ABSTRACT
Tumor initiating cells or cancer stem cells (CSCs) play an important role in the initiation, development, metastasis, and recurrence of tumors. However, traditional therapies have limited effects against CSCs and targeting these cells is crucial when developing new therapeutic strategies against cancer. One potentially targetable factor is CD47, a member of the immunoglobulin superfamily. This protein acts as an anti-phagocytic “don't eat me” signal and is often found expressed by cancer cells, particularly CSCs. CD47 functions by activating signal regulatory protein-α (SIRP-α) expressed on macrophages, preventing phagocytosis. However, the role of CD47 in glioma stem cells (GSCs) has been not been thoroughly investigated. Our study therefore examined the expression and function of this protein in glioma cells and GSCs. We found that CD47 was highly expressed on glioma cells, especially GSCs, and that expression associated with worse clinical outcomes. We also found that CD47+ glioma cells possessed stem/progenitor cell-like characteristics and knocking down CD47 expression resulted in a reduction in these characteristics. Treatment with anti-CD47 antibody led to increased phagocytosis of glioma cells and GSCs by macrophages. We next examined the effects of anti-CD47 antibody on glioma cells/GSCs in an immune competent mouse glioma model, revealing significant inhibition of tumor growth and prolonged survival times. Importantly, there were no apparent side effects in the animal model. In summary, we have shown that CD47 is a potentially safe and effective therapeutic target for glioma.
Abbreviations
CCK-8 | = | Cell Counting Kit-8 |
CNS | = | central nervous system |
CSCs | = | cancer stem cells |
DMEM | = | Dulbecco's modified Eagle's medium medium |
ECL | = | enhanced chemiluminescence |
FACS | = | Fluorescence activated cell sorting. |
FBS | = | fetal bovine serum |
GSCs | = | glioma stem cells |
HRP | = | horseradish peroxidase |
HSCs | = | hematopoietic stem cells |
M-CSF | = | macrophage-colony stimulating factor |
NSCs | = | neural stem cells |
PVDF | = | polyvinylidene difluoride |
RBCs | = | red blood cells |
SDS-PAGE | = | sodium dodecyl sulfate polyacrylamide gel electrophoresis |
SIRP-α | = | signal regulatory protein-α |
Introduction
Glioblastomas are some of the most common malignant primary brain tumors found in adults.Citation1 Although conventional therapies for glioma, including surgical resection, chemotherapy, and radiation, have improved over the years, the prognosis for patients remains poor.Citation2 It is therefore essential to develop new treatments that improve the survival of affected patients. One of particular interest is an increasing body of evidence for the involvement of specific tumor initiating cells or cancer stem cells (CSCs) in various cancers, including pancreatic,Citation3–4 colon,Citation5 melanoma,Citation6 and brain cancer.Citation7 This subpopulation of cells possesses several characteristics unique to stem/progenitor cells. Importantly, these characteristics also associate with resistance to chemo-radiotherapy and increased rates of relapse after treatment.Citation7 For glioma, specific stem cells can be identified using several cell-surface markers, such as CD133, CD44, and A2B5, and initial studies targeting these molecules and pathways have had promising results.Citation7–9 Therefore, it is needed to identify of therapeutic targets against GSCs.
Another molecule associated with CSCs is CD47 (integrin associated protein), a ubiquitously expressed transmembrane glycoprotein. CD47 acts as an anti-phagocytic “don't eat me” signal, binding SIRP-α on the surfaces of macrophages. Activation of SIRP-α results in the inhibition of phagocytosis.Citation10 The role that CD47 plays in phagocytosis was first shown in red blood cells (RBCs) when it was found that CD47 expressed on RBCs protected them from phagocytosis by phagocytes.Citation11–12 CD47 is also over-expressed in circulating hematopoietic stem cells (HSCs), contributing to their avoidance of macrophage-mediated cleanrance.Citation13 Recently it has also been found that CD47 is highly expressed on numerous tumor cell types and that anti-CD47 antibody therapy can significantly inhibit the growth of cancer cells in vitro and in vivo.Citation14 More specifically, CD47 has been shown to be highly expressed on pancreatic and lung cancer stem cells and targeting CD47 can enhance the phagocytosis of these particular CSCs.Citation15–16
To establish the potential role of CD47 in gliomas and GSCs, and to investigate its potential as a therapeutic target, we investigated the expression of CD47 in various mouse and human glioma cells lines, in addition to primary cells collected from patients. This revealed that CD47 was highly expressed in glioma cells, especially GSCs, and that high levels of expression associated with worse prognoses. We also found that anti-CD47 antibody therapy enhanced macrophage-mediated phagocytosis of both glioma cells and GSCs, with no apparent side effects to normal cells. Finally, in vivo analysis showed that anti-CD47 antibodies can significantly inhibit tumor growth and prolong the survival time of mice in a glioma cells/GSCs model. These data indicate that CD47 blockade is promising potential therapeutic option to target glioma stem cells.
Results
CD47 was highly expressed by human/mouse glioma cell lines and GSCs
We evaluated the expression of CD47 on human and mouse glioma cell lines using immunofluorescence and western blotting. This showed that each of the glioma cell lines examined in the study expressed CD47, with two lines (U138 and GL261) showing exceptionally high expression. Human astrocytes (HA1800) and primary mouse astrocytes were used as the control (Fig. 1A, B). We also examined CD47 expression by western blot in primary glioma cells collected from human patients, and purified mouse GSCs from a cell line (CD133+ GL261) and primary glioma cells (Fig. 1C). CD47 expression were also detected by the FACS (Fig. 1D–E). The expression of CD47 mRNA was assessed in these primary glioma tissues using quantitative PCR CD47 mRNA expression was significantly higher in primary glioma tissue compared to adjacent non-tumor tissues (p<0.0001) (Fig. 1F). Finally, CD47 protein expression was immunohistochemically examined using paraffin sections of glioma tissues. This showed that the level of protein in primary tissues correlated with CD47 mRNA expression (Fig. 1G). Furthermore, EGFR protein was also detected in the tissues to identify tumor and healthy cells (Fig. 1H).
Figure 1. Expression of CD47 on human/mouse glioma cell lines and GSCs. (A) Immunofluorescence analysis revealed that CD47 was expressed on all of the human/mouse glioma cell lines examined in the study (U87, U251, U138, U118, A172, and GL261). Human astrocytes (HA1800) and primary mouse astrocytes were used as the controls (scale bars, 20 µm). (B) CD47 expression was relatively higher for U138 and GL261 when assessed by western blot. (C) CD47 protein levels were also assessed in primary glioma stem cells and a purified CD133+ GSC population from GL261. (D) Representative flow cytometric plots and (E) histogram plots of CD47 expression. (F) CD47 mRNA levels were measured using quantitative PCR, showing that CD47 expression was higher in primary glioma tissue (p<0.0001) compared to adjacent non-tumor tissues. (G) Representative images of CD47-specific staining and EGFR in paraffin sections of primary samples. (H) High levels of CD47 mRNA expression correlated with decreased survival of patients (p = 0.0065).
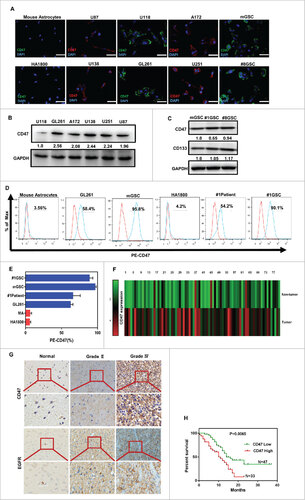
CD47 expression in primary tumor samples correlated with prognosis
The relationships between clinicopathological characteristics and CD47 expression levels in patients with glioma are summarized in . We did not find a significant association of CD47 expression levels with patient's age, sex, tumor size in 104 glioma cases. However, we observed that CD47 expression was inversely correlated with clinical stage (I-II versus III-IV) in glioma patients () (p<0.0001) This showed that high levels of CD47 significantly associated with lower overall survival rates compared to patients with low CD47 expression (p = 0.0065; Fig. 1H).
Table 1. Correlation between the clinicopathologic characteristics and expression of CD47 protein in glioma patients.
CD47+ glioma cells possessed many characteristics that associate with cancer stem cells
As CD47 appeared overexpressed in glioma stem cells, we sorted glioma cells into CD47+ and CD47- populations using fluorescence activated cell-sorting (FACS). CD47+ or CD47- glioma cells isolated from the patient #1 primary glioma cells and GL261 cells were stable in vitro along time. After passages (>10), the cell surface expression of CD47 were analyzed by flow cytometry in vitro (Fig. S1 A–B). To determine whether CD47+ glioma cells possess characteristics similar to stem/progenitor cells, we conducted several assays. Firstly, in vitro CCK-8 (Fig. 2A) and 5-ethynyl-20-deoxyuridine (EdU) assays (Fig. 2B) showed that CD47+ glioma cells had a higher proliferative activity compared to CD47- cells. Secondly, we conducted an in vivo tumor formation assay by orthotopically injecting mice with either CD47+ or CD47- glioma cells. This indicated that CD47+ glioma cells were more tumorigenic (Fig. 2C). We also found that the proliferating cell nuclear antigen (PCNA) was more highly expressed in tumors (Fig. 2D) compared to controls. The expression of CD47 were shown to further reinforce the model of CD47 expression (Fig. 2D). Thirdly, we analyzed the tumor sphere formation capacity of CD47+ or CD47- glioma cells under serum-free conditions. As shown in Fig. 2F, CD47+ glioma cells formed more spheres than CD47- cells. Further gene expression analysis also revealed that several stem cell markers, including Sox2, Oct4, and Nanog, were also significantly increased in CD47+ glioma cells (Fig. 2E). Finally, we found that CD47+ cells displayed a 3.6-fold (for GL261), 4.0-fold (for Patient 1), and 3.6-fold (for Patient 8) increase in migration efficiency using Transwell assays (Fig. 2G). A significant increase in invasive ability was also shown for CD47+ cells, with a 4.0-fold (for GL261), 2.4-fold (for Patient 1), and 3.7-fold (for Patient 8) increase compared to CD47- cells (Fig. 2H). These data demonstrate that CD47+ glioma cells exhibit many of the characteristics associated with cancer stem cells, suggesting that the CD47+ population contains glioma stem cells (GSCs).
Figure 2. CD47+ glioma cells exhibit many of the features of cancer stem cells. (A) Using CCK-8 and (B) EdU assays, we demonstrated that the proliferative activities of CD47+ glioma cells were higher than CD47- cells (scale bars, 15 µm). (C) Representative images of tumor formation using primary glioma cells from Patient 1 and GL261 cells. (D) Paraffin-embedded immunohistochemical tumor tissue sections stained with H&E, PCNA, and CD47 (20× and 40× ) . (E) Expression of stem cell markers (Sox2, Oct4, and Nanog) measured using western blot. (F) Tumor sphere formation capacities of several cells lines (Scale bar represents 100 µm). (G) Transwell migration and (H) invasion assays demonstrating that CD47+ glioma cells were more migratory and invasive, compared to CD47- cells. #p<0.05, ##p<0.01. Data are presented as means ± SD.
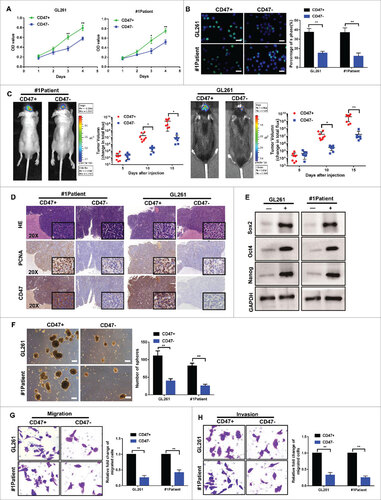
CD47 knockdown reduced the stem/progenitor cell associated characteristics of GSCs
To evaluate the functional significance of CD47 and the various stem cell-like characteristics, we used a shCD47 (short hairpin RNA) lentiviral vector to knockdown CD47 in the GL261 cell line and primary glioma cells from Patient 1. CD47 knockdown was confirmed by quantitative PCR and western blot (Fig. 3A). By CCK-8 (Fig. 3B) and EdU assays (Fig. 3C–D), we found that the proliferative activity of transfected cells was markedly reduced. To further confirm the suppressive effects of CD47 knockdown, we performed a tumor formation assay by orthotopically injecting mice with shCD47 cells and scrambled controls. This revealed that tumor volumes were significantly decreased in mice implanted with shCD47 cells and that survival increased (Fig. 3E–G). Immunohistochemistry showed that PCNA expression also decreased in these tumors (Fig. 3H). Additionally, sphere formation assays showed that shCD47 cells formed fewer and smaller spheres (Fig. 3I) and knockdown of CD47 decreased cell migration (Fig. 3J) and invasion (Fig. 3K).
Figure 3. CD47 knockdown reduced stem/progenitor features of GSCs. (A) Analysis of CD47 mRNA and CD47 protein expression in cells transfected with a shCD47 (short hairpin RNA) lentiviral vector and controls. (B) CCK-8 assays and (C) EdU assays revealed growth was suppressed in CD47 knockdown cells (scale bars, 15 µm) (D) EdU assays were also conducted by the FACS. (E-F) Representative images of mice orthotopically injected with shCD47 cells or controls. (G) Tumor volumes were significantly decreased and survival times prolonged in shCD47 cells. (H) Paraffin-embedded immunohistochemical tumor tissue sections stained with H&E and PCNA (40× ) , PCNA expression also decreased in CD47 knockdown tumor tissues. (I) The size and number of spheres were lower in shCD47 cells (Scale bar,100 µm). (J) Migration and (K) invasion were also reduced in shCD47 cells. #p<0.05, ##p<0.01. Data are presented as means ± SD.
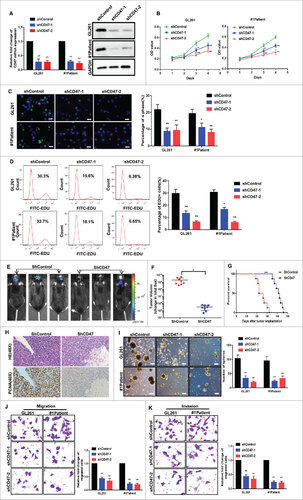
Anti-CD47 mAb treatment induced phagocytosis and was cytotoxic to glioma cells and GSCs
We next detected whether an anti-mouse CD47 monoclonal antibody (mAb: MIAP301) was able to induce phagocytosis by mouse macrophages of glioma and glioma stem cells in vitro. After 2 hours incubation with anti-mouse CD47 mAb, our data showed that GL261 cells were more efficiently phagocytized by macrophages compared to cells treated with an isotype-matched mouse IgG antibody. The phagocytic index of anti-mouse CD47 antibody treated GL261 was 23.3, much higher than the control treatment index of 5.3 (Fig. 4A). To determine whether anti-CD47 mAb could also eliminate glioma stem cells, we isolated CD133+ cells from GL261 using FACS. The enhanced phagocytic effect was also observed in these CD133+ cells (Fig. 4A). Flow cytometry method was also used to evaluated the ability of anti-CD47 mAb to induce the phagocytosis of glioma cells and glioma stem cells in vitro. Compared to the controls, anti-CD47 treatment enhanced phagocytic effect of glioma cells and glioma stem cells (Fig. 4B). CD47- GL261 cells were used to avoid any aspecific effect of anti-CD47 antibodies. There were no significant differences in the phagocytosis between anti-CD47 treatment group and controls(Fig. 4A–B). LDH release assay was conducted to establish any macrophage-mediated cytotoxicity against glioma cells and GSCs. This revealed that anti-CD47 mAb treatment significantly increased cell lysis of glioma cells and GSCs in the presence of macrophages (Fig. 4C). Finally, to confirm whether anti-CD47 mAb also promoted apoptosis of glioma cells and GSCs, we incubated these cell types in the absence of macrophages for 12 hours. No induction of apoptosis was observed in glioma cells or GSCs compared to IgG controls, confirming the effect is macrophage dependent (Fig. 4D).
Figure 4. Anti-CD47 treatment increased phagocytosis of glioma cells and GSCs and was cytotoxic. (A) Representative images (left) and phagocytic indices (right) of macrophages phagocytosing labelled tumor cells following treatment with anti-CD47 antibody. CD47- glioma cells were used to avoid any aspecific effect of anti-CD47 antibodies. Arrows indicate phagocytosed tumor cells. (B) Phagocytosis assay were also conducted by flow cytometric (left) and histogram (right) plots. (C) LDH release assay was performed in glioma cells (left) and GSCs (right) at effector:target ratios of 5:1, 10:1, 20:1, and 30:1. (D) FACS analysis of apoptosis determined using an Annexin V/PI staining kit with glioma cells and GSCs. Data are presented as means ± SD, #p<0.05, ##p< 0.01, ###p<0.001.
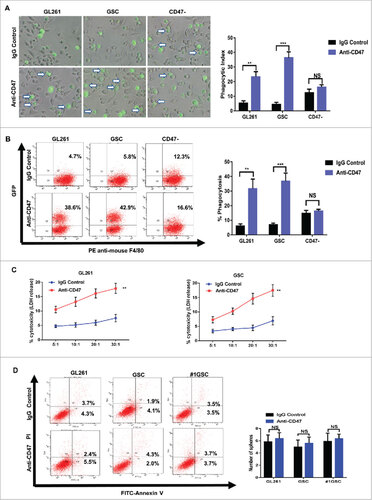
Anti-CD47 mAb inhibited tumor growth and prolonged survival in an immunocompetent syngeneic glioma cell/GSC model
To detect whether targeting CD47 could serve as an efficient therapeutic strategy for glioma and GSCs in vivo, we established a glioma cell/GSC orthotopic model to investigate the antitumor efficacy of an anti-CD47 mAb (MIAP301). An overview of the treatment scheme for glioma cell/GSC model is shown respectively in Fig. 5A, J. Luciferase-expressing GL261 cells were transplanted into the brains of C57 BL/6 mice. Tumor formation was verified using bioluminescence imaging (BLI). After a week, mice received an intraperitoneal injection of anti-CD47 mAb or IgG control antibody (16 mg/kg) every other day. Various symptoms of neurological deficit were also monitored.Citation17 This showed that anti-CD47 mAb significantly inhibited tumor growth in vivo versus control groups (Fig. 5B, C) and also prolonged survival time (Fig. 5D). Using the same method, we also transplanted CD133+ GSCs (sorted from GL261 cells) into the brains of mice. The results showed that anti-CD47 mAb also significantly inhibited tumor growth (Fig. 5K, L) and prolonged survival time in this model (Fig. 5M), suggesting that anti-CD47 mAb treatment can also target GSCs.
Figure 5a. Anti-CD47 mAb inhibited tumor growth in an immunocompetent syngeneic glioma cell/GSC model. (A, J) Treatment scheme of the GL261/GSC-implanted mice. (B, K) Representative BLI images and (C, L) measurements of tumor volume. (D, M) Anti-CD47 treatment significantly prolonged survival time. (E, N) The production of IFN-γ was detected in peripheral blood by ELISA. (F, O) H&E staining of spleen tissue showed a marked expansion in the germinal centers of the anti-CD47 treatment groups (scale bars, 100 µm). (G, P) Tumor-induced neurological deficit symptoms over time. The grading scheme used was grade 0, healthy mice; grade 1, slight unilateral paralysis; grade 2, moderate unilateral paralysis and/or the beginning of a hunched back; grade 3, severe unilateral or bilateral paralysis and pronounced a hunched back; grade 4, moribund and/or dead mice. (H, Q) Immunohistochemistry of tumors sections stained with Nissl stain and anti-GFAP antibody showing no visible damage to neurons and astrocytes in the anti-CD47 mAb groups (scale bars, 50 µm). (I, R) Immunohistochemistry of tumors sections stained with F4/80 macrophage and CD8+ T cell specific antibodies demonstrating increased infiltration at the tumor site (scale bars, 50 µm).
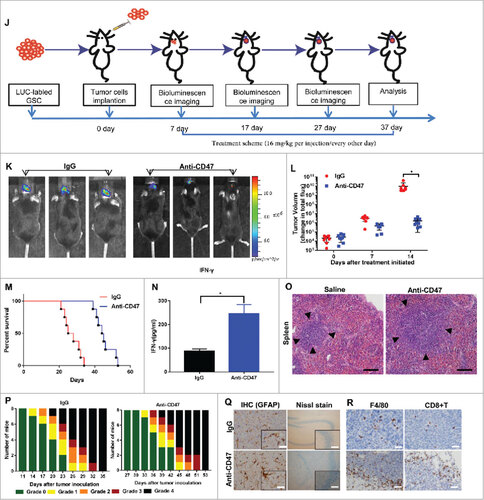
Neurological deficit scores/grades during the experiment revealed a significant delay in the beginning of clinically relevant symptoms in anti-CD47 treatment groups (Fig. 5G, P). CD8+ T cell and F4/80 macrophage specific staining also revealed increased accumulation of these cells around the tumors of mice treated with anti-CD47 mAb compared to controls (Fig. 5I, R). H&E staining of spleen tissue showed a marked expansion in the germinal centers of the anti-CD47 mAb treatment group (Fig. 5F, O). Finally, an ELISA also indicated that anti-CD47 treatment promoted the production of IFN-γ (Fig. 5E, N).
Anti-CD47 mAb had no visible side effects in a treatment model
To further assess whether anti-CD47 mAb treatment elicited any side effects in off-target cells, various concentrations of anti-CD47 mAb were added to cultured cells maintained in vitro for 4 days. The cell viability and proliferation of neurons or astrocytes were found to be unchanged (Fig.S2E). There were also no effects to neurons or astrocytes co-cultured with macrophages compared with the controls (Fig. S2F). For in vivo analysis, C57 BL/6 mice were administered an intraperitoneal injection of anti-CD47 mAb (16 mg/kg) or IgG control. Subsequent analysis of the blood showed that there was only a short period of anemia in group that received anti-CD47 mAb (Fig. S2 A–D). In the glioma cell/GSC model, Nissl and anti-GFAP staining revealed no visible damage to neurons and astrocytes after treatment with anti-CD47 mAb (Fig. 5H, Q). H&E staining samples from the heart, lung, liver, and kidneys showed no pathological differences between the two groups (Fig. S2G).
Discussion
Previous studies have suggested that targeting components of the immune system can have significant therapeutic benefits in some types of cancer. For example, CD47 is often co-opted by cancer cells as an anti-phagocytic “don't eat me” signal that binds to SIRPα on the surface of macrophages.Citation17,18 Disrupting this CD47-SIRPα axis using monoclonal antibodies has been shown to increase the phagocytosis of cancer cellsCitation20 and also inhibit the progression of solid tumorsCitation14 and hematological malignancies.Citation19,20 Recently, CD47 has been reported to be particularly overexpressed on tumor-initiating cells or cancer stem cells (CSCs).Citation15,16,21,22 However, whether the role of CD47 in glioma and glioma stem cells (GSCs) has not yet been established. CSCs are a subset of cells that possess stem-cell like properties and have been identified in various cancers, including pancreatic,Citation3,4 colon,Citation5 melanoma,Citation6 and brain cancer.Citation7 Due to these stem cell-like characteristics, CSCs are resistant to chemo-radiotherapy, contributing to relapses after treatment.Citation10 As tumors can arise from even a single CSC it is important to develop therapies that target the entire CSC population when treating cancer. However, identification of specific GSC surface markers is challenging because their expression can change after isolation.Citation23 Despite this, several cell-surface markers have been identified that appear upregulated in glioma stem cells, such as CD133, CD44, and A2B5.Citation7–9 To establish the role of CD47 in glioma cells and GSCs, we used the glioma stem cell marker CD133+ to sort cell lines and primary glioma cells to generate specific populations for further research.
In our study, we found that CD47 was highly expressed by glioma cells and, importantly, that high levels of expression associated with a poor prognosis. We also found that the CD47+ sub-population of glioma cells had many of the features of cancer stem cells, suggesting a link between CD47 expression and GSC activity. Furthermore, we showed that CD47 knockdown reduced the stem cell characteristics of GSCs and blockade of CD47 using a monoclonal antibody led to increase in vitro phagocytosis and cytotoxicity in both glioma cells and GSCs. To investigate the potential in vivo roles of CD47, we established a glioma cell/GSC orthotopic mouse model. Using this model, we confirmed that anti-CD47 mAb inhibited tumor growth and prolonged survival. Finally, immunohistochemistry demonstrated increased infiltration of CD8+ T cells and macrophages in glioma tumors after anti-CD47 treatment. Taken as a whole, these results suggest that CD47 is a potential therapeutic target for the treatment of glioma.
To further establish the potential for translation, we assessed whether anti-CD47 mAb treatment had any side effects on normal cells found in the central nervous system (CNS) cells, including neurons and astrocytes. This is important as CD47 is not only expressed on tumor cells, but also many other cell types to varying degrees. This showed that anti-CD47 treatment did not result in any severe side effects to these cells, both in vitro and in vivo. This supports similar studies showing that blockade of CD47 could inhibit tumor growth in pediatric brain tumor models without affecting cells of the CNS.Citation24 We also found that various blood indices and hepatic-renal functions were within normal levels after anti-CD47 mAb treatment, except for a short period of anemia that was consistent with previous reports.Citation14 This suggests that anti-CD47 mAb specifically induces phagocytosis and cytotoxicity in cancer cells. This may be because cancer cells express both pro-phagocytic “eat me” and anti-phagocytic “don't eat me” signaling molecules and blocking of the CD47 “don't eat me” signal leads to phagocytosis. However, normal cells lack the secondary pro-phagocytic “eat me” signal and blockade of CD47 has no effect.Citation18 Our data therefore suggest that anti-CD47 treatment can inhibit tumor growth and prolong survival in an immunocompetent glioma cell/GSC model, with no obvious signs of toxicity.
Recently, various therapies targeting the immune system have shown clinical efficacy for the treatment of various cancers. Factors of both the innate and adaptive antitumor immune response have been shown to participate in these processes. For example, anti-CD47 treatment in vitro promotes the phagocytosis of tumor cells but also induces an antitumor CD8+ T cell response.Citation25 Additionally, an increase in macrophage-mediated phagocytosis after anti-CD47 therapy stimulates both an innate and adaptive antitumor response in syngeneic mouse models.Citation26 Indeed, in our study, we found a greater number of CD8+ T cells infiltrating glioma tumors after anti-CD47 treatment and there was an associated increase in IFN-γ titers in the peripheral blood. A combination of checkpoint inhibitors, or other adaptive immune adjuvants, may therefore enhance the efficacy of immune-based therapies targeting glioma in the future. However, despite the fact that anti-CD47 mAb monotherapy has shown some promising therapeutic effects in human xenograft models, it is still insufficient to fully eradicate tumors in an syngeneic immune-competent mouse model.Citation14,26 A potential limitation of our study is that anti-CD47 treatment does not currently completely eradicate the glioma despite inhibiting tumor growth and prolonging survival.
In conclusion, we have shown that CD47 is highly expressed on glioma cells and GSCs and that expression associated with worse clinical outcomes for patients. Our results suggest that CD47+ glioma cells possess many of the characteristics specific to cancer stem cells. We have also demonstrated that disrupting the CD47-SIRPα axis is an effective treatment option for glioma and GSCs, both in vitro and in vivo. Although the exact mechanisms that underlie the anti-tumor effects of anti-CD47 still require further investigation, our study indicates that CD47 is a potentially safe and effective therapeutic target for the treatment of glioma.
Materials and methods
Cell lines and animals
Six to eight-week-old C57 BL/6 male mice, bred under specific pathogen free conditions, were purchased from the Central Animal Facility of Southern Medical University. The protocols used in the research were approved by the Animal Care and Use Committee of Southern Medical University. A murine glioma cell line (GL261), five human glioma cell lines (U87, U251, U138, U118, and A172), and human astrocytes (HA1800) were obtained from the Department of Neurosurgery, Zhujiang Hospital, Southern Medical University, Guangzhou, China. Cells lines were cultured in Dulbecco's modified Eagle's medium (DMEM) with 10% fetal bovine serum (FBS) (Gibco) in a humidified incubator at 37°C with 5% CO2. GSCs were cultured in serum-free DMEM/F12, supplemented with 20 ng/mL EGF, 20 ng/mL bFGF, and B27 (1:50) (Gibco).
Primary tumor specimens, mouse macrophages and astrocytes
Primary human tumor tissue samples were collected from the Department of Neurosurgery, Zhujiang Hospital, Southern Medical University, Guangzhou, China and immediately preserved in liquid nitrogen. All of the clinical samples were obtained from consenting patients under a regime approved by the Ethics Committee of Zhujiang hospital (No.2014-XHNK-003). Mouse bone marrow-derived monocytes (BM-MDM) were isolated from C57 BL/6 mice and seeded on non-tissue culture-treated 100 mm dishes for 7 days in RPMI-1640 (Gibco) medium containing 10% FBS, supplemented with 20 ng/mL macrophage-colony stimulating factor (M-CSF) (PeproTech). Primary cortical astrocytes were obtained from the brains of 1-day-old as C57 BL/6 mice as previously reportedCitation27 with some modifications.
Quantitative real-time PCR
Total RNA from human primary glioma cells were extracted using TRIzol (Life Technologies), according to the manufacturer's instructions. These samples were used to synthesize cDNA using SuperScript II reverse transcriptase (Life Technologies). Quantitative real-time PCR was performed using a SYBR Green PCR master mix (Life Technologies) and a LightCycler 480 II PCR system (Roche). CD47 Forward primer (5’-3’): GGCAATGACGAAGGAGGTT, Reverse primer (5’-3’): ATCCGGTGGTATGGATGAGA. β-ACTIN Forward primer (5’-3’): CATCCACGAAACTACCTTCAACTCC Reverse primer (5’-3’): GAGCCGCCGATCCACACG. Data were normalized to endogenous β-ACTIN expression levels and relative expression was calculated using the 2−δδ Ct method.Citation28 All assays were performed in triplicates and repeated three times.
In vitro phagocytosis assay
For in vitro phagocytic assays, 5× 104 monocyte-derived macrophages were seeded onto a 24-well tissue-culture plate. Tumor cells were then labeled using GFP (GENE, Shanghai, China), according to the manufacturer's protocols. Macrophages were incubated in serum-free medium for 2 h before 2× 105 GFP-labeled GL261 cells or GSC were added. Anti-CD47 antibody (Santa Cruz, MIAP301) (10μg/mL) was then added and cells were incubated for 2 h at 37°C. Afterwards, macrophages were washed several times and imaged using an inverted microscope (OLYMPUS IX71). A phagocytic index was then calculated based on the number of GFP positive cells per 100 macrophages.Citation14 In vitro phagocytosis assay was also performed by FACS. mouse macrophages were identified using PE anti-mouse F4/80 (BioLegend). Phagocytosis assays were performed in triplicates and repeated at three times.
Flow cytometry
To identify GSCs and distinguish between CD47+ and CD47- cell populations, cells were stained with PE-CD133 (Miltenyi Biotec) and PE-CD47 (BD Biosciences) for flow cytometry and sorting. CD47 expression of each cell type was also detected by the FACS. To assess apoptosis, cells were incubated with a FITC-Annexin V/PI Detection Kit (BD Biosciences), according to the manufacturer's protocols. Flow cytometry was performed on a BD FACSAria (BD Biosciences). All assays were performed independently three times.
EdU incorporation assay
For the 5-ethynyl-2′-deoxyuridine (EdU) incorporation assay, cells were assessed using a Cell-Light EdU Apollo 488 In Vitro Imaging Kit (RiboBio), according to the manufacturer's protocols. Briefly, cells were incubated with 10 mM EdU for 2 h and then fixed with 4% paraformaldehyde, permeabilized with 0.3% Triton X-100, and then stained using Apollo fluorescent dyes. Incubation with DAPI (Sigma) for 10 mins was used to stain cell nuclei. The numbers of EdU-positive cells were counted using a fluorescent microscope (OLYMPUS IX71) across five random fields. EdU assays were also conducted by the FACS. All assays were performed independently three times.
Sphere formation assay
To assess sphere formation efficiency, cells were seeded onto ultra-low attachment six-well plates and cultured in serum-free DMEM/F12 supplemented with 20 ng/mL EGF, 20 ng/mL bFGF, and B27 (1:50) (Gibco). After a week of culture, the numbers of tumor spheres greater than 100 µm in size were counted under an inverted microscope. All the experiments were performed independently three times.
Migration and invasion assay
For the transwell migration assays, 1× 105 cells were seeded into the upper chambers (BD Biosciences) with serum-free medium. A Boyden invasion assay was also performed using Matrigel (BD Biosciences) in the upper chamber. The lower chambers of both experiments contained DMEM supplemented with 10% FBS. Cells that had migrated to the lower surface of the membrane were fixed with methanol and stained with crystal violet (Sigma). Cells were then counted using an inverted microscope across five random fields. Assays were performed independently three times.
Cytokine detection by ELISA
After the intraperitoneal injection of anti-CD47 mAb (2 weeks after the treatment beginning), the peripheral blood of mice in each group was collected. The cytokine levels of IFN-γ collected from the serum was determined by ELISA kit (PeproTech) according to the manufacturer's instructions. The experiments were conducted independently three times.
Western blot analysis
Cells were lysed in RIPA and the protein concentration measured using an BCA Protein Assay Kit (Thermo Scientific). Protein lysates were separated by sodium dodecyl sulfate polyacrylamide gel electrophoresis (SDS-PAGE) and transferred to a polyvinylidene difluoride (PVDF) membrane. Membranes were blocked in 5% skimmed milk for 1 h at room temperature and then incubated overnight with primary antibodies at 4°C. This was followed by incubation with horseradish peroxidase(HRP)-labeled secondary antibodies. GAPDH was selected as an internal control. Finally, the membranes were visualized using an enhanced chemiluminescence (ECL) kit (Thermo Scientific). All the experiments were conducted independently three times.
Murine brain tumor model
Cells transduced with the lentivirus vector pUbi-MCS-LUC-IRES-puromycin (GENE) were used for the orthotopic model. After anesthesia (induced using ketamine and xylazine hydrochloride), mice were placed in a stereotactic frame. A burr hole was drilled through the skull and glioma cells injected with a 26-gauge syringe (Hamilton) at a position 1 mm lateral and 2 mm posterior of the bregma and to a depth 3 mm below the dura mater. After intracranial inoculation, mice were continuously monitored and clinical symptoms were scored three times a week. This consisted of a neurological deficit grading scale adapted from a previously described autoimmune encephalomyelitis experimental model.Citation29
Immunohistochemistry
Paraffin sections of human GBM tissue were stained with anti-CD47 antibody and anti-EGFR antibody to immunohistochemically detect CD47 and EGFR expression. In addition, sections from tissue collected during the animal experiments were prepared to assess the protein levels of CD8, PCNA, and F4/80. The primary antibodies used were mouse anti-CD47 antibody (B6H12; 1:100; Santa Cruz), rat anti-CD47 antibody (MIAP301; 1:100; Santa Cruz), rabbit anti-EGFR antibody (Ab52894; 1:100; Abcam), rabbit anti-PCNA antibody (Ab92552; 1:100; Abcam), rabbit anti-F4/80 antibody (Ab111101; 1:100; Abcam), rabbit anti-CD8 antibody (Ab209775; 1:1000; Abcam), and rabbit anti-GFAP antibody (Ab68428; 1:500; Abcam). The secondary antibodies used were Goat Anti-Mouse IgG H&L (HRP polymer) (Ab214879; pre-diluted; Abcam) and Goat Anti-Rabbit IgG H&L (HRP polymer) (Ab214880; pre-diluted; Abcam). Additional tissue sections were also processed for standard hematoxylin and eosin (H&E) staining.
Cell viability and cytotoxicity assays
For the cell viability assays, 1× 104 cells/well were seeded onto 96-well plates. A CCK-8 assay (Dojindo Laboratories) was used to assess cell viability. The macrophage-mediated cytotoxicities of anti-CD47 antibody to GL261 cells and GSCs were measured using a 6 h lactate dehydrogenase (LDH) release assay using a CytoTox 96 Non-Radioactive Assay Kit (Promega), following the manufacturer's instructions. All of these experiments were independently repeated three times.
Statistical analysis
All data are presented as means ± standard deviation (SD). Statistical analysis was performed using SPSS 20.0 (IBM) and GraphPad7.0. A threshold of p<0.05 was used to assess statistical significance (#p < 0.05, ##p < 0.01, ###p < 0.001).
2017ONCOIMM0638R-f02-z-4c.pptx
Download MS Power Point (1.7 MB)Disclosure statement
The authors have declared no conflicts of interest.
Funding
This study was supported by grants from the Natural Science Funds of China (Grant No.81171179 and 81272439), Funds for Key Projects of Guangdong Science and Technology (Grant No. 2016B030230004 and S2013020012754) and the Fund of Guangdong Educational Commission (Grant No.2013CXZDA008), Key Projects of Health Collaborative Innovation of Guangzhou (Grant No.201400000003-2) to Professor Xiaodan Jiang; also part of Fund from the Guangdong Provincial Clinical Medical Centre for Neurosurgery (Grant No.2013B020400005).
References
- Louis DN, Ohgaki H, Wiestler OD, Cavenee WK, Burger PC, Jouvet A, Scheithauer BW, Kleihues P. The 2007 WHO classification of tumours of the central nervous system. Acta Neuropathol. 2007;114:97–109. doi:10.1007/s00401-007-0243-4. PMID:17618441.
- Brown T J, Brennan MC, Li M, Church EW, Brandmeir NJ, Rakszawski KL, Patel AS, Rizk EB, Suki D, Sawaya R et al. Association of the Extent of Resection With Survival in Glioblastoma: A Systematic Review and Meta-analysis. JAMA Oncol. 2016;2:1460–69. doi:10.1001/jamaoncol.2016.1373. PMID:27310651.
- Li C, Heidt DG, Dalerba P, Burant CF, Zhang L, Adsay V, Wicha M, Clarke MF, Simeone DM. Identification of pancreatic cancer stem cells. Cancer Res. 2007;67:1030–37. doi:10.1158/0008-5472.CAN-06-2030. PMID:17283135.
- Raj D, Aicher A, Heeschen C. Concise Review: Stem Cells in Pancreatic Cancer: From Concept to Translation. Stem Cells. 2015;33:2893–902. doi:10.1002/stem.2114. PMID:26202953.
- O'Brien CA, Pollett A, Gallinger S, Dick JE. A human colon cancer cell capable of initiating tumour growth in immunodeficient mice. Nature. 2007;445:106–10. doi:10.1038/nature05372. PMID:17122772.
- Schatton T, Murphy GF, Frank NY, Yamaura K, Waaga-Gasser AM, Gasser M, Zhan Q, Jordan S, Duncan LM, Weishaupt C, et al. Identification of cells initiating human melanomas. Nature. 2008;451:345–49. doi:10.1038/nature06489. PMID:18202660.
- Singh SK, Clarke ID, Terasaki M, Bonn VE, Hawkins C, Squire J, Dirks PB. Identification of a cancer stem cell in human brain tumors. Cancer Res. 2003;63:5821–28;PMID:14522905.
- Guadagno E, Borrelli G, Califano M, Cali G, Solari D, Del B D CM. Immunohistochemical expression of stem cell markers CD44 and nestin in glioblastomas: Evaluation of their prognostic significance. Pathol Res Pract. 2016;212:825–32. doi:10.1016/j.prp.2016.07.002. PMID:27450656.
- Li Y, Wang H, Sun T, Chen J, Guo L, Shen H, Du Z, Zhou Y. Biological characteristics of a new human glioma cell line transformed into A2B5(+) stem cells. Mol Cancer. 2015;14:75. doi:10.1186/s12943-015-0343-z. PMID:25879429.
- Brown EJ, Frazier WA. Integrin-associated protein (CD47) and its ligands. Trends Cell Biol. 2001;11:130–35. doi:10.1016/S0962-8924(00)01906-1. PMID:11306274.
- Oldenborg PA, Zheleznyak A, Fang YF, Lagenaur CF, Gresham HD, Lindberg FP. Role of CD47 as a marker of self on red blood cells. Science. 2000;288:2051–54. doi:10.1126/science.288.5473.2051. PMID:10856220.
- Oldenborg PA, Gresham HD, Lindberg FP. CD47-signal regulatory protein alpha (SIRPalpha) regulates Fcgamma and complement receptor-mediated phagocytosis. J Exp Med. 2001;193:855–62;PMID:11283158;https://doi.org/10.1084/jem.193.7.855 doi:10.1084/jem.193.7.855. PMID:11283158.
- Jaiswal S, Jamieson C H, Pang W W, Park C Y, Chao M P, Majeti R, Traver D, van Rooijen N, Weissman I L. CD47 is upregulated on circulating hematopoietic stem cells and leukemia cells to avoid phagocytosis. Cell. 2009;138:271–85. doi:10.1016/j.cell.2009.05.046. PMID:19632178.
- Willingham SB, Volkmer JP, Gentles AJ, Sahoo D, Dalerba P, Mitra SS, Wang J, Contreras-Trujillo H, Martin R, Cohen JD et al. The CD47-signal regulatory protein alpha (SIRPα) interaction is a therapeutic target for human solid tumors. Proc Natl Acad Sci USA. 2012;109:6662–67. doi:10.1073/pnas.1121623109. PMID:22451913.
- Cioffi M, Trabulo S, Hidalgo M, Costello E, Greenhalf W, Erkan M, Kleeff J, Sainz BJ, Heeschen C. Inhibition of CD47 Effectively Targets Pancreatic Cancer Stem Cells via Dual Mechanisms. Clin Cancer Res. 2015;21:2325–37. doi:10.1158/1078-0432.CCR-14-1399. PMID:25717063.
- Liu L, Zhang L, Yang L, Li H, Li R, Yu J, Yang L, Wei F, Yan C, Sun Q et al. Anti-CD47 Antibody As a Targeted Therapeutic Agent for Human Lung Cancer and Cancer Stem Cells. Front Immunol. 2017;8:404. doi:10.3389/fimmu.2017.00404. PMID:28484448.
- Chao MP, Alizadeh AA, Tang C, Myklebust JH, Varghese B, Gill S, Jan M, Cha AC, Chan CK, Tan BT, et al. Anti-CD47 antibody synergizes with rituximab to promote phagocytosis and eradicate non-Hodgkin lymphoma. Cell. 2010;142:699–713. doi:10.1016/j.cell.2010.07.044. PMID:20813259.
- Chao MP, Jaiswal S, Weissman-Tsukamoto R, Alizadeh AA, Gentles AJ, Volkmer J, Weiskopf K, Willingham SB, Raveh T, Park CY, et al. Calreticulin is the dominant pro-phagocytic signal on multiple human cancers and is counterbalanced by CD47. Sci Transl Med. 2010;2:63r–94r. doi:10.1126/scitranslmed.3001375. PMID:21178137.
- Chao MP, Tang C, Pachynski RK, Chin R, Majeti R, Weissman IL. Extranodal dissemination of non-Hodgkin lymphoma requires CD47 and is inhibited by anti-CD47 antibody therapy. Blood. 2011;118:4890–901. doi:10.1182/blood-2011-02-338020. PMID:21828138.
- Chao MP, Alizadeh AA, Tang C, Jan M, Weissman-Tsukamoto R, Zhao F, Park CY, Weissman IL, Majeti R. Therapeutic antibody targeting of CD47 eliminates human acute lymphoblastic leukemia. Cancer Res. 2011;71:1374–84. doi:10.1158/0008-5472.CAN-10-2238. PMID:21177380.
- Chan K S, Espinosa I, Chao M, Wong D, Ailles L, Diehn M, Gill H, Presti J J, Chang H Y, van de Rijn M, et al. Identification, molecular characterization, clinical prognosis, and therapeutic targeting of human bladder tumor-initiating cells. Proc Natl Acad Sci U S A. 2009;106:14016–21. doi:10.1073/pnas.0906549106. PMID:19666525.
- Lee TK, Cheung VC, Lu P, Lau EY, Ma S, Tang KH, Tong M, Lo J, Ng IO. Blockade of CD47-mediated cathepsin S/protease-activated receptor 2 signaling provides a therapeutic target for hepatocellular carcinoma. Hepatology. 2014;60:179–91;PMID:24523067;https://doi.org/10.1002/hep.27070 doi:10.1002/hep.27070. PMID:24523067.
- Xie Q, Flavahan WA, Bao S, Rich J. The tailless root of glioma: cancer stem cells. Cell Stem Cell. 2014;15:114–16. doi:10.1016/j.stem.2014.07.004. PMID:25105574.
- Gholamin S, Mitra SS, Feroze AH, Liu J, Kahn SA, Zhang M, Esparza R, Richard C, Ramaswamy V, Remke M, et al. Disrupting the CD47-SIRPalpha anti-phagocytic axis by a humanized anti-CD47 antibody is an efficacious treatment for malignant pediatric brain tumors. Sci Transl Med. 2017;9(381). doi:10.1126/scitranslmed.aaf2968. PMID:28298418
- Mccracken MN, Cha AC, Weissman IL. Molecular Pathways: Activating T Cells after Cancer Cell Phagocytosis from Blockade of CD47 “Don't Eat Me” Signals. Clin Cancer Res. 2015;21:3597–601. doi:10.1158/1078-0432.CCR-14-2520. PMID:26116271.
- Liu X, Pu Y, Cron K, Deng L, Kline J, Frazier WA, Xu H, Peng H, Fu YX, Xu MM. CD47 blockade triggers T cell-mediated destruction of immunogenic tumors. Nat Med. 2015;21:1209–15. doi:10.1038/nm.3931. PMID:26322579.
- Huang W, Lv B, Zeng H, Shi D, Liu Y, Chen F, Li F, Liu X, Zhu R, Yu L et al. Paracrine Factors Secreted by MSCs Promote Astrocyte Survival Associated With GFAP Downregulation After Ischemic Stroke via p38 MAPK and JNK. J Cell Physiol. 2015;230:2461–75. doi:10.1002/jcp.24981. PMID:25752945.
- Livak KJ, Schmittgen TD. Analysis of relative gene expression data using real-time quantitative PCR and the 2(-Delta Delta C(T)) Method. Methods. 2001;25:402–08. doi:10.1006/meth.2001.1262. PMID:11846609.
- Maes W, Rosas GG, Verbinnen B, Boon L, De Vleeschouwer S, Ceuppens JL, Van Gool SW. DC vaccination with anti-CD25 treatment leads to long-term immunity against experimental glioma. Neuro Oncol. 2009;11:529–42. doi:10.1215/15228517-2009-004. PMID:19336528.