ABSTRACT
Regulatory B cells (Bregs) are involved in the pathogenesis of graft-versus-host disease (GVHD). However, whether Bregs can alleviate acute GVHD without compromising graft-versus-leukemia (GVL) effects remains unclear. Here, we evaluated the role of Bregs in acute GVHD and GVL activity in both a mouse model and a clinical cohort study. In the acute GVHD mouse model, co-transplantation of Bregs prevents onset through inhibiting Th1 and Th17 differentiation and expanding regulatory T cells. In the GVL mouse model, Bregs contributed to the suppression of acute GVHD but had no adverse effect on GVL activity. In the clinical cohort study, a higher dose of Bregs in allografts was associated with a lower cumulative incidence of acute GVHD but not with increased risk of relapse. Our data demonstrate that Bregs can prevent acute GVHD and maintain GVL effects and suggest that Bregs have potential as a novel strategy for acute GVHD alleviation.
Introduction
Allogeneic stem cell transplantation (allo-SCT) is limited by the major lethal complication of graft-versus-host disease (GVHD).Citation1-4 Prophylaxis and treatment of GVHD have been restricted to either functional inactivation of donor T cells using immunosuppressive drugs, or depletion of donor T cells.Citation1,3 These strategies might lead to significant toxicities of the immunosuppressants, delayed immune reconstitution, a high rate of opportunistic infections, and increased risk of hematological malignant relapse. Therefore, several regulatory cells, including CD4+CD25+ regulatory T cells (Tregs), myeloid-derived suppressor cells,Citation5 and mesenchymal stromal cells (MSCs),Citation6-8 have been investigated to elucidate whether these cells could be used for prevention or therapy of GVHD. Although several studies using a mouse bone marrow transplant (BMT) modelCitation9 have identified Tregs, myeloid-derived suppressor cells, and MSCs as available strategies for GVHD alleviation, none of them can be routinely applied in clinic.Citation10,11
Regulatory B cells (Bregs) are a newly described subset of B cells that appears to play important roles in autoimmunity.Citation12-17 Human Bregs were enriched in both transitional (CD24hiCD38hi) and memory (CD24hiCD27+) B cells; in mice, they are enriched within the CD1dhighCD5+CD19high subpopulation. In 2006, Rowe et al.Citation18 reported that host B cells produce interleukin (IL)-10 following total body irradiation and attenuate acute GVHD after allogeneic BMT. Le Huu et al.Citation19 observed that donor-derived B10 cells have a suppressive role in Sclerodermatous chronic GVHD development. In addition, the quantity and quality impairment of Bregs was also observed in human chronic GVHD.Citation20,21 However, it remains unclear whether Bregs could alleviate acute GVHD while maintaining graft-versus-leukemia (GVL) effects. Here, we show that the immunosuppressive potential of Bregs can be used to suppress acute GVHD without compromising the effects of GVL activity based on results from a mouse model and a clinical cohort study.
Results
Bregs significantly reduce the severity of acute GVHD in a mouse model
To test whether Bregs can be implemented as cellular GVHD therapy following BMT, we used a classical GVHD mouse model (B6 into BALB/c). Lethally irradiated BALB/c mice were transplanted with 5 × 106 B6 TCD-BM cells with or without 3 × 106 B6 spleen T cells. BMT recipients were injected i.v. in the tail with either Bregs (3 × 106) or phosphate-buffered saline immediately after the transplant. As expected, BALB/c mice receiving B6 TCD-BM alone developed no sign of GVHD whereas all control BALB/c mice receiving B6 donor TCD-BM + spleen T cells died of GVHD (). In contrast, injection of Bregs inhibited GVHD in T cell recipients, with 20% more of them surviving without severe GVHD (). This survival was accompanied by a lower clinical score in Bregs recipients (). We performed histological examination of GVHD target organs (liver, skin, intestine, and colon) at 1 and 2 weeks after transplantation. There was a significant reduction in pathological damage in all sites of Bregs recipients compared with control mice (). Thus, injection of Bregs had a salutary effect on the severity of acute GVHD.
Figure 1. Injection of Breg ameliorates GVHD. Lethally irradiated BALB/c recipients (8 Gy) were transplanted with 5 × 106 TCD-BM derived from B6 mice (n = 11) or with TCD-BM plus spleen T cells (n = 14). Breg (3 × 106) was injected i.p. into T cell recipients at the time of transplantation (n = 15). (A) The survival of BMT recipients was monitored over time (p < 0.01). (B) Recipient mice were assessed every 2 d for clinical severity of GVHD; clinical scores are shown (p < 0.05). (C) Histopathology of skin, liver, intestine, and colon of BMT recipients 14 d after transplantation (original magnification × 200). Upper panel is TCD-BM + T cells + Breg group; middle panel is TCD-BM + T cells group; and lower panel is TCD-BM group. (D) Pathologic damage in the intestine, colon, skin, and liver was assessed using a semi-quantitative scoring system, as described in materials and methods. Results are representative of three independent experiments. Data are mean ± SEM.
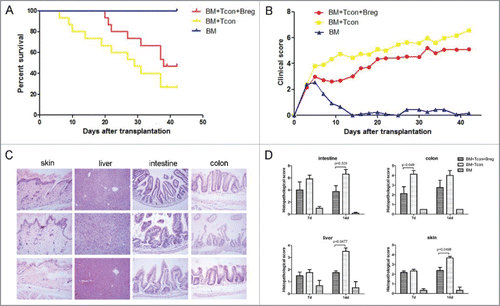
Bregs modulate Th cell balance
GVHD is characterized by the differentiation of T cells present in the graft.Citation22 Excessive production of cytokines such as IL-1β, IL-6, IL-17, and IFNγ by differentiated T cells can lead to an inflammatory response and cause damage to several host tissues in GVHD.Citation22 To examine whether Bregs could regulate Th cell response, we analyzed Th cell subsets in peripheral blood, bone marrow, and spleen of recipients on the indicated days after transplantation. Flow cytometry analysis showed that compared with control, IL-4-positive CD4+ T cells derived from spleen significantly increased in the Breg injection group at the early stage after transplantation (). We also detected substantially lower serum levels of Th1- and Th17-related cytokines (TNF-a and IFNγ) in the Breg injection group ().
Figure 2. Bregs modulate Th cell balance in GVHD. Lethally irradiated BALB/c recipients were transplanted with TCD-BM derived from B6 mice or with TCD-BM plus spleen T cells. Breg (3 × 106) was injected into T cell recipients at the time of transplantation. (A) Flow cytometry analysis of intracellular IL-4 on CD4+ T cells from spleen on indicated days. (B) TNF-α and IFNγ concentrations were determined in the serum of recipient mice 7 d after BMT. (C) Flow cytometry analysis of transcription factor T-bet, GATA3, and RORγt on CD4+ T cells from spleen (SP) of recipients with and without Breg injection on the indicated days. (D) Th2/Th1 and Th2/(Th1+Th17) ratios in peripheral blood, bone marrow, and spleen of recipients with and without Breg injection on the indicated days. Results are representative of three independent experiments with three mice per group per experiment. Data are mean ± SEM. *p < 0.05, **p < 0.01, ***p < 0.001.
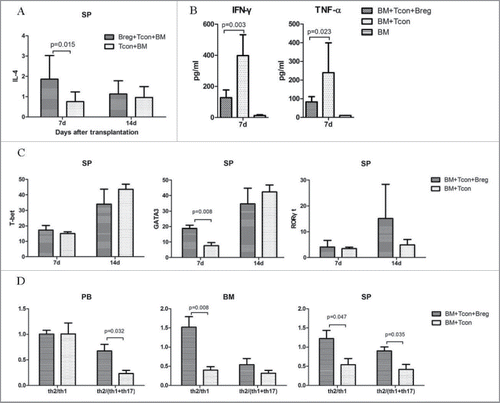
The expression of RORγt and T-bet, which is the key transcription factor of Th17 and Th1 cell differentiation, was decreased in blood and bone marrow CD4+ T cells in the Breg injection group (Fig. S1). On the other hand, the expression of the Th2-specific gene GATA3 in splenic CD4+ T cells was significantly higher than in the controls (). Breg injection markedly raised the Th2/Th1 and Th2/(Th1+Th17) ratios in peripheral blood, bone marrow, and spleen (). Taken together, these data suggest that the polarization of T cells from Th1 to Th2 may be the primary cause of Breg-mediated GVHD inhibition.
Bregs attenuate GVHD via regulatory Tregs
While playing key roles in suppressing autoimmunity and in maintaining immune homeostasis, Tregs can reduce the severity of GVHD. Therefore, we wondered whether the effect of Bregs on GVHD resided in their effect of promoting Tregs and quantified the frequencies and absolute numbers of Foxp3-expressing CD4+ T cells in peripheral blood on the indicated days after transplantation. Recipients injected with Bregs showed a significantly higher frequency of Foxp3+ cells (). Ratios of Treg/Th1 and Treg/(Th1+Th17) also were significantly increased in Breg injection recipients compared with the control group on day 7 (). Therefore, these data suggest that the effect of Bregs on GVHD prevention is associated with Tregs.
Figure 3. Bregs attenuate GVHD via Tregs in vivo. (A) Flow cytometry analysis results showing representative percentages of donor-derived peripheral blood and bone marrow T cells expressing Foxp3 on days 7 and 14. (B) Treg/Th1 and Treg/(Th1+Th17) ratios in peripheral blood of recipients with and without Breg injection on the indicated days. (C) Immunohistochemical staining to assess the number of T-bet-, GATA3-, RORγt-, and Foxp3-expressing cells in the skin, intestine, colon, liver, and lung on days 7 and 14. Results are representative of three independent experiments with three mice per group per experiment. Data are mean ± SEM. *p < 0.05, **p < 0.01. ns, p > 0.05.
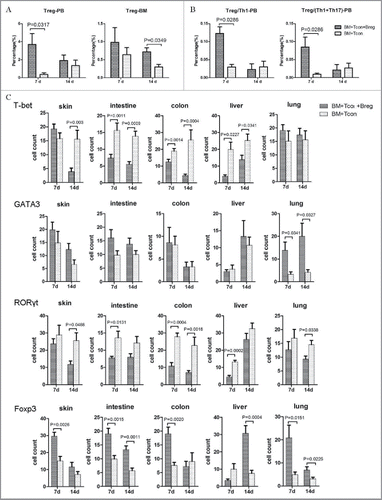
To ascertain the protective functions of Bregs in GVHD target organs, the expression of T-bet, GATA3, RORγt, and Foxp3 in the skin, intestine, colon, liver, and lung were assessed by immunohistochemical staining. Microscopy analysis revealed that the number of T-bet+ and RORγt+ cells was significantly reduced when Bregs were injected. However, the number of cells expressing GATA3 in lung from the Breg injection group was increased significantly (p < 0.05) compared with that of the controls (). In addition, the Foxp3-expressing cells also increased in the Breg injection group (). Taken together, the results indicate that Bregs significantly inhibited T-bet and RORγt expression and expanded the Treg population in GVHD target organs of recipient mice.
Breg preserves GVL activity after BMT
To evaluate the impact of Breg injection on the preservation of GVL activity, we challenged BALB/c recipients with host-type GFP+ acute myeloid leukemia cells (H-2d) to mimic residual leukemia in patients receiving allogeneic BMT. All mice transplanted with TCD-BM and challenged with leukemia cells died from leukemia (). In vivo injection of spleen T cells prolonged the survival of mice transplanted with TCD-BM and challenged with leukemia cells (), indicating a potent GVL effect. However, CD4+ T cells failed to eliminate leukemia, and these mice eventually died from severe GVHD and leukemia. In contrast, Breg injection from the day of transplantation had no adverse effect on the control of leukemia and prolonged the survival of leukemia cell-challenged mice receiving TCD-BM + spleen T cells. The mice injected with Bregs showed less associated GVHD clinical features () and weight loss () than other leukemia injection groups. A representative dot plot for GFP+ leukemia cells was showed (). About 60% GFP+ leukemia cells were found in the leukemia injection group on day 14. In contrast, the Breg and T cell injection limited the leukemia cells to 10–20% (). Autopsy showed spleen and liver enlargement and indicated signs of controlled leukemia in BMT recipients, confirming the GVL effect (). Therefore, Breg injection had no adverse effect on GVL activity.
Figure 4. Bregs preserve the (T)cell-mediated GVL effect while inhibiting GVHD. Lethally irradiated BALB/c recipients were transplanted with B6 TCD-BM, with or without T cells, and challenged or not with leukemia cells (5 × 105/mouse). Bregs were injected at the time of transplantation. (A) Survival was monitored over time. (B) Recipient mice were assessed every 2 d for clinical severity of GVHD; clinical scores are shown. (C) Mean percentage initial body weight of recipients over the first 30 d. (D) A representative dot plot for GFP+ leukemia cells. (E) GFP+ leukemia cells in peripheral blood and bone marrow were counted by flow cytometry on days 7 and 14. (F) On day 14, mice from different groups were killed, and autopsy was performed to observe the enlargement of liver (upper panel) and spleen (lower panel). (G) Comparable mouse general physical status was found in the Breg injection group and bone marrow-transplanted group. From left to right, TCD-BM + T cells + leukemia cells + Breg, TCD-BM + T cells + leukemia cells, TCD-BM + leukemia cells, TCD-BM. Results are representative of three independent experiments with three mice per group per experiment. Data are mean ± SEM. *p < 0.05, **p < 0.01, ***p < 0.001, ****p < 0.0001.
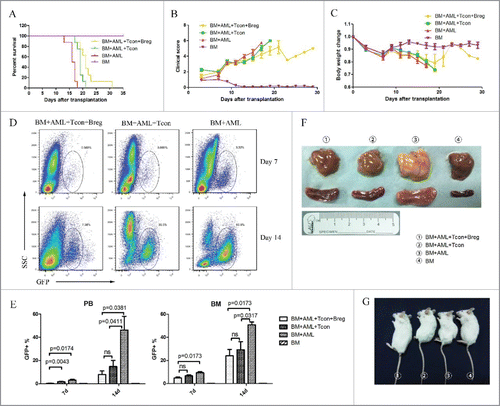
Correlation of Breg in allografts with acute GVHD, but not relapse
All but one subject (who died of infection) exhibited hematopoietic recovery after transplantation. Patients were classified into two groups according to the median dose of Breg in allografts (1.63 × 107 Breg cells infused/kg). These groups had equivalent subject and donor characteristics, including the conditioning regimens, except that cases in the high-dose Breg group received a higher dose of CD34+ cells (p = 0.044), CD19+ cells (p < 0.001), naïve B cells (p < 0.001), and memory B cells (p = 0.031) (). The cumulative, 100-d incidence of acute GVHD grades II–IV in the low-dose Breg group (Group A, 39%; 95% confidence interval (CI), 23–55%) was significantly higher than in the high-dose Breg group (Group B, 16%; 95% CI, 4–28%; p = 0.038; ). In multivariate analysis, infusion of > 1.63 × 107 Breg cells infused/kg decreased the risk of grades II to IV acute GVHD (hazard ratio (HR) = 0.379, 95% CI = 0.146–0.988, p = 0.047).
Table 1. Patient and donor characteristics.
Figure 5. Outcome of allogeneic stem cell transplantations in the cohort of patients. (A) Grades II–IV acute GVHD, (B) relapse, (C) non-relapse mortality, (D) disease-free survival, and (E) overall survival. Patients receiving a high dose of Bregs in allografts (more than or equal to 1.63 × 107 Breg cells infused/kg) (n = 37): green line; subjects receiving a high dose of Bregs in allografts (less than 1.63 × 107 Breg cells infused/kg) (n = 37): blue line.
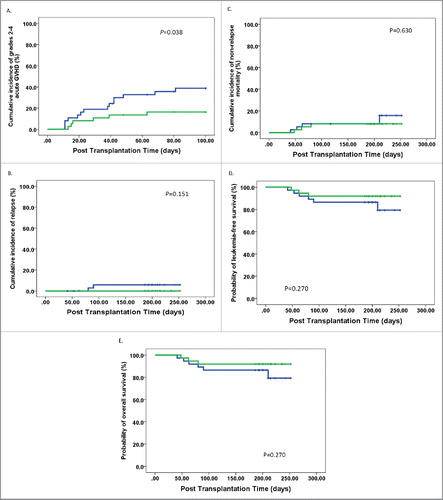
After a median follow-up of 206 d (range 186–252) for surviving patients, the 1-y cumulative incidences of relapse and NRM were 3% (95% CI, 0–7%) and 11% (95% CI, 3–19%), respectively. The 1-y probabilities of disease-free survival (DFS) and overall survival (OS) were 86% (95% CI, 77–95%) and 86% (95% CI, 77–95%), respectively. There was no significant difference in relapse, NRM, DFS, and OS between patients receiving high-dose Bregs and those receiving low-dose Bregs (, ). Multivariate analyses adjusted for variables in , including other B cell subsets, showed that there were no effects of Bregs in allografts on relapse, NRM, DFS, or OS.
Table 2. Transplant outcome of patients that underwent allo-HSCT.
Discussion
Bregs show significant immunosuppressive capacity in vitro and in vivoCitation23 and for this reason, these cells have been tested for treating severe autoimmune diseases.Citation24 In the present study, we observed for the first time that co-transplantation of Bregs significantly ameliorated effective T cell functions by modulating Th cell balance and Tregs and preventing acute GVHD without abrogating the GVT effects in an allogeneic BMT mouse model. In addition, our clinical study also demonstrated that Bregs could alleviate acute GVHD and have no effects on relapse. These data suggest that Bregs have potential as a novel strategy for acute GVHD alleviation.
Acute GVHD is mediated by donor-derived T cells. On activation with alloantigens expressed on host tissues, donor-derived naive CD4+ T cells differentiate into Th cell subsets of effector T cells, such as Th1 and Th17, expressing distinct sets of transcriptional factors and cytokines, which mediate organ-specific GVHD.Citation4,25,26 In this study, we found that co-transplantation of donor-derived Bregs led to a significantly higher ratio of Th2/Th1+Th17 compared with the control group (). Previous work has demonstrated that Th2 cells can alleviate acute GVHD in a mouse model.Citation27,28 In vitro and in vivo experiments also have shown that Bregs can limit Th1 and Th17 differentiation.Citation29 The data reported by others and observed in our study suggest that Bregs can prevent acute GVHD via modulating Th cell balance, which is in agreement with other studies.Citation9,30 The results of both of those earlier studiesCitation9,30 suggested that downregulating Th1 and Th17 cells could ameliorate GVHD.
The effects of Tregs in inhibiting acute GVHD have been reported in several previous studies.Citation9,31,32 Li et al.Citation31 demonstrated that induced Tregs specific for HY miHAgs are highly effective in controlling GVHD in an Ag-dependent manner while sparing the GVL effect. In the present study, we showed that compared with the control group, co-transplantation of donor-derived Bregs significantly increased the percentages of Tregs in skin, intestine, colon, liver, and lung (). Considering the evidence that Bregs could induce and/or maintain Tregs,Citation29,33 our results suggest that Bregs may ameliorate acute GVHD by inducing Tregs in vivo. In addition, given the plasticity between Th17 and Treg, another hypothesis, maybe more relevant, would be a polarization of T cells from Th17 to Treg.
The finding that donor-derived Bregs can successfully prevent acute GVHD led us to investigate the effects of this subset on GVL effects. Interestingly, we found a significantly lower percentage of GFP+ leukemia cells and longer survival of mice in the Breg group compared with those in the Tcon group (). These results suggest that co-transplantation of donor-derived Bregs modulates the function of alloreactive T cells and prevents GVHD without impairing the GVL effects of Tcon. Previously, Endinger et al.Citation9 confirmed that Treg could preserve GVL activity while inhibiting GVHD after BMT. The role of human MSCs in attenuating GVHD and maintaining GVL effects was also demonstrated following experimental allogeneic BMT.Citation8 Therefore, our results provide novel evidence and indicate that except for Tregs and MSCs, Bregs can prevent GVHD and maintain GVL activity.
More recently, Sarvaria et al.Citation34 demonstrated that Bregs are present in both naive and transitional B cell compartments of cord blood and can suppress T cell proliferation and effector function through IL-10 production as well as cell-to-cell contact involving CTLA-4. They also reported a protective role for cord blood-derived Bregs against GVHD development in cord blood recipients. The role of Bregs in acute GVHD was further elucidated by Chakupurakal et al.Citation35 In agreement with these studies, we found that a high dose of Bregs decreased the incidence of grades II–IV acute GVHD but had no negative effects on relapse after unmanipulated allografts ( and ). Overall, the results of the human studies further support the results obtained using a mouse model: that Bregs alleviate GVHD without compromising GVL activity.
The present study had several limitations. First, the more detailed Breg-related mechanisms in preventing GVHD and maintaining GVL effects remain to be elucidated. Second, the follow-up time of the cases in our clinical cohort study was short. A multicenter study with large sample and a long-term follow-up in allo-SCT settings, including ISD and unrelated donor, to investigate the effects of Breg on GVHD and relapse should be performed.
In summary, our findings demonstrate a role for Bregs in inhibiting Th1 and Th17 cells and modulating Tregs that contributed to preventing acute GVHD without compromising the GVL effects. Therefore, further exploitation of mechanisms underlying the separation of GVHD and GVL activity by Bregs may improve transplantation outcomes with allo-SCT and facilitate the potential use of Bregs in the clinic, which could enhance the safe use of allografts.
Materials and methods
Mice
Female C57BL/6 (B6; H-2b) and BALB/c (H-2d) mice were purchased from Beijing Vital Laboratory Animal Technology Company, Ltd. (Beijing, China). The mice were maintained in specific pathogen-free conditions and fed sterilized water and food at the animal facility of Peking University People's Hospital. Mice were between 8 and 10 weeks of age at the start of the experiments. All experiments were performed according to the National Institutes of Health's Guide for the Care and Use of Laboratory Animals.
GVHD and GVL model
BALB/c mice received 8 Gy total body irradiation from a [60Co] source for 8 min. On day 0, recipient mice were transplanted with 5 × 106 T cell-depleted bone marrow (TCD-BM) cells and 3 × 106 spleen T cells from B6 donors. T cell depletion was performed with anti-CD5 MicroBeads (Miltenyi Biotec) according to the manufacturer's protocols, as previously reported.Citation36 CD3+CD4+CD25− spleen T cells were purified using a FACSAria flow cytometer (BD Biosciences). Donor cells were injected through the tail vein. In GVL experiments, 5 × 105 GFP-expressing acute myeloid leukemia cells (H-2d),Citation37 kindly provided by Professor Yue-Ying Wang of Rui-Jin Hospital, Shanghai Jiao Tong University School of Medicine, were given along with the transplantation. The development of leukemia was monitored by counting the percentage of peripheral blood GFP+ cells through flow cytometry. Death caused by leukemia was defined by enlargement of the spleen and liver on autopsy, whereas death caused by GVHD was defined as the absence of tumor and the presence of GVHD.
Breg generation and enrichment
For adoptive transfer experiments, donor mice were treated with granulocyte colony-stimulating factor (G-CSF, 2 mg daily i.p.) for 10 d. These donor mice were killed at 14 d after G-CSF treatments. Splenic CD19+ B cells were isolated from mice by positive selection using magnetic beads (Miltenyi) with an obtained purity ≥ 95%. CD1dhiCD5+ Breg cells were purified (95–98%) using a FACSAria flow cytometer (BD Biosciences). After purification, CD1dhiCD5+ Breg cells (3 × 106) were immediately transferred into the appropriate recipient mice by tail vein injection.
Evaluation of GVHD and histopathological analysis
After BMT, animals were weighed every 2 d, and the degree of systemic GVHD was assessed by a clinical scoring system including five clinical parameters (weight loss, posture, activity, fur ruffling, and skin integrity), as published previously.Citation38 For histopathological analysis, left lung, liver, intestine, colon, and skin specimens of recipients were fixed with 4% paraformaldehyde, embedded in paraffin, sectioned, mounted on slides, and stained with hematoxylin and eosin. Two slides/organs were evaluated and scored by a pathologist blinded to experimental group using a scoring system described previously.Citation39 Immunohistochemical analysis of specimens were performed using GATA-3 (Cell Signaling Technology), T-bet, RORγt, and Foxp3 (Bioss) antibodies.
Flow cytometry assay
At designated time points, recipient mice were killed and organs collected. Single-cell suspensions were obtained from the blood, spleen, and bone marrow of mice. For detection of cytokine production, cells were stimulated for 6 h with 2μL/mL leukocyte activation cocktail (BD, GolgiPlug ™, Catalog No. 550583) before being stained for flow cytometric analysis. Cells were assayed for cytokine production by intracellular flow cytometry, and the following antibodies were used: CD3-BV510 anti-mouse, CD4+-APC-CY7 anti-mouse, CD8+-PerCP anti-mouse, CD25- PE-Cy7 anti-mouse, interferon-γ (IFNγ)-FITC anti-mouse, interleukin-4 (IL-4)-PE anti-mouse, IL-17-BV421 anti-mouse (BD Biosciences), Foxp3-Alexa Fluor 467 (eBioscience); and GATA3-PE-CF594 anti-mouse, RORγt-BV421 anti-mouse, and T-bet-PE anti-mouse (BD Biosciences). Intracellular cytokine staining was performed using a BD Cytofix/Cytoperm Fixation/Permeabilization Solution Kit (BD Biosciences), and the intracellular staining of Foxp3 was performed with a Foxp3/Transcription Factor Staining Buffer Set (eBioscience). Data were acquired with a LSRFortessa (BD Biosciences) and analyzed with FlowJo software (TreeStar).
Cytokine measurement
Mouse serum was collected on days 7 and 14 after BMT, and the levels of cytokines were measured using Multi-Analyte Flow Assay Kit, according to the manufacturer's instructions (BioLegend).
Patients and transplant protocol
Between January 2016 and March 2016, 74 patients who underwent an allo-SCT in our center entered this study (). All patients and their donors provided an informed consent for biologic research purposes. The study was conducted in accordance with the Declaration of Helsinki and was approved by the Institutional Review Board of Peking University. Diagnosis and grading of acute and chronic GVHD were performed as described by physicians in charge of the patients who were not aware of the results of laboratory tests. Unmanipulated haploidentical allografts and HLA-identical sibling donor transplantation were performed according to the protocols reported previously by our group.Citation40,41 In brief, the conditioning regimen for the haploidentical donor group (HID, n = 63) was as follows: cytarabine (4 g/m2 per day) IV on days −10 to −9; busulfan (3.2 mg/kg per day) IV on days −8 to −6; cyclophosphamide (1.8 g/m2 per day), IV on days −5 to −4; methyl chloride hexamethylene urea nitrate (Me-CCNU) (250 mg/m2 per day), orally once on day −3; and ATG (2.5 mg/kg per day; Sang Stat,Lyon, France) IV on days −5 to −2. Patients in the human leukocyte antigen identical sibling donor transplantation group (ISD, n = 11) received hydroxycarbamide (80 mg/kg) orally on day −10 and a lower dose of cytarabine (2 g/m2 per day) on day −9, but otherwise, an identical regimen to the HID patients without ATG was used. GVHD prophylaxis regimen consisted of mycophenolate mofetil, cyclosporine A, and short-term methotrexate.
Human Breg phenotype
Immune cell phenotyping of G-CSF-stimulated bone marrow grafts and peripheral blood stem cell grafts was performed in all 74 patients. Anti-human fluorochrome-conjugated antibodies for Breg analysis included: CD45-V500 (BD Biosciences), CD19-APC (BioLegend), CD19-APC-Cy7 (BioLegend), CD20-PerCP-Cy7 (BioLegend), CD24-PE-Cy7 (BD Biosciences), CD27-V450 (BD Biosciences), CD38-APC (BD), and CD38-FITC (BD). B cell subsets (including Bregs), T cell subgroups, and CD34+ cells were identified as described previously.Citation16,42-44 A representative dot plot for B cell subsets was showed in Fig. S2.
Statistics
Laboratory data analysis was performed using Prism software. Survival comparisons were performed using the log-rank test. Other differences between experimental groups were analyzed using two-tailed unpaired Student t tests.
For the patient analyses, comparisons were made with the χ2 statistic for categorical variables and the Mann–Whitney test for continuous variables. Cumulative incidence curves were used in a competing risk setting, with relapse treated as a competing event to calculate non-relapse mortality (NRM) probabilities, and with death from any cause as a competing risk for GVHD, engraftment, and relapse. Time to GVHD was defined as the time from transplantation to the onset of GVHD of any grade. Probabilities of survival were estimated with the Kaplan–Meier method. All variables in were included in the univariate analysis; then, only variables with p < 0.1 were included in a Cox proportional hazards model with time-dependent variables. Unless otherwise specified, p values were based on two-sided hypothesis tests. α was set at 0.05. Most analyses were performed with SPSS 16.0 (SPSS Inc., Chicago, IL, USA).
Disclosure of potential conflicts of interest
No potential conflicts of interest were disclosed.
Author contributions
Contribution: X.-J.H. designed the study; Y.-J.C. and Y. H. collected data; Y.H., Y.-J.C., and X.-J.H. analyzed the data and drafted the manuscript; and all authors contributed to data interpretation, manuscript preparation, and approval of the final version.
KONI_A_1284721_Supplementary_materials.zip
Download Zip (316.5 KB)Acknowledgments
We thank all of the faculty members who participated in these studies. We would also like to thank San Francisco Edit (www.sefedit.net) for assistance in editing this manuscript.
Funding
This work was supported (in part) by the National Natural Science Foundation of China (Grant Nos. 81470342; 81670168), the Key Program of the National Natural Science Foundation of China (Grant No. 81230013), the Beijing Natural Science Foundation (7162196), and the Foundation for Innovative Research Groups of the National Natural Science Foundation of China (Grant No. 81621001).
References
- Ferrara JL, Levine JE, Reddy P, Holler E. Graft-versus-host disease. Lancet 2009; 373:1550-61; PMID:19282026; http://dx.doi.org/10.1016/S0140-6736(09)60237-3
- Chang YJ, Xu LP, Wang Y, Zhang XH, Chen H, Chen YH, Wang FR, Han W, Sun YQ, Yan CH et al. Controlled, randomized, open-label trial of risk-stratified corticosteroid prevention of acute graft-versus-host disease after haploidentical transplantation. J Clin Oncol 2016; 34:1855-63; PMID:27091717; http://dx.doi.org/10.1200/JCO.2015.63.8817
- Negrin RS. Graft-versus-host disease versus graft-versus-leukemia. Hematology Am Soc Hematol Educ Program 2015; 2015:225-30; PMID:26637726; http://dx.doi.org/10.1182/asheducation-2015.1.225
- Zhao XY, Xu LL, Lu SY, Huang XJ. IL-17-producing T cells contribute to acute graft-versus-host disease in patients undergoing unmanipulated blood and marrow transplantation. Eur J Immunol 2011; 41:514-26; PMID:21268020; http://dx.doi.org/10.1002/eji.201040793
- Highfill SL, Rodriguez PC, Zhou Q, Goetz CA, Koehn BH, Veenstra R, Taylor PA, Panoskaltsis-Mortari A, Serody JS, Munn DH et al. Bone marrow myeloid-derived suppressor cells (MDSCs) inhibit graft-versus-host disease (GVHD) via an arginase-1-dependent mechanism that is up-regulated by interleukin-13. Blood 2010; 116:5738-47; PMID:20807889; http://dx.doi.org/10.1182/blood-2010-06-287839
- Christensen ME, Turner BE, Sinfield LJ, Kollar K, Cullup H, Waterhouse NJ, Hart DN, Atkinson K, Rice AM. Mesenchymal stromal cells transiently alter the inflammatory milieu post-transplant to delay graft-versus-host disease. Haematologica 2010; 95:2102-10; PMID:20801899; http://dx.doi.org/10.3324/haematol.2010.028910
- Sudres M, Norol F, Trenado A, Grégoire S, Charlotte F, Levacher B, Lataillade JJ, Bourin P, Holy X, Vernant JP et al. Bone marrow mesenchymal stem cells suppress lymphocyte proliferation in vitro but fail to prevent graft-versus-host disease in mice. J Immunol 2006; 176:7761-7; PMID:16751424; http://dx.doi.org/10.4049/jimmunol.176.12.7761
- Auletta JJ, Eid SK, Wuttisarnwattana P, Human mese Silva I, Metheny L, Keller MD, Guardia-Wolff R, Liu C, Wang F, Bowen T et al. Human mesenchymal stromal cells attenuate graft-versus-host disease and maintain graft-versus-leukemia activity following experimental allogeneic bone marrow transplantation. Stem Cells 2015; 33:601-14; PMID:25336340; http://dx.doi.org/10.1002/stem.1867
- Edinger M, Hoffmann P, Ermann J, Drago K, Fathman CG, Strober S, Negrin RS. CD4+CD25+ regulatory T cells preserve graft-versus-tumor activity while inhibiting graft-versus-host disease after bone marrow transplantation. Nat Med 2003; 9:1144-50; PMID:12925844; http://dx.doi.org/10.1038/nm915
- Ning H, Yang F, Jiang M, Hu L, Feng K, Zhang J, Yu Z, Li B, Xu C, Li Y et al. The correlation between cotransplantation of mesenchymal stem cells and higher recurrence rate in hematologic malignancy patients: outcome of a pilot clinical study. Leukemia 2008; 22:593-9; PMID:18185520; http://dx.doi.org/10.1038/sj.leu.2405090
- Gao L, Zhang Y, Hu B, Liu J, Kong P, Lou S, Su Y, Yang T, Li H, Liu Y et al. Phase II multicenter, randomized, double-blind controlled study of efficacy and safety of umbilical cord-derived mesenchymal stromal cells in the prophylaxis of chronic graft-versus-host disease after HLA-haploidentical stem-cell transplantation. J Clin Oncol 2016; 34:2843-50; PMID:27400949; http://dx.doi.org/10.1200/JCO.2015.65.3642
- Miyagaki T, Fujimoto M, Sato S. Regulatory B cells in human inflammatory and autoimmune diseases: from mouse models to clinical research. Int Immunol 2015; 27:495-504; PMID:25957264; http://dx.doi.org/10.1093/intimm/dxv026
- Mauri C, Blair PA. The incognito journey of a regulatory B cell. Immunity 2014; 41:878-80; PMID:25526301; http://dx.doi.org/10.1016/j.immuni.2014.12.003
- Matsumoto M, Baba A, Yokota T, Nishikawa H, Ohkawa Y, Kayama H, Kallies A, Nutt SL, Sakaguchi S, Takeda K et al. Interleukin-10-producing plasmablasts exert regulatory function in autoimmune inflammation. Immunity 2014; 41:1040-51; PMID:25484301; http://dx.doi.org/10.1016/j.immuni.2014.10.016
- Iwata Y, Matsushita T, Horikawa M, Dilillo DJ, Yanaba K, Venturi GM, Szabolcs PM, Bernstein SH, Magro CM, Williams AD et al. Characterization of a rare IL-10-competent B-cell subset in humans that parallels mouse regulatory B10 cells. Blood 2011; 117:530-41; PMID:20962324; http://dx.doi.org/10.1182/blood-2010-07-294249
- Blair PA, Norena LY, Flores-Borja F, Rawlings DJ, Isenberg DA, Ehrenstein MR, Mauri C. CD19(+)CD24(hi)CD38(hi) B cells exhibit regulatory capacity in healthy individuals but are functionally impaired in systemic Lupus Erythematosus patients. Immunity 2010; 32:129-40; PMID:20079667; http://dx.doi.org/10.1016/j.immuni.2009.11.009
- Weber M, Stein P, Prufer S, Rudolph B, Kreft A, Schmitt E, Bopp T, Roers A, Schild H, Fillatreau S et al. Donor and host B cell-derived IL-10 contributes to suppression of graft-versus-host disease. Eur J Immunol 2014; 44:1857-65; PMID:24643745; http://dx.doi.org/10.1002/eji.201344081
- Rowe V, Banovic T, MacDonald KP, Kuns R, Don AL, Morris ES, Burman AC, Bofinger HM, Clouston AD, Hill GR. Host B cells produce IL-10 following TBI and attenuate acute GVHD after allogeneic bone marrow transplantation. Blood 2006; 108:2485-92; PMID:16788097; http://dx.doi.org/10.1182/blood-2006-04-016063
- Le Huu D, Matsushita T, Jin G, Hamaguchi Y, Hasegawa M, Takehara K, Tedder TF, Fujimoto M. Donor-derived regulatory B cells are important for suppression of murine sclerodermatous chronic graft-versus-host disease. Blood 2013; 121:3274-83; PMID:23422748; http://dx.doi.org/10.1182/blood-2012-11-465658
- Khoder A, Sarvaria A, Alsuliman A, Chew C, Sekine T, Cooper N, Mielke S, de Lavallade H, Muftuoglu M, Fernandez Curbelo I et al. Regulatory B cells are enriched within the IgM memory and transitional subsets in healthy donors but are deficient in chronic GVHD. Blood 2014; 124:2034-45; PMID:25051962; http://dx.doi.org/10.1182/blood-2014-04-571125
- de Masson A, Bouaziz JD, Le Buanec H, Robin M, O'Meara A, Parquet N, Rybojad M, Hau E, Monfort JB, Branchtein M et al. CD24(hi)CD27(+) and plasmablast-like regulatory B cells in human chronic graft-versus-host disease. Blood 2015; 125:1830-9; PMID:25605369; http://dx.doi.org/10.1182/blood-2014-09-599159
- Shlomchik WD. Graft-versus-host disease. Nat Rev Immunol 2007; 7:340-52; PMID:17438575; http://dx.doi.org/10.1038/nri2000
- Lund FE, Randall TD. Effector and regulatory B cells: modulators of CD4+ T cell immunity. Nat Rev Immunol 2010; 10:236-47; PMID:20224569; http://dx.doi.org/10.1038/nri2729
- Yoshizaki A, Miyagaki T, DiLillo DJ, Matsushita T, Horikawa M, Kountikov EI, Spolski R, Poe JC, Leonard WJ, Tedder TF. Regulatory B cells control T-cell autoimmunity through IL-21-dependent cognate interactions. Nature 2012; 491:264-8; PMID:23064231; http://dx.doi.org/10.1038/nature11501
- Yu Y, Wang D, Liu C, Kaosaard K, Semple K, Anasetti C, Yu XZ. Prevention of GVHD while sparing GVL effect by targeting Th1 and Th17 transcription factor T-bet and RORgammat in mice. Blood 2011; 118:5011-20; PMID:21856864; http://dx.doi.org/10.1182/blood-2011-03-340315
- Yi T, Chen Y, Wang L, Du G, Huang D, Zhao D, Johnston H, Young J, Todorov I, Umetsu DT et al. Reciprocal differentiation and tissue-specific pathogenesis of Th1, Th2, and Th17 cells in graft-versus-host disease. Blood 2009; 114:3101-12; PMID:19602708; http://dx.doi.org/10.1182/blood-2009-05-219402
- Krenger W, Snyder KM, Byon JC, Falzarano G, Ferrara JL. Polarized type 2 alloreactive CD4+ and CD8+ donor T cells fail to induce experimental acute graft-versus-host disease. J Immunol 1995; 155:585-93; PMID:7608537; http://www.jimmunol.org/content/155/2/585
- Hashimoto D, Asakura S, Miyake S, Yamamura T, Van Kaer L, Liu C, Tanimoto M, Teshima T. Stimulation of host NKT cells by synthetic glycolipid regulates acute graft-versus-host disease by inducing Th2 polarization of donor T cells. J Immunol 2005; 174:551-6; PMID:15611282; http://dx.doi.org/10.4049/jimmunol.174.1.551
- Flores-Borja F, Bosma A, Ng D, Reddy V, Ehrenstein MR, Isenberg DA, Mauri C. CD19+CD24hiCD38hi B cells maintain regulatory T cells while limiting TH1 and TH17 differentiation. Sci Transl Med 2013; 5:173ra123; http://dx.doi.org/10.1126/scitranslmed.3005407
- Long J, Chang L, Shen Y, Gao WH, Wu YN, Dou HB, Huang MM, Wang Y, Fang WY, Shan JH et al. Valproic Acid Ameliorates Graft-versus-Host Disease by Downregulating Th1 and Th17 Cells. J Immunol 2015; 195:1849-57; PMID:26179902; http://dx.doi.org/10.4049/jimmunol.1500578
- Li J, Heinrichs J, Haarberg K, Semple K, Veerapathran A, Liu C, Anasetti C, Yu XZ. HY-Specific Induced Regulatory T Cells Display High Specificity and Efficacy in the Prevention of Acute Graft-versus-Host Disease. J Immunol 2015; 195:717-25; PMID:26048147; http://dx.doi.org/10.4049/jimmunol.1401250
- Heinrichs J, Li J, Nguyen H, Wu Y, Bastian D, Daethanasanmak A, Sofi MH, Schutt S, Liu C, Jin J et al. CD8(+) Tregs promote GVHD prevention and overcome the impaired GVL effect mediated by CD4(+) Tregs in mice. Oncoimmunology 2016; 5:e1146842; PMID:27471614; http://dx.doi.org/10.1080/2162402X.2016.1146842
- Nouel A, Pochard P, Simon Q, Ségalen I, Le Meur Y, Pers JO, Hillion S. B-Cells induce regulatory T cells through TGF-beta/IDO production in A CTLA-4 dependent manner. J Autoimmun 2015; 59:53-60; PMID:25753821; http://dx.doi.org/10.1016/j.jaut.2015.02.004
- Sarvaria A, Basar R, Mehta RS, Shaim H, Muftuoglu M, Khoder A, Sekine T, Gokdemir E, Kondo K, Marin D et al. IL-10+ regulatory B cells are enriched in cord blood and may protect against cGVHD after cord blood transplantation. Blood 2016; 128:1346-61; PMID:27439912; http://dx.doi.org/10.1182/blood-2016-01-695122
- Chakupurakal G, Garcia-Marquez MA, Shimabukuro-Vornhagen A, Kluth S, Schlosser H, Theurich S, Scheid C, Hallek M, Holtick U, von Bergwelt-Baildon M. Regulatory B10-cells display an altered homeostasis in acute Graft versus Host disease. Eur J Haematol 2016; 98:128-133; PMID:27717015; http://dx.doi.org/10.1111/ejh.12810
- Taki S, Sato T, Ogasawara K, Fukuda T, Sato M, Hida S, Suzuki G, Mitsuyama M, Shin EH, Kojima S et al. Multistage regulation of Th1-type immune responses by the transcription factor IRF-1. Immunity 1997; 6:673-9; PMID:9208840; http://dx.doi.org/10.1016/S1074-7613(00)80443-4
- Wang YY, Zhao LJ, Wu CF, Liu P, Shi L, Liang Y, Xiong SM, Mi JQ, Chen Z, Ren R et al. C-KIT mutation cooperates with full-length AML1-ETO to induce acute myeloid leukemia in mice. Proc Natl Acad Sci U S A 2011; 108:2450-5; PMID:21262832; http://dx.doi.org/10.1073/pnas.1019625108
- Cooke KR, Kobzik L, Martin TR, Brewer J, Delmonte J Jr, Crawford JM, Ferrara JL. An experimental model of idiopathic pneumonia syndrome after bone marrow transplantation: I. The roles of minor H antigens and endotoxin. Blood 1996; 88:3230-9; PMID:8963063; http://www.bloodjournal.org/content/88/8/3230.full.html
- Hill GR, Crawford JM, Cooke KR, Brinson YS, Pan L, Ferrara JL. Total body irradiation and acute graft-versus-host disease: the role of gastrointestinal damage and inflammatory cytokines. Blood 1997; 90:3204-13; PMID:9376604; http://www.bloodjournal.org/content/90/8/3204.full.html
- Wang Y, Liu QF, Xu LP, Liu KY, Zhang XH, Ma X, Fan ZP, Wu DP, Huang XJ. Haploidentical vs identical-sibling transplant for AML in remission: a multicenter, prospective study. Blood 2015; 125:3956-62; PMID:25940714; http://dx.doi.org/10.1182/blood-2015-02-627786
- Wang Y, Liu QF, Xu LP, Liu KY, Zhang XH, Ma X, Wu MQ, Wu DP, Huang XJ. Haploidentical versus matched-sibling transplant in adults with Philadelphia-negative high-risk acute lymphoblastic leukemia: A biologically phase III randomized study. Clin Cancer Res 2016;22:3467-76; PMID:26927664; http://dx.doi.org/10.1158/1078-0432.CCR-15-2335
- Maecker HT, McCoy JP, Nussenblatt R. Standardizing immunophenotyping for the Human Immunology Project. Nat Rev Immunol 2012; 12:191-200; PMID:22343568; http://dx.doi.org/10.1038/nri3158
- Chang YJ, Zhao XY, Huo MR, Xu LP, Liu DH, Liu KY, Huang XJ. Immune reconstitution following unmanipulated HLA-mismatched/haploidentical transplantation compared with HLA-identical sibling transplantation. J Clin Immunol 2012; 32:268-80; PMID:22173879; http://dx.doi.org/10.1007/s10875-011-9630-7
- Chang YJ, Zhao XS, Lv M, Zhao XY, Han TT, Wang HT, Huang XJ. CD19+CD24highCD38high B cells exhibit higher ability in TGF-{beta} and IL-10 secretion and suppressing of CD4+ T cell proliferation but are quantitatively decreased in G-BM of healthy donors (P2146). J Immunol 2013; 190:69.11