ABSTRACT
Malignant pleural mesothelioma (MPM) is an aggressive cancer with a poor prognosis and an increasing incidence, for which novel therapeutic strategies are urgently required. Since the immune system has been described to play a presumed role in the protection against MPM, characterization of its tumor immune microenvironment (TME) and immune checkpoints can identify new immunotherapeutic targets and their predictive and/or prognostic value.
To characterize the TME and the immune checkpoint expression profile, we performed immunohistochemistry (IHC) on formalin-fixed paraffin embedded (FFPE) tissue sections from 54 MPM patients (40 at time of diagnosis; 14 treated with chemotherapy). We stained for PD-1, PD-L1, TIM-3, LAG-3, CD4, CD8, CD45RO, granzyme B, FoxP3 and CD68. Furthermore, we analyzed the relationship between the immunological parameters and survival, as well as response to chemotherapy. We found that TIM-3, PD-1 and PD-L1 were expressed on both immune and tumor cells. Strikingly, PD-1 and PD-L1 expression on tumor cells was only seen in unpretreated samples. No LAG-3 expression was observed. CD45RO expression in the stroma was an independent negative predictive factor for response on chemotherapy, while CD4 and TIM-3 expression in lymphoid aggregates were independent prognostic factors for better outcome. Our data propose TIM-3 as a promising new target in mesothelioma. Chemotherapy influences the expression of immune checkpoints and therefore further research on the best combination treatment schedule is required.
Abbreviations
APC | = | antigen-presenting cell |
FFPE | = | formalin-fixed paraffin embedded |
IFNγ | = | interferon-gamma |
IHC | = | immunohistochemistry |
IL | = | interleukin |
LAG-3 | = | lymphocyte activation gene-3 |
MPM | = | malignant pleural mesothelioma |
NSCLC | = | non-small-cell lung cancer |
PD-1 | = | programmed death-1 |
PD-L1 | = | programmed death-ligand 1 |
TAM | = | tumor-associated macrophage |
TGF | = | transforming growth factor |
TILs | = | tumor-infiltrating lymphocytes |
TIM-3 | = | T-cell immunoglobulin mucin-3 |
TME | = | tumor microenvironment |
Treg | = | regulatory T-cell |
Introduction
Malignant pleural mesothelioma (MPM) is an aggressive and fatal cancer that is causally associated with previous asbestos exposure in most afflicted patients. Although a rare disease, MPM incidence has been increasing in recent years and this trend is expected to continue over the next decades. This is mainly due to the ongoing asbestos consumption in developing countries, as well as the long latency period between exposure to asbestos and disease onset.Citation1 Palliative platinum-antifolate chemotherapy has a significant but moderate impact on patients' outcome, resulting in a median overall survival of about 1 y compared with the 8–10 mo observed for chemotherapy-naive patients.Citation2-4 Based on its poor prognosis and increasing incidence, novel therapeutic strategies for MPM are required.
The discovery of immune checkpoints such as cytotoxic T-lymphocyte antigen-4, programmed death-1 (PD-1), T-cell immunoglobulin mucin-3 (TIM-3) and lymphocyte activation gene-3 (LAG-3), introduced a new era in targeted cancer therapy. Several monoclonal-blocking antibodies have already shown promising results in different cancer types.Citation5 Their rationale is to reactivate silenced immune responses by neutralizing the so-called immune checkpoints, which are proteins that induce immune cell exhaustion and tolerance. Characterization of the tumor immune microenvironment (TME) could be of great value to unravel these silenced immune responses. Expression of programmed death-ligand 1 (PD-L1) on tumor cells and TILs has been described in literature.Citation6-11 However, only one series described PD-1 expression on tumor-infiltrating lymphocytes (TILs)Citation8 and nothing has been reported yet on TIM-3 and LAG-3 in human MPM tissue.
Chemotherapy influences the TME, including the expression of immune checkpoints. An upregulation of PD-1 and/or PD-L1 after chemotherapy for leukemia, thymic epithelial tumors and ovarian cancer has been reported.Citation12-15 Identification of the effect of chemotherapy on the TME in MPM can guide the rational design of combination strategies of immune checkpoint inhibition with chemotherapeutics.Citation16-18
We investigated the expression of TIM-3 and LAG-3 in human MPM tumor tissue using immunohistochemistry (IHC), along with several other immune cell markers of the TME, and addressed their potential role as targets for immunotherapy. In order to elucidate the effect of chemotherapy on the TME, we compared tissue sections from unpretreated and chemotherapy pretreated patients. We furthermore analyzed the prognostic and predictive value of different immunological parameters.
Results
Clinicopathological features of the MPM patient cohort
The clinicopathological characteristics of our MPM cohort are summarized in . All 54 patients were diagnosed between 2000 and 2015. Forty samples were taken at the time of diagnosis and 14 samples were treated with chemotherapy (detailed information on the pretreated samples see Table S1). The unpretreated samples consisted of 9 biphasic, 26 epitheloid and 5 sarcomatoid cases, whereas the pretreated samples comprised 1 biphasic and 13 epitheloid cases. The median age of the unpretreated patients was 69 y and 63 y for the pretreated patients. In both groups, patients were predominantly male. At the time of last follow-up (1 March 2016), 22% of the unpretreated and 36% of the pretreated patients were still alive. Except for age (p = 0.043), no significant differences were found between the clinicopathological parameters of both groups.
Table 1. Clinicopathological parameters of unpretreated and chemotherapy pretreated mesothelioma patients.
Immune composition of MPM tissue samples and biomarker identification
The tissue sections were analyzed for the presence of lymphocytes, lymphoid aggregates and stroma (). Lymphocytic infiltration was found in all tissue samples (). Samples from pretreated patients showed more infiltration than from unpretreated ones: a stromal score of 1 was observed in more than half of the unpretreated samples, while the majority of the pretreated samples had a stromal score of 2 or 3. Lymphoid aggregates were present in more than half of the unpretreated and pretreated samples (65% and 71%, respectively). Germinal centers within the aggregates were seen in around one-third of the samples (27% unpretreated, 30% pretreated).
Table 2. Expression of immune checkpoints and immune cell markers in FFPE tissue from unpretreated and pretreated MPM patients.
Figure 1. Lymphocyte infiltration of MPM tissue samples. Pictures were taken from slides of two different MPM patients (A) and (B). The lymphocyte infiltration of both tissue samples is representative for those in our overall MPM cohort. The images show the presence of lymphoid aggregates, CD4+ (red) and CD8+ (brown) lymphocytes in the tumoral stroma and around the tumor cells. In patient (A), tumor cells are epitheloid and pleiomorfic, directly surrounded by TILs. The stroma has a low density, while in patient (B) the stroma has a higher density. Tumor cells are smaller and surrounded by stroma with TILs in it. Original magnification: left column pictures 100×; right column pictures 200×. LA, lymphoid aggregate; TC, tumor cells; TILs, tumor-infiltrating lymphocytes; ST, stroma.
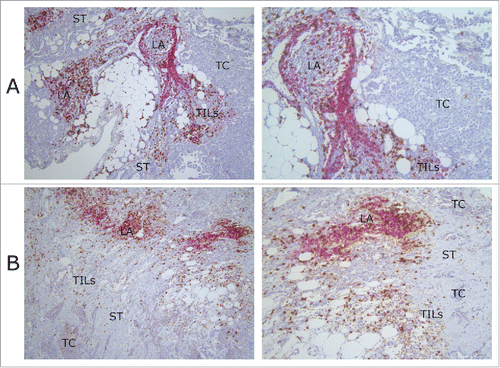
Tissue sections were stained for six different immune cell markers (; ). Percentages of TILs in the stroma ranged from 20% to 80%. CD4+ and CD8+ cells showed a strong intensity in the TILs and in hot spots of the lymphoid aggregates (). CD8+ TILs were present in all samples and were the predominant cell type of the immune infiltrate over CD68+ cells, CD45RO+ cells and CD4+ TILs, with 70% of the unpretreated and 57% of the pretreated samples showing CD8+ expression on more than 50% of the lymphocytes.
Figure 2. Immunohistochemical staining patterns of different immunomarkers in MPM tissue. (A) CD4+ (red) and CD8+ (brown) lymphocytes in stroma; (B) CD68+ histiocytes stained with strong intensity in the cytoplasm surrounding negative tumor cells; (C) CD45RO+ lymphocytes in stroma with moderate to strong intensity; (D) CD4+FoxP3+ lymphocytes in stroma with brown nuclear staining for FoxP3 and red cytoplasmic staining for CD4+; (E) stromal cells expressing granzyme B in their cytoplasm surrounding negative tumor cells; (F) PD-L1+ tumor cells with membrane accentuation; (G) PD-1+ lymphocytes stained with strong intensity in the cytoplasm; (H) tumor cells and lymphocytes in stroma with strong TIM-3 staining in the cytoplasm; (I) Absence of LAG-3 staining in MPM tissue; (J) LAG-3+ lymphocytes in human tonsil showing strong cytoplasmic staining. All tissue sections were counterstained with hematoxylin (blue color). Original magnification 1,000×. LA, lymphoid aggregate; TC, tumor cells; TIL, tumor-infiltrating lymphocytes; ST, stroma.
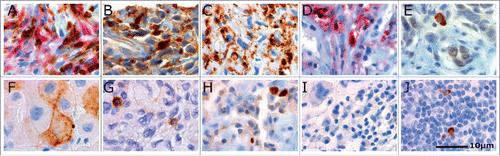
Although present to a lesser extent than CD8+ TILs, CD68 expression on histiocytes and macrophages was also seen in all samples (). The majority of samples had less than 50% CD68+ cells. Similar observations were made for CD45RO, a marker for effector and memory T cells (). Both CD68 and CD45RO expression in the stroma were significantly correlated with stromal presence of CD4+FoxP3+ cells [correlation coefficient (R) = 0.41, p = 0.002; R = 0.27, p = 0.046]. Results of a multivariate analysis revealed that an increase in the CD45RO expression on stromal lymphocytes was significantly associated with a lower likelihood of partial or complete response to chemotherapy [odds ratio (OR) = 0.06, p = 0.008; ; Table S2].
Figure 3. Predicted probability plots for the significant predictive factors CD45RO and PD-1. Plot of response on chemotherapy (y-axis) versus: (A) CD45RO expression on lymphocytes in the stroma (x-axis), and (B) PD-1 expression on lymphocytes in the stroma (x-axis). Observations within our cohort are represented by the empty dots (right y-axis). The full dots show the observed chance on partial/complete response within each expression category. The curve depicts the estimated probability of partial/complete response based on a univariate logistic regression model (p = 0.017, p = 0.0514; left y-axis). After multivariate adjustments CD45RO expression on stromal lymphocytes remained an independent good predictive factor (p = 0.0076).
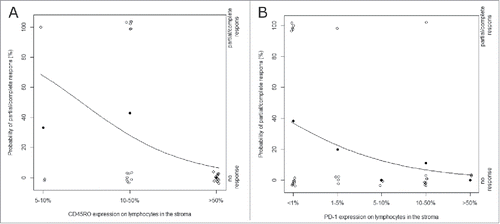
CD4+ TILs in the stroma were seen in 75% of the unpretreated and 71% of the pretreated samples. After multivariate analysis, the presence of CD4+ lymphocytes in the lymphoid aggregates was a significant good prognostic factor [risk ratio (RR) = 0.13, p = 0.014; ; Table S3]. For each increase of CD4 expression with one category, there is a lower risk of death. No significant differences were found between the unpretreated and pretreated samples. A subset of the CD4+ cells was also FoxP3+ () with a range from 1% to 50% CD4+FoxP3+ positive cells in the samples (data not shown). Those CD4+FoxP3+ cells were positively correlated with the presence of CD4+ TILs in the stroma (R = 0.52, p < 0.001).
Figure 4. Kaplan-Meier overall survival according to CD4, PD-1 and TIM-3 expression in the lymphoid aggregates. Univariate analysis showed prognostic significance for: (A) CD4 (p = 0.008), (B) PD-1 (p = 0.029) and (C) TIM-3 (p = 0.001) expression in the lymphoid aggregates. After multivariate adjustments CD4 and TIM-3 expression in the aggregates remained independent good prognostic factors (p = 0.015 and p = 0.002).
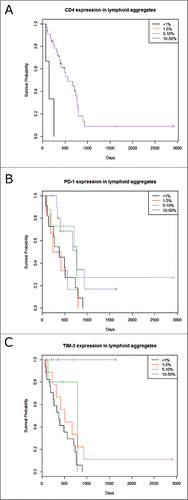
Moderate positivity for granzyme B was observed in the cytoplasm of immune cells in the stroma (mainly plasma cells and mast cells,Citation19,20) of less than half of the samples (33% unpretreated, 43% pretreated) ().
Immune checkpoint expression in MPM tissue
A cytoplasmic granular staining with a moderate to strong intensity was observed for PD-L1. Four samples (three unpretreated and one pretreated) also showed membrane staining (). PD-L1 was seen on TILs in the stroma, in lymphoid aggregates and in germinal centers of both unpretreated and pretreated samples. Significant differences for PD-L1 expression in the stroma were observed according to the different histological subtypes. Sarcomatoid histology showed more PD-L1 expression than the epitheloid and biphasic subtypes (p < 0.001, p = 0.008; data not shown). Strikingly, PD-L1 expression on tumor cells was only detectable in unpretreated tumor samples. 28% of the unpretreated samples with PD-L1+ tumor cells also had PD-L1+ TILs in their stroma (). Presence of PD-1+ tumor cells and CD8+ TILs was seen in 40% of those samples. CD4+FoxP3+ cells were seen in the majority of samples that had PD-1+ tumor cells and CD4+ TILs ().
Table 3. Within patient combined expression of PD-1, PD-L1 and TIM-3 on tumor cells and TIL subtypes.
PD-1 expression was localized in the cytoplasm showing membrane accentuation with a moderate intensity. A strong intensity was found on lymphocytes within the germinal centers of lymphoid aggregates (). PD-1 was seen on TILs of both unpretreated and pretreated samples (65% and 71%, respectively), whereas the expression on tumor cells was found only in 10% of the unpretreated samples. In 10% of those samples, expression of PD-1 on both tumor cells and TILs was observed (). CD8+ TILs together with PD-1+ tumor cells were also seen in 10% of the unpretreated samples (). The same percentage was found for the expression of CD4 and the co-expression of CD4 and FoxP3 in unpretreated samples (). According to a univariate analysis, the presence of PD-1+ TILs in the stroma was associated with smaller likelihood of response after chemotherapy (OR = 0.50, p = 0.0514; ; Table S2). PD-1+ TILs were positively correlated with CD4+FoxP3+ and granzyme B+ cells in the stroma (R = 0.36, p = 0.008; R = 0.3, p = 0.014). 62% of the unpretreated samples with PD-1+ TILs also had PD-L1+ TILs and 50% of those samples showed PD-L1+ tumor cells (data not shown). In contrast, only 30% of the pretreated samples with PD-1+ TILs also had PD-L1+ TILs (data not shown). No PD-L1+ tumor cells were observed in the pretreated samples. After univariate analysis, high expression of PD-1 in the aggregates was a good prognostic factor (RR = 0.70, p = 0.029) associated with a lower risk of death than the lower expression category (; Table S3). No significant differences were found between the unpretreated and pretreated samples. We observed that PD-1, PD-L1 and TIM-3 were expressed in lymphoid aggregates but only PD-1 and PD-L1 were also expressed in their germinal centers.
TIM-3 scoring was based on the cytoplasmic () and membrane staining of cells. However, often a weak nuclear staining was seen in more than half of the tumor cells in the tissue section, possibly indicating the translocation of TIM-3 proteins from the cytoplasm to the nucleus. TIM-3 expression was found on tumor cells in unpretreated and pretreated samples (40% and 36%, respectively). It was also expressed on TILs and on plasma cells in the stroma, however, less often in pretreated samples compare with the unpretreated (29% vs. 40%). The presence of TIM-3+ tumor cells in combination with CD8+ TILs was most observed, followed by the expression of PD-1+ TILs in combination with TIM-3+ tumor cells (). A strong correlation was found between the presence of TIM-3+ TILs and PD-1+ TILs in the stroma (RR = 0.48, p < 0.001). TIM-3+ lymphocytes were found in lymphoid aggregates of more than half of the unpretreated and pretreated samples (54% and 60%) and were correlated with both TIM-3+ TILs and CD4+ TILs in the stroma (RR = 0.64, p < 0.001; RR = 0.42, p = 0.010). Expression of TIM-3 on lymphocytes in the aggregates was found to be an independent good prognostic factor after multivariate adjustment (; Table S3). Overall survival was better for patients with high TIM-3 expression in their aggregates (RR = 0.47, p = 0.002). No significant differences were found between the unpretreated and pretreated samples.
All samples were negative for LAG-3 (), in contrast to the control sample showing cytoplasmic staining with strong intensity on lymphocytes ().
Discussion
In this series, we report a comprehensive description of the TME in MPM. In summary, we are the first to describe the presence of TIM-3 and absence of LAG-3 expression in MPM tissue, as well as PD-1 expression on MPM tumor cells. PD-1 and CD45RO expression in the stroma were associated with worse response to chemotherapy. After multivariate analysis, stromal CD45RO expression remained a negative predictive factor for response to chemotherapy. Expression of PD-1, CD4 and TIM-3 in lymphoid aggregates were good prognostic factors after univariate analysis. CD4 and TIM-3 expression in lymphoid aggregates remained independent good prognostic factors after multivariate adjustment.
PD-L1 expression was observed in 68% of the unpretreated samples using a cut-off value of ≥1%. Differences with other series resultsCitation7,9-11,21 might be due to the use of different antibody clones, sample sizes or cut-off values. Teng et al.Citation22 described a classification of tumors into four groups based on their pretreatment PD-L1 expression status and the presence of TILs, that might be used to predict a patient's response to anti PD-1/PD-L1 blockade. In our own MPM cohort TILs were present in all unpretreated samples. 40% of the tumor samples can be classified as type I (PD-L1+TILs+), while the others are type IV (PD-L1-TILs+). Type I tumors with adaptive immune resistance have been described to be the most likely type to benefit from anti PD-1/PD-L1 therapy,Citation23 suggesting that 40% of our unpretreated MPM patients would respond to this checkpoint blockade. It is suggested that other suppressors might be present in the type IV TME leading to immune tolerance, thus targeting other suppressive pathways might offer an alternative treatment approach for these types of cancer. We saw that PD-L1 on tumor cells was not always expressed simultaneously with PD-L1 on TILs and other stromal components, which is in concordance with the findings in other tumor types.Citation23 Presence of both PD-1+ and PD-L1+ TILs was observed in unpretreated and pretreated samples. This might reflect a potential immunosuppressive microenvironment created by the interaction between PD-1 and PD-L1.
PD-1 was expressed to the same extent on immune cells in unpretreated and pretreated samples. We found PD-1 expression on TILs in 65% of the unpretreated samples, which is in line with the 62% reported by Combaz-Lair et al. in MPM.Citation8 Our data are the first to report PD-1 expression on TILs in the stroma as a negative predictive factor associated with worse response on chemotherapy in MPM. This is in line with the finding of Zhang et al. that large B-cell lymphoma patients with low PD-1 expression on T cells are more likely to respond to chemotherapy.Citation24 Results derived from an immunodeficient mesothelioma mouse model suggest that the effect of pemetrexed is mediated through activation of CD8+ T cells, rather than direct killing of tumor cells.Citation25 Since more PD-1 expression can point at exhausted CD8+ T cells, this might decrease the antitumor efficiency of pemetrexed. PD-1 on lymphocytes in aggregates, on the other hand, was a prognostic factor for better overall survival. Percentages of positive lymphocytes in the aggregates ranged from 1% to 50%, stained with a weak to moderate intensity suggesting these are activated PD-1+LOW cells. It has been described that these cells are still functional, able to secrete interferon (IFN)-γ, resulting in activation of other immune cells that play a role in the antitumor response.Citation26 We observed PD-1 expression on tumor cells in unpretreated but not in pretreated samples, which has not been described in other cancer types so far. Since a rather low number of pretreated samples was used in our series, future studies including larger validation cohorts are needed to draw any meaningful conclusions.
Although until now TIM-3 expression has been predominantly shown on T-cells, our data demonstrate TIM-3 expression also on MPM tumor cells which is consistent with findings in melanoma, NSCLC and renal cell carcinoma.Citation27-29 While PD-1 was only expressed on tumor cells in 10% of the unpretreated samples, TIM-3 expression was observed on tumor cells in both unpretreated and pretreated samples. More unpretreated samples had TIM-3+ TILs compare with the pretreated, taken into consideration that our number of pretreated samples is rather low. TIM-3 blockade shows promising results in vitro and in vivo in several cancer types,Citation30,31 but nothing has been described for mesothelioma so far. We are the first to report TIM-3 expression in lymphoid aggregates as an independent prognostic factor associated with better overall survival in MPM. Our data support further research on TIM-3 as a target of new treatment strategies of MPM and advocate to prioritize clinical translation of TIM-3 above LAG-3, which has not been detected in our tumor samples. In this context, patients are currently being recruited for a phase 1 trial of an anti-TIM-3-blocking antibody in patients with solid tumors (NCT02817633, ClinicalTrials.gov).
The effect of chemotherapy on tumoral PD-L1 expression has been investigated in several cancer types, showing divergent results. For this series, samples of only 14 pretreated patients were at our disposal and thus no strong conclusions can be drawn based on our results. In our hands, PD-L1 expression on tumor cells was observed only in unpretreated samples, which is in contrast with recent communications. Preliminary data from the KEYNOTE-0286 and the JAVELINCitation32 trial in mesothelioma indicate that PD-L1 expression can be seen irrespective of prior chemotherapy treatment. However, similar to our own observation, a downregulation of PD-L1 following chemotherapy treatment has also been noted in other cancer types, such as non-small-cell lung cancer (NSCLC) and breast cancer.Citation33,34 Expression of PD-L1 on the cell surface has been associated with the activation of the PI3K/Akt signaling pathway and PD-L1 has been described to be a downstream target of Akt.Citation35 Ghebeh et al.Citation34 reported a significant downregulation of PD-L1 on the surface of breast cancer cell lines after doxorubicin treatment which was accompanied by an upregulation of PD-L1 in the nucleus. They saw that the redistribution of PD-L1 from the cell surface to the nucleus was associated with a translocation of phosphorylated Akt from the membrane to the nucleus. They also reported that inhibition of Akt partially decreased PD-L1 expression on the surface, findings that are supported by Latswika et al.Citation35 Further research on the effect of chemotherapeutics on Akt signaling is warranted in order to fully unravel the underlying mechanisms that might be responsible for PD-L1 downregulation. Compared to the unpretreated, fewer pretreated patients had TIM-3+ TILs in their stroma, which is in line with findings from Zhang et al. in diffuse large B-cell lymphoma.Citation24 A stromal immune cell score of 3 was more often found for pretreated samples, suggesting that chemotherapy causes an increase in immune infiltration. This is in concordance with findings in other tumor types, showing that cisplatin promotes recruitment and proliferation of effector immune cells.Citation36 Future studies with a larger number of samples are required to unravel the best treatment schedule to combine chemotherapy with immunotherapy.
Although data about the prognostic role of TILs in MPM are controversial,Citation37-40 it is clear that these cells are important for antitumor immunity. After multivariate adjustments CD4+ expression on lymphocytes in lymphoid aggregates was demonstrated to be a good independent prognostic factor, with better overall survival observed for patients with more CD4+ lymphocytes in their aggregates, confirming the findings of Yamada et al. in mesothelioma.Citation39 Also, in NSCLC CD4+ TILs have been described as a positive prognostic factor.Citation41-43 Their influence on patients' prognosis can be explained by the important role of CD4+ TILs in antitumor immunity. Via secretion of several immunoregulatory cytokines, such as IFNγ and interleukin-2, they provide help for stimulating CD8+ TILs and natural killer cells. CD4+ TILs also express CD40-ligand on their surface which binds to CD40 expressed by antigen-presenting cells (APC).Citation44-46 This interaction causes activation of APC, which also contributes to priming of CD8+ TILs. Taken together, CD4+ TILs have both a direct and indirect impact on the generation of a T cell-mediated antitumor response.
In our series, the two immune-related parameters with prognostic significance after multivariate adjustments (CD4+ TILs and TIM-3) are situated in the lymphoid aggregates, showing that these are important structures influencing a patient's outcome. We observed CD4+, CD8+, CD45RO and TIM-3 expression in lymphoid aggregates, suggesting that these structures might function as a site for initiationCitation47 or restimulation of antitumor adaptive immune responses. As such, these aggregates show functional similarity with tertiary lymphoid structures, in which T and B lymphocytes are segregated into two adjacent regions surrounded by high endothelial venules.Citation48,49
A subset of the CD4+ cells in the tissue sections was also FoxP3+. These double positive cells were found in 80% of the unpretreated and 56% of the pretreated samples with CD4+ TILs in the stroma, suggesting that cisplatin and/or pemetrexed have a negative effect on the number of CD4+FoxP3+ cells, which might be Treg. This idea is supported by data from Wu et al.Citation50 who reported a decreased number of Tregs in a mesothelioma mouse model after treatment with cisplatin. We found a positive correlation between Tregs and PD-1+, PD-L1+ and CD4+ TILs in the stroma. The more activation of the PD-1/PD-L1 pathway, the less FoxP3 transcription is controlled, eventually resulting in an increased amount of CD4+FoxP3+ cells.Citation30 However, FoxP3 expression is also described in activated non-suppressive T cell populations, so it is not a 100% specific Treg marker.Citation51 More CD4+FoxP3+ cells in the stroma were also associated with more CD68+ macrophages in the stroma, suggesting that the latter affect the adaptive immune response by secreting several molecules that lead to recruitment and stimulation of CD4+ T cells, as previously described by Solinas et al.Citation52 CD4+FoxP3+ cells were negatively correlated with CD8+ TILs in the stroma, as also found for CD4+ and CD8+ TILs: the more CD4+ TILs, the more CD4+FoxP3+ cells and the less CD8+ TILs. As observed for CD8+ TILs, CD45RO+ memory T cells were also seen in the stroma of all samples. The latter were significantly associated with response to chemotherapy. More specifically, our data show CD45RO expression in the stroma to be an independent negative predictive factor. MPM patients with many CD45RO+ T cells had a higher likelihood of non-responding to chemotherapy compare with those with few CD45RO+ T cells, suggesting that CD45RO is an interesting predictive marker for response to chemotherapy in MPM patients.
Stromal expression of CD45RO was significantly correlated with the presence of CD4+FoxP3+ cells in the stroma. Under the assumption that the latter are Tregs, the negative predictive value of CD45RO expression might be explained by a Treg-mediated suppression of the immune cells that are recruited after cisplatin and pemetrexed treatment.Citation25,53 In addition, a significant correlation was also found for CD4+FoxP3+ cells and CD68+ macrophages in the stroma (R = 0.410, p = 0.002). Tumor-associated macrophages (TAMs) in mesothelioma have been described to be of the tumor promoting M2-phenotype.Citation54,55 M2 macrophages secrete several molecules, such as IL-10 and transforming growth factor-bèta (TGF-β) that results in downregulation of adaptive immunity, for example, via stimulation and recruitment of Tregs.Citation52 The latter might explain our correlation observed for Treg and macrophages in the stroma. Though much has been described about the prognostic role of CD45RO, more research is necessary on its predictive role.
Conclusion
In conclusion, we report that the immune composition of the TME and expression of immune checkpoints and their ligands is strongly patient dependent. In future research it would be interesting to investigate the within patient dynamics of the TME in a prospective study. Our results point to TIM-3 as a promising new target in mesothelioma. Regarding combination of chemotherapy with immunotherapy, larger validation cohorts are needed to determine the best treatment schedule.
Materials and methods
Patient selection and samples
Formalin-fixed paraffin embedded (FFPE) tissue samples from 54 MPM patients were retrieved from the tumor biobank at the Antwerp University Hospital. Of those, 40 patients were unpretreated and 14 were pretreated with standard chemotherapy (platinum and pemetrexed). Exclusion criteria for the untreated group were prior surgery or chemotherapy treatment and tissue samples older than 10 y. The latter exclusion criterion was also used for the pretreated group. Response was assessed with modified RECIST criteria and confirmed after at least 4 weeks.Citation56 All the tissue samples had been obtained through surgical biopsy, fixed in 4% formaldehyde for 6–18 h and paraffin embedded on a routine basis. This retrospective study has been approved by the Ethics Committee of the Antwerp University Hospital/University of Antwerp.
Immunohistochemistry
Five µm-thick sections were prepared from FFPE tissue blocks. Prior to staining, the sections were baked in an oven for 1 h at 60°C to facilitate attachment and to soften the paraffin. IHC was performed on a Benchmark Ultra XT© autostainer (Ventana Medical Systems Inc.) with standard antigen retrieval methods (CC1, pH 8.0; Ventana, #950–124). The UltraView or OptiView DAB detection kit (Ventana, #760–500 or #760–700) and, in case of double stainings for CD4+/CD8+ and CD4+/FoxP3, the UltraView universal alkaline phosphatase red detection kit (Ventana, #760–501) were used according to the manufacturer's instructions. Sections were counterstained with hematoxylin as part of the automated staining protocol. After staining, slides were washed in reaction buffer (Ventana, #950–300), dehydrated in graded alcohol, cleared in xylene, mounted with Quick-D Mounting Medium (Klinipath, #7280) and coverslipped. IHC staining protocols from the manufacturer's datasheets were used for the following antibodies from Ventana: anti PD-1 (clone NAT105, #760–4895), anti CD4+ (clone SP35, #790–4423), anti CD8+ (clone SP57, #790–4460), anti CD45RO (clone UCHL-1, #790–2930). The protocols for PD-L1 (clone SP142, 1:50, Spring Bioscience, #7309457001), CD68 (clone KP-1, 52′ CC1, 12′ antibody incubation, Ventana, #790–2931) and granzyme B (polyclonal, 32′ antibody incubation, Ventana, #760–4283) were slightly adapted from the datasheet. This was also the case for the antibodies used from Abcamࣨ (Abcamࣨ): anti LAG-3 (clone 11E3, 1:100, 36′ CC1, 60′ antibody incubation, #ab4065), anti-TIM-3 (polyclonal, 1:500, 52′ CC1, 28′ antibody incubation, #185703) and anti FoxP3 (clone 236A/E7, 1:50, 36′ CC1, #ab20034).
Positive controls were included in each staining run. Human placenta was included as positive control for endogenous PD-L1, while human tonsil was used as positive control for the other markers. We looked at the presence of lymphocytes, lymphoid aggregates (score 0 = 0 aggregates; 1 = 1–5 aggregates; 2 = 5–10 aggregates; 3 = >10 aggregates) and the density of the stroma (score 0 = scarce; 1 = low density; 2 = intermediate density; 3 = high densityCitation57). A lymphoid aggregate was defined as 50 or more lymphocytes clustered together. Expression of each marker in the tissue was divided into five categories (0 = <1%; 1 = 1–5%; 2 = 5–10%; 3 = 10–50%: 4 = >50%). All sections were scored by two observers, of whom one pathologist. Samples were considered to be positive in case of ≥1% positive cellsCitation7,8 with specific staining of any intensity (0 = no expression, 1 = weak, 2 = moderate, 3 = strong) and any distribution (membrane and/or cytoplasm).Citation7 These criteria were used for IHC scoring of all the different markers. An Olympus BX41 microscope was used for scoring of the tissue sections. Pictures were made using the Leica acquisition software v4.
Statistics
Spearman correlation coefficients were calculated to investigate the association between the expression of immune cell markers and immune checkpoints on MPM tissue samples. Overall survival was assessed from the date of diagnosis to the date of last contact or decease. Survival rates were visualized using Kaplan-Meier curves. The influence of immunological and clinicopathological parameters on survival was assessed using Cox proportional hazards models. Variables that showed significance (10%) in univariate analyses were considered for the multivariate model. We used tests based on the scaled Schoenfeld residuals to assess the proportional hazards assumption. The association of immunological and clinicopathological variables with response to chemotherapy was analyzed using logistic regression on 32 of 54 samples (treatment or response data not available for the other samples). Variable selection was performed by assessing significance on the 10% level in univariate analyses. Depending on the number of selected variables, forward or backward model building was used in the multivariate models. p-values ≤ 0.05 were considered statistically significant. All statistical analyses were performed using R statistical software version 3.2.2.
Disclosure of potential conflicts of interest
No potential conflicts of interest were disclosed.
KONI_A_1261241Supplementary_materials.zip
Download Zip (53.5 KB)Acknowledgments
The human biological material used in this publication was provided by the UZA Tumor bank, Antwerp University Hospital, Belgium, which is funded by the National Cancer Plan. The authors furthermore express their gratitude to Prof. Dr Paul Van Schil from the Department of Thoracic and Vascular Surgery for the collection of the samples and to the data managers Lesley De Backer and Greetje Vanhoutte from the Multidisciplinary Center for Oncological Research for their help with collecting the clinicopathological data.
Funding
This work was performed with the support of the Belgian Foundation Against Cancer (grant number: FA/2014/263), the Research Foundation Flanders (grant number: 1510215N), AstraZeneca and a Methusalem grant of the University of Antwerp and Hasselt awarded to Prof. Herman Goossens and Prof Geert Molenberghs. E. Marcq is research fellow of Flanders Innovation & Entrepreneurship (fellowship number: 141433), J. De Waele and J. Van Audenaerde of the Research Foundation Flanders (fellowship numbers: 1121016N and 1S32316N).
References
- Robinson BW, Lake RA. Advances in malignant mesothelioma. N Engl J Med 2005; 353:1591-603; PMID:16221782; http://dx.doi.org/10.1056/NEJMra050152
- van Meerbeeck JP, Gaafar R, Manegold C, Van Klaveren RJ, Van Marck EA, Vincent M, Legrand C, Bottomley A, Debruyne C, Giaccone G. Randomized phase III study of cisplatin with or without raltitrexed in patients with malignant pleural mesothelioma: an intergroup study of the European organisation for research and treatment of cancer lung cancer group and the National Cancer Institute of Canada. J Clin Oncol 2005; 23:6881-9; PMID:16192580; http://dx.doi.org/10.1200/JCO.20005.14.589
- Vogelzang NJ, Rusthoven JJ, Symanowski J, Denham C, Kaukel E, Ruffie P, Gatzemeier U, Boyer M, Emri S, Manegold C et al. Phase III study of pemetrexed in combination with cisplatin versus cisplatin alone in patients with malignant pleural mesothelioma. J Clin Oncol 2003; 21:2636-44; PMID:12860938; http://dx.doi.org/10.1200/JCO.2003.11.136
- Muers MF, Stephens RJ, Fisher P, Darlison L, Higgs CM, Lowry E, Nicholson AG, O'Brien M, Peake M, Rudd R et al. Active symptom control with or without chemotherapy in the treatment of patients with malignant pleural mesothelioma (MS01): a multicentre randomised trial. Lancet 2008; 371:1685-94; PMID:18486741; http://dx.doi.org/10.1016/S0140-6736(08)60727-8
- Marcq E, Pauwels P, van Meerbeeck JP, Smits EL. Targeting immune checkpoints: New opportunity for mesothelioma treatment? Cancer Treat Rev 2015; 41:914-24; PMID:26433514; http://dx.doi.org/10.1016/j.ctrv.2015.09.006
- Alley EW, Molife LR, Santoro A, Beckey K, Yuan S, Cheng JD, Piperdi B, Schellens J.H.M. Clinical safety and efficacy of pembrolizumab (MK-3475) in patients with malignant pleural mesothelioma: Preliminary results from KEYNOTE-028.[abstract]. Proc 16th Annual Meeting Am Association Cancer Res 2015; 18-22; 75(15 Suppl):Abstract nr CT103; http://dx.doi.org/10.1158/1538-7445.AM2015-CT103.
- Cedres S, Ponce-Aix S, Zugazagoitia J, Sansano I, Enguita A, Navarro-Mendivil A, Martinez-Marti A, Martinez P, Felip E. Analysis of Expression of Programmed Cell Death 1 Ligand 1 (PD-L1) in Malignant Pleural Mesothelioma (MPM). PLoS One 2015; 10:e0121071; PMID:25774992; http://dx.doi.org/10.1371/journal.pone.0121071
- Combaz-Lair C, Galateau-Salle F, McLeer-Florin A, Le SN, David-Boudet L, Duruisseaux M, Ferretti GR, Brambilla E, Lebecque S, Lantuejoul S. Immune biomarkers PD-1/PD-L1 and TLR3 in malignant pleural mesotheliomas. Hum Pathol 2016; 52:9-18; PMID:26980049; http://dx.doi.org/10.1016/j.humpath.2016.01.010
- Cowan ML, Forde PM, Taube JM, Illei PB. PD-L1 expression in malignant mesothelioma: an immunohistochemical analysis of 33 cases. [abstract]. Lab Invest 2014; 94:472-500; http://dx.doi.org/10.1038/labinvest.2014.36
- Kindler HL, Zuo Z, Khattri A, Keck MK, Vigneswaran W, Husain AN, Seiwert TY. T-cell inflamed phenotype and PDL1 expression in malignant mesothelioma. [abstract]. J Clin Oncol 2014; 32:5s; 2014 (suppl; abstr 7589)
- Mansfield AS, Roden AC, Peikert T, Sheinin YM, Harrington SM, Krco CJ, Dong H, Kwon ED. B7-H1 expression in malignant pleural mesothelioma is associated with sarcomatoid histology and poor prognosis. J Thorac Oncol 2014; 9:1036-40; PMID:24926549; http://dx.doi.org/10.1097/JTO.0000000000000177
- Grabosh S, Zeng F, Zhang L, Strange M, Brozick J, Edwards RP, Vlad A. PD-L1 biology in response to chemotherapy in vitro and in vivo in ovarian cancer. J ImmunoTherapy Cancer 2015; 3(Suppl 2):P302; http://dx.doi.org/10.1186/2051-1426-3-S2-P302
- Katsuya Y, Horinouchi H, Asao T, Kitahara S, Goto Y, Kanda S, Fujiwara Y, Nokihara H, Yamamoto N, Watanabe S et al. Expression of programmed death 1 (PD-1) and its ligand (PD-L1) in thymic epithelial tumors: impact on treatment efficacy and alteration in expression after chemotherapy. Lung Cancer 2016; 99:4-10; PMID:27565906; http://dx.doi.org/10.1016/j.lungcan.2016.05.007
- Gassner FJ, Zaborsky N, Neureiter D, Huemer M, Melchardt T, Egle A, Rebhandl S, Catakovic K, Hartmann TN, Greil R et al. Chemotherapy-induced augmentation of T cells expressing inhibitory receptors is reversed by treatment with lenalidomide in chronic lymphocytic leukemia. Haematologica 2014; 99:67-9; PMID:24561794; http://dx.doi.org/10.3324/haematol.2013.098459
- Peng J, Hamanishi J, Matsumura N, Abiko K, Murat K, Baba T, Yamaguchi K, Horikawa N, Hosoe Y, Murphy SK et al. Chemotherapy Induces Programmed Cell Death-Ligand 1 Overexpression via the Nuclear Factor-kappaB to Foster an Immunosuppressive Tumor Microenvironment in Ovarian Cancer. Cancer Res 2015; 75:5034-45; PMID:26573793; http://dx.doi.org/10.1158/0008-5472.CAN-14-3098
- Apetoh L, Ladoire S, Coukos G, Ghiringhelli F. Combining immunotherapy and anticancer agents: the right path to achieve cancer cure? Ann Oncol 2015; 26:1813-23; PMID:25922066; http://dx.doi.org/10.1093/annonc/mdv209
- Lesterhuis WJ, Salmons J, Nowak AK, Rozali EN, Khong A, Dick IM, Harken JA, Robinson BW, Lake RA. Synergistic effect of CTLA-4 blockade and cancer chemotherapy in the induction of anti-tumor immunity. PLoS One 2013; 8:e61895; PMID:23626745; http://dx.doi.org/10.1371/journal.pone.0061895
- Mokyr MB, Kalinichenko T, Gorelik L, Bluestone JA. Realization of the therapeutic potential of CTLA-4 blockade in low-dose chemotherapy-treated tumor-bearing mice. Cancer Res 1998; 58:5301-4; PMID:9850053
- Xu W, Narayanan P, Kang N, Clayton S, Ohne Y, Shi P, Herve MC, Balderas R, Picard C, Casanova JL et al. Human plasma cells express granzyme B. Eur J Immunol 2014; 44:275-84; PMID:24114594; http://dx.doi.org/10.1002/eji.201343711
- Strik MC, de Koning PJ, Kleijmeer MJ, Bladergroen BA, Wolbink AM, Griffith JM, Wouters D, Fukuoka Y, Schwartz LB, Hack CE et al. Human mast cells produce and release the cytotoxic lymphocyte associated protease granzyme B upon activation. Mol Immunol 2007; 44:3462-72; PMID:17485116; http://dx.doi.org/10.1016/j.molimm.2007.03.024
- Voss A, Xiu J, Millis S, Gatalica Z. Pleural malignant mesotheliomas over-express PD-L1. [ abstract]. Annals Oncol 2015; 26 (suppl_1):48-50; http://dx.doi.org/10.1093/annonc/mdv052
- Teng MW, Ngiow SF, Ribas A, Smyth MJ. Classifying Cancers Based on T-cell Infiltration and PD-L1. Cancer Res 2015; 75:2139-45; PMID:25977340; http://dx.doi.org/10.1158/0008-5472.CAN-15-0255
- Taube JM, Klein A, Brahmer JR, Xu H, Pan X, Kim JH, Chen L, Pardoll DM, Topalian SL, Anders RA. Association of PD-1, PD-1 ligands, and other features of the tumor immune microenvironment with response to anti-PD-1 therapy. Clin Cancer Res 2014; 20:5064-74; PMID:24714771; http://dx.doi.org/10.1158/1078-0432.CCR-13-3271
- Zhang L, Du H, Xiao TW, Liu JZ, Liu GZ, Wang JX, Li GY, Wang LX. Prognostic value of PD-1 and TIM-3 on CD3+ T cells from diffuse large B-cell lymphoma. Biomed Pharmacother 2015; 75:83-7; PMID:26463635; http://dx.doi.org/10.1016/j.biopha.2015.08.037
- Anraku M, Tagawa T, Wu L, Yun Z, Keshavjee S, Zhang L, Johnston MR, de PM. Synergistic antitumor effects of regulatory T cell blockade combined with pemetrexed in murine malignant mesothelioma. J Immunol 2010; 185:956-66; PMID:20548032; http://dx.doi.org/10.4049/jimmunol.0900437
- Wei F, Zhong S, Ma Z, Kong H, Medvec A, Ahmed R, Freeman GJ, Krogsgaard M, Riley JL. Strength of PD-1 signaling differentially affects T-cell effector functions. Proc Natl Acad Sci U S A 2013; 110:E2480-E2489; PMID:23610399; http://dx.doi.org/10.1073/pnas.1305394110
- Wiener Z, Kohalmi B, Pocza P, Jeager J, Tolgyesi G, Toth S, Gorbe E, Papp Z, Falus A. TIM-3 is expressed in melanoma cells and is upregulated in TGF-beta stimulated mast cells. J Invest Dermatol 2007; 127:906-14; PMID:17096021; http://dx.doi.org/10.1038/sj.jid.5700616
- Komohara Y, Morita T, Annan DA, Horlad H, Ohnishi K, Yamada S, Nakayama T, Kitada S, Suzu S, Kinoshita I et al. The Coordinated Actions of TIM-3 on Cancer and Myeloid Cells in the Regulation of Tumorigenicity and Clinical Prognosis in Clear Cell Renal Cell Carcinomas. Cancer Immunol Res 2015; 3:999-1007; PMID:25783986; http://dx.doi.org/10.1158/2326-6066.CIR-14-0156
- Zhuang X, Zhang X, Xia X, Zhang C, Liang X, Gao L, Zhang X, Ma C. Ectopic expression of TIM-3 in lung cancers: a potential independent prognostic factor for patients with NSCLC. Am J Clin Pathol 2012; 137:978-85; PMID:22586058; http://dx.doi.org/10.1309/AJCP9Q6OVLVSHTMY
- Ngiow SF, von SB, Akiba H, Yagita H, Teng MW, Smyth MJ. Anti-TIM3 antibody promotes T cell IFN-gamma-mediated antitumor immunity and suppresses established tumors. Cancer Res 2011; 71:3540-51; PMID:21430066; http://dx.doi.org/10.1158/0008-5472.CAN-11-0096
- Sakuishi K, Apetoh L, Sullivan JM, Blazar BR, Kuchroo VK, Anderson AC. Targeting Tim-3 and PD-1 pathways to reverse T cell exhaustion and restore anti-tumor immunity. J Exp Med 2010; 207:2187-94; PMID:20819927; http://dx.doi.org/10.1084/jem.20100643
- Hassan R, THomas A, Patel MR, Nemunaitis JJ, Bennouana J, Powderly JD, Taylor MH, Dowlati A, Chen F, Leach J et al. Avelumab (MSB0010718C; anti-PD-L1) in patients with advanced unresectable mesothelioma from the JAVELIN solid tumor phase Ib trial: Safety, clinical activity, and PD-L1 expression. [abstract]. J Clin Oncol 2016; 34; PMID:27863199
- Sheng J, Fang W, Yu J, Chen N, Zhan J, Ma Y, Yang Y, Huang Y, Zhao H, Zhang L. Erratum: Expression of programmed death ligand-1 on tumor cells varies pre and post chemotherapy in non-small cell lung cancer. Sci Rep 2016; 6:23850; PMID:27072409; http://dx.doi.org/10.1038/srep23850
- Ghebeh H, Mohammed S, Al-Omair A, Qattan A, Lehe C, Al-Qudaihi G, Elkum N, Alshabanah M, Bin AS, Tulbah A et al. The B7-H1 (PD-L1) T lymphocyte-inhibitory molecule is expressed in breast cancer patients with infiltrating ductal carcinoma: correlation with important high-risk prognostic factors. Neoplasia 2006; 8:190-8; PMID:16611412; http://dx.doi.org/10.1593/neo.05733
- Lastwika KJ, Wilson W, III, Li QK, Norris J, Xu H, Ghazarian SR, Kitagawa H, Kawabata S, Taube JM, Yao S et al. Control of PD-L1 Expression by Oncogenic Activation of the AKT-mTOR Pathway in Non-Small Cell Lung Cancer. Cancer Res 2016; 76:227-38; PMID:26637667; http://dx.doi.org/10.1158/0008-5472.CAN-14-3362
- de Biasi AR, Villena-Vargas J, Adusumilli PS. Cisplatin-induced antitumor immunomodulation: a review of preclinical and clinical evidence. Clin Cancer Res 2014; 20:5384-91; PMID:25204552; http://dx.doi.org/10.1158/1078-0432.CCR-14-1298
- Anraku M, Cunningham KS, Yun Z, Tsao MS, Zhang L, Keshavjee S, Johnston MR, de PM. Impact of tumor-infiltrating T cells on survival in patients with malignant pleural mesothelioma. J Thorac Cardiovasc Surg 2008; 135:823-9; PMID:18374762; http://dx.doi.org/10.1016/j.jtcvs.2007.10.026
- Mudhar HS, Fisher PM, Wallace WA. No relationship between tumour infiltrating lymphocytes and overall survival is seen in malignant mesothelioma of the pleura. Eur J Surg Oncol 2002; 28:564-5; PMID:12217312; http://dx.doi.org/10.1053/ejso.2002.1294
- Yamada N, Oizumi S, Kikuchi E, Shinagawa N, Konishi-Sakakibara J, Ishimine A, Aoe K, Gemba K, Kishimoto T, Torigoe T et al. CD8+ tumor-infiltrating lymphocytes predict favorable prognosis in malignant pleural mesothelioma after resection. Cancer Immunol Immunother 2010; 59:1543-9; PMID:20567822; http://dx.doi.org/10.1007/s00262-010-0881-6
- Leigh AR, Webster I. Lymphocytic infiltration of pleural mesothelioma and its significance for survival. S Afr Med J 1982; 61:1007-9; PMID:7089768
- Hiraoka K, Miyamoto M, Cho Y, Suzuoki M, Oshikiri T, Nakakubo Y, Itoh T, Ohbuchi T, Kondo S, Katoh H. Concurrent infiltration by CD8+ T cells and CD4+ T cells is a favourable prognostic factor in non-small-cell lung carcinoma. Br J Cancer 2006; 94:275-80; PMID:16421594; http://dx.doi.org/10.1038/sj.bjc.6602934
- Al-Shibli KI, Donnem T, Al-Saad S, Persson M, Bremnes RM, Busund LT. Prognostic effect of epithelial and stromal lymphocyte infiltration in non-small cell lung cancer. Clin Cancer Res 2008; 14:5220-7; PMID:18698040; http://dx.doi.org/10.1158/1078-0432.CCR-08-0133
- Wakabayashi O, Yamazaki K, Oizumi S, Hommura F, Kinoshita I, Ogura S, Dosaka-Akita H, Nishimura M. CD4+ T cells in cancer stroma, not CD8+ T cells in cancer cell nests, are associated with favorable prognosis in human non-small cell lung cancers. Cancer Sci 2003; 94:1003-9; PMID:14611679; http://dx.doi.org/10.1111/j.1349-7006.2003.tb01392.x
- Zhu J, Paul WE. CD4 T cells: fates, functions, and faults. Blood 2008; 112:1557-69; PMID:18725574; http://dx.doi.org/10.1182/blood-2008-05-078154
- Neurath MF, Finotto S. The emerging role of T cell cytokines in non-small cell lung cancer. Cytokine Growth Factor Rev 2012; 23:315-22; PMID:23022528; http://dx.doi.org/10.1016/j.cytogfr.2012.08.009
- Friedman KM, Prieto PA, Devillier LE, Gross CA, Yang JC, Wunderlich JR, Rosenberg SA, Dudley ME. Tumor-specific CD4+ melanoma tumor-infiltrating lymphocytes. J Immunother 2012; 35:400-8; PMID:22576345; http://dx.doi.org/10.1097/CJI.0b013e31825898c5
- Pages F, Galon J, Dieu-Nosjean MC, Tartour E, Sautes-Fridman C, Fridman WH. Immune infiltration in human tumors: a prognostic factor that should not be ignored. Oncogene 2010; 29:1093-102; PMID:19946335; http://dx.doi.org/10.1038/onc.2009.416
- Fridman WH, Pages F, Sautes-Fridman C, Galon J. The immune contexture in human tumours: impact on clinical outcome. Nat Rev Cancer 2012; 12:298-306; PMID:22419253; http://dx.doi.org/10.1038/nrc3245
- Goc J, Fridman WH, Sautes-Fridman C, Dieu-Nosjean MC. Characteristics of tertiary lymphoid structures in primary cancers. Oncoimmunology 2013; 2:e26836; PMID:24498556; http://dx.doi.org/10.4161/onci.26836
- Wu L, Yun Z, Tagawa T, Rey-McIntyre K, Anraku M, de PM. Tumor cell repopulation between cycles of chemotherapy is inhibited by regulatory T-cell depletion in a murine mesothelioma model. J Thorac Oncol 2011; 6:1578-86; PMID:21642867; http://dx.doi.org/10.1097/JTO.0b013e3182208ee0
- Wang J, Ioan-Facsinay A, van der Voort EI, Huizinga TW, Toes RE. Transient expression of FOXP3 in human activated nonregulatory CD4+ T cells. Eur J Immunol 2007; 37:129-38; PMID:17154262; http://dx.doi.org/10.1002/eji.200636435
- Solinas G, Germano G, Mantovani A, Allavena P. Tumor-associated macrophages (TAM) as major players of the cancer-related inflammation. J Leukoc Biol 2009; 86:1065-73; PMID:19741157; http://dx.doi.org/10.1189/jlb.0609385
- Bracci L, Schiavoni G, Sistigu A, Belardelli F. Immune-based mechanisms of cytotoxic chemotherapy: implications for the design of novel and rationale-based combined treatments against cancer. Cell Death Differ 2014; 21:15-25; PMID:23787994; http://dx.doi.org/10.1038/cdd.2013.67
- Izzi V, Masuelli L, Tresoldi I, Foti C, Modesti A, Bei R. Immunity and malignant mesothelioma: from mesothelial cell damage to tumor development and immune response-based therapies. Cancer Lett 2012; 322:18-34; PMID:22394996; http://dx.doi.org/10.1016/j.canlet.2012.02.034
- Lievense LA, Bezemer K, Aerts JG, Hegmans JP. Tumor-associated macrophages in thoracic malignancies. Lung Cancer 2013; 80:256-62; PMID:23489559; http://dx.doi.org/10.1016/j.lungcan.2013.02.017
- Byrne MJ, Nowak AK. Modified RECIST criteria for assessment of response in malignant pleural mesothelioma. Ann Oncol 2004; 15:257-60; PMID:14760119; http://dx.doi.org/10.1093/annonc/mdh059
- Donnem T, Al-Saad S, Al-Shibli K, Andersen S, Busund LT, Bremnes RM. Prognostic impact of platelet-derived growth factors in non-small cell lung cancer tumor and stromal cells. J Thorac Oncol 2008; 3:963-70; PMID:18758297; http://dx.doi.org/10.1097/JTO.0b013e3181834f52