ABSTRACT
Checkpoint inhibition has established immunotherapy as a major modality of cancer treatment. However, the success of cancer immunotherapy is still limited as immune regulation of tumor immunity is very complicated and mechanisms involved may also differ among cancer types. Beside checkpoints, other good candidates for immunotherapy are immunosuppressive cytokines. TGF-β is a very potent immunosuppressive cytokine involved in suppression of tumor immunity and also necessary for the function of some regulatory cells. TGF-β has three isoforms, TGF-β 1, 2 and 3. It has been demonstrated in multiple mouse tumor models that inhibition of all three isoforms of TGF-β facilitates natural tumor immunosurveillance and tumor vaccine efficacy. However, individual isoforms of TGF-β are not well studied yet. Here, by using monoclonal antibodies (mAbs) specific for TGF-β isoforms, we asked whether it is necessary to inhibit TGF-β3 to enhance tumor immunity. We found that blockade of TGF-β1 and 2 and of all isoforms provided similar effects on tumor natural immunosurveillance and therapeutic vaccine-induced tumor immunity. The protection was CD8+ T cell-dependent. Blockade of TGF-β increased vaccine-induced Th1-type response measured by IFNγ production or T-bet expression in both tumor draining lymph nodes and tumors, although it did not increase tumor antigen-specific CD8+ T cell numbers. Therefore, protection correlated with qualitative rather than quantitative changes in T cells. Furthermore, when combined with PD-1 blockade, blockade of TGF-β1 and 2 further increased vaccine efficacy. In conclusion, blocking TGF-β1 and 2 is sufficient to enhance tumor immunity, and it can be further enhanced with PD-1 blockade.
KEYWORDS:
Introduction
Since the FDA approval of checkpoint inhibitors, ipilimumab (anti-CTLA4), nivolumab (anti-PD-1), pembrolizumab (anti-PD-1) and atezolizumab (anti-PD-L1) for the treatment of metastatic melanoma followed by the approval for treating several other cancers, immunotherapy of cancer has become a new modality of cancer treatment. These drugs opened the door for a new era of immunotherapy of cancer. However, there are still a significant fraction of cancer patients who do not benefit from the existing checkpoint inhibitor therapies.
TGF-β is a pleiotropic cytokine. In the context of immunity, this cytokine has been shown to be involved in multiple pathways regulating immune cells. For instance, TGF-β has been reported to play a critical role in the IgA class switch of B cellsCitation1 and accumulation of MDSCs.Citation2 Together with other cytokines such as IL-2 and IL-6, it is known to induce Treg and IL-17-producing T cells, respectively.Citation3,4 In addition to those functions modulating differentiation of immune cells, TGF-β has direct strong suppressive activity against conventional T cells and NK cells. Some Treg cells use surface TGF-β to suppress T cells through cell-to-cell contact.Citation5 Together with the observations that cancer cells often overproduce TGF-β, it has been suggested that cancer-derived TGF-β plays a critical role in immune suppression occurring in cancer patients. However, TGF-β can be produced by many types of cells, including activated T cells. Importantly, elevated levels of TGF-β, irrespective of cell source, have been implicated in poor disease outcome in multiple types of cancer.Citation6
Although TGF-β comprises three isoforms that all bind to the same receptor, they have significantly distinct physiologic functions that can be observed as different phenotypes of TGF-β isoform gene deficient mice.Citation7 Among the three isoforms of TGF-β, most immunological studies used TGF-β1 as TGF-β. The immunological activities of TGF-β described above are mostly based on the studies with TGF-β1. Similar to TGF-β1, TGF-β2 has been reported to have an immunosuppressive function by altering APC functions in anterior chamber-induced immune deviation in the eye.Citation8,9 In contrast to these two isoforms, only a very limited amount of information is available on the function of TGF-β3 in immunology.Citation7 Lee et al. reported that Th17 cells induced by the combination of TGF-β3 and IL-6 are more pathogenic than the cells induced by the combination of TGF-β1 and IL-6 in mouse EAE, a model of multiple sclerosis.Citation10 TGF-β3 produced by LAG3+ Treg (Tr1) cells suppresses B cell proliferation and antibody production.Citation11 TGF-β3 enhancement of PD-1 expression on B cells was necessary for the suppression.
We and others have reported that blockade of TGF-β facilitates natural tumor immunosurveillance as well as cancer vaccine efficacy in murine tumor models.Citation12-16 Either mAbs or type I TGF-β receptor kinase inhibitors used in the studies inhibit signaling from all three TGF-β isoforms. In two phase I clinical trials of GC1008 (fresolimumab), which neutralizes all three isoforms of TGF-β, treating cancer patients, some patients developed cutaneous lesions, called keratoacanthomas.Citation17 Although the lesions were benign, as they resolved following the completion of the treatment, it is always better for any treatments to have fewer side effects. Interestingly, there is a clinical study suggesting that high expression of TGF-β3 is associated with good outcome in breast cancer.Citation7 Thus, we wanted to assess whether it is necessary to block all three isoforms of TGF-β, especially TGF-β3, to facilitate tumor immunity, either natural immunosurveillance or vaccine-induced immunity.
In this study, we compared the activity of mAbs that can neutralize all three isoforms or only two isoforms, TGF-β1 and 2 (XPA089), in mouse tumor models. In a CT26 lung metastasis model, we observed that the degree of protection induced by XPA089 was similar to that induced by anti-pan TGF-β mAbs. Similarly, XPA089 as well as anti-pan TGF-β enhanced the efficacy of a therapeutic tumor vaccine in a TC1 tumor model. These results indicate that it is not necessary to block TGF-β3 among the three isoforms of TGF-β to facilitate natural tumor immunosurveillance and tumor immunity induced by the therapeutic tumor vaccine. Furthermore, the combination of anti-TGF-β and anti-PD-1 increased the vaccine efficacy to an even greater extent.
Materials and methods
Mice
BALB/c and C57BL/6 mice were purchased from Animal Production Colonies, Frederick Cancer Research Facility, NCI (Frederick, MD, USA). Animal care was in accordance with the guidelines of the NIH Animal Research Advisory Committee, and all animal experiments were approved by Animal Care and User Committee of NCI.
Tumor models
A CT26 tumor cell line was maintained as described previously.Citation13 On day 0, a half million cells were injected i.v. into the tail vein of syngeneic BALB/c mice. Starting the day of tumor injection, some mice received one of the following anti-TGF-β antibodies three times a week; 1D11 (mouse IgG, 100 µg/shot), XPA.42.068.001 (human IgG, 150 µg/shot), XPA.42.089 (human IgG, 150 µg/shot) or control human IgG (150 µg/shot). When monitored mice developed >50 tumor nodules in the lungs, all mice were killed and lungs were infused with 10% India ink solution to visualize tumor nodules. After removing excess ink, the lungs were fixed in Feke's solution.
A TC1 cell line, C57BL/6-derived lung epithelial cell line transfected with human papilloma virus (HPV) 16 E6 and E7 genes,Citation18 was maintained as described previously.Citation14 On day 0, 30 or 40 thousand cells were injected s.c. into the right flank of syngeneic C57BL/6 mice. When tumor size reached at least 5 mm in diameter, the mice were grouped to make average tumor size equal among different groups. The therapeutic vaccine consists of 160 µg of HPV16 E743–77 peptide (GQAEPDRAHYNIVTFCCKCDSTLRLCVQSTHVDIR),Citation19 1 µg of mouse GM-CSF (Peptrotech, Rocky Hill, NJ), 2.5 nmol of α-galactosylceramide (KRN7000, Funakoshi) mixed with DOTAP (Roche) was inoculated s.c. into an adjacent site of the tumor. Some mice were treated with either 1D11 (100 µg/shot), XPA.42.068.001 (150 µg/shot) or XPA.42.089 (150 µg/shot) three times a week for two weeks starting at the time of vaccination. Anti-PD-1 (clone BE0146, BioXCell, 100 µg/shot) was inoculated three times a week starting the day of vaccination. For in vivo depletion of CD8+ cells, anti-CD8+ (clone 2.43, 100 µg/shot) was injected i.p. on days 0, 1, 3, 7 after vaccination.
Tumor draining lymph node cells and tumor infiltrating leukocytes
One week after the vaccination, cells from tumor draining lymph nodes (axillary and inguinal) and tumor infiltrating leukocytes (TILs) were prepared. For TIL preparation, a tumor mass was removed from a mouse and homogenized in the digestion buffer, RPMI1640 containing 50 µg/mL Liberase TM and 0.2 mg/mL DNase I, using a gentleMACS C Tube, and incubated at 37 C for 40 min. After removing debris by passing through a nylon membrane and washing the cells, leukocytes were fractionated using Histopaque 1083 (SIGMA).
Flow cytometry
Fluorescent protein labeled mAbs against CD8+, CD3, CD4+, CD25, PD-1, TIM-3, CD11b, Gr-1, Foxp3, IFNγ, T-bet, RORγ-t were purchased from BioLegend, San Diego, CA and eBioscience, San Diego, CA. HPV16 E749–57 peptide loaded H-2Db tetramer and PBS57-loaded CD1d-tetramer were provided by the NIH tetramer core facility. For intracellular IFNγ staining, lymph node cells from tumor draining lymph nodes isolated from tumor injected mice were stimulated 5hr with PMA (20 ng/mL, Sigma) and ionomycin (1 µM, Sigma) in culture medium containing 10 µg/mL of brefeldin A (BD Biosciences, San Diago, CA). For intracellular staining and intranuclear staining, BD PharMingen intracellular staining buffers (BD Biosciences) and True-Nuclear staining buffer (BioLegned) or Foxp3/Transcritption Factor staining buffer set (Affimetrix eBioscience) was used, respectively. The fluorescence of stained cells was measured by FACSCalibur or LSRII (BD Bioscience), and data were analyzed by Flowjo (Tree Star).
Immunohistostaining of PD-L1
One week after vaccination, TC1 tumors were embedded in optimal cutting temperature compound, snap-frozen on dry ice and transferred to −80°C. Tumor sections (5 μm) on glass slides were fixed in methanol at −20°C, air-dried, hydrated and washed in PBS. Endogenous peroxidase activity (Vector Laboratories) and non-specific binding (2.5% goat serum) were blocked before staining with anti-PD-L1 (0.06μg/mL, 10F.9G2, Biolegend) and a rat IgG2b isotype control at 4°C over night. ImmPress™ HRP anti-rat IgG, mouse absorbed (Peroxidase) Polymer Detection Kit made in Goat (Vector Laboratories) was used as secondary antibody per manufacturer's instructions. ImmPACT DAB Peroxidase (HRP) substrate (Vector Laboratories) was used to detect PD-L1 staining. Slides were counter stained with hematoxylin (Vector Laboratories), dehyrdrated and mounted with Poly-Mount (Polysciences). Whole slides were scanned at x40 object magnification with the Axio Scan Z1 (Zeiss), and whole tumor and PD-L1 stained area were quantified using ImageJ (NIH).
Statistical analysis
Data were analyzed by Mann–Whitney test, one-way analysis of variance (ANOVA), repeated measures ANOVA, or proportional hazards regression using Prism (GraphPad Software Inc.) or JMP software (SAS Institutes, Cary, NC).
Results
Blockade of TGF-β3 is not necessary to enhance natural immunosurveillance
As we have previously reported that blockade of all three isoforms of TGF-β facilitates natural immunosurveillance against tumors in lung metastasis models in mice, we compared the effect of different anti-TGF-β mAbs with different isoform specificities and affinities () in a CT26 lung metastasis model (). The mice were given anti-TGF-β i.p. starting the day of tumor injection. Consistent with our previous report,Citation13 mice treated with 1D11, which neutralizes all three isoforms of TGF-β, had a significantly reduced number of tumor nodules (). Another mAb that neutralizes all three isoforms, XPA068, also reduced tumor burden in the lung. Similar to the two mAbs that neutralize TGF-β1, 2 and 3, XPA089, which neutralizes only TGF-β1 and 2 but not TGF-β3, significantly reduced tumor burden. This result suggests that among the three isoforms of TGF-β, inhibition of only two of them, TGF-β1 and 2, is sufficient for the enhancement of tumor natural immunosurveillance in the lung.
Table 1. Three anti-TGF-β clones with different affinity to each isoforms (Kd).
Figure 1. Blockade of TGF-β1 and 2 was sufficient to facilitate tumor natural immunosurveillance. BALB/c mice (five mice per group) were challenged with 5 × 105 CT26 cells. Some mice were inoculated i.p. with control human IgG (150 µg), 1D11 (100 µg), XPA068 (150 µg) or XPA089 (150 µg) three times a week for two weeks starting on the day of tumor challenge. *p = 0.0043 vs untreated group.
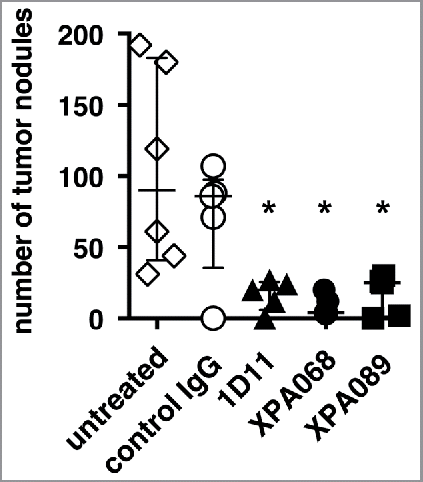
Blockade of TGF-β3 is not necessary to enhance the efficacy of a therapeutic tumor vaccine
To develop immunotherapy of cancer, it is widely recognized that combining multiple modalities increases the efficacy of the treatment. Thus, we decided to ask whether or not blockade of TGF-β3 is necessary to facilitate therapeutic tumor vaccine efficacy by using a s.c. TC1 tumor model. TC1 is a mouse lung epithelial cell line transfected with oncogenes HPV16 E6 and E7.Citation18 When tumor size reached at least 5 mm in diameter, mice were immunized with a vaccine containing HPV16 E743–77 peptide, which contains both CTL and helper epitopes,Citation19 GM-CSF and α-galactosylceramide (αGalCer), an NKT cell agonist, mixed together in DOTAP as an adjuvant, given subcutaneously. Anti-TGF-β mAbs were inoculated i.p. three times a week for two weeks starting at the time of immunization. In the vaccinated mice, the tumor growth curve diverged from that of untreated control mice approximately 7 d after the immunization, indicating that there is a time lag between the treatment (vaccination) and the clinical response ( and ). None of the three clones of anti-TGF-β antibodies tested had any impact on tumor growth when each was used as a single agent (). The combination of 1D11 with the vaccine significantly slowed tumor progression compared with the vaccine alone. Similarly, XPA068, which neutralizes all three isoforms of TGF-β, increased the vaccine efficacy, although the tumor size was not significantly different from tumors in the vaccine alone group. Additionally, XPA089, which neutralizes only TGF-β1 and 2, significantly enhanced the vaccine efficacy. This result suggests that the blockade of TGF-β1 and 2 is sufficient to enhance the therapeutic tumor vaccine efficacy and it is not necessary to block TGF-β3 to increase clinical efficacy of the therapeutic vaccine.
Figure 2. Blockade of TGF-β1 and 2 was sufficient to facilitate tumor immunity induced by a therapeutic cancer vaccine. When TC1 tumors in C57BL/6 mice (five mice per group) reached to >5 mm in diameter, some mice were inoculated s.c. with the vaccine based on E743–77 peptide (see Materials and methods section). Some mice received either control human IgG (150 µg, 1D11 (100 µg), XPA068 (150 µg) or XPA089 (150 µg) three times a week for two weeks starting on the day of vaccination. A, tumor progression curves of individual mouse are shown. B, averages tumor size of each group are shown. C, average tumor sizes of untreated mice and mice that received one of the anti-TGF-β antibodies alone without vaccine are shown. **p < 0.0001 vs vaccine alone group. *p < 0.05 vs vaccine alone group.
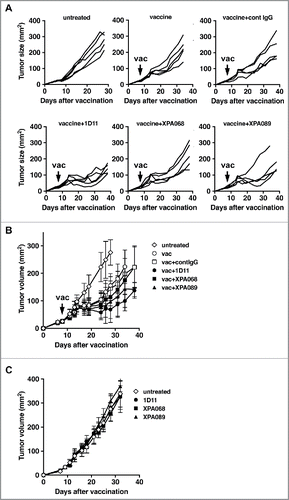
The number of Treg and MDSC was not affected by TGF-β blockade or the vaccine
To analyze the mechanism of anti-TGF-β mAbs to enhance vaccine efficacy, we examined the effect of TGF-β blockade on immunoregulatory cells, Treg cells and MDSCs, in which TGF-β has been reported to be involved in development, accumulation and function ().
Figure 3. TGF-β blockade did not affect the numbers of Foxp3+CD4+Treg cells and CD11b+Gr-1+ cells. One week after vaccination, tumor draining lymph node cells (A) and tumor infiltrating leukocytes (B and C) were harvested and stained Foxp3+CD4+Treg cells (A and B) and CD11b+Gr-1+ cells (A and C).
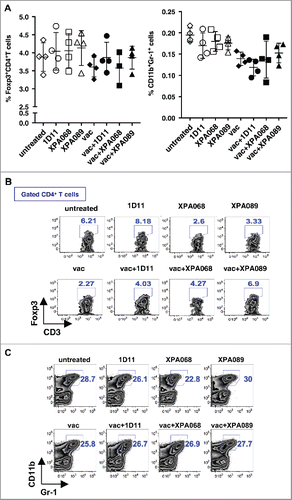
Inducible Treg cells have been shown to be induced by and express immune suppressive function through TGF-β.Citation3,5 Thus, it is possible that TGF-β blockade affects the number of Treg cells in tumor-bearing animals, which could possibly in turn increase the vaccine efficacy. To test this hypothesis, we enumerated the proportion of Foxp3+CD4+ cells in tumor-bearing mice one week after the treatment, the time before tumor growth curves among groups with different treatment diverged (to avoid effects of different tumor sizes). In both tumors and tumor draining lymph nodes, the numbers of Treg cells did not change with any treatments (anti-TGF-β alone, vaccine alone or vaccine plus anti-TGF-β) compared with that of untreated mice ( and ).
Another immunosuppressive cell population, whose recruitment to the tumor site and immunosuppressive function have been reported to involve TGF-β, is CD11b+Gr-1+ MDSCs.Citation2 Thus, the effect of TGF-β blockade on MDSCs was also assessed in tumors and draining lymph nodes of TC1-bearing mice treated with either anti-TGF-β or vaccine alone or both one week after the treatment ( and ). Similar to Treg cells, there was no effect of TGF-β blockade with/without vaccine on the number of MDSCs ( and ). The observations on Treg cells and MDSCs are consistent with our previous observation that in the TC1 tumor models, TGF-β blockade with 1D11 does not affect the number of these immunosuppressive cells.Citation14 Thus, the clinical effect of TGF-β blockade to enhance therapeutic vaccine efficacy is not due to removing immunosuppressive cell populations in either tumor draining lymph nodes or the tumor microenvironment.
Type I NKT cells in draining lymph nodes and tumors were increased by the vaccine
The vaccine used in this study contains α-GalCer, a well-characterized type I NKT (iNKT) cell agonist, as a molecular adjuvant, since α-GalCer has been reported to provide help to prime CD8+ T cells and facilitate cross-presentation of antigens to CD8+ T cells.Citation20-22 Therefore, in addition to conventional T cells, we also examined the changes in type I NKT cells in tumor draining lymph nodes (). In inguinal and axillary lymph nodes of naïve mice, draining lymph nodes of s.c. flank tumors, had approximately 0.1% of type I NKT cells defined as PBS57/CD1d-tetramer-reactive T cells among total lymph node cells (data not shown). The proportion of type I NKT cells did not change in untreated tumor bearing mice. A single agent treatment with any of the anti-TGF-β clones tested did not affect the number of type I NKT cells (). On the other hand, vaccination significantly increased the number of type I NKT cells to approximately 0.7–1.5%. However, TGF-β blockade with any of the clones used did not further increase the number of type I NKT cells in tumor draining lymph nodes. Thus, the primary mediator of the type I NKT cell increase is probably the α-GalCer adjuvant.
Figure 4. TGF-β blockade did not change the number of type I NKT cells in tumor draining lymph nodes. A–D, one week after vaccination, type I NKT cells in tumor draining lymph nodes were analyzed for the expression of CD4+, CD44 and CD69. *p = 0.0286 vs untreated by Mann–Whitney. E, when TC1 tumors in C57BL/6 (five mice per group) reached >5 mm in diameter, some mice were inoculated s.c. with the vaccine based on E743–77 peptide (see Materials and methods section) with/without α-GalCer. Average tumor sizes of each group are shown. p < 0.0001 between untreated vs vaccine and p = 0.0017 between vaccine without α-GalCer vs vaccine groups.
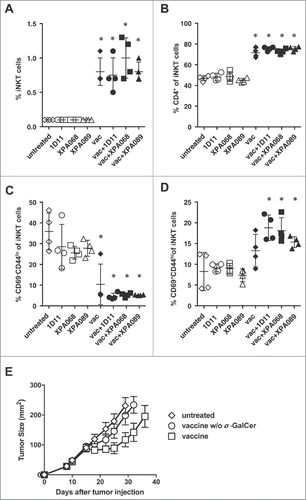
Type I NKT cells in mice have two major subsets, CD4+CD8− and CD4−CD8−. In inguinal and axillary lymph nodes, CD4+ type I NKT cells comprised approximately 40% of total type I NKT cells. This number did not change in tumor-bearing mice without treatment or with single agent treatment with any of the anti-TGF-β antibodies (). Interestingly, vaccination significantly increased the proportion of CD4+ type I NKT cells although addition of anti-TGF-β did not further change the number ().
Type I NKT cells in naïve mice are known to have CD62L−CD69+CD44hi phenotype, which is usually defined as activated T cells. Interestingly, type I NKT cells of lymph node cells from naïve mice contained approximately 30% of cells with CD69−CD44lo naïve T cell phenotype. Tumor development itself did not change this number (). While anti-TGF-β alone did not affect the numbers of type I NKT cells displaying this phenotype in tumor-bearing mice, vaccination significantly reduced the number of CD69−CD44lo type I NKT cells. Vaccine + anti-TGF-β significantly increased the number of type I NKT cells with activated phenotype CD69+CD44hi (). This result is consistent with the idea that α-GalCer in the vaccine activates type I NKT cells. However, blockade of TGF-β (all three or just two isoforms) did not further increase the magnitude of activation of type I NKT cells.
The significant role of type I NKT cells (approximately half of which are CD4+) in the vaccine efficacy was confirmed in vivo by comparing the efficacy of the vaccine with or without α-GalCer (). Only the vaccine containing α-GalCer showed significant delay of tumor progression compared with untreated mice. These results indicate that NKT cells are necessary for the protection induced by the vaccine.
TGF-β blockade enhanced induction of Th1 by the vaccine
Since the peptide used in this study contains CD4+ helper epitopes, it is possible that blockade of TGF-β enhances Th1 induction by the vaccine. To test this hypothesis, we analyzed ability of CD4+ T cells to produce IFNγ in the tumor draining lymph nodes (). A week after the vaccination, cells from draining lymph nodes of tumors were stimulated with PMA and ionomycin and stained for intracellular IFNγ. CD4+ T cells from tumor draining lymph nodes of control tumor bearing mice contained approximately 1% of IFNγ producing cells, and this frequency was not affected by single agent treatment with anti-TGF-β. In mice treated with the vaccine alone, the proportion of IFNγ+ cells was increased among CD4+ T cells (statistically significant in one experiment and not significant in another experiment). Combining vaccine with XPA068 anti-TGF-β antibody that neutralizes all three isoforms, further increased the number of IFNγ producing cells (p = 0.029 vs vaccine alone group). When combined with 089, which neutralizes only TGF-β1 and 2, the increase was not statistically significant, but there was a trend of increase (p = 0.114 vs vaccine alone group). When the numbers of IFNγ producing CD4+ T cells from all the groups of mice that received the vaccine with one of the anti-TGF-β antibodies were pooled and compared with the numbers from mice that received the vaccine alone, combination treatment significantly increased the number of IFNγ+ CD4+ T cells (, p = 0.013).
Figure 5. TGF-β blockade increased Th1 cells induced by the vaccine. A, one week after the vaccination, IFNγ production in CD4+ T cells from tumor draining lymph nodes was analyzed after PMA+ionomycin stimulation. (*p = 0.0286 vs untreated group by Mann–Whitney. p = 0.0286 vaccine alone vs vaccine + XPA068 by Mann–Whitney) In the right panel, proportions of IFNγ+CD4+ T cells of mice that received vaccine + anti-TGF-β were pooled and compared with the proportion of the cells of vaccine alone group. p = 0.0324, vaccine alone vs vaccine + anti-TGF-β. B, one week after the vaccination, T-bet and RORγ-t expression in CD4+T cells from tumor infiltrating leukocytes were analyzed. In the right panel, proportions of T-bet+CD4+ T cells of mice that received vaccine + anti-TGF-β were pooled and compared with the proportion of the cells of vaccine alone group. p = 0.025, vaccine alone vs vaccine + anti-TGF-β.
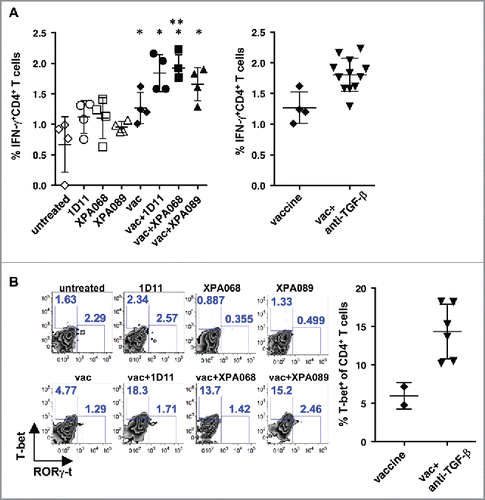
We also examined the expression of T-bet, the major Th1-specific transcription factor, in CD4+ T cells infiltrating tumors because it was difficult to obtain enough cells to perform an ex vivo stimulation assay to assess cytokine production by TILs (). Consistent with the observation with tumor draining lymph node cells, the vaccine increased the number of T-bet expressing CD4+ T cells, and the combination with anti-TGF-β further increased the proportion of T-bet expressing cells, when all mice that received vaccine + anti-TGF-β were pooled (). In contrast, there was no difference in the proportion of RORγ-t expressing cells, Th17, in any group. These results indicated that blockade of TGF-β enhances a Th1 response, which is known to contribute to antitumor immune responses, induced by the vaccine in the draining lymph nodes and among T cells infiltrating the tumors.
TGF-β blockade enhanced induction of IFNγ producing CD8+ T cells induced by the vaccine
It has previously shown that CD8+ T cells play a critical role in antitumor immunity induced by the E749–57 CTL epitope, which is included in the E743–77 peptide used in this study. Thus, we asked whether TGF-β blockade enhances induction of E749–57-specific CD8+ T cells in tumor draining lymph nodes (). First, there were virtually no E749–57-specific CD8+ T cells in the lymph nodes of TC1-bearing mice without any treatment or with single agent treatment with anti-TGF-β suggesting that even though TC1 expresses a foreign viral protein HPV16 E7, tumor cells themselves are not immunogenic enough to induce a detectable level of E749–57-specific CD8+ T cells. The vaccine significantly increased the number of E749–57-specific CD8+ T cells, and adding anti-TGF-β on top of the vaccine significantly increased the frequency of those cells compared with vaccine alone when all mice from groups of vaccine + anti-TGF-β were pooled (p = 0.05). Similarly, the number of E749–57-specific CD8+ T cells infiltrating into the tumor was increased by the vaccination, but combining anti-TGF-β with the vaccine did not further increase these cells (). Thus, TGF-β blockade on the top of the vaccine does not increase the quantity of the tumor antigen-specific CD8+ T cell in tumors.
Figure 6. TGF-β blockade increased T-bet-expressing CD8+ T cells induced by the vaccine in tumors and IFNγ-producing specific CD8+ T cells in draining lymph nodes. A, one week after the vaccination, tumor antigen-specific CD8+ T cells in tumor draining lymph nodes were enumerated by using E749–57/Db-tetramers. B and C, IFNγ production in E749–57/Db-tetramer-reactive CD8+ (B) and total CD8+ T cells (C) from tumor draining lymph nodes were analyzed after PMA+ionomycin stimulation. (*p = 0.0286 vs untreated group by Mann–Whitney.) In the right panel, proportions of IFNγ+ E749–57/Db-tetramer-reactive CD8+ T cells of mice that received vaccine + anti-TGF-β were pooled and compared with the proportion of the cells of vaccine alone group. p = 0.0324. D and D, one week after the vaccination, E749–57/Db-tetramer-reactive CD8+ T cells (D) and T-bet and RORγ-t expression in CD8+T cells (E) from tumor infiltrating leukocytes were analyzed. In the right panel, proportions of T-bet+CD8+ T cells of mice that received vaccine + anti-TGF-β were pooled and compared with the proportion of the cells of vaccine alone group. p = 0.0063, vaccine alone vs vaccine + anti-TGF-β.
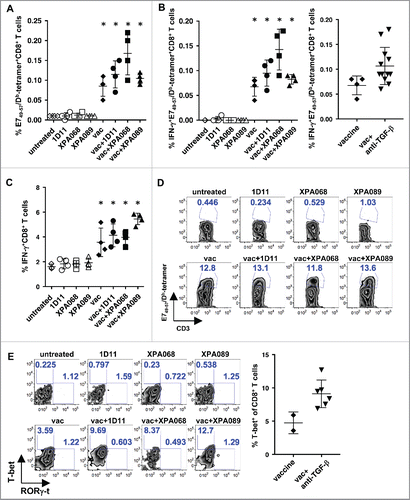
We also examined the impact of TGF-β blockade on the quality of CD8+ T cells by measuring their IFNγ production in tumor draining lymph nodes. The vaccine significantly increased the number of IFNγ+ cells among CD8+ T cells. Although anti-TGF-β alone did not increase the number of IFNγ producing CD8+ T cells and IFNγ producing E749–57-specific CD8+ T cells, when combined with the vaccine, there was a trend of further increase compared with the vaccine alone group with total CD8+ T cells (p = 0.1033) and significant increase among E749–57-specific CD8+ T cells (p = 0.0324) ().
Anti-TGF-β combined with the vaccine also increased the frequency of T-bet expressing CD8+ T cells among TILs compared with the vaccine alone when all samples from mice that received the combination were pooled (). Vaccine alone also increased the number of those cells. In contrast to T-bet expressing cells, there was no change in the frequency of RORγ-t expressing Tc17 type CD8+ T cells in tumors. These data suggest that although blockade of TGF-β did not increase the number of tumor antigen-specific CD8+ T cells induced by the vaccine, it did enhance the function measured by IFNγ production in tumor draining lymph nodes and by T-bet expression in tumors.
CD8+ T cells are necessary for the vaccine effect
So far, the data presented here indicated that blockade of TGF-β, either all three isoforms or just TGF-β1/2, combined with the therapeutic vaccine skews the quality of immune responses induced by the vaccine toward Th1, which is preferable for tumor elimination. To determine the role of CD8+ T cells in the clinical efficacy of the vaccine, we depleted CD8+ cells by administrating anti-CD8+ depletion mAb starting at the time of vaccination. As shown in , the vaccine effect was completely eliminated by CD8+ T cell depletion regardless of anti-TGF-β treatment. Thus, we conclude that CD8+ T cells are the effector cells necessary for reduction of tumor size induced by the vaccine alone or in combination with anti-TGF-β.
Figure 7. CD8+ cells were necessary for the protection induced by the vaccine + anti-TGF-β. When TC1 tumors in C57BL/6 mice (five mice per group) reached >5 mm in diameter, some mice were inoculated s.c. with the vaccine based on the E743–77 peptide (see Materials and methods section). Some vaccinated mice also received either XPA089 (150 µg) alone or XPA089 three times a week for two weeks starting on the day of vaccination and anti-CD8+ (100 µg) on days 0, 1, 7 after vaccination. A, tumor progression curves of individual mice are shown. B, average tumor sizes of each group are shown.
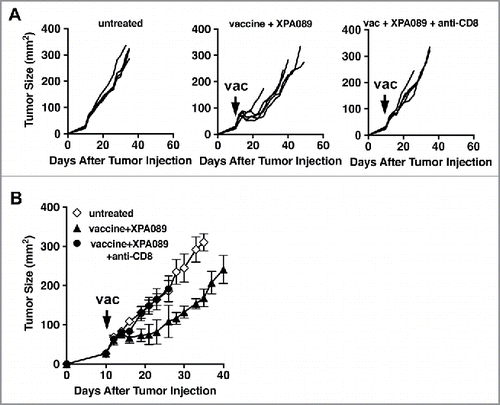
Combination with anti-PD-1
The therapeutic vaccine combined with anti-TGF-β suggest that blockade of TGF-β improves the quality of the immune responses rather than enhancing the quantity, suggesting that anti-TGF-β acts at the site of immune induction, not the effector site.
As noted, we observed an increase of IFNγ production in tumor draining lymph nodes of vaccinated mice suggesting activation of CD8+ T cells induced by the vaccine. Since PD-1 is known to be a checkpoint receptor induced upon T cell activation, we examined the expression of PD-1 and Tim-3, another checkpoint receptor on CD8+ T cells. It has been reported that among PD-1+ CD8+ T cells, the cells that also express Tim-3 are mostly the exhausted cells.Citation23 In tumor draining lymph nodes, the proportions of PD-1+ cells among CD8+ T cells and E749–57-specific CD8+ T cells were not different among all groups of mice, indicating that neither anti-TGF-β nor the vaccine alters the expression of PD-1 on CD8+ T cells (). However, interestingly the proportions of PD-1+Tim-3+ cells was significantly increased and conversely PD-1+Tim-3− cells were decreased in vaccinated mice in E749–57-specific CD8+ T cells ().
Figure 8. Expression of PD-1 on CD8+ T cells in draining lymph nodes did not change with TGF-β blockade. One week after the vaccination, the expression of PD-1 and Tim-3 on CD8+ T cells (A) and E749–57-specific CD8+ T cells (B) was analyzed. (*p = 0.0286 vs untreated group by Mann–Whitney.)
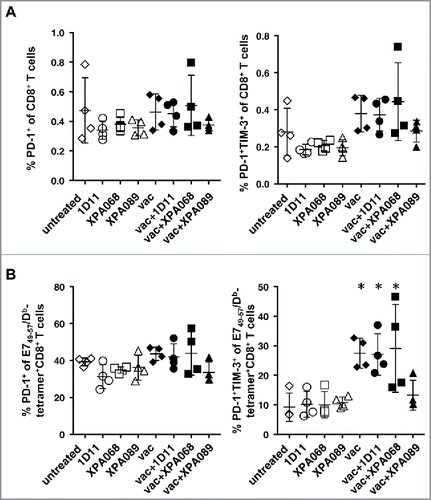
Thus, we thought that combining anti-TGF-β with a reagent that blocks a mechanism inhibiting the activity of effector CD8+T cells at the tumor site, such as anti-PD-1, may enhance the clinical effect of the therapeutic vaccine. To test this hypothesis, we examined the combination of the therapeutic vaccine with both anti-TGF-β and anti-PD-1. The combinations of either anti-TGF-β or anti-PD-1 with the vaccine were examined simultaneously to evaluate possible synergism between two blocking antibodies (). As a control, we also gave a combination of anti-TGF-β and anti-PD1 without vaccine to tumor bearing mice, and no effect on tumor progression was observed. This is consistent with a minimal expression level of PD-L1 in tumors without any treatment (). All four vaccinated groups showed regression of tumor from the peak value (). However, the groups that received vaccine plus either anti-TGF-β or anti-PD1 or both showed greater reduction and a lower nadir (p = 0.0088). We also examined the time required for the regressed tumor to regrow to 100 mm2, corresponding to approximately the level of peak size. When anti-PD-1 was combined with the vaccine, tumor growth was slowed (p = 0.0070 vs vaccine alone by proportional hazard regression). The combination of anti-TGF-β with vaccine also significantly slowed growth compared with vaccine alone (p = 0.0093). When both antibodies were combined with the vaccine, the reduction of tumor progression was even more significant versus vaccine alone (p < 0.0001), and showed a strong trend toward being better than vaccine plus a single agent (p = 0.053). Thus, the two types of checkpoint inhibitors complement each other to improve vaccine efficacy.
Figure 9. The combination of anti-TGF-β and anti-PD-1 further enhanced the efficacy of the therapeutic tumor vaccine. When TC1 tumors in C57BL/6 mice reached >5 mm in diameter, some mice were inoculated s.c. with the vaccine based on E743–77 peptide (see Materials and methods section). Some mice received either XPA089 (150 µg) and/or anti-PD-1 (100 µg) three times a week for two weeks starting on the day of vaccination. A, Tumor progression curves of individual mice are shown. B, average tumor sizes of each group are shown. See statistical analysis in Results.
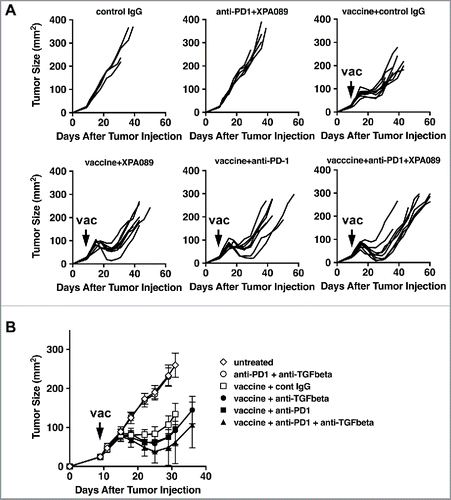
Figure 10. PD-L1 expression in tumors was low but was upregulated by the vaccine. When TC1 tumors in C57BL/6 mice reached >5 mm in diameter, some mice were inoculated s.c. with the vaccine based on E743–77 peptide (see Materials and methods section) with/without anti-TGF-β (XPA089). A week after the vaccination, tumors were harvested and stained against PD-L1. A, Percentages of PD-L1 positive area out of total tumor area. B, Representative microscopic images of tumor sections with low (upper panels) and high (lower panels) magnification. Isotype staining: tumor section stained with an isotype control antibody. *p<0.05, **p<0.01.
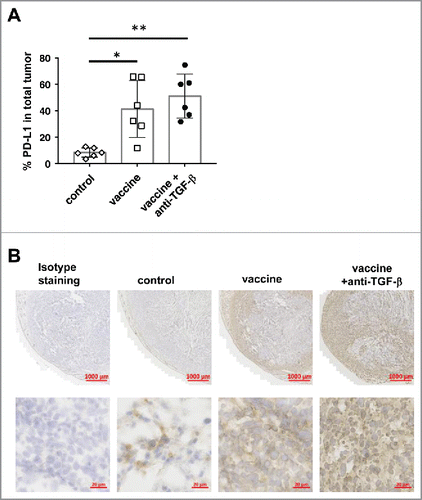
When PD-L1 expression in tumors was examined, we observed a minimal level of PD-L1 expression in untreated tumors (). The expression of PD-L1 was significantly increased in tumors of mice that received the vaccine. PD-L1 was induced in the rim of tumors, and both tumor cells and stroma cells expressed PD-L1. However, the expression level and the pattern were not further increased by adding anti-TGF-β treatment. This observation helps to explain the finding that there is no synergistic effect between anti-TGF-β and anti-PD-1 on antitumor activity.
Discussion
Hereby using a mAb that neutralizes only TGF-β1 and 2 but not TGF-β3, we found that it is not necessary to block TGF-β3 to enhance natural tumor immunosurveillance against lung metastases and to improve tumor immunity induced by the therapeutic cancer vaccine. Because TGF-β is a strong immunosuppressive cytokine, targeted therapy has been studied in many different approaches. With a few exceptions, most approaches studied do not distinguish among different isoforms. As TGF-β has multiple critical functions in homeostasis outside of immune suppression, it was important to understand whether or not it is necessary to block all isoforms of TGF-β to enhance tumor immunity to minimize potential side effects of TGF-β targeted therapies when translated into patients. Besides the study presented here, the recent result of TβM1, a mAb specific for TGF-β1, in a phase I clinical trial of cancer patients was reported.Citation24 In this report, no major adverse side effects were observed, and the activities of the drug were reported. However, it is not clear if neutralizing TGF-β1 is sufficient to enhance tumor immunity since no significant antitumor effect was observed in the patients, although the study was only a phase I study. In contrast, a phase I clinical study of GC1008, an anti-TGF-β mAb that neutralizes all isoforms, was reported to have signs of antitumor effects in some patients.Citation25 With the fact that TGF-β2 has been reported to have immunosuppressive activity, neutralizing TGF-β2 may be necessary to obtain significant antitumor effects. The results presented in the current study showing that the mAb against TGF-β1 and 2 was as effective as mAbs against all isoforms of TGF-β for its ability to facilitate tumor immunity, including both enhancement of immunosurveillance as a single agent and enhancement of cancer vaccine efficacy, indicate that it is not necessary to block TGF-β3 to inhibit immunosuppression. This finding encourages the translation of a more restricted antibody against just TGF-β1 and TGF-β2 as a checkpoint inhibitor into clinic.
This is the first study, to our knowledge, that examined the effect of a combination of anti-TGF-β and anti-PD-1 on a therapeutic cancer vaccine. Importantly, the combination of anti-TGF-β and anti-PD-1 with the vaccine additively increased therapeutic efficacy compared with the combination with either one alone with the vaccine. This result is consistent with a previous report showing that combination treatment with anti-PD-L1 and anti-TGF-β during in vitro stimulation of T cells from tumor draining lymph nodes induced better antitumor effect when the T cells were used for adoptive transfer therapy compared with T cells treated with either one alone.Citation26 These results suggest that PD-1/PD-L1 pathway and TGF-β pathway are non-redundant mechanisms damping CD8+ T cell-mediated tumor immunity, and thus blockade of the two pathways is complementary.
There are recent studies suggesting a link between TGF-β, especially TGF-β1, and PD-1 expression on T cells. It was reported that impaired T cell function in malignant pleural effusion is caused by TGF-β production by TAMs.Citation27 Co-culture of T cells with the TAMs caused significant induction of Tim-3, PD-1 and CTLA-4 and downregulated IFNγ and granzyme B production. However, blockade of TGF-β in the co-culture significantly reduced the level of Tim-3, PD-1 and CTLA-4 expression and restored the cytotoxic activity of CD8+ T cells.Citation27 Another study demonstrated that TGF-β1 induces PD-1 expression on TILs through a Smad3-dependent but Smad2-independent pathway. In the study, it was shown that the protective effect of PD-1 blockade against B16 melanoma was not seen in mice that lack one of the downstream signal transducers of TGF-β receptor, Smad3 but not Smad2 in T cells, supporting the conclusion that the TGF-β1/Smad3 pathway is necessary for the PD-1-mediated immune suppression of tumor draining lymph node cells and TILs.Citation28 This pathway seems to be conserved between mice and humans.Citation28,29 In a model of pancreatic islet allograft transplantation with anti-CD3 therapy, it was reported that TGF-β1, PD-1 and PD-L1 expression by tissue resident CD8+ T cells maintains tolerance after selective depletion of cytotoxic CD8+ T cells. Blockade of TGF-β downregulated expression of PD-1 and PD-L1, which in turn leads to the graft rejection.Citation30 In contrast to these studies, we did not observe any significant changes of PD-1 expression on CD8+ T cells in tumor draining lymph nodes. This might be due to differences among different tumor models. Another potential explanation is that in the current study, not only TGF-β1 but also at least TGF-β2 was simultaneously neutralized along with the T cell stimulation by the therapeutic vaccine. In the present study, the vaccine with or without anti-TGF-β was administered when s.c. tumors were well established (>5 mm in diameter). Therefore, T cells in tumor-challenged mice are likely already poised with multiple factors that can induce PD-1 expression, such as type I IFN.Citation31 Thus, blockade of TGF-β alone may not be sufficient to change the expression levels of PD-1 on T cells.
Interestingly enough, we did observe an increase in cells co-expressing Tim-3 among PD-1 expressing E749–57-specific CD8+ T cells in vaccinated mice, suggesting that the vaccine induced more CD8+ T cells with an “exhausted” phenotype and that TGF-β blockade did not change it. It is important to note that a recent study of single cell profiling of PD-1+Tim-3− CD8+ T cells and PD-1+Tim-3+CD8+ T cells suggested that PD-1+Tim-3+CD8+ T cells can be simply activated but not exhausted cells.Citation23 Taking into consideration that treatment with vaccine ± anti-TGF-β reduced tumor progression, it is possible that PD-1+Tim-3+ E749–57-specific CD8+ T cells in tumor draining lymph nodes of vaccinated mice are more activated cells.
The in vivo depletion study demonstrated that CD8+ T cells are effector cells required for the protection induced by the combination treatment with the vaccine plus anti-TGF-β. Besides CD8+ T cells, the treatment with the vaccine and anti-TGF-β induced changes in multiple CD4+ T cell subsets, including T-bet+CD4+ T cells and CD4+NKT cells. When α-GalCer, a strong NKT cell agonist, was omitted from the vaccine formulation, the vaccine had virtually no effect on tumor progression. This result clearly indicated a critical contribution of α-GalCer-activated NKT cells to the protection. The role of other CD4+ T cell subsets in the protection needs to be explored in a future study using approaches to target-specific CD4+ T cell subsets in vivo.
TGF-β has been demonstrated to play critical roles in the induction of iTreg cellsCitation3 and accumulation of MDSCs.Citation2 However, there were no changes in the number of either cell population in either tumor draining lymph nodes or tumors. This is consistent with previous reports that there is no impact of TGF-β blockade on several these suppressive cells. The observation that the tumor itself did not induce changes in the number of these cells in tumor draining lymph nodes may suggest that in contrast to some other tumors such as CT26 and 4T1 that are widely used to study the contribution of Treg cells and MDSCs in tumor progression, the TC1 model seems not to induce those cells to subvert the immune system. Interestingly enough, even though the TC1 tumor cell line expresses foreign viral antigens, HPV16 E6 and E7, tumor inoculation itself does not induce tumor antigen (E749–57/Db)-specific CD8+ T cells. This is different from some other mouse tumor cell lines expressing viral antigens that induce significant numbers of tumor antigen-specific T cells. Thus, it is possible that in the TC1 tumor model, Treg cells and MDSCs do not play a major role in tumor-induced immune suppression. This hypothesis is supported by our previous observation that anti-CD25 (PC61) treatment to suppress Treg cell activity does not change the progression rate of the TC1 tumor.Citation14
The therapeutic vaccine used in this study contained the type I NKT cell agonist, α-GalCer. α-GalCer has been shown by many studies not only to activate NKT cells to produce IFNγ but also, as a result of type I NKT cell activation, to induce maturation of DCs and IL-12 production by DCs, and to facilitate cross-presentation of antigens by CD8α+ DCs.Citation22,32 Although α-GalCer's ability to activate type I NKT cells is well studied by using splenic or liver NKT cells, there is limited information on NKT cells in tumor draining lymph nodes. In our study, the number of type I NKT cells in tumor draining lymph nodes was comparable to the frequency (0.1–0.2% of total lymph node cells) observed in naïve lymph nodes. Without intervention, less than 15% of type I NKT cells in tumor draining lymph nodes are CD69+CD44hi, compared with spleens and livers, where the CD69+CD44hi activated phenotype predominates.Citation33 As expected, vaccination significantly increased the total number of type I NKT cells and the proportion of cells with CD69+CD44hi activated phenotype in tumor draining lymph nodes. Interestingly, the vaccine significantly increased the proportion of the CD4+ subset. As CD4+ type I NKT cells contain NKT1 and NKT2, whereas CD4−CD8− type I NKT cells predominantly contain NKT17 (unpublished observation), it is likely that the increase of CD4+ subset is the result of an increase in the NKT1 subset. However, further analysis is required to fully address this point.
The TC1 tumor model used in this study expresses viral oncogene products, HPV16 E6 and E7. Thus, it may be immunogenic and the results obtained here may not be applicable to “non-immunogenic” tumors. However, there was no detectable level of CD8+ T cells specific for an immunodominant epitope of E7. This may be because the expression of MHC class I and II pathway genes in this tumor is comparable to a “non-immunogenic” B16 melanoma tumor.Citation34 This hypothesis can be supported by the minimal expression of PD-L1, which can be induced by IFNγ, in TC1 tumors and the ineffective monotherapy of anti-PD-1. Although the effect of anti-TGF-β combined with a vaccine needs to be further examined in “non-immunogenic” tumor models, since HPV16 is a major cause of human cervical cancer and head and neck cancer, the study is relevant to the treatment of certain types of human cancers. Furthermore, a major goal of this study was to examine the combination of a cancer vaccine with anti-TGF-β blocking different isoforms. The use of a tumor expressing defined antigens like HPV16 E6 and E7 facilitated the studies with a defined cancer vaccine to combine with anti-TGF-β.
In summary, our data demonstrate that blockade of TGF-β1 and 2 provides equivalent positive effects on both natural tumor immunosurveillance and a therapeutic vaccine as blockade of all isoforms does. Avoiding neutralization TGF-β3 may reduce the risk of potential side effects of TGF-β targeted therapies. In addition, our data now reveal that the combination of anti-TGF-β and anti-PD-1 shows a complementary effect of the two agents on the efficacy of the vaccine. Our current study provides a proof-of-principle to translate this combination to a clinical setting.
Disclosure of potential conflicts of interest
No potential conflicts of interest were disclosed.
Acknowledgments
We thank Cornelis Melief and Briggite Weiles for providing the information of the HPV16 E7-derived peptide, and T.C. Wu for providing the TC1 cell line, and the NIH tetramer facility for providing tetramers. We also thank the Vaccine Branch FACS core facility, Mr. Lake of the LGCP Microscopy core facility, all members of the tumor immunology team of the laboratory and Lisa Smith for secretarial help.
Funding
This work was supported by the Intramural Research Program of the NIH, National Cancer Institute, Center for Cancer Research and the Gui Foundation. This work was performed as part of a Collaborative Research and Development Agreement (CRADA) between NCI and the XOMA Corporation.
References
- Lebman DA, Lee FD, Coffman RL. Mechanism for transforming growth factor beta and IL-2 enhancement of IgA expression in lipopolysaccharide-stimulated B cell cultures. J Immunol 1990; 144:952-9; PMID:2295822
- Yang L, Huang J, Ren X, Gorska AE, Chytil A, Aakre M, Carbone DP, Matrisian LM, Richmond A, Lin PC et al. Abrogation of TGF beta signaling in mammary carcinomas recruits Gr-1+CD11b+ myeloid cells that promote metastasis. Cancer Cell 2008; 13:23-35; PMID:18167337; https://doi.org/10.1016/j.ccr.2007.12.004
- Li MO, Flavell RA. TGF-beta: a master of all T cell trades. Cell 2008; 134:392-404; PMID:18692464; https://doi.org/10.1016/j.cell.2008.07.025
- Bettelli E, Carrier Y, Gao W, Korn T, Strom TB, Oukka M, Weiner HL, Kuchroo VK. Reciprocal developmental pathways for the generation of pathogenic effector TH17 and regulatory T cells. Nature 2006; 441:235-8; PMID:16648838; https://doi.org/10.1038/nature04753
- Nakamura K, Kitani A, Strober W. Cell contact-dependent immunosuppression by CD4(+)CD25(+) regulatory T cells is mediated by cell surface-bound transforming growth factor beta. J Exp Med 2001; 194:629-44; PMID:11535631; https://doi.org/10.1084/jem.194.5.629
- Teicher BA. Transforming growth factor-beta and the immune response to malignant disease. Clin Cancer Res 2007; 13:6247-51; PMID:17975134; https://doi.org/10.1158/1078-0432.CCR-07-1654
- Laverty HG, Wakefield LM, Occleston NL, O'Kane S, Ferguson MW. TGF-beta3 and cancer: a review. Cytokine Growth Factor Rev 2009; 20:305-17; PMID:19656717; https://doi.org/10.1016/j.cytogfr.2009.07.002
- Takeuchi M, Alard P, Streilein JW. TGF-beta promotes immune deviation by altering accessory signals of antigen-presenting cells. J Immunol 1998; 160:1589-97; PMID:9247573; https://doi.org/10.1002/eji.1830270709
- Takeuchi M, Kosiewicz MM, Alard P, Streilein JW. On the mechanisms by which transforming growth factor-beta 2 alters antigen-presenting abilities of macrophages on T cell activation. Euro J Immunol 1997; 27:1648-56; PMID:9247573; https://doi.org/10.1002/eji.1830270709
- Lee Y, Awasthi A, Yosef N, Quintana FJ, Xiao S, Peters A, Wu C, Kleinewietfeld M, Kunder S, Hafler DA et al. Induction and molecular signature of pathogenic TH17 cells. Nat Immunol 2012; 13:991-9; PMID:22961052; https://doi.org/10.1038/ni.2416
- Okamura T, Sumitomo S, Morita K, Iwasaki Y, Inoue M, Nakachi S, Komai T, Shoda H, Miyazaki J, Fujio K et al. TGF-beta3-expressing CD4+CD25(−)LAG3+ regulatory T cells control humoral immune responses. Nat Commun 2015; 6:6329; PMID:25695838; https://doi.org/10.1038/ncomms7329
- Takaku S, Terabe M, Ambrosino E, Peng J, Lonning S, McPherson JM. Berzofsky JA. Blockade of TGF-beta enhances tumor vaccine efficacy mediated by CD8(+) T cells. Int J Cancer 2009; 126:1666-74; PMID:19830696; https://doi.org/10.1002/ijc.24961
- Terabe M, Matsui S, Park J-M, Mamura M, Noben-Trauth N, Donaldson DD, Chen W, Wahl SM, Ledbetter S, Pratt B et al. Transforming growth factor-b production and myeloid cells are an effector mechanism through which CD1d-restricted T cells block cytotoxic t lymphocyte-mediated tumor immunosurveillance: abrogation prevents tumor recurrence. J Exp Med 2003; 198:1741-52; PMID:14657224; https://doi.org/10.1084/jem.20022227
- Terabe M, Ambrosino E, Takaku S, O'Konek JJ, Venzon D, Lonning S, McPherson JM, Berzofsky JA. Synergistic enhancement of CD8+ T cell-mediated tumor vaccine efficacy by an anti-transforming growth factor-beta monoclonal antibody. Clin Cancer Res 2009; 15:6560-9; PMID:19861451; https://doi.org/10.1158/1078-0432.CCR-09-1066
- Ueda R, Fujita M, Zhu X, Sasaki K, Kastenhuber ER, Kohanbash G, McDonald HA, Harper J, Lonning S, Okada H. Systemic inhibition of transforming growth factor-beta in glioma-bearing mice improves the therapeutic efficacy of glioma-associated antigen peptide vaccines. Clin Cancer Res 2009; 15:6551-9; PMID:19861464; https://doi.org/10.1158/1078-0432.CCR-09-1067
- Kim S, Buchlis G, Fridlender ZG, Sun J, Kapoor V, Cheng G, Haas A, Cheung HK, Zhang X, Corbley M et al. Systemic blockade of transforming growth factor-beta signaling augments the efficacy of immunogene therapy. Cancer Res 2008; 68:10247-56; PMID:19074893; https://doi.org/10.1158/0008-5472.CAN-08-1494
- Lacouture ME, Morris JC, Lawrence DP, Tan AR, Olencki TE, Shapiro GI, Dezube BJ, Berzofsky JA, Hsu FJ, Guitart J. Cutaneous keratoacanthomas/squamous cell carcinomas associated with neutralization of transforming growth factor beta by the monoclonal antibody fresolimumab (GC1008). Cancer Immunol Immunother 2015; 64(4):437-46; PMID:25579378; https://doi.org/10.1007/s00262-015-1653-0
- Hung CF, Cheng WF, Hsu KF, Chai CY, He L, Ling M, Wu TC. Cancer immunotherapy using a DNA vaccine encoding the translocation domain of a bacterial toxin linked to a tumor antigen. Cancer Res 2001; 61:3698-703; PMID:11325841
- Zwaveling S, Ferreira Mota SC, Nouta J, Johnson M, Lipford GB, Offringa R, van der Burg SH, Melief CJ. Established human papillomavirus type 16-expressing tumors are effectively eradicated following vaccination with long peptides. J Immunol 2002; 169:350-8; PMID:12077264; https://doi.org/10.4049/jimmunol.169.1.350
- Hermans IF, Silk JD, Gileadi U, Salio M, Mathew B, Ritter G, Schmidt R, Harris AL, Old L, Cerundolo V. NKT cells enhance CD4+ and CD8+ T cell responses to soluble antigen in vivo through direct interaction with dendritic cells. J Immunol 2003; 171:5140-7; PMID:14607913; https://doi.org/10.4049/jimmunol.171.10.5140
- Gonzalez-Aseguinolaza G, Van Kaer L, Bergmann CC, Wilson JM, Schmieg J, Kronenberg M, Nakayama T, Taniguchi M, Koezuka Y, Tsuji M. Natural killer T cell ligand alpha-galactosylceramide enhances protective immunity induced by malaria vaccines. J Exp Med 2002; 195:617-24; PMID:11877484; https://doi.org/10.1084/jem.20011889
- Osmond TL, Farrand KJ, Painter GF, Ruedl C, Petersen TR, Hermans IF. Activated NKT cells can condition different splenic dendritic cell subsets to respond more effectively to TLR engagement and enhance cross-priming. J Immunol 2015; 195:821-31; PMID:26078270; https://doi.org/10.4049/jimmunol.1401751
- Singer M, Wang C, Cong L, Marjanovic ND, Kowalczyk MS, Zhang H, Nyman J, Sakuishi K, Kurtulus S, Gennert D et al. A Distinct gene module for dysfunction uncoupled from activation in tumor-infiltrating T cells. Cell 2016; 166:1500-11 e9; PMID:27610572; https://doi.org/10.1016/j.cell.2016.08.052
- Cohn A, Lahn MM, Williams KE, Cleverly AL, Pitou C, Kadam SK, Farmen MW, Desaiah D, Raju R, Conkling P et al. A phase I dose-escalation study to a predefined dose of a transforming growth factor-beta1 monoclonal antibody (TbetaM1) in patients with metastatic cancer. Int J Oncol 2014; 45:2221-31; PMID:25270361; https://doi.org/10.3892/ijo.2014.2679
- Morris JC, Tan AR, Olencki TE, Shapiro GI, Dezube BJ, Reiss M, Hsu FJ, Berzofsky JA, Lawrence DP. Phase I study of GC1008 (fresolimumab): a human anti-transforming growth factor-beta (TGFbeta) monoclonal antibody in patients with advanced malignant melanoma or renal cell carcinoma. PloS One 2014; 9:e90353; PMID:24618589; https://doi.org/10.1371/journal.pone.0090353
- Wei S, Shreiner AB, Takeshita N, Chen L, Zou W, Chang AE. Tumor-induced immune suppression of in vivo effector T-cell priming is mediated by the B7-H1/PD-1 axis and transforming growth factor beta. Cancer Res 2008; 68:5432-8; PMID:18593946; https://doi.org/10.1158/0008-5472.CAN-07-6598
- Li L, Yang L, Wang L, Wang F, Zhang Z, Li J, Yue D, Chen X, Ping Y, Huang L et al. Impaired T cell function in malignant pleural effusion is caused by TGF-beta derived predominantly from macrophages. Int J Cancer 2016; 139:2261-9; PMID:27459735; https://doi.org/10.1002/ijc.30289
- Park BV, Freeman ZT, Ghasemzadeh A, Chattergoon MA, Rutebemberwa A, Steigner J, Winter ME, Huynh TV, Sebald SM, Lee SJ et al. TGF-beta1-mediated Smad3 enhances PD-1 expression on antigen-specific T cells in cancer. Cancer Discov 2016; 6(12):1366-81; PMID:27683557; https://doi.org/10.1158/2159-8290.CD-15-1347
- Rekik R, Belhadj Hmida N, Ben Hmid A, Zamali I, Kammoun N, Ben Ahmed M. PD-1 induction through TCR activation is partially regulated by endogenous TGF-beta. Cell Mol Immunol 2015; 12:648-9; PMID:25363526; https://doi.org/10.1038/cmi.2014.104
- Baas M, Besancon A, Goncalves T, Valette F, Yagita H, Sawitzki B, Volk HD, Waeckel-Enée E, Rocha B, Chatenoud L et al. TGFbeta-dependent expression of PD-1 and PD-L1 controls CD8(+) T cell anergy in transplant tolerance. Elife 2016; 5:e08133; PMID:26824266; https://doi.org/10.7554/eLife.08133
- Terawaki S, Chikuma S, Shibayama S, Hayashi T, Yoshida T, Okazaki T, Honjo T. IFN-alpha directly promotes programmed cell death-1 transcription and limits the duration of T cell-mediated immunity. J Immunol 2011; 186:2772-9; PMID:21263073; https://doi.org/10.4049/jimmunol.1003208
- Fujii S, Shimizu K, Smith C, Bonifaz L, Steinman RM. Activation of natural killer T cells by alpha-galactosylceramide rapidly induces the full maturation of dendritic cells in vivo and thereby acts as an adjuvant for combined CD4 and CD8 T cell immunity to a coadministered protein. J Exp Med 2003; 198:267-79; PMID:12874260; https://doi.org/10.1084/jem.20030324
- Terabe M, Berzofsky JA. The role of NKT cells in tumor immunity. Adv Cancer Res 2008; 101:277-348; PMID:19055947; https://doi.org/10.1084/jem.20030324
- Mosely SI, Prime JE, Sainson RC, Koopmann JO, Wang DY, Greenawalt DM, Ahdesmaki MJ, Leyland R, Mullins S, Pacelli L et al. Rational selection of syngeneic preclinical tumor models for immunotherapeutic drug discovery. Cancer Immunol Res 2017; 5:29-41; PMID:27923825; https://doi.org/10.1158/2326-6066.CIR-16-0114