum 51Cr release, respectively. SR was calculated from target cells incubated with culture medium alone and MR corresponds to the 51Cr released by target cells lyzed with culture medium containing 5% Triton X-100. Cell infection or treatment did not affect the SR.
Statistical analysis
Statistical analysis was performed using GraphPad Prism 5 software (GraphPad Software Inc.). To compare two groups, a nonparametric, one-tailed, unpaired Mann–Whitney comparison test was used. To compare more than two groups, non-parametric one-way ANOVA (Kruskal-Wallis test) was used, with Dunn's post-test. All data are presented as mean ± SEM. p values less than 0.05 were considered to be statistically significant. *p < 0.05, **p < 0.01, ***p < 0.001.
Disclosure of potential conflicts of interest
J.F.F., M.G. and F.T own patents on the use of attenuated MV for antitumor virotherapy.
KONI_A_1261240_Supplementary_materials.pdf
Download PDF (402.2 KB)Acknowledgments
We thank Delphine Coulais and Anne-Claire Branchu for their technical assistance and the Platform of Clinical Transfer and Development facility for their help in sorting the dendritic cells. We also thank Juliette Desfrançois-Noël, Nadège Marec and the core facility of flow cytometry (Cytocell). We thank Joël Plumas and Laurence Chaperot for Gen2.2 cells.
Funding
This work was supported by “La Ligue Régionale Grand Ouest contre le Cancer” (CSIRGO: CD16, CD22, CD44, CD49, CD72, CD79 and CD85), “La Ligue Nationale contre le Cancer,” “ l'association ARSMESO44,” “La Fondation du Souffle et le Fonds de Dotation Recherche en Santé Respiratoire,” “La fondation ARC,” “la Fondation pour la Recherche Médicale (FRM),” Agence Nationale pour la Recherche (ANR-12-JSV3–003–01) and Ville de Paris EMERGENCES Program.
References
- Guillerme JB, Gregoire M, Tangy F, Fonteneau JF. Antitumor virotherapy by attenuated measles virus (MV). Biology (Basel) 2013; 2:587-602; PMID:24832799; http://dx.doi.org/10.3390/biology2020587
- Anderson BD, Nakamura T, Russell SJ, Peng KW. High CD46 receptor density determines preferential killing of tumor cells by oncolytic measles virus. Cancer Res 2004; 64:4919-26; PMID:15256464; http://dx.doi.org/10.1158/0008-5472.CAN-04-0884
- Achard C, Boisgerault N, Delaunay T, Roulois D, Nedellec S, Royer PJ, Pain M, Combredet C, Mesel-Lemoine M, Cellerin L et al. Sensitivity of human pleural mesothelioma to oncolytic measles virus depends on defects of the type I interferon response. Oncotarget 2015; 6:44892-904; PMID:26539644; http://dx.doi.org/10.18632/oncotarget.6285
- Heinzerling L, Kunzi V, Oberholzer PA, Kundig T, Naim H, Dummer R. Oncolytic measles virus in cutaneous T-cell lymphomas mounts antitumor immune responses in vivo and targets interferon-resistant tumor cells. Blood 2005; 106:2287-94; PMID:15961518; http://dx.doi.org/10.1182/blood-2004-11-4558
- Galanis E, Hartmann LC, Cliby WA, Long HJ, Peethambaram PP, Barrette BA, Kaur JS, Haluska PJ, Jr., Aderca I, Zollman PJ et al. Phase I trial of intraperitoneal administration of an oncolytic measles virus strain engineered to express carcinoembryonic antigen for recurrent ovarian cancer. Cancer Res 2010; 70:875-82; PMID:20103634; http://dx.doi.org/10.1158/0008-5472.CAN-09-2762
- Galanis E, Atherton PJ, Maurer MJ, Knutson KL, Dowdy SC, Cliby WA, Haluska P, Jr., Long HJ, Oberg A, Aderca I et al. Oncolytic measles virus expressing the sodium iodide symporter to treat drug-resistant ovarian cancer. Cancer Res 2015; 75:22-30; PMID:25398436; http://dx.doi.org/10.1158/0008-5472.CAN-14-2533
- Russell SJ, Federspiel MJ, Peng KW, Tong C, Dingli D, Morice WG, Lowe V, O'Connor MK, Kyle RA, Leung N et al. Remission of disseminated cancer after systemic oncolytic virotherapy. Mayo Clin Proc 2014; 89:926-33; PMID:24835528; http://dx.doi.org/10.1016/j.mayocp.2014.04.003
- Lichty BD, Breitbach CJ, Stojdl DF, Bell JC. Going viral with cancer immunotherapy. Nat Rev Cancer 2014; 14:559-67; PMID:24990523; http://dx.doi.org/10.1038/nrc3770
- Fonteneau JF, Achard C, Zaupa C, Foloppe J, Erbs P. Oncolytic immunotherapy: the new clinical outbreak. Oncoimmunology 2016; 5; e1066961; PMID:26942085; http://dx.doi.org/10.1080/2162402X.2015.1066961
- Gauvrit A, Brandler S, Sapede-Peroz C, Boisgerault N, Tangy F, Gregoire M. Measles virus induces oncolysis of mesothelioma cells and allows dendritic cells to cross-prime tumor-specific CD8 response. Cancer Res 2008; 68:4882-92; PMID:18559536; http://dx.doi.org/10.1158/0008-5472.CAN-07-6265
- Donnelly OG, Errington-Mais F, Steele L, Hadac E, Jennings V, Scott K, Peach H, Phillips RM, Bond J, Pandha H et al. Measles virus causes immunogenic cell death in human melanoma. Gene Ther 2013; 20:7-15; PMID:22170342; http://dx.doi.org/10.1038/gt.2011.205
- Guillerme JB, Boisgerault N, Roulois D, Menager J, Combredet C, Tangy F, Fonteneau JF, Gregoire M. Measles virus vaccine-infected tumor cells induce tumor antigen cross-presentation by human plasmacytoid dendritic cells. Clin Cancer Res 2013; 19:1147-58; PMID:23339127; http://dx.doi.org/10.1158/1078-0432.CCR-12-2733
- Durand M, Segura E. The known unknowns of the human dendritic cell network. Front Immunol 2015; 6:129; PMID:25852695; http://dx.doi.org/10.3389/fimmu.2015.00129
- Ziegler-Heitbrock L, Ancuta P, Crowe S, Dalod M, Grau V, Hart DN, Leenen PJ, Liu YJ, MacPherson G, Randolph GJ et al. Nomenclature of monocytes and dendritic cells in blood. Blood 2010; 116:e74-80; PMID:20628149; http://dx.doi.org/10.1182/blood-2010-02-258558
- Tel J, Anguille S, Waterborg CE, Smits EL, Figdor CG, de Vries IJ. Tumoricidal activity of human dendritic cells. Trends Immunol 2014; 35:38-46; PMID:24262387; http://dx.doi.org/10.1016/j.it.2013.10.007
- Gonzalvez F, Ashkenazi A. New insights into apoptosis signaling by Apo2L/TRAIL. Oncogene 2010; 29:4752-65; PMID:20531300; http://dx.doi.org/10.1038/onc.2010.221
- Johnstone RW, Frew AJ, Smyth MJ. The TRAIL apoptotic pathway in cancer onset, progression and therapy. Nat Rev Cancer 2008; 8:782-98; PMID:18813321; http://dx.doi.org/10.1038/nrc2465
- Vidalain PO, Azocar O, Lamouille B, Astier A, Rabourdin-Combe C, Servet-Delprat C. Measles virus induces functional TRAIL production by human dendritic cells. J Virol 2000; 74:556-9; PMID:10590149; http://dx.doi.org/10.1128/JVI.74.1.556-559.2000
- Vidalain PO, Azocar O, Rabourdin-Combe C, Servet-Delprat C. Measle virus-infected dendritic cells develop immunosuppressive and cytotoxic activities. Immunobiology 2001; 204:629-38; PMID:11846228; http://dx.doi.org/10.1078/0171-2985-00102
- Chaperot L, Blum A, Manches O, Lui G, Angel J, Molens JP, Plumas J. Virus or TLR agonists induce TRAIL-mediated cytotoxic activity of plasmacytoid dendritic cells. J Immunol 2006; 176:248-55; PMID:16365416; http://dx.doi.org/10.4049/jimmunol.176.1.248
- Stary G, Klein I, Kohlhofer S, Koszik F, Scherzer T, Mullauer L, Quendler H, Kohrgruber N, Stingl G. Plasmacytoid dendritic cells express TRAIL and induce CD4+ T-cell apoptosis in HIV-1 viremic patients. Blood 2009; 114:3854-63; PMID:19690337; http://dx.doi.org/10.1182/blood-2009-04-217927
- Kalb ML, Glaser A, Stary G, Koszik F, Stingl G. TRAIL(+) human plasmacytoid dendritic cells kill tumor cells in vitro: mechanisms of imiquimod- and IFN-alpha-mediated antitumor reactivity. J Immunol 2012; 188:1583-91; PMID:22231699; http://dx.doi.org/10.4049/jimmunol.1102437
- Tel J, Smits EL, Anguille S, Joshi RN, Figdor CG, de Vries IJ. Human plasmacytoid dendritic cells are equipped with antigen-presenting and tumoricidal capacities. Blood 2012; 120:3936-44; PMID:22966165; http://dx.doi.org/10.1182/blood-2012-06-435941
- Akira S, Uematsu S, Takeuchi O. Pathogen recognition and innate immunity. Cell 2006; 124:783-801; PMID:16497588; http://dx.doi.org/10.1016/j.cell.2006.02.015
- Ikegame S, Takeda M, Ohno S, Nakatsu Y, Nakanishi Y, Yanagi Y. Both RIG-I and MDA5 RNA helicases contribute to the induction of alpha/beta interferon in measles virus-infected human cells. J Virol 2010; 84:372-9; PMID:19846522; http://dx.doi.org/10.1128/JVI.01690-09
- Runge S, Sparrer KM, Lassig C, Hembach K, Baum A, Garcia-Sastre A, Soding J, Conzelmann KK, Hopfner KP. In vivo ligands of MDA5 and RIG-I in measles virus-infected cells. PLoS Pathog 2014; 10:e1004081; PMID:24743923; http://dx.doi.org/10.1371/journal.ppat.1004081
- Goubau D, Deddouche S, Reise Sousa C. Cytosolic sensing of viruses. Immunity 2013; 38:855-69; PMID:23706667; http://dx.doi.org/10.1016/j.immuni.2013.05.007
- Chawla-Sarkar M, Lindner DJ, Liu YF, Williams BR, Sen GC, Silverman RH, Borden EC. Apoptosis and interferons: role of interferon-stimulated genes as mediators of apoptosis. Apoptosis 2003; 8:237-49; PMID:12766484; http://dx.doi.org/10.1023/A:1023668705040
- Barrat FJ, Meeker T, Gregorio J, Chan JH, Uematsu S, Akira S, Chang B, Duramad O, Coffman RL. Nucleic acids of mammalian origin can act as endogenous ligands for Toll-like receptors and may promote systemic lupus erythematosus. J Exp Med 2005; 202:1131-9; PMID:16230478; http://dx.doi.org/10.1084/jem.20050914
- Clark K, Peggie M, Plater L, Sorcek RJ, Young ER, Madwed JB, Hough J, McIver EG, Cohen P. Novel cross-talk within the IKK family controls innate immunity. Biochem J 2011; 434:93-104; PMID:21138416; http://dx.doi.org/10.1042/BJ20101701
- Duhen T, Herschke F, Azocar O, Druelle J, Plumet S, Delprat C, Schicklin S, Wild TF, Rabourdin-Combe C, Gerlier D et al. Cellular receptors, differentiation and endocytosis requirements are key factors for type I IFN response by human epithelial, conventional and plasmacytoid dendritic infected cells by measles virus. Virus Res 2010; 152:115-25; PMID:20600391; http://dx.doi.org/10.1016/j.virusres.2010.06.013
- Lee HK, Lund JM, Ramanathan B, Mizushima N, Iwasaki A. Autophagy-dependent viral recognition by plasmacytoid dendritic cells. Science 2007; 315:1398-401; PMID:17272685; http://dx.doi.org/10.1126/science.1136880
- Veron P, Boutin S, Martin S, Chaperot L, Plumas J, Davoust J, Masurier C. Highly efficient transduction of human plasmacytoid dendritic cells without phenotypic and functional maturation. J Transl Med 2009; 7:10; PMID:19173717; http://dx.doi.org/10.1186/1479-5876-7-10
- Lepelley A, Louis S, Sourisseau M, Law HK, Pothlichet J, Schilte C, Chaperot L, Plumas J, Randall RE, Si-Tahar M et al. Innate sensing of HIV-infected cells. PLoS Pathog 2011; 7:e1001284; PMID:21379343; http://dx.doi.org/10.1371/journal.ppat.1001284
- Rua R, Lepelley A, Gessain A, Schwartz O. Innate sensing of foamy viruses by human hematopoietic cells. J Virol 2012; 86:909-18; PMID:22090096; http://dx.doi.org/10.1128/JVI.06235-11
- Bruni D, Chazal M, Sinigaglia L, Chauveau L, Schwartz O, Despres P, Jouvenet N. Viral entry route determines how human plasmacytoid dendritic cells produce type I interferons. Sci Signal 2015; 8:ra25; PMID:25737587; http://dx.doi.org/10.1126/scisignal.aaa1552
- Di Domizio J, Blum A, Gallagher-Gambarelli M, Molens JP, Chaperot L, Plumas J. TLR7 stimulation in human plasmacytoid dendritic cells leads to the induction of early IFN-inducible genes in the absence of type I IFN. Blood 2009; 114:1794-802; PMID:19553637; http://dx.doi.org/10.1182/blood-2009-04-216770
- Kato H, Sato S, Yoneyama M, Yamamoto M, Uematsu S, Matsui K, Tsujimura T, Takeda K, Fujita T, Takeuchi O et al. Cell type-specific involvement of RIG-I in antiviral response. Immunity 2005; 23:19-28; PMID:16039576; http://dx.doi.org/10.1016/j.immuni.2005.04.010
- Matsui K, Kumagai Y, Kato H, Sato S, Kawagoe T, Uematsu S, Takeuchi O, Akira S. Cutting edge: role of TANK-binding kinase 1 and inducible IkappaB kinase in IFN responses against viruses in innate immune cells. J Immunol 2006; 177:5785-9; PMID:17056502; http://dx.doi.org/10.4049/jimmunol.177.9.5785
- Sun Q, Sun L, Liu HH, Chen X, Seth RB, Forman J, Chen ZJ. The specific and essential role of MAVS in antiviral innate immune responses. Immunity 2006; 24:633-42; PMID:16713980; http://dx.doi.org/10.1016/j.immuni.2006.04.004
- Hornung V, Schlender J, Guenthner-Biller M, Rothenfusser S, Endres S, Conzelmann KK, Hartmann G. Replication-dependent potent IFN-alpha induction in human plasmacytoid dendritic cells by a single-stranded RNA virus. J Immunol 2004; 173:5935-43; PMID:15528327; http://dx.doi.org/10.4049/jimmunol.173.10.5935
- Clark K, Takeuchi O, Akira S, Cohen P. The TRAF-associated protein TANK facilitates cross-talk within the IkappaB kinase family during Toll-like receptor signaling. Proc Natl Acad Sci U S A 2011; 108:17093-8; PMID:21949249; http://dx.doi.org/10.1073/pnas.1114194108
- Oganesyan G, Saha SK, Guo B, He JQ, Shahangian A, Zarnegar B, Perry A, Cheng G. Critical role of TRAF3 in the Toll-like receptor-dependent and -independent antiviral response. Nature 2006; 439:208-11; PMID:16306936; http://dx.doi.org/10.1038/nature04374
- Hacker H, Redecke V, Blagoev B, Kratchmarova I, Hsu LC, Wang GG, Kamps MP, Raz E, Wagner H, Hacker G et al. Specificity in Toll-like receptor signalling through distinct effector functions of TRAF3 and TRAF6. Nature 2006; 439:204-7; PMID:16306937; http://dx.doi.org/10.1038/nature04369
- Hemont C, Neel A, Heslan M, Braudeau C, Josien R. Human blood mDC subsets exhibit distinct TLR repertoire and responsiveness. J Leukoc Biol 2013; 93:599-609; PMID:23341538; http://dx.doi.org/10.1189/jlb.0912452
- Flacher V, Bouschbacher M, Verronese E, Massacrier C, Sisirak V, Berthier-Vergnes O, de Saint-Vis B, Caux C, Dezutter-Dambuyant C, Lebecque S et al. Human Langerhans cells express a specific TLR profile and differentially respond to viruses and Gram-positive bacteria. J Immunol 2006; 177:7959-67; PMID:17114468; http://dx.doi.org/10.4049/jimmunol.177.11.7959
- Ito T, Amakawa R, Kaisho T, Hemmi H, Tajima K, Uehira K, Ozaki Y, Tomizawa H, Akira S, Fukuhara S. Interferon-alpha and interleukin-12 are induced differentially by Toll-like receptor 7 ligands in human blood dendritic cell subsets. J Exp Med 2002; 195:1507-12; PMID:12045249; http://dx.doi.org/10.1084/jem.20020207
- Jarrossay D, Napolitani G, Colonna M, Sallusto F, Lanzavecchia A. Specialization and complementarity in microbial molecule recognition by human myeloid and plasmacytoid dendritic cells. Eur J Immunol 2001; 31:3388-93; PMID:11745357; http://dx.doi.org/10.1002/1521-4141(200111)31:11%3c3388::AID-IMMU3388%3e3.0.CO;2-Q
- Kadowaki N, Ho S, Antonenko S, Malefyt RW, Kastelein RA, Bazan F, Liu YJ. Subsets of human dendritic cell precursors express different toll-like receptors and respond to different microbial antigens. J Exp Med 2001; 194:863-9; PMID:11561001; http://dx.doi.org/10.1084/jem.194.6.863
- Ning S, Pagano JS, Barber GN. IRF7: activation, regulation, modification and function. Genes Immun 2011; 12:399-414; PMID:21490621; http://dx.doi.org/10.1038/gene.2011.21
- Izaguirre A, Barnes BJ, Amrute S, Yeow WS, Megjugorac N, Dai J, Feng D, Chung E, Pitha PM, Fitzgerald-Bocarsly P. Comparative analysis of IRF and IFN-alpha expression in human plasmacytoid and monocyte-derived dendritic cells. J Leukoc Biol 2003; 74:1125-38; PMID:12960254; http://dx.doi.org/10.1189/jlb.0603255
- O'Brien M, Manches O, Sabado RL, Baranda SJ, Wang Y, Marie I, Rolnitzky L, Markowitz M, Margolis DM, Levy D et al. Spatiotemporal trafficking of HIV in human plasmacytoid dendritic cells defines a persistently IFN-alpha-producing and partially matured phenotype. J Clin Invest 2011; 121:1088-101; PMID:21339641; http://dx.doi.org/10.1172/JCI44960
- Stary G, Bangert C, Tauber M, Strohal R, Kopp T, Stingl G. Tumoricidal activity of TLR7/8-activated inflammatory dendritic cells. J Exp Med 2007; 204:1441-51; PMID:17535975; http://dx.doi.org/10.1084/jem.20070021
- Susanto O, Trapani JA, Brasacchio D. Controversies in granzyme biology. Tissue Antigens 2012; 80:477-87; PMID:23137319; http://dx.doi.org/10.1111/tan.12014
- Prakash MD, Munoz MA, Jain R, Tong PL, Koskinen A, Regner M, Kleifeld O, Ho B, Olson M, Turner SJ et al. Granzyme B promotes cytotoxic lymphocyte transmigration via basement membrane remodeling. Immunity 2014; 41:960-72; PMID:25526309; http://dx.doi.org/10.1016/j.immuni.2014.11.012
- Roulois D, Vignard V, Gueugnon F, Labarriere N, Gregoire M, Fonteneau JF. Recognition of pleural mesothelioma by mucin-1(950-958)/human leukocyte antigen A*0201-specific CD8+ T-cells. Eur Respir J 2011; 38:1117-26; PMID:21540305; http://dx.doi.org/10.1183/09031936.00160210
- Palamara F, Meindl S, Holcmann M, Luhrs P, Stingl G, Sibilia M. Identification and characterization of pDC-like cells in normal mouse skin and melanomas treated with imiquimod. J Immunol 2004; 173:3051-61; PMID:15322165; http://dx.doi.org/10.4049/jimmunol.173.5.3051
- Drobits B, Holcmann M, Amberg N, Swiecki M, Grundtner R, Hammer M, Colonna M, Sibilia M. Imiquimod clears tumors in mice independent of adaptive immunity by converting pDCs into tumor-killing effector cells. J Clin Invest 2012; 122:575-85; PMID:22251703; http://dx.doi.org/10.1172/JCI61034
- Zitvogel L, Galluzzi L, Kepp O, Smyth MJ, Kroemer G. Type I interferons in anticancer immunity. Nat Rev Immunol 2015; 15:405-14; PMID:26027717; http://dx.doi.org/10.1038/nri3845
- Coulais D, Panterne C, Fonteneau JF, Gregoire M. Purification of circulating plasmacytoid dendritic cells using counterflow centrifugal elutriation and immunomagnetic beads. Cytotherapy 2012; 14:887-96; PMID:22687187; http://dx.doi.org/10.3109/14653249.2012.689129
- Combredet C, Labrousse V, Mollet L, Lorin C, Delebecque F, Hurtrel B, McClure H, Feinberg MB, Brahic M, Tangy F. A molecularly cloned Schwarz strain of measles virus vaccine induces strong immune responses in macaques and transgenic mice. J Virol 2003; 77:11546-54; PMID:14557640; http://dx.doi.org/10.1128/JVI.77.21.11546-11554.2003