ABSTRACT
Breast cancer is the most common cancer in women and the second leading cause of female cancer-related deaths worldwide. Inflammation is an established hallmark of tumorigenesis and an important determinant of tumor outcome and response to therapy. With advances in cancer immunotherapy, there is an urgent need to dissect the contribution of specific immune effectors in cancer development. Here, we genetically investigated the role of the Interleukin-1 (IL-1) receptor 1 (IL-1R1) pathway in breast cancer tumorigenesis and metastasis using the MMTV-PyMT mouse model. Our results indicate that IL-1R1 signaling suppresses mammary tumor cell proliferation early in tumorigenesis and curbs breast cancer outgrowth and pulmonary metastasis. We show that PyMT/Il1r1−/− mice had a higher primary tumor burden and increased mortality rate compared with IL-1R1-sufficient PyMT control mice. This phenotype was independent of the inflammatory caspases-1/-11 but driven by IL-1α, as PyMT/Il1a−/− mice phenocopied PyMT/Il1r1−/− mice. Collectively, our results suggest that IL-1α-mediated IL-1R1 signaling is tumor-suppressive in PyMT-driven breast cancer.
Introduction
Breast cancer is the most common cancer in women worldwide with almost 1.7 million new diagnoses and over 520,000 deaths in 2012.Citation1 Breast cancer is a highly heterogeneous disease. It can be sub-typed according to genetic profiling or by immunohistochemical (IHC) staining techniques. There are four widely accepted molecular subtypes of breast cancer, with diverse epidemiological risk factors and different responses to therapies: luminal A, luminal B, human epidermal growth factor receptor (HER)-enriched and basal-like.Citation2,3 Differential expression of estrogen receptor (ER), progesterone receptor (PR) and HER2 correlates with these different subtypes.Citation2
Inflammation is an established hallmark of cancer. It acts as a double edged sword in tumorigenesis, as chronic inflammation elicited by infection, obesity or other lifestyle factors has been linked to tumor promotion and progression, whereas low grade inflammation in the tumor microenvironment promotes the maturation of antigen-presenting cells (APCs) and the induction of antitumor immunity.Citation4,5 Indeed, unleashing antitumor immunity through immune checkpoint inhibitors, including monoclonal antibodies against cytotoxic T lymphocyte-associated antigen 4 (CTLA4), programmed cell death protein 1 (PD-1) or PD1 ligand (PD-L1), has recently shown significant clinical success in multiple cancers.Citation6
The two cytokines Interleukin (IL)-1α and IL-1β are important initiators and propagators of the inflammatory response. They are highly expressed in multiple cancers and are produced by tumor cells themselves, infiltrating myeloid cells and tumor-associated fibroblasts.Citation7-10 Both cytokines are synthesized as precursors that are subsequently cleaved into their mature form.Citation11 Although they mediate their activities by binding to the same cell-surface signaling receptor (IL-1R1),Citation12-14 their individual requirement and impact on disease states are context-dependent. Indeed, the two cytokines have different components that control their availability and maturation. Whereas IL-1α is constitutively expressed and active in its precursor and mature forms,Citation15 the expression of IL-1β is induced by inflammatory signals that converge on NF-κB, and its biological activity relies on proteolytic processing primarily by the inflammatory caspase-1 and -11 within the inflammasome.Citation16 IL-1α is cleaved intracellularly by the calcium-dependent protease calpain.Citation17 Other proteases, including granzyme B, elastase, chymase and neutrophil elastase, are also able to cleave it extracellularly. While IL-1β functions as a secreted cytokine, IL-1α acts intracellularly and extracellularly. It can function as a membrane-bound cytokineCitation18 or in the nucleusCitation19 as a transcriptional regulator.Citation11 During necrotic cell death, IL-1α is released to the extracellular space where it acts as an alarminCitation11 that propagates the inflammatory response.
To date, the role of IL-1R1 signaling in cancer has been controversial and highly debated, partly due to differences in study design and the differential functions of the two IL-1 cytokines. Most of the information we have on the role of IL-1 signaling in cancer is derived from studies that have probed the function of single IL-1 cytokines, mainly in their recombinant or extracellular forms and not in their cell-associated context, and of established malignant cells, either from cancer patients or from transplantable mouse models where the transplanted carcinoma is fully equipped with IL-1 signaling components, while the host is either sufficient or not in these factors. Furthermore, several studies have reported opposing roles of IL-1β and IL-1α in cancer, in general as pro- and anti-tumorigenic, respectively.Citation20,21 As an example, it was shown that Il1b−/− mice had reduced tumor growth and increased survival rate compared with wild-type (WT) controls after intravenous (i.v.) injection of B16 melanoma cells.Citation22 In a model of chemically induced skin carcinogenesis, 3-methylcolanthrene (3-MCA), Il1b−/−, but not Il1a−/− mice, had delayed tumor onset compared with WT control mice.Citation23 In yet another model of skin carcinogenesis, 7,12-dimethylbenzanthracene (DMBA)/12-O-tetradecanoylphorbol-13-acetate (TPA), Il1ra−/− mice, which lack the IL-1R1 antagonist, were shown to have increased cancer burden.Citation24 These and other studiesCitation25,26 have led to the proposal that IL-1R1 blockade might constitute a potential cancer therapeutic.Citation9,10,27 However, although IL-1β signaling has been reported to drive carcinogenesis, many studies have shown an antitumorigenic role for IL-1α.Citation7,21,28-31 For instance, NIH3T3 fibrosarcoma cells transfected with IL-1α failed to grow in vivo following i.v. injection, whereas IL-1β transfected cells were more aggressive than untransfected control cells.Citation21 Similarly, transgenic mice overexpressing IL-1α in keratinocytes had decreased DMBA/TPA-induced skin carcinogenesis compared with control mice, suggesting a tumor-suppressive role for IL-1α. Consistently, stimulation of multiple cancer cell-lines, including MCF-7, A375 and prostate stem cells, with IL-1α inhibited their proliferation by causing G0–G1 arrest.Citation32-35 IL-1α was also shown to have anti-proliferative effects in murine primary mammary cells.Citation36
Given the context specific functions of IL-1 cytokines in cancer, we wished to interrogate whether IL-1R1 signaling is beneficial or harmful to the host during spontaneous carcinogenesis. Specifically, we investigated the role of IL-1R1 in a spontaneous model of breast cancer, induced by the expression of polyoma middle T antigen (PyMT) under the control of the mouse mammary tumor virus (MMTV) promoter (MMTV-PyMT; herein referred to as PyMT).Citation37 This transgene causes cellular transformation of mammary epithelial cells by acting as a signaling scaffold that chronically activates several cancer drivers including AKT, MAPK and RAS pathways,Citation38 leading to luminal-type breast cancer, which eventually metastasizes to the lungs.Citation37,39 By generating PyMT/Il1r1−/− mice (or PyMT/Il1a−/−), both host and tumor cells are deficient in IL-1 pathway components, and the role of IL-1 signaling can be studied before the development of malignancy.
Results
IL-1R1 deficiency increases susceptibility to PyMT-driven breast cancer and lung metastasis
IL-1R1 is ubiquitously expressed;Citation40 however, whether its expression regulates mammary pad development has not been thoroughly explored. We show that genetic ablation of IL-1R1 did not impact mammary pad morphogenesis, as revealed by equivalent ductal length and average fat cell size in Il1r1−/− mice, compared with WT controls (Fig. S1A–D). This was supported by immunofluorescence analysis of cytokeratin (CK)8 and CK14 staining that mark luminal and basal cells, respectively (Fig. S1E).Citation41 This analysis showed that the ductal structure was normal in both genotypes, with two distinct layers, an inner layer of luminal cells surrounded by an outer layer of basal cells. Importantly, the function of the mammary pad was unaffected by loss of Il1r1, as the number of pups per litter was similar for Il1r1−/− and WT mice (Fig. S1F).
To study the role of IL-1R1 in breast cancer tumorigenesis and metastasis, we took advantage of the well-characterized MMTV-PyMT model of breast cancer and generated PyMT/Il1r1−/− mice.Citation37 Palpation twice a week for the appearance of the first mammary tumor indicated an earlier tumor onset in PyMT/Il1r1−/− mice compared with PyMT mice (), which correlated with faster mortality rate of PyMT/Il1r1−/− mice (). By clinical end point, which we defined as the time point by which the total primary tumor burden reached a volume of 6 cm3 or when the volume of one tumor mass grew beyond 2 cm3, at least 9 out of 10 of the mammary pads had developed tumors in PyMT/Il1r1−/− mice, whereas only 8 on average had tumors in PyMT control mice (). When quantifying tumors at a fixed time point of approximately 150 d of age, PyMT/Il1r1−/− mice had an increased tumor burden compared with PyMT controls (). At this stage, only 20% of PyMT mice had lung metastatic lesions, whereas all of the PyMT/Il1r1−/− mice had metastases (). In metastasis-bearing mice, the number of lung metastatic lesions was however similar at this time point between the two genotypes (). Given that the primary tumor burden was higher in PyMT/Il1r1−/− mice, it was not surprising to observe enhanced metastasis incidence in these mice compared with PyMT controls. To determine whether IL-1R1 signaling regulated the metastatic process per se, i.e., independently of its role in primary tumorigenesis, we analyzed the lung metastatic burden at the respective clinical end point of each genotype. Although almost all mice bore metastases at this stage ( and ), PyMT/Il1r1−/− mice had an increase in the number of lung lesions compared with that of PyMT controls (). These results suggest that IL-1R1 plays a key role both in breast cancer development and subsequent metastasis.
Figure 1. PyMT/Il1r1−/− mice have an earlier tumor onset and increased metastasis compared with PyMT mice. (A) Kaplan–Meier tumor-free survival curves of PyMT (median = 99 d) or PyMT/Il1r1−/− mice (median = 72.5) (n = 10–20 per genotype, Mantel–Cox p < 0.0001). (B) Kaplan–Meier survival curves of PyMT (median = 170 d) or PyMT/Il1r1−/− mice (median = 140.5) (n = 20–30 per genotype, Mantel–Cox p < 0.0001). (C) Number of mammary gland with tumors at clinical end point (n = 15–20, Student's t-test p < 0.001). (D–F) Primary tumor burden (D), percentage of mice bearing metastasis (E) and average number of lung lesions per metastasis-bearing animals (F) at 5 mo of age of PyMT or PyMT/Il1r1−/− mice (n = 7–19 mice per genotype). Each symbol represents a mouse; the line represents the mean. (G-I) Primary tumor burden (G), percentage of mice bearing metastasis (H) and average number of lung lesions per metastasis-bearing animals (I) at clinical end point of PyMT or PyMT/Il1r1−/− mice (n = 16–17 mice per genotype, Student's t-test p < 0.01). Each symbol represents a mouse; the line represents the mean.
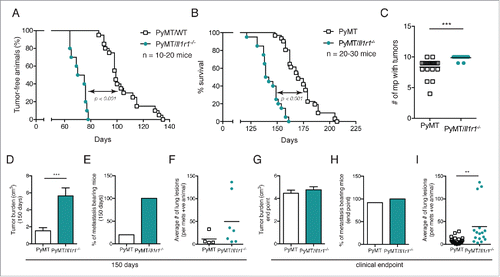
Figure 2. Increased foci coverage in PyMT/Il1r1−/− mice compared with PyMT mice at early time points. (A) Whole mounts of mammary fat pads of PyMT or PyMT/Il1r1−/− mice at 6 and 10 weeks of age were stained with hematoxylin and analyzed for foci coverage (n = 4–5 mice per time point per genotype, Student's t-test p < 0.001). (B) Representative pictures of whole mount of PyMT or PyMT/Il1r1−/− mice at 6 and 10 weeks of age. Boxed region represents insets. (C) H&E of mammary fat pad section of PyMT or PyMT/Il1r1−/− mice at 10 weeks of age. (D) Western blots depicting E-cadherin, Cyclin D1 and GAPDH expression in lysates from mammary fat pads of WT, Il1r1−/−, PyMT or PyMT/Il1r1−/− mice at 6 weeks of age. (E) Densitometry analysis of the ratios of E-cadherin/GAPDH and Cyclin D1/GAPDH, using the ImageJ software. (F) Mice were palpated twice a week to determine the first appearance of tumors. Tumor volume was measured weekly for the following 6 weeks (n = 8–12 mice per genotype, Student's t-test per time point).
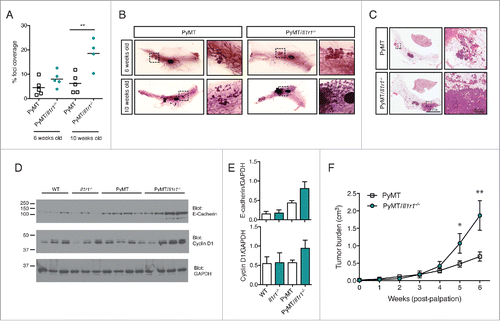
No significant differences in proliferation, cell death or migration in advanced tumors in PyMT or PyMT/Il1r1−/− mice
Given the enhanced tumorigenesis in PyMT/Il1r1−/− mice, we decided to investigate whether tumor cells lacking IL-1R1 were more invasive. To do so, 1 cm3 tumors from PyMT or PyMT/Il1r1−/− mice were dissected and dissociated into single cell suspension. Tumor cells were then analyzed for migration, proliferation and survival, all key hallmarks of cancer.Citation4 Phalloidin, which stains F-actin, reveals actin stress fibers and focal adhesions, which are altered particularly in aggressive tumors.Citation42 Staining of PyMT or PyMT/Il1r1−/− cells with phalloidin showed similar actin networks between the two genotypes (Fig. S2A). Metastatic cells gain migratory capacity to reach their secondary location.Citation43 To study tumor cell migration ex vivo, we performed a wound-healing assay. Cells derived from tumors of both genotypes were allowed to grow to confluency. They were then scratched with a pipette tip and their migration was recorded by time-lapse microscopy. The gap closed at a similar rate, and within 12 h was fully healed, suggesting that tumor cells from both genotypes had a similar migration rate (Fig. S2B and C).
PyMT-derived cells were then treated with increasing concentrations of either recombinant murine IL-1α or IL-1β. As expected, both cytokines induced phosphorylation of the MAPK p38 in a dose-dependent manner (Fig. S2D). However, treatment with either cytokine did not cause an increase in their proliferation rate. Indeed, there were no differences in the expression of cyclin D1 24 h after cytokine stimulation, as revealed by immunoblot (Fig. S2E). Additionally, the proliferation rate was assessed by CSFE dilution. Cells were stimulated with IL-1α or IL-1β and allowed to proliferate for 24, 48 or 72 h. There were no observed differences in CSFE dilution in cells of the two genotypes (Fig. S2F). This suggests that IL-1 signaling within fully transformed tumor cells does not significantly impact their proliferation. Lastly, we assessed the survival of cells following cytokine stimulation by Live/Dead viability dye (Invitrogen). No genotype-dependent differences were observed (Fig. S2G). Collectively, we did not observe any differences in ex vivo measurements of migration, proliferation or survival of fully transformed tumor cells. This was confirmed by assessing proliferation and cell death directly in tumor lysates. Tumors that were approximately 1 cm3 in volume were dissected and homogenized in lysis buffer. Tumor homogenates were then analyzed by immunoblot for expression of cyclin D1, a marker of proliferation, and cleavage of caspase-3, a marker of apoptosis. These readouts were also similar in the two genotypes (Fig. S2H). In these samples, we detected comparable levels of the epithelial cell marker E-cadherin, suggesting a similar number of tumor cells across the two genotypes (Fig. S2H). Additionally, we did not find any differences in the levels of AKT activation as evident by equal AKT phosphorylation levels in the two genotypes (Fig. S2I).
Increased proliferation rate of mammary epithelial cells from PyMT/Il1r1−/− mice at the onset of tumorigenesis
Since we did not observe any IL-1R1-dependent differences in migration, proliferation or cell death of fully transformed tumor cells, we decided to investigate the role of this pathway in the onset of tumorigenesis, i.e., at early time points before any palpable tumors. Mice were sacrificed at either 6 or 10 weeks of age. Mammary pads were excised, mounted on a microscope slide and stained with hematoxylin to quantify the number of hyperplastic lesions within the whole mounts. At 6 weeks of age, there was a trend toward increased foci coverage in the mammary pad whole mounts of PyMT/Il1r1−/− mice compared with PyMT mice. This difference was significant by 10 weeks of age ( and ). The increased primary tumor burden is also evident histologically using tissue sections stained with H&E (). Of note, the PyMT mRNA was similar in the two genotypes, indicating that the difference observed in primary tumor onset is not due to differential transgene expression (Fig. S3C). Although there were no significant differences in foci coverage at 6 weeks of age, we did observe an increase in the expression levels of E-cadherin and cyclin D1 by immunoblot, suggesting enhanced proliferation of epithelial cells ( and ). In contrast, cleaved caspase-3 levels were similar in the two genotypes (Fig. S3A). Phosphorylation of AKT was highly variable among mice irrespective of genotype (Fig. S3A and B). Next, to follow tumor growth, we palpated for the appearance of the first mammary tumor in PyMT and PyMT/Il1r1−/− mice and quantified the total tumor burden over the next 6 weeks. At 5 weeks post-palpation, the tumor burden in PyMT/Il1r1−/− mice significantly surpassed that of PyMT mice, suggesting that IL-1R1 deficiency conferred a higher rate of tumor cell proliferation ().
To assess mammary epithelial cell proliferation before the development of palpable tumors, we injected 10-week old PyMT or PyMT/Il1r1−/− mice with BrdU 2 hours before sacrifice. BrdU gets incorporated in dividing cells. Mammary fat pad sections were then stained with an anti-EpCAM antibodies to mark epithelial cells and anti-BrdU antibodies to specifically label proliferating cells. Mammary ducts were divided into four grades according to disease severity, grade 0, representing a normal duct, and grade 3, representing highly dense and de-differentiated cells (). PyMT/Il1r1−/− mice contained a higher percentage of BrdU+ mammary epithelial cells than in PyMT mice, and this increase in proliferation was observed in grades 0–2 ( and ). In contrast, no differences were observed in grade 3 when cells were fully de-differentiated. TUNEL staining revealed that, in contrast to proliferation, mammary epithelial cell death was independent of IL-1R1 ( and ). Similarly, we did not observe any IL-1R1-mediated differences in the mRNA expression of genes involved in epithelial to mesenchymal transition, including snail, slug and twist. (Fig. S3D).
Figure 3. Increase in proliferation rate, but not cell death, in PyMT/Il1r1−/− mice at early time points. (A) PyMT or PyMT/Il1r1−/− mice at 10 weeks of age were injected i.p. with BrdU 2 h before sacrifice. Epifluorescence images of mammary fat pad were stained with antibodies against BrdU and EpCAM. Mammary ducts were classified into four different grades (grade 0 = healthy, grade 1 = hyperplastic ducts, grade 2 = early adenoma, and grade 3 = late adenoma/carcinoma). (B) Quantification of BrdU and EpCAM double positive nuclei, determined using Volocity, and represented as a percentage of total EpCAM positive nuclei. Each symbol represents one duct (n = 3 mice per genotype, Student's t-test). (C) Representative images of PyMT or PyMT/Il1r1−/− mice of a grade 2 mammary duct. (D) Epifluorescence images of mammary fat pads from 10-week-old mice were stained with TUNEL as a measure of cell death. (E) Quantification of TUNEL positive nuclei, determined using Volocity (n = 2–3 mice per genotype, Student's t-test).
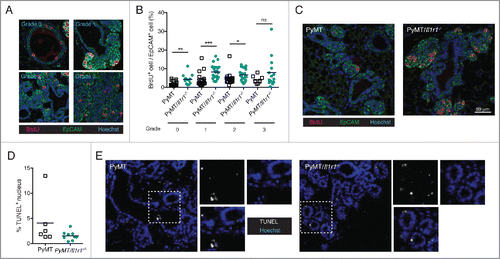
Figure 4. Il-1r1 ablation does not affect the proportions of immune infiltrates in the mammary fat pad during early or late tumorigenesis. (A) Flow cytometric analysis of immune infiltrates as a percent of total CD45+ cells in mammary fat pad of PyMT or PyMT/Il1r1−/− mice at 6 and 10 weeks of age (n = 7–8 mice per genotype, Student's t-test). (B) Flow cytometric analysis of intra-tumoral immune infiltrate populations as a percent of total CD45+ cells in mammary carcinomas of PyMT or PyMT/Il1r1−/− mice when tumors reached a volume of 1 cm3 (n = 5 mice per genotype, Student's t-test).
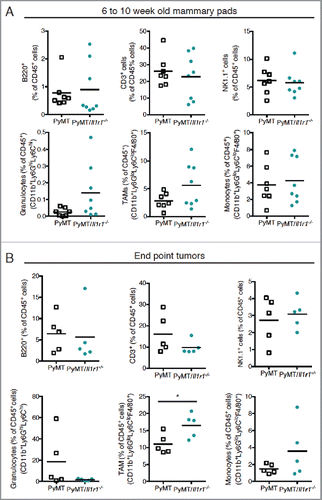
IL-1R1 deficiency does not drastically impact the tumor microenvironment in PyMT mice
Since the immune microenvironment plays a key role in MMTV-PyMT tumorigenesis,Citation44-46 we next evaluated the impact of IL-1R1 gene ablation on the frequencies of immune infiltrates within the mammary fat pad of PyMT mice between 6 and 10 weeks of age. Despite differential tumorigenesis between the two genotypes, the frequencies of B cells (B220+ cells), T cells (CD3+ cells), NK cells (NK1.1+ cells), granulocytes (CD11b+Ly6GhiLy6Chi), monocytes (CD11b+Ly6GloLy6ChiF4/80+) and tumor-associated macrophages (TAMs) (CD11b+Ly6GloLy6CloF4/80+) were comparable (). We observed a trend toward increased TAM frequency in PyMT/Il1r1−/− mice compared with PyMT controls, but this did not reach statistical significance. Similar intratumoral immune cell frequencies were also observed at the endpoints of the two genotypes ().Citation47 However, at this stage, tumors of PyMT/Il1r1−/− mice contained a significantly higher TAM frequency compared with tumors of PyMT mice ().
To further explore the effect of IL-1R1 on modulating inflammation and immunity at the onset of PyMT-driven tumorigenesis, we next profiled the mRNA expression of pro- and anti-inflammatory cytokines (Il1a, Il1b, Il18, Tnf and Il10), as well as that of immune effector genes (Ifng, Prf1 and Grzb) in the mammary pad of 6 and 10 week old PyMT and PyMT/Il1r1−/− mice. We did not observe genotype-specific differences in gene expression of these analytes, with the exception of Grzb, which was significantly more expressed in 10-week-old PyMT/Il1r1−/− mice compared with PyMT control mice (Fig. S3E). A similar trend was observed for the Il1a transcript but this did not reach statistical significance (Fig. S3E).
PyMT mice deficient in IL-1α phenocopy PyMT/Ilr1−/− mice
To assess which of the IL-1 cytokines is responsible for tumor suppression in this model, we first quantified intra-tumoral levels of IL-1α and IL-1β by performing an ELISA on lysates of 1 cm3 tumors from PyMT mice. Whereas IL-1α was abundant in almost all tumors tested, IL-1β levels were below the detection limit of the assay (). Next, we generated PyMT/Ice−/− mice (deficient in both Casp1/Casp11). Surprisingly, these mice were phenotypically equivalent to PyMT control mice. Indeed, they had similar survival rate, primary tumor burden and pulmonary metastasis coverage (Fig. S4). Although these results do not fully rule out a possible function of IL-1β in this model, they rather support a potential role of IL-1α in modulating PyMT-driven tumorigenesis. To test this hypothesis genetically, we generated PyMT/Il1a−/− mice. As early as 10 weeks of age, PyMT/Il1a−/− mice exhibited a significant increase in breast cancer foci coverage compared with PyMT controls, as shown by whole mount analysis (). This phenotype was similar to that of PyMT/Il1r1−/− mice, suggesting that IL-1α is, at least in part, responsible for tumor suppression upstream of IL-1R1 signaling.
Figure 5. IL-1α expression leads to decreased foci coverage in mice and correlates with better patient survival. (A) ELISA for IL-1α and IL-1β were performed on lysates from ∼1 cm3 mammary tumors from PyMT mice. Each symbol on the graph represents one mouse; the horizontal line represents the mean. (B) Whole mounts of mammary fat pads from PyMT, PyMT/Il1r1−/− or PyMT/Il1a−/− mice at 10 weeks of age were stained with hematoxylin and analyzed for foci coverage (n = 4–5 mice per time point per genotype, Student's t-test p < 0.001). (C) Representative pictures of whole mounts of mammary fat pads from PyMT, PyMT/Il1r1−/− or PyMT/Il1a−/− mice at 10 weeks of age. Boxed region represents insets. (D) H&E of mammary fat pad section from PyMT, PyMT/Il1r1−/− or PyMT/Il1a−/− mice at 10 weeks. Boxed region represents insets. (E, F) Kaplan–Meier survival curves showing probability of relapse-free survival (FRS) in patients with all intrinsic subtypes (E) or luminal B (F) breast cancer with low or high expression of IL-1α expression. The optimal expression threshold was auto-selected by K–M plotter software. Hazard ratio (with confidence intervals) and log-rank probability values are included on each graph.
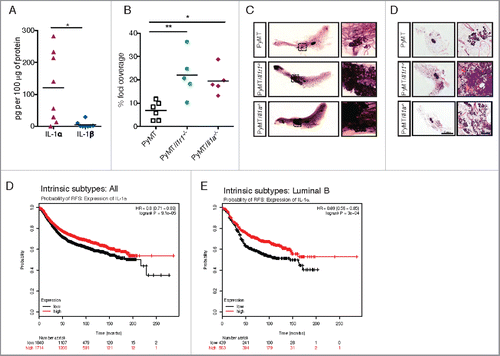
High expression of IL-1α correlates with better prognosis in human breast cancer
To assess whether expression levels of IL-1α correlated with better or worse prognosis in cancer patients, we performed the KM Plotter analysis.Citation48 Consistent with our data from PyMT mouse experiments, we found that high expression of IL-1α correlated with a better prognosis in breast cancer patients (). Given that the MMTV-PyMT model of breast cancer is considered a luminal type breast cancer, we next analyzed the link between IL-1α expression and the prognosis of this subtype specifically, and found that high IL-1α expression correlated with better prognosis in luminal B breast cancer ().
Discussion
Here, we show that signaling through IL-1R1 is critical in controlling tumorigenesis in MMTV-PyMT-driven breast cancer. This model is of particular interest as both host and cancer cells are deficient in IL-1R1, which is in contrast with previous studies that used transplantable tumor models and IL-1R1-sufficient established malignant cells.Citation7 By using a spontaneous model of breast cancer, we were able to study the role of IL-1R1 signaling at the onset of tumorigenesis. We showed that IL-1R1 deficiency resulted in an increase in mammary epithelial cell proliferation before any palpable tumors. The difference in proliferation was lost in highly dense hyperplastic areas (grade 3), suggesting that the effect of IL-1R1 was more important in the early phase of the disease. Additionally, once the tumors were fully transformed, the impact of IL-1R1 on proliferation regulation became less important.
IL-1R1 responds to both IL-1α and IL-1β. Although, these two cytokines transduce signaling similarly, opposing roles have been reported in multiple diseases.Citation20,21,49 In cancer, IL-1β has been more clearly established as a tumor promoting cytokine.Citation8,22 On the other hand, the role of IL-1α is debated.Citation7,21,28-31 In this study, we show that PyMT/Il1a−/− mice phenocopied PyMT/Il1r1−/− mice, suggesting that IL-1α is the primary cytokine responsible for tumor suppression upstream of IL-1R1 in this system. IL-1α can signal through the IL-1R1 in its unprocessed or mature form, as a secreted cytokine or a membrane-bound protein. In this model, we have yet to elucidate which of these IL-1α forms mediates IL-1R1 activity.
The activation of caspase-1 and -11 has been previously reported to mediate IL-1α secretion, which was more recently shown to occur following pyroptosis, primarily of myeloid cells.Citation50 Our results revealed that, unlike PyMT/Il1r1−/− and PyMT/Il1a−/− mice, which were more susceptible to PyMT-driven tumorigenesis, PyMT/Ice−/− mice were phenotypically similar to PyMT controls. Together, these findings suggest that IL-1α activity is independent of caspase-1/-11. The release of IL-1α is not limited to pyroptosis, as it can be released passively from stressed cells or following cellular damage and necrosis.Citation11,50
Our data using PyMT/Ice−/− do not rule out a possible role of caspase-1/-11-independent IL-1β in this model. This would require future exploration using PyMT/Il1b−/− mice. Notably, IL-1β expression has been previously associated with increased lung metastasis in a different mouse model of breast cancer. Mice conditionally deficient in E-cadherin and p53 under the CK14 promoter (KEP; K14cre/Cdh1F/F/Trp53F/F) develop aggressive lobular mammary carcinoma.Citation51 The tumors can be harvested and orthotopically injected into syngenic WT mice where they proliferate and eventually colonize the lungs. In this model, IL-1β-activated γδ T cells secrete IL-17 and promote granulocyte colony-stimulating factor (G-CSF)-dependent neutrophil expansion, which suppresses CD8+ T-cell-mediated immunosurveillance.Citation52 Importantly, this pathway impacts lungs metastasis without affecting the primary tumor burden. These findings further emphasize the context-specific properties of IL-1 cytokines.
It is hypothesized that IL-1R1 signaling acts as a double-edged sword. It can promote tumor growth, angiogenesis and metastasis in some contexts, while stimulating antitumor immunity or directly suppressing tumorigenesis in others.Citation20 This complexity has resulted in contrasting findings regarding correlations between IL-1R1 signaling and human cancer prognosis. For instance, the IL-1 antagonist, IL-1Ra has been shown to be overexpressed in multiple cancers, including multiple myeloma, leukemia, cervical, ovarian, colorectal, pancreatic and breast cancer,Citation53-58 but to be downregulated in others.Citation59 Similarly, high expression of the IL-1ra correlates with either betterCitation54,56,60-63 or worse cancer prognosisCitation62,64,65 This variability could stem from differential requirements of the two IL-1 cytokines in different cancers. Using KM plotter we have found that breast cancer patients expressing high levels of IL-1α had a better prognosis compared with patients expressing low levels of this cytokine,Citation48 consistent with a role of IL-1α as a negative regulator of tumorigenesis.
MMTV-PyMT tumors are infiltrated with both innate and adaptive immune cells, which control disease severity. PyMT/Rag1−/− mice, which lack both T and B cells, have a decreased metastatic burden, due to CD4+ T cells enhancing the pro-tumorigenic properties of TAMs.Citation44 Similarly, PyMT mice expressing a recessive null mutation in colony stimulating factor 1 (Csf1) have a blunted metastasis phenotype.Citation45 This is due to the active role of macrophages, specifically Gr1+ inflammatory monocytes,Citation46 in enabling tumor extravasation at secondary metastatic sites and in promoting metastasis survival and growth.Citation66 Our results indicating increased TAM frequency in PyMT/Il1r1−/− mice compared with PyMT controls suggest a potential role of TAMs in mediating the enhanced metastatic burden observed in PyMT/Il1r1−/− mice.
IL-1 signaling can play an important role in immune or stromal cells as well as within the cancer cells themselves.Citation27 In the current study, we did not pinpoint the location of either the cytokine or the receptor within the host. Future studies would be required to understand in which cell type IL-1α and IL-1R1 are important and whether IL-1α acts in an autocrine or paracrine fashion. To do so, conditional tissue-specific knockout mice for both Il1r1−/− and Il1a−/− would be needed.
Materials and methods
Animal studies
All experiments were performed under guidelines of the animal ethics committee of McGill University (Canada). Il1r1−/− mice were purchased from Jackson Laboratory (Stock # 003245). PyMT, PyMT/Il1r1−/− and PyMT/Il1a−/− were bred as separate colonies on a C57Bl/6J background. All mice used for tumor analysis were virgin females containing a single copy of the MMTV-PyMT transgene. For tumor detection, mice were palpated twice a week starting 8 weeks of age. Tumor volume was measured according to the following formula: tumor volume (mm3) = (4/3∗(3.14159)∗(Length/2)∗(Width/2)Citation2). Mice were sacrificed either at the time point mentioned in the text or when the tumor burden reached clinical end point, which we defined as either having a total primary tumor burden of 6 cm3 or having one mass equal to or larger than 2 cm3.
Immunofluorescence, H&E and whole mount analysis
Right side number 4 mammary pads were excised and placed on a microscope slide. They were then fixed overnight in acetone. Mammary pad was then squished between two slides to remove excess fat and then returned to acetone overnight. They were then transferred to Harris Modified Hematoxylin with acetic acid (Fisher, Cat# SH26500D) for at least 3 h to overnight. Whole mounts were then destained in 70% EtOH; 1% HCl. They were then transferred to 70% EtOH for 30 min and then to 100% EtOH for an additional 30 min. They were then put in Xylene overnight after which they were mounted using Permount (Fisher, Cat# SP15-100) and coverslip. For H&E staining, left side number 4 mammary pads or lungs were excised and fixed in 10% buffered formalin overnight and paraffin embedded. Subsequently, 4-μm thick sections were cut onto glass slides and processed for H&E staining or immunofluorescence. H&E sections were scanned using a ScanScope XT digital scanner (Aperio Technologies). For metastasis, four sections, 50-μm apart, were scanned and analyzed using ImageScope software. Lesions were counted on all four sections and averaged per section. For immunofluorescence, the slides were de-waxed with xylene twice for 5 min. The sections were rehydrated in ethanol starting at 100%, followed by 90%, 70% and 50% ethanol for 5 min each. The slides were then incubated in water for 5 min and heated at 95°C for 15 min in a 0.1 M citrate buffer (pH 6.0) for antigen retrieval. PBS 0.05 % Tween 20 was used to wash the slides. Slides were permeabilized with 0.25% Triton X-100 in PBS for 20 min at room temperature. If required, Click-iT® TUNEL Alexa Fluor® 647 (Invitrogen, Cat# C10247) staining was performed according to manufacturer's instructions. Slides were then blocked (10% FBS, 3% BSA) for 30 min at 37°C, and tissues were incubated with primary antibodies in PBS containing 3% BSA, overnight at room temperature. The antibodies against BrdU (Santa Cruz, Cat# sc-32323) and EpCAM were used (BD Biosciences, Cat# 563477). This step was followed by a 1 h incubation with conjugated secondary antibodies coupled with Alexa Fluor® 488, 594 or 647 (Invitrogen). The slides were rinsed with PBS and stained with Hoechst 33342 (Invitrogen, Cat# H3570). Tissues were mounted with coverslips and analyzed on a Zeiss Axioskop upright wide-field microscope (20×0.5 or 40×/0.75 Plan-Neofluar objectives) equipped with a high-resolution monochromatic AxioCam HRm camera and driven by AxioVision version 4.9.1 (Carl Zeiss Microscopy). When BrdU was used as a proliferative marker, mice were injected with 1 mg of BrdU (Sigma, Cat# B5002) i.p. 2 h before sacrifice.
To quantify proliferation or cell death, we used Volocity 6.0 (Quorum Technologies) to perform object-based analysis as described previously.Citation67 Briefly, the duct area was selected from epifluorescence images of mammary fat pad sections stained with proliferative (BrdU) or cell death markers (TUNEL). Fluorescent objects (representing either proliferative or dead cells) were found by applying a set threshold and objects were delineated using a segmentation procedure based on a watershed algorithm. The number of BrdU- or TUNEL-positive cells (also positive for epithelial marker EpCAM) was determined and expressed as the number of positive cells per total epithelial cells within an area. Images were collected and image processing and analysis for this manuscript was performed in the McGill University Life Sciences Complex Advanced BioImaging Facility (ABIF).
Western blot
Tissues or cells were lysed in buffer B150 (20 mM Tris–HCl pH 8.0, 150 mM KCl, 10% glycerol, 5 mM MgCl2 and 0.1% NP40) supplemented with Complete-mini protease inhibitors (Roche Applied Science, Cat# 11836153001) and phosphatase inhibitors (Sigma Cat# S7920, 71768, G6376). Protein lysates were separated on SDS-PAGE and transferred to nitrocellulose membrane. Blots were probed with antibodies against Phosphop38 (Cell Signaling, Cat# 9211), Total p38 (Cell signaling, Cat# 9212), Cyclin D1 (Thermo Scientific, Cat# RM-9104), GAPDH (Santa Cruz, Cat# sc-32233), E-Cadherin (BD, Cat# 610182), cleaved Casapse-3 (Cell Signaling, Cat# 9661), Phospho-AKT (Cell Signaling, Cat# 4060) and Total AKT (Cell Signaling, Cat# 4691).
Quantitative real-time PCR
Two micrograms of total RNA was reverse transcribed with M-MLV reverse transcriptase (Invitrogen, Cat# 28025-013) and random hexamers in a volume of 20 μL according to the manufacturer's protocol. The primers used for quantitative real-time PCR are available upon request. All reactions were normalized to the housekeeping gene L32 to quantify the relative gene expression and were then analyzed using the 2−ΔΔCT method.
Single cell suspension and FACS analysis
Single cell suspension was done as described previously.Citation44 Briefly, tumors that were approximately 1 cm3 in volume were excised and minced with a scalpel. Cell were then incubated for 1 h DMEM with Collagenase A (3 mg/mL, Roche, Cat# 11088793001) and DNase I (Roche, Cat# 10104159001) at 37°C under stirring conditions. Digestion mixtures were quenched by adding DMEM containing 10% FBS and then filtered through 0.7-μm nylon strainers (Fisher, Cat# 087712). Cells were then counted and incubated with the following antibodies at 4°C for 20 min. The following antibodies were used: CD45 (eBioscience, Cat# 25-0451-81), B220 (eBioscience, Cat# 17-0452-81), CD3 (eBioscience, Cat# 45-0031-82), NK1.1 (BD, Cat# 550627), CD11b (eBioscience, Cat# 48-0112-82), Ly6G (BD, Cat# 557661), Ly6C (eBioscience, Cat# 12-5932-82), F4/80 (eBioscience, Cat# 45-4801-82) and Live/Dead Fixable Aqua Dead Cell Stain (L34957, Invitrogen). Samples were analyzed using BD FACSCanto and FlowJo analysis software.
Ex vivo analysis
For the wound healing assay, cells derived from PyMT or PyMT/Il1r1−/− tumors were cultured in a six-well plate overnight in DMEM supplemented with 10% FBS (Wisent BioProducts, Cat# 080150), glutamine (Wisent BioProducts, Cat# 450-200-EL) and penicillin streptomycin (Wisent BioProducts, Cat# 609-065-EL). Upon confluency, cells were scratched using a P200 pipette tip. Plates were then placed in a heated chamber to maintain optimal culture condition (37°C and CO2). Wound closing was visualized using a Zeiss Axiovert 200M (10×/0.3 Plan-Neofluar objective) fully automated inverted microscope and pictures were automatically taken every 15 min for 16 h using a Zeiss Axiocam monochrome camera (3,900×3,900 pixels) driven by Axiovision 3. To calculate the percentage of wound healing, ImageJ software (National Institutes of Health, Bethesda, MD) was used.
For CSFE dilution assay, cells derived from PyMT tumors were stained with CSFE according to manufacturers' instructions and then plated on a six-well plate. Cells were treated with rIL-1α (10 ng/mL, Peprotech Cat# 211-11A), rIL-1β (10 ng/mL, Peprotech Cat# 211-11B) or both and then allowed to proliferate for 24 h, 48 h or 48 h in DMEM supplemented with 10% FBS, glutamine and penicillin streptomycin. For cell death assay, cell-derived PyMT or PyMT/Il1r1−/− tumors were cultured in a six-well plate overnight in DMEM supplemented with 10% FBS, glutamine and penicillin streptomycin. Cells were then were treated with rIL-1α (10 ng/mL, Peprotech Cat# 211-11A), rIL-1β (10 ng/mL, Peprotech Cat# 211-11B) or both for 24 h, 48 h or 72 h. Cells were then stained with Live/Dead Fixable Aqua Dead Cell Stain (L34957, Invitrogen). Samples were analyzed using BD FACSCanto and FlowJo analysis software.
Statistical analysis
Data is represented as average ± standard error. Two-tailed Student's t-test and ANOVA were used for evaluating statistical significance between groups. Kaplan–Meier was used for survival analysis. *p < 0.05; **p < 0.01; ***p < 0.001; n.s., not significant.
Disclosure of potential conflicts of interest
No potential conflicts of interest were disclosed.
KONI_A_1287247_supplementary_data.zip
Download Zip (2.3 MB)Acknowledgments
We thank Dr. Jerry Pelletier for providing PyMT mice on the C57/Bl6 background. We also thank Dr. Yoichiro Iwakura and Dr. Thirumala-Devi Kanneganti for providing Il1a−/− mice.
Funding
This work was supported by grants from the Canadian Institutes for Health Research (CIHR) and the Burroughs Wellcome Fund to M.S. who is a Fonds de Recherche en Santé du Québec (FRSQ) Senior Investigator and a McGill University William Dawson Scholar. M.D. and J.D. were supported by doctoral awards from the (CIHR), A.M. was supported by a CIHR/CAG/Abbott post-doctoral fellowship and is part of the Mitacs Accelerate program.
References
- Ferlay J, Soerjomataram I, Dikshit R, Eser S, Mathers C, Rebelo M, Parkin DM, Forman D, Bray F. Cancer incidence and mortality worldwide: sources, methods and major patterns in GLOBOCAN 2012. Int J Cancer 2015; 136:E359-86; PMID:25220842; http://dx.doi.org/10.1002/ijc.29210
- Hon JD, Singh B, Sahin A, Du G, Wang J, Wang VY, Deng FM, Zhang DY, Monaco ME, Lee P. Breast cancer molecular subtypes: from TNBC to QNBC. Am J Cancer Res 2016; 6:1864-72; PMID:27725895
- Goldhirsch A, Wood WC, Coates AS, Gelber RD, Thurlimann B, Senn HJ, Panel members. Strategies for subtypes-dealing with the diversity of breast cancer: highlights of the St. Gallen International Expert Consensus on the Primary Therapy of Early Breast Cancer 2011. Ann Oncol 2011; 22:1736-47; PMID:21709140; http://dx.doi.org/10.1093/annonc/mdr304
- Hanahan D, Weinberg RA. Hallmarks of cancer: the next generation. Cell 2011; 144:646-74; PMID:21376230; http://dx.doi.org/10.1016/j.cell.2011.02.013
- Jemal A, Siegel R, Xu J, Ward E. Cancer statistics, 2010. CA Cancer J Clin 2010; 60:277-300; PMID:20610543; http://dx.doi.org/10.3322/caac.20073
- Hoos A. Development of immuno-oncology drugs – from CTLA4 to PD1 to the next generations. Nat Rev Drug Discov 2016; 15:235-47; PMID:26965203; http://dx.doi.org/10.1038/nrd.2015.35
- Apte RN, Dotan S, Elkabets M, White MR, Reich E, Carmi Y, Song X, Dvozkin T, Krelin Y, Voronov E. The involvement of IL-1 in tumorigenesis, tumor invasiveness, metastasis and tumor-host interactions. Cancer Metastasis Rev 2006; 25:387-408; PMID:17043764; http://dx.doi.org/10.1007/s10555-006-9004-4
- Dinarello CA. Why not treat human cancer with interleukin-1 blockade? Cancer Metastasis Rev 2010; 29:317-29; PMID:20422276; http://dx.doi.org/10.1007/s10555-010-9229-0
- Dinarello CA. An expanding role for interleukin-1 blockade from gout to cancer. Mol Med 2014; 20 Suppl 1:S43-58; PMID:25549233; http://dx.doi.org/10.2119/molmed.2014.00232
- Zitvogel L, Kepp O, Galluzzi L, Kroemer G. Inflammasomes in carcinogenesis and anticancer immune responses. Nat Immunol 2012; 13:343-51; PMID:22430787; http://dx.doi.org/10.1038/ni.2224
- Di Paolo NC, Shayakhmetov DM. Interleukin 1alpha and the inflammatory process. Nat Immunol 2016; 17:906-13; PMID:27434011; http://dx.doi.org/10.1038/ni.3503
- Auron PE, Webb AC, Rosenwasser LJ, Mucci SF, Rich A, Wolff SM, Dinarello CA. Nucleotide sequence of human monocyte interleukin 1 precursor cDNA. Proc Natl Acad Sci USA 1984; 81:7907-11; PMID:6083565; http://dx.doi.org/10.1073/pnas.81.24.7907
- Lomedico PT, Gubler U, Hellmann CP, Dukovich M, Giri JG, Pan YC, Collier K, Semionow R, Chua AO, Mizel SB. Cloning and expression of murine interleukin-1 cDNA in Escherichia coli. Nature 1984; 312:458-62; PMID:6209582; http://dx.doi.org/10.1038/312458a0
- March CJ, Mosley B, Larsen A, Cerretti DP, Braedt G, Price V, Gillis S, Henney CS, Kronheim SR, Grabstein K et al. Cloning, sequence and expression of two distinct human interleukin-1 complementary DNAs. Nature 1985; 315:641-7; PMID:2989698; http://dx.doi.org/10.1038/315641a0
- Kim B, Lee Y, Kim E, Kwak A, Ryoo S, Bae SH, Azam T, Kim S, Dinarello CA. The Interleukin-1α precursor is biologically active and is likely a key alarmin in the IL-1 family of cytokines. Front Immunol 2013; 4:391; PMID:24312098; http://dx.doi.org/10.3389/fimmu.2013.00391
- He Y, Hara H, Nunez G. Mechanism and regulation of NLRP3 inflammasome activation. Trends Biochem Sci 2016; 41(12):1012-21; PMID:27669650; http://dx.doi.org/10.1016/j.tibs.2016.09.002
- Afonina IS, Tynan GA, Logue SE, Cullen SP, Bots M, Luthi AU, Reeves EP, McElvaney NG, Medema JP, Lavelle EC et al. Granzyme B-dependent proteolysis acts as a switch to enhance the proinflammatory activity of IL-1alpha. Mol Cell 2011; 44:265-78; PMID:22017873; http://dx.doi.org/10.1016/j.molcel.2011.07.037
- Kurt-Jones EA, Beller DI, Mizel SB, Unanue ER. Identification of a membrane-associated interleukin 1 in macrophages. Proc Natl Acad Sci USA 1985; 82:1204-8; PMID:3919388; http://dx.doi.org/10.1073/pnas.82.4.1204
- Wessendorf JH, Garfinkel S, Zhan X, Brown S, Maciag T. Identification of a nuclear localization sequence within the structure of the human interleukin-1 alpha precursor. J Biol Chem 1993; 268:22100-4; PMID:8408068
- Apte RN, Dvorkin T, Song X, Fima E, Krelin Y, Yulevitch A, Gurfinkel R, Werman A, White RM, Argov S et al. Opposing effects of IL-1 alpha and IL-1 beta on malignancy patterns. Tumor cell-associated IL-1 alpha potentiates anti-tumor immune responses and tumor regression, whereas IL-1 beta potentiates invasiveness. Adv Exp Med Biol 2000; 479:277-88; PMID:10897428
- Song X, Voronov E, Dvorkin T, Fima E, Cagnano E, Benharroch D, Shendler Y, Bjorkdahl O, Segal S, Dinarello CA et al. Differential effects of IL-1 alpha and IL-1 beta on tumorigenicity patterns and invasiveness. J Immunol 2003; 171:6448-56; PMID:14662844; http://dx.doi.org/10.4049/jimmunol.171.12.6448
- Voronov E, Shouval DS, Krelin Y, Cagnano E, Benharroch D, Iwakura Y, Dinarello CA, Apte RN. IL-1 is required for tumor invasiveness and angiogenesis. Proc Natl Acad Sci USA 2003; 100:2645-50; PMID:12598651; http://dx.doi.org/10.1073/pnas.0437939100
- Krelin Y, Voronov E, Dotan S, Elkabets M, Reich E, Fogel M, Huszar M, Iwakura Y, Segal S, Dinarello CA et al. Interleukin-1beta-driven inflammation promotes the development and invasiveness of chemical carcinogen-induced tumors. Cancer Res 2007; 67:1062-71; PMID:17283139; http://dx.doi.org/10.1158/0008-5472.CAN-06-2956
- Murphy JE, Morales RE, Scott J, Kupper TS. IL-1 alpha, innate immunity, and skin carcinogenesis: the effect of constitutive expression of IL-1 alpha in epidermis on chemical carcinogenesis. J Immunol 2003; 170:5697-703; PMID:12759452; http://dx.doi.org/10.4049/jimmunol.170.11.5697
- Giavazzi R, Garofalo A, Bani MR, Abbate M, Ghezzi P, Boraschi D, Mantovani A, Dejana E. Interleukin 1-induced augmentation of experimental metastases from a human melanoma in nude mice. Cancer Res 1990; 50:4771-5; PMID:2196116
- Lauri D, Bertomeu MC, Orr FW, Bastida E, Sauder D, Buchanan MR. Interleukin-1 increases tumor cell adhesion to endothelial cells through an RGD dependent mechanism: in vitro and in vivo studies. Clin Exp Metastasis 1990; 8:27-32; PMID:2293911; http://dx.doi.org/10.1007/BF00155590
- Lewis AM, Varghese S, Xu H, Alexander HR. Interleukin-1 and cancer progression: the emerging role of interleukin-1 receptor antagonist as a novel therapeutic agent in cancer treatment. J Transl Med 2006; 4:48; PMID:17096856; http://dx.doi.org/10.1186/1479-5876-4-48
- Douvdevani A, Huleihel M, Zoller M, Segal S, Apte RN. Reduced tumorigenicity of fibrosarcomas which constitutively generate IL-1 alpha either spontaneously or following IL-1 alpha gene transfer. Int J Cancer 1992; 51:822-30; PMID:1612788; http://dx.doi.org/10.1002/ijc.2910510526
- Apte RN, Douvdevani A, Zoller M, White RM, Dvorkin T, Shimoni N, Fima E, Hacham M, Huleihel M, Benharroch D et al. Cytokine-induced tumor immunogenicity: endogenous interleukin-1 alpha expressed by fibrosarcoma cells confers reduced tumorigenicity. Immunol Lett 1993; 39:45-52; PMID:8144190; http://dx.doi.org/10.1016/0165-2478(93)90163-V
- Voronov E, Weinstein Y, Benharroch D, Cagnano E, Ofir R, Dobkin M, White RM, Zoller M, Barak V, Segal S et al. Antitumor and immunotherapeutic effects of activated invasive T lymphoma cells that display short-term interleukin 1alpha expression. Cancer Res 1999; 59:1029-35; PMID:10070959
- Dvorkin T, Song X, Argov S, White RM, Zoller M, Segal S, Dinarello CA, Voronov E, Apte RN. Immune phenomena involved in the in vivo regression of fibrosarcoma cells expressing cell-associated IL-1alpha. J Leukoc Biol 2006; 80:96-106; PMID:16707560; http://dx.doi.org/10.1189/jlb.0905509
- Danforth DN Jr, Sgagias MK. Interleukin-1 alpha and interleukin-6 act additively to inhibit growth of MCF-7 breast cancer cells in vitro. Cancer Res 1993; 53:1538-45; PMID:8453620
- Sgagias MK, Kasid A, Danforth DN, Jr. Interleukin-1 alpha and tumor necrosis factor-alpha (TNF alpha) inhibit growth and induce TNF messenger RNA in MCF-7 human breast cancer cells. Mol Endocrinol 1991; 5:1740-7; PMID:1779975; http://dx.doi.org/10.1210/mend-5-11-1740
- Morinaga Y, Hayashi H, Takeuchi A, Onozaki K. Antiproliferative effect of interleukin 1 (IL-1) on tumor cells: G0-G1 arrest of a human melanoma cell line by IL-1. Biochem Biophys Res Commun 1990; 173:186-92; PMID:2256913; http://dx.doi.org/10.1016/S0006-291X(05)81039-3
- Maund SL, Barclay WW, Hover LD, Axanova LS, Sui G, Hipp JD, Fleet JC, Thorburn A, Cramer SD. Interleukin-1alpha mediates the antiproliferative effects of 1,25-dihydroxyvitamin D3 in prostate progenitor/stem cells. Cancer Res 2011; 71:5276-86; PMID:21653679; http://dx.doi.org/10.1158/0008-5472.CAN-10-2160
- Maund SL, Shi L, Cramer SD. A role for interleukin-1 alpha in the 1,25 dihydroxyvitamin D3 response in mammary epithelial cells. PLoS One 2013; 8:e81367; PMID:24244740; http://dx.doi.org/10.1371/journal.pone.0081367
- Guy CT, Cardiff RD, Muller WJ. Induction of mammary tumors by expression of polyomavirus middle T oncogene: a transgenic mouse model for metastatic disease. Mol Cell Biol 1992; 12:954-61; PMID:1312220; http://dx.doi.org/10.1128/MCB.12.3.954
- Marcotte R, Muller WJ. Signal transduction in transgenic mouse models of human breast cancer – implications for human breast cancer. J Mammary Gland Biol Neoplasia 2008; 13:323-35; PMID:18651209; http://dx.doi.org/10.1007/s10911-008-9087-3
- Herschkowitz JI, Simin K, Weigman VJ, Mikaelian I, Usary J, Hu Z, Rasmussen KE, Jones LP, Assefnia S, Chandrasekharan S et al. Identification of conserved gene expression features between murine mammary carcinoma models and human breast tumors. Genome Biol 2007; 8:R76; PMID:17493263; http://dx.doi.org/10.1186/gb-2007-8-5-r76
- Uhlen M, Fagerberg L, Hallstrom BM, Lindskog C, Oksvold P, Mardinoglu A, Sivertsson Å, Kampf C, Sjöstedt E, Asplund A et al. Proteomics. Tissue-based map of the human proteome. Science 2015; 347:1260419; PMID:25613900; http://dx.doi.org/10.1126/science.1260419
- Mikaelian I, Hovick M, Silva KA, Burzenski LM, Shultz LD, Ackert-Bicknell CL, Cox GA, Sundberg JP. Expression of terminal differentiation proteins defines stages of mouse mammary gland development. Vet Pathol 2006; 43:36-49; PMID:16407485; http://dx.doi.org/10.1354/vp.43-1-36
- Verderame M, Alcorta D, Egnor M, Smith K, Pollack R. Cytoskeletal F-actin patterns quantitated with fluorescein isothiocyanate-phalloidin in normal and transformed cells. Proc Natl Acad Sci USA 1980; 77:6624-8; PMID:6256751; http://dx.doi.org/10.1073/pnas.77.11.6624
- Francia G, Cruz-Munoz W, Man S, Xu P, Kerbel RS. Mouse models of advanced spontaneous metastasis for experimental therapeutics. Nat Rev Cancer 2011; 11:135-41; PMID:21258397; http://dx.doi.org/10.1038/nrc3001
- DeNardo DG, Barreto JB, Andreu P, Vasquez L, Tawfik D, Kolhatkar N, Coussens LM. CD4(+) T cells regulate pulmonary metastasis of mammary carcinomas by enhancing protumor properties of macrophages. Cancer Cell 2009; 16:91-102; PMID:19647220; http://dx.doi.org/10.1016/j.ccr.2009.06.018
- Lin EY, Nguyen AV, Russell RG, Pollard JW. Colony-stimulating factor 1 promotes progression of mammary tumors to malignancy. J Exp Med 2001; 193:727-40; PMID:11257139; http://dx.doi.org/10.1084/jem.193.6.727
- Qian BZ, Li J, Zhang H, Kitamura T, Zhang J, Campion LR, Kaiser EA, Snyder LA, Pollard JW. CCL2 recruits inflammatory monocytes to facilitate breast-tumour metastasis. Nature 2011; 475:222-5; PMID:21654748; http://dx.doi.org/10.1038/nature10138
- DeNardo DG, Brennan DJ, Rexhepaj E, Ruffell B, Shiao SL, Madden SF, Gallagher WM, Wadhwani N, Keil SD, Junaid SA et al. Leukocyte complexity predicts breast cancer survival and functionally regulates response to chemotherapy. Cancer Discov 2011; 1:54-67; PMID:22039576; http://dx.doi.org/10.1158/2159-8274.CD-10-0028
- Szasz AM, Lanczky A, Nagy A, Forster S, Hark K, Green JE, Boussioutas A, Busuttil R, Szabó A, Győrffy B. Cross-validation of survival associated biomarkers in gastric cancer using transcriptomic data of 1,065 patients. Oncotarget 2016; 7(31):49322-33; PMID:27384994; http://dx.doi.org/10.18632/oncotarget.10337
- Rider P, Carmi Y, Guttman O, Braiman A, Cohen I, Voronov E, White MR, Dinarello CA, Apte RN. IL-1alpha and IL-1beta recruit different myeloid cells and promote different stages of sterile inflammation. J Immunol 2011; 187:4835-43; PMID:21930960; http://dx.doi.org/10.4049/jimmunol.1102048
- Gross O, Yazdi AS, Thomas CJ, Masin M, Heinz LX, Guarda G, Quadroni M, Drexler SK, Tschopp J. Inflammasome activators induce interleukin-1alpha secretion via distinct pathways with differential requirement for the protease function of caspase-1. Immunity 2012; 36:388-400; PMID:22444631; http://dx.doi.org/10.1016/j.immuni.2012.01.018
- Derksen PW, Liu X, Saridin F, van der Gulden H, Zevenhoven J, Evers B, van Beijnum JR, Griffioen AW, Vink J, Krimpenfort P et al. Somatic inactivation of E-cadherin and p53 in mice leads to metastatic lobular mammary carcinoma through induction of anoikis resistance and angiogenesis. Cancer Cell 2006; 10:437-49; PMID:17097565; http://dx.doi.org/10.1016/j.ccr.2006.09.013
- Coffelt SB, Kersten K, Doornebal CW, Weiden J, Vrijland K, Hau CS, Verstegen NJ, Ciampricotti M, Hawinkels LJ, Jonkers J et al. IL-17-producing γδ T cells and neutrophils conspire to promote breast cancer metastasis. Nature 2015; 522:345-8; PMID:25822788; http://dx.doi.org/10.1038/nature14282
- Barak V, Nisman B, Polliack A, Vannier E, Dinarello CA. Correlation of serum levels of interleukin-1 family members with disease activity and response to treatment in hairy cell leukemia. Eur Cytokine Netw 1998; 9:33-9; PMID:9613675
- Fujiwaki R, Iida K, Nakayama K, Kanasaki H, Hata K, Katabuchi H, Okamura H, Miyazaki K. Clinical significance of interleukin-1 receptor antagonist in patients with cervical carcinoma. Gynecol Oncol 2003; 89:77-83; PMID:12694657; http://dx.doi.org/10.1016/S0090-8258(02)00154-3
- Mustea A, Pirvulescu C, Konsgen D, Braicu EI, Yuan S, Sun P, Lichtenegger W, Sehouli J. Decreased IL-1 RA concentration in ascites is associated with a significant improvement in overall survival in ovarian cancer. Cytokine 2008; 42:77-84; PMID:18329282; http://dx.doi.org/10.1016/j.cyto.2008.01.011
- Kaminska J, Kowalska MM, Nowacki MP, Chwalinski MG, Rysinska A, Fuksiewicz M. CRP, TNF-alpha, IL-1ra, IL-6, IL-8 and IL-10 in blood serum of colorectal cancer patients. Pathol Oncol Res 2000; 6:38-41; PMID:10749586; http://dx.doi.org/10.1007/BF03032656
- Ebrahimi B, Tucker SL, Li D, Abbruzzese JL, Kurzrock R. Cytokines in pancreatic carcinoma: correlation with phenotypic characteristics and prognosis. Cancer 2004; 101:2727-36; PMID:15526319; http://dx.doi.org/10.1002/cncr.20672
- Lv M, Xiaoping X, Cai H, Li D, Wang J, Fu X, Yu F, Sun M, Lv Z. Cytokines as prognostic tool in breast carcinoma. Front Biosci (Landmark Ed) 2011; 16:2515-26; PMID:21622192; http://dx.doi.org/10.2741/3869
- Ricote M, Garcia-Tunon I, Bethencourt FR, Fraile B, Paniagua R, Royuela M. Interleukin-1 (IL-1alpha and IL-1beta) and its receptors (IL-1RI, IL-1RII, and IL-1Ra) in prostate carcinoma. Cancer 2004; 100:1388-96; PMID:15042672; http://dx.doi.org/10.1002/cncr.20142
- Iwagaki H, Hizuta A, Tanaka N. Interleukin-1 receptor antagonists and other markers in colorectal cancer patients. Scand J Gastroenterol 1997; 32:577-81; PMID:9200291; http://dx.doi.org/10.3109/00365529709025103
- Ito H, Miki C. Profile of circulating levels of interleukin-1 receptor antagonist and interleukin-6 in colorectal cancer patients. Scand J Gastroenterol 1999; 34:1139-43; PMID:10582766; http://dx.doi.org/10.1080/003655299750024959
- Parekh DJ, Ankerst DP, Baillargeon J, Higgins B, Platz EA, Troyer D, Hernandez J, Leach RJ, Lokshin A, Thompson IM. Assessment of 54 biomarkers for biopsy-detectable prostate cancer. Cancer Epidemiol Biomark Prev 2007; 16:1966-72; PMID:17932343; http://dx.doi.org/10.1158/1055-9965.EPI-07-0302
- Niedzwiecki S, Stepien T, Kuzdak K, Stepien H, Krupinski R, Seehofer D, Rayes N, Ulrich F. Serum levels of interleukin-1 receptor antagonist (IL-1ra) in thyroid cancer patients. Langenbecks Arch Surg 2008; 393:275-80; PMID:18064485; http://dx.doi.org/10.1007/s00423-007-0251-9
- Gherardi RK, Belec L, Soubrier M, Malapert D, Zuber M, Viard JP, Intrator L, Degos JD, Authier FJ. Overproduction of proinflammatory cytokines imbalanced by their antagonists in POEMS syndrome. Blood 1996; 87:1458-65; PMID:8608236
- Poch B, Lotspeich E, Ramadani M, Gansauge S, Beger HG, Gansauge F. Systemic immune dysfunction in pancreatic cancer patients. Langenbecks Arch Surg 2007; 392:353-8; PMID:17235586; http://dx.doi.org/10.1007/s00423-006-0140-7
- Qian B, Deng Y, Im JH, Muschel RJ, Zou Y, Li J, Lang RA, Pollard JW. A distinct macrophage population mediates metastatic breast cancer cell extravasation, establishment and growth. PLoS One 2009; 4:e6562; PMID:19668347; http://dx.doi.org/10.1371/journal.pone.0006562
- Dagenais M, Dupaul-Chicoine J, Champagne C, Skeldon A, Morizot A, Saleh M. A critical role for cellular inhibitor of protein 2 (cIAP2) in colitis-associated colorectal cancer and intestinal homeostasis mediated by the inflammasome and survival pathways. Mucosal Immunol 2016; 9:146-58; PMID:26037070; http://dx.doi.org/10.1038/mi.2015.46