ABSTRACT
The relationship between anti-tumoral immunity and cancer progression is complex. Recently, immune editing has emerged as a model to explain the interplay between the immune system and the selection of genetic alterations in cancer. In this model, the immune system selects cancer cells that grow as these are fit to escape immune surveillance during tumor development. Gliomas and glioblastoma, the most aggressive and most common of all primary malignant brain tumors are genetically heterogeneous, are relatively less antigenic, and are less responsive to immunotherapy than other cancers. In this review, we provide an overview of the relationship between glioma´s immune suppressive features, anti-tumoral immunity and cancer genomics. In this context, we provide a critical discussion of evidence suggestive of immune editing in this disease and discuss possible alternative explanations for these findings.
Introduction
Burgeoning work of next-generation sequencing analysis has provided the framework to visualize the genomic landscape of cancer, these studies have revealed a remarkable genetic heterogeneity within lower-grade gliomas (LGG) and glioblastomas (GBM).Citation1-Citation3 Gliomas elicit a strong immunosuppressive state through the expression of inhibitory ligands that induce anergy and apoptosis of cytotoxic lymphocytes, expression of immune checkpoints that impair the anti-tumoral immune response, and through eliciting tumoral infiltration by immunosuppressive cells.Citation4-Citation7 There is also evidence of immune-cell infiltration following immunotherapy in human and murine gliomas,Citation8,Citation9 in which bone marrow-derived dendritic cells play a role.Citation9 Despite these findings, immunotherapy for gliomas has not shown a significant efficacy in the clinical setting.Citation10-Citation12 These facts rise the possibility that the dominance of tumor clones that evade immune recognition could be related to the emergence and selection of specific genetic alterations that confer cancer cells this feature during tumor progression. However, the possibility of immune editing during the progression of gliomas has not been explored. Herein we provide an overview of immune editing and some illustrative examples that suggest that this might be happening in gliomas.
The cancer immune editing concept
The first hints of immunity against cancer were given by Paul EhrlichCitation13 and later supported by Burnet and Thomas.Citation14-Citation16 However, in light of some studies that introduced doubts about the paradigm of immune control of cancer,Citation17-Citation19 the attention of cancer immune surveillance gained broader recognition again more recently by experiments where mice devoid of T, B and NK cells or immune-regulatory cytokines were more susceptible to tumor formation than immunocompetent mice.Citation20-Citation24 Particularly, the reviving of interest on tumor immunology arose from studies showing that cancer cells can escape immune recognition and destruction,Citation20,Citation25,Citation26 framing this mechanism as one of the hallmarks of cancer in the latest iteration of a comprehensive review of cancer biology by Hanahan and Weinberg in recent years.Citation27 The growing recognition of the immunological control of neoplasia prompted a more integrative concept of tumor-immune system interaction called cancer immune editing in which cancer immune-related phenotype is shaped by selection of tumor cells capable of avoiding recognition and killing by the immune system. Cancer immune editing has been conceptualized into three phases: elimination, a process in which the immune system recognizes and eradicates developing cancer cells before the latter can reach a clinical stage; equilibrium, a state of tumor latency in which tumor cells remain occult or sub-clinical, are not completely eradicated but do not increase in number; and escape, a phase in which tumor cells capable of evading immune recognition and eradication (i.e. edited cells) grow progressively into a clinical stage of cancer.Citation28,Citation29 When this process is carried out in its entirety, tumor cell variants with a less immunogenic phenotype emerge resulting in cancer that cannot be eradicated by the immune system.Citation30,Citation31 Comprehensive reviews have described the details of the evidence suggestive of the process of cancer immune editing.Citation30-Citation32
Immune editing in Gliomas: Elimination, equilibrium, and escape
Elimination
Clinical observations have provided the inference that the immune editing phases might be occurring in cancer patients. Particularly, the observation that immune suppressed patients with AIDS or transplant recipients receiving immunosuppressive therapies are more prone to some cancer types suggests the presence of the elimination phase in the immune competent population.Citation33-Citation35 The innate and adaptive immune system eliminate an emergent cancer through several processes: some of the best characterized mechanisms include the tumoral expression of NKG2D ligands that are recognized by NK cells, the recognition of tumor-specific antigens by CD4+ and CD8+ T-cells, the production of pro-inflammatory and immunomodulatory cytokines that enhance immune anti-tumoral responses, and the induction of tumor cell apoptosis by immune effector cells.Citation28,Citation30,Citation31
A key question within the study of relationship between the immune system and the cancer genome is whether immune cells can recognize genetic alterations in the tumor. Recent studies have suggested this to be the case. For instance, the proliferation of hyperploid cancer cells alerts the immune system of developing cancer through the recognition of the abnormally exposed calreticulin on the cell surface, which renders polyploid cancer cells as targets of cytotoxic T-cells.Citation36 However, a recent analysis of TCGA data from 12 human cancer types showed that high levels of tumor aneuploidy is associated with decreased expression of genes encoding for markers of infiltrating cytotoxic immune cells, genes encoding for components of the T and B-cell receptor, genes mediating cytotoxic activities, genes related to the IFN-γ pathway, as well as genes associated with the maintenance of the immune response and the production of cytokines.Citation37 Moreover, a higher number of neoantigens resulting from non-silent mutations were found in highly aneuploid tumors compared to less aneuploid tumors, indicating that the former did not undergo immune editing during progression due to a reduced anti-tumoral imunity.Citation37 Highly aneuploid GBM and LGG did not reveal any decrease in the expression of genes involved in immune cytotoxic activities.Citation37 In any case, according to the immune editing model, if a particular cancer cell is not initially eliminated due to the fact that is not antigenic or given that it has acquired the ability to suppress anti-tumor immunity, it will prevail into the equilibrium phase.Citation28,Citation30
No studies provide any evidence to address whether the elimination phase is occurring in gliomas, that is, evidence of gliomas disappearing at a pre-clinical stage. Regardless of some reports suggesting a higher incidence of gliomas in immunosuppressed individuals,Citation38,Citation39 in general, gliomas do not arise from immunosuppressed states as opposed to other cancers like non-Hodgkin lymphoma or Kaposi´s sarcoma.Citation34 Direct demonstration of the elimination phase in patients is particularly difficult as it would require evidence of tumor-infiltrating immune cells on preclinical stages of gliomas followed by the spontaneous disappearance of the tumors. To prove this, transgenic mouse gliomas have emerged as novel tools that can model the acquisition of many of the genetic alterations seen in human gliomasCitation40-Citation42 and offer an opportunity to investigate whether gliomas at early stages of formation undergo infiltration by immune cells, a pre-requisite for the existence of the elimination phase. One of these studies analyzed early immune responses at occult stages of glioma development in a transgenic mouse glioma model of spontaneous cancer (GFAP-V12HA-ras).Citation41 To induce the development of astrocytomas, the glial fibrillary acidic protein (GFAP) promoter was used to express the V12HA-ras specifically in astrocytes. At 12 weeks, asymptomatic mice had infiltration of CD4+ and CD8+ T-cells in the tumor, suggesting that the immune system can detect a developing glioma at early stages. However, no evidence of spontaneous disappearance of these tumors was reported.Citation41 Whereas it is difficult to rule out elimination of gliomas by the immune system, there is no substantial evidence to suggest this phenomenon is taking place.
Equilibrium
The equilibrium phase was inferred from reports of transplant patients who developed metastases in a transplanted organ that was donated by a cancer patient.Citation43-Citation45 Particularly, there have been reports of transplant recipients diagnosed with glioma metastases on the grafted organs after receiving liver, kidney or lung transplants from GBM donor patients, suggesting the possibility of an equilibrium phase in these tumors, at least with respect to systemic grafting of lesions.Citation39,Citation46-Citation48 In general, gliomas do not metastasize outside the central nervous system in spite of the fact that tumor cells have been found circulating in the blood stream.Citation49 In the context of these organ transplant case reports,Citation39,Citation46-Citation48 it appears that glioma cells in the donor patient may have been in a dormant state due to the host immune pressure, but after transplantation, these lesions grew in the organ that was transplanted into a host undergoing pharmacological immunosuppression. Another example of evidence suggestive of the existence of an equilibrium phase in brain tumors is that of a pediatric patient who was diagnosed with medulloblastoma at 17 months of age, who 10 years later was treated with immunosuppressive drugs for a lung transplant due to the development of chemotherapy-induced pulmonary fibrosis. 12 months after transplantation, liver metastases were detected revealing recurrence of the medulloblastoma in the histopathological report.Citation50
Mouse models have suggested that adaptive immunity and T-cells in particular are responsible for controlling tumor growth during the equilibrium phase.Citation51,Citation52 As opposed to NK cells, depletion of IFN-γ, CD4+ and CD8+ T-cells in mice treated with methyl-colantrene promoted the development of sarcomas.Citation51 In addition, depletion of CD4+ and/or CD8+ T-cells led to spontaneous lung metastases in a fibrosarcoma mouse model that do not develop these metastases in an immune competent setting.Citation52 These metastases were highly positive for MHC class I (MHC-I),Citation52 indicating the importance of the interaction between MHC-I molecules and T-cell receptors in tumor dormancy/equilibrium maintenance.
Escape
In the escape phase, the immune system fails to limit tumor growth, and tumor cells capable of evading the immune recognition and elimination prevail into a clinically evident state.Citation28,Citation30-Citation32 The interaction between cancer and the immune system gives rise to a new group of tumor cells displaying reduced immunogenicity, and tumors that elicit an immunosuppressive microenvironment that endows them to survive and proliferate in the immunocompetent host.Citation28,Citation53,Citation54 Taking into account that the correct functionality of the MHC-I complex is required for an effective anti-tumoral immune response,Citation55 immune escape capabilities have been well associated with defects on MHC-I expression on tumor cells.Citation53,Citation56 Relative reduction in expression of MHC-I by tumor cells can cause immune selection and outgrowth of a tumor clone resistant to immunotherapies.Citation57,Citation58 Furthermore, recent evidence suggests a positive selection of loss-of-function mutations of HLA class I genes compared with mutations in non-HLA class I genes in a pan-cancer analysis.Citation59 This analysis showed few HLA class I mutations in human gliomas compared to other cancers. Nevertheless, an association between HLA class I mutations and upregulation of killer lymphocyte effector gene expression was found in several cancer types, including gliomas ().Citation59,Citation60 This finding suggests that immune pressure exerted by lymphocyte cytolytic activity selects glioma cells harboring loss-of-function HLA class I mutations.Citation59,Citation60 Moreover, the decreased expression of HLA class I and the antigen processing and presentation machinery (APM) components correlates with malignancy grade in human and murine GBM.Citation61,Citation62 The reduction of HLA class I and II gene expression could be explained by the EGFR activation.Citation63 Indeed, inhibitory signals originated from the activation of EGFR have been proposed to affect the expression of the IFN-γ receptor complex, as well as to act on the promoter of HLA class I and II genes and MHC class II transactivator (CIITA) gene, a transcriptional activator of HLA class I and II genes.Citation63 This mechanism might explain the relative lymphocytic depletion of GBM of the classical subtype, which characteristically have EGFR amplification.Citation64 On the other hand, the sole expression of HLA class I molecules without loaded antigen due to dysfunctional APM has been described in astrocytomas as another potential escape mechanism that inhibits the anti-tumoral response of NK cells.Citation65 Given the relative uncommon frequency of HLA class I gene mutations in human gliomas in spite of the implications of these genes in tumor recognition by the immune system, we investigated the aggregate frequency of down-regulation, non-silent mutation or deletion of HLA class I and related APM genes across gliomas from TCGA. As a group, these genes are found affected by these inactivating alterations in 53.7% of GBM (n = 396) and 32.4% of LGG (n = 511), suggesting that gliomas commonly suffer hits of this pathway that is critical for immune recognition (). The evidence presented above indicates and further substantiates the idea of immune selection of glioma cell populations with HLA class I molecules presentation defects.
Figure 1. Heatmap displaying a differential gene expression in a color scale in HLA class I-mutant gliomas vs. wild-type HLA class I gliomas. Tumors harboring HLA class I mutations overexpress lymphocyte killer effector genes. As previously defined, “Cytolytic activity” is included as a single gene and determined by Granzyme A (GZMA) and Perforin (PRF1), two cytolytic genes expressed in activated CD8+ T-cells.Citation60 *P < 0.05 represents the significance of the association between the expression of each gene and HLA class I mutant-gliomas. [Adapted by permission from Springer Nature: Nature Biotechnology, Comprehensive analysis of cancer-associated somatic mutations in class I HLA genes, Shukla et al., copyright 2015]Citation59.
![Figure 1. Heatmap displaying a differential gene expression in a color scale in HLA class I-mutant gliomas vs. wild-type HLA class I gliomas. Tumors harboring HLA class I mutations overexpress lymphocyte killer effector genes. As previously defined, “Cytolytic activity” is included as a single gene and determined by Granzyme A (GZMA) and Perforin (PRF1), two cytolytic genes expressed in activated CD8+ T-cells.Citation60 *P < 0.05 represents the significance of the association between the expression of each gene and HLA class I mutant-gliomas. [Adapted by permission from Springer Nature: Nature Biotechnology, Comprehensive analysis of cancer-associated somatic mutations in class I HLA genes, Shukla et al., copyright 2015]Citation59.](/cms/asset/2de7f07e-d6a3-4342-85f3-3691c7537d56/koni_a_1445458_f0001_oc.jpg)
Figure 2b. Cumulative HLA class I and APM defects across gliomas from TCGA. (A) 213/396 GBM and (B) 166/511 LGG samples were found to have alterations in the formation of the HLA class I peptide complex that enable glioma cells to escape immune recognition by CD8+ T-cells. (C) Highlighted in red are the components showing different types of alterations in antigen processing and presentation ranging from defects in the IFN-γ pathway (JAK1, JAK2, STAT1) and the HLA class I enhanceosome (NLRC5, CIITA, IRF1, RFX5, RFXANK, RFXAP, X2BP, NF-Y) that mediate the transcriptional activation of HLA class I genes; PSME1, PSME2, PSME3 genes coding for the alpha, beta, and gamma subunits that make up the proteasome activator complex PA28 for peptide generation; ERAP1 and ERAP2 that trim longer precursor of antigenic peptides; HSPA and HSPC that chaperones the peptides to their loading on the MHC class I in the endoplasmic reticulum; and TAP1, TAP2, TAPBP, calreticulin, calnexin, PDIA3, β2-microglobulin and HLA class I genes that participate in the formation of the MHC class I peptide complex.
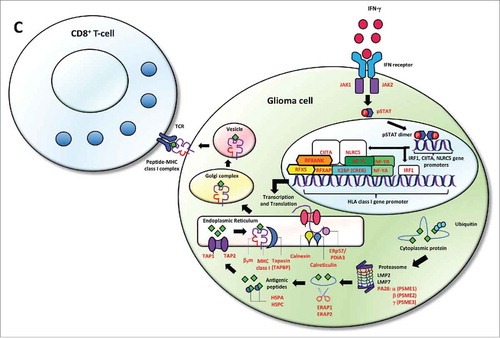
Cancer immune editing implications in glioma therapy
Immune checkpoint inhibitors have shown clinically relevant efficacy for some cancers such as melanoma and lung cancer, and have emerged as an innovative therapeutic approach within the field of oncology.Citation66-Citation69 Particularly, tumors with high mutational load and mismatch repair (MMR) defects have been shown to vary among cancer types and correlate with good clinical responses to immune checkpoint inhibitors.Citation70-Citation73 In this context, GBM patients with germline MMR defects and the accompanying hypermutator phenotype have shown striking and long-term responses to the PD1 inhibitor nivolumab.Citation74 Temozolomide, the chemotherapeutic standard of care of GBM, can generate mutations in the tumor that can lead to a hypermutator phenotype following treatment with this chemotherapy in 17% of cases.Citation75-Citation78 Whereas high tumor mutational load and loss of MMR protein expression are infrequent,Citation79,Citation80 the possibility of this phenotype/genotype being more responsive to immunotherapy rises interest for personalizing the use of this treatment modality for a subset of glioma patients.
Similar to the T-cell-dependent selection of tumor cells lacking highly immunogenic antigens during tumor progression,Citation81,Citation82 the major implication of immune editing for glioma therapy is that immunotherapies designed to target tumor-specific antigens often fail, as there are tumor clones that will be selected for the lack of expression of these antigens or defects in antigen presentation. In a phase II clinical trial evaluating EGFRvIII-targeted vaccination in glioma patients, it was found that EGFRvIII expression was lost at tumor recurrence.Citation10,Citation83 Additionally, recent reports testing chimeric antigen receptor (CAR)-engineered T-cells targeting the cancer-associated antigens interleukin-13 receptor alpha 2 (IL13Rα2) and EGFRvIII have shown promising results in GBM patients with recurrent disease.Citation8,Citation84 Although regression of multifocal GBM was achieved in one particular patient, recurrent lesions at new brain sites emerged with minimal to absent expression of IL13Rα2.Citation84 In the study evaluating CAR T-EGFRvIII, 5 of 7 GBM patients whom tumor was available for evaluation after CAR T-cells infusion had a decrease in the expression of EGFRvIII.Citation8 Thus, recurrent tumor lesions lacking glioma-specific antigens suggest the possibility that antigen loss variants were selected to grow during immunotherapy. Because of this mechanism of immune editing and the intrinsic molecular heterogeneity of gliomas, different strategies for immunotherapy should be used in order to target multiple antigens to mitigate antigen escape and avoid tumor recurrence.Citation85-Citation87
If immune editing is occurring during glioma progression, perhaps transient manipulation of the selection pressure elicited by the immune system might lead to more immunogenic tumors that might be more susceptible to immunotherapies. A scheme summarizing the existing evidence of immune editing in gliomas is shown ().
Figure 3. Experimental or clinical evidence regarding immune editing in gliomas with numbers representing the references related to this evidence. No evidence exists about the elimination phase in gliomas. Commonly, gliomas do not spread outside the central nervous system. However, glioma metastases have been detected in transplanted organs of immunosuppressed transplant recipients, suggesting that the equilibrium phase is taking place in the systemic compartmentCitation39,Citation46-Citation48 Associations between loss-of-function HLA class I mutations and upregulation of lymphocyte killer effector genes,Citation59 as well as HLA class I and APM defects have been found in gliomas, evidencing the escape phase. In addition, loss of glioma-specific antigens after using targeted immunotherapies lead to a negative selection of tumor variants cells expressing these antigens.Citation8,Citation10,Citation83,Citation84
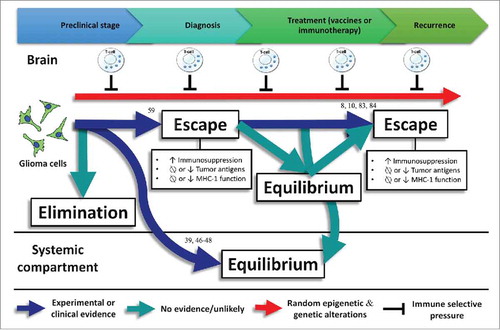
Conclusions
Gliomas are notorious for the multiple mechanisms of immune suppression and lack of response to immunotherapy compared to other cancers. Gliomas are molecularly complex and have several genetic and epigenetic alterations that are recurrently seen during tumor progression. Whereas some of these offer a tumor growth advantage, others might have been selected by the anti-tumoral immunity. This is an interesting possibility that has not been systematically investigated in gliomas. Hopefully, further research addressing the possibility of immune editing in gliomas will lead to manipulation of this process and more efficient immunotherapy strategies transforming the way these patients are treated.
Disclosure of potential conflicts of interest
No potential conflicts of interest were disclosed.
Acknowledgment and funding support
This work was supported by the ¨Matthew Larson Foundation Award for Research on Pediatric Brain Tumors¨ and by NIH research grants OD DP5OD021356-03 (A.S.) and U54 1U54CA193313 (R.R. and J.Z.)
References
- Brat DJ, Verhaak RG, Aldape KD, Yung WK, Salama SR, Cooper LA, Rheinbay E, Miller CR, Vitucci M, Morozova O, et al. Comprehensive, Integrative Genomic Analysis of Diffuse Lower-Grade Gliomas. N Engl J Med. 2015;372(26):2481–98. doi:10.1056/NEJMoa1402121. PMID:26061751.
- Suzuki H, Aoki K, Chiba K, Sato Y, Shiozawa Y, Shiraishi Y, Shimamura T, Niida A, Motomura K, Ohka F, et al. Mutational landscape and clonal architecture in grade II and III gliomas. Nat Genet. 2015;47(5):458–68. doi:10.1038/ng.3273. PMID:25848751.
- Brennan CW, Verhaak RG, McKenna A, Campos B, Noushmehr H, Salama SR, Zheng S, Chakravarty D, Sanborn JZ, Berman SH, et al. The somatic genomic landscape of glioblastoma. Cell. 2013;155(2):462–77. doi:10.1016/j.cell.2013.09.034. PMID:24120142.
- Wintterle S, Schreiner B, Mitsdoerffer M, Schneider D, Chen L, Meyermann R, Weller M, Wiendl H. Expression of the B7-related molecule B7-H1 by glioma cells: a potential mechanism of immune paralysis. Cancer Res. 2003;63(21):7462–7. PMID:14612546.
- Jacobs JF, Idema AJ, Bol KF, Nierkens S, Grauer OM, Wesseling P, Grotenhuis JA, Hoogerbrugge PM, de Vries IJ, Adema GJ. Regulatory T cells and the PD-L1/PD-1 pathway mediate immune suppression in malignant human brain tumors. Neuro Oncol. 2009;11(4):394–402. doi:10.1215/15228517-2008-104. PMID:19028999.
- Chang AL, Miska J, Wainwright DA, Dey M, Rivetta CV, Yu D, Kanojia D, Pituch KC, Qiao J, Pytel P, et al. CCL2 Produced by the Glioma Microenvironment Is Essential for the Recruitment of Regulatory T Cells and Myeloid-Derived Suppressor Cells. Cancer Res. 2016;76(19):5671–82. doi:10.1158/0008-5472.CAN-16-0144. PMID:27530322.
- Hambardzumyan D, Gutmann DH, Kettenmann H. The role of microglia and macrophages in glioma maintenance and progression. Nat Neurosci. 2016;19(1):20–27. doi:10.1038/nn.4185. PMID:26713745.
- O'Rourke DM, Nasrallah MP, Desai A, Melenhorst JJ, Mansfield K, Morrissette JJD, Martinez-Lage M, Brem S, Maloney E, Shen A, et al. A single dose of peripherally infused EGFRvIII-directed CAR T cells mediates antigen loss and induces adaptive resistance in patients with recurrent glioblastoma. Sci Transl Med. 2017;9(399):eaaa0984. PMID:28724573.
- Curtin JF, Liu N, Candolfi M, Xiong W, Assi H, Yagiz K, Edwards MR, Michelsen KS, Kroeger KM, Liu C, et al. HMGB1 mediates endogenous TLR2 activation and brain tumor regression. PLoS Med. 2009;6(1):e10. doi:10.1371/journal.pmed.1000010. PMID:19143470.
- Weller M, Butowski N, Tran DD, Recht LD, Lim M, Hirte H, Ashby L, Mechtler L, Goldlust SA, Iwamoto F, et al. Rindopepimut with temozolomide for patients with newly diagnosed, EGFRvIII-expressing glioblastoma (ACT IV): a randomised, double-blind, international phase 3 trial. Lancet Oncol. 2017;18(10):1373–1385. Elsevier. doi:10.1016/S1470-2045(17)30517-X. PMID:28844499.
- Blumenthal DT, Yalon M, Vainer GW, Lossos A, Yust S, Tzach L, Cagnano E, Limon D, Bokstein F. Pembrolizumab: first experience with recurrent primary central nervous system (CNS) tumors. J Neurooncol. 2016;129(3):453–60. doi:10.1007/s11060-016-2190-1. PMID:27377654.
- Reardon DA, Omuro A, Brandes AA, Rieger J, Wick A, Sepulveda J, Phuphanich S, de Souza P, Ahluwalia MS, Lim M, et al. OS10.3 Randomized Phase 3 Study Evaluating the Efficacy and Safety of Nivolumab vs Bevacizumab in Patients With Recurrent Glioblastoma: CheckMate 143. Neuro-Oncology. 2017;19(3_suppl):iii21. https://doi.org/10.1093/neuonc/nox036.071.
- Ehrlich P. Über den jetzigen stand der karzinomforschung. 1909;5:273–90.
- Burnet FM. Immunological aspects of malignant disease. Lancet. 1967;1(7501):1171–4. doi:10.1016/S0140-6736(67)92837-1. PMID:4165129.
- Burnet FM. The concept of immunological surveillance. Prog Exp Tumor Res. 1970;13:1–27. doi:10.1159/000386035. PMID:4921480.
- Thomas L. On immunosurveillance in human cancer. Yale J Biol Med. 1982;55(3-4):329–33. PMID:6758376.
- Stutman O. Tumor development after 3-methylcholanthrene in immunologically deficient athymic-nude mice. Science. 1974;183(4124):534–6. doi:10.1126/science.183.4124.534. PMID:4588620.
- Stutman O. Chemical carcinogenesis in nude mice: comparison between nude mice from homozygous matings and heterozygous matings and effect of age and carcinogen dose. J Natl Cancer Inst. 1979;62(2):353–8. PMID:283266.
- Stutman O. Spontaneous tumors in nude mice: Effect of the viable yellow gene. Exp Cell Biol. 1979;47(2):129–35. PMID:446837.
- Shankaran V, Ikeda H, Bruce AT, White JM, Swanson PE, Old LJ, Schreiber RD. IFNgamma and lymphocytes prevent primary tumour development and shape tumour immunogenicity. Nature. 2001;410(6832):1107–11. doi:10.1038/35074122. PMID:11323675.
- Svane IM, Engel AM, Nielsen MB, Ljunggren HG, Rygaard J, Werdelin O. Chemically induced sarcomas from nude mice are more immunogenic than similar sarcomas from congenic normal mice. Eur J Immunol. 1996;26(8):1844–50. doi:10.1002/eji.1830260827. PMID:8765030.
- van den Broek ME, Kägi D, Ossendorp F, Toes R, Vamvakas S, Lutz WK, Melief CJ, Zinkernagel RM, Hengartner H. Decreased tumor surveillance in perforin-deficient mice. J Exp Med. 1996;184(5):1781–90. doi:10.1084/jem.184.5.1781. PMID:8920866.
- Street SE, Cretney E, Smyth MJ. Perforin and interferon-gamma activities independently control tumor initiation, growth, and metastasis. Blood. 2001;97(1):192–7. doi:10.1182/blood.V97.1.192. PMID:11133760.
- Kaplan DH, Shankaran V, Dighe AS, Stockert E, Aguet M, Old LJ, Schreiber RD. Demonstration of an interferon gamma-dependent tumor surveillance system in immunocompetent mice. Proc Natl Acad Sci U S A. 1998;95(13):7556–61. doi:10.1073/pnas.95.13.7556. PMID:9636188.
- Gao Y, Yang W, Pan M, Scully E, Girardi M, Augenlicht LH, Craft J, Yin Z. Gamma delta T cells provide an early source of interferon gamma in tumor immunity. J Exp Med. 2003;198(3):433–42. doi:10.1084/jem.20030584. PMID:12900519.
- Girardi M, Oppenheim DE, Steele CR, Lewis JM, Glusac E, Filler R, Hobby P, Sutton B, Tigelaar RE, Hayday AC. Regulation of cutaneous malignancy by gammadelta T cells. Science. 2001;294(5542):605–9. doi:10.1126/science.1063916. PMID:11567106.
- Hanahan D, Weinberg RA. Hallmarks of cancer: the next generation. Cell. 2011;144(5):646–74. doi:10.1016/j.cell.2011.02.013. PMID:21376230.
- Dunn GP, Bruce AT, Ikeda H, Old LJ, Schreiber RD. Cancer immunoediting: from immunosurveillance to tumor escape. Nat Immunol. 2002;3(11):991–8. doi:10.1038/ni1102-991. PMID:12407406.
- Dunn GP, Old LJ, Schreiber RD. The three Es of cancer immunoediting. Annu Rev Immunol. 2004;22:329–60. doi:10.1146/annurev.immunol.22.012703.104803. PMID:15032581.
- Schreiber RD, Old LJ, Smyth MJ. Cancer immunoediting: integrating immunity's roles in cancer suppression and promotion. Science. 2011;331(6024):1565–70. doi:10.1126/science.1203486. PMID:21436444.
- Mittal D, Gubin MM, Schreiber RD, Smyth MJ. New insights into cancer immunoediting and its three component phases–elimination, equilibrium and escape. Curr Opin Immunol. 2014;27:16–25. doi:10.1016/j.coi.2014.01.004. PMID:24531241.
- Dunn GP, Old LJ, Schreiber RD. The immunobiology of cancer immunosurveillance and immunoediting. Immunity. 2004;21(2):137–48. doi:10.1016/j.immuni.2004.07.017. PMID:15308095.
- Burgi A, Brodine S, Wegner S, Milazzo M, Wallace MR, Spooner K, Blazes DL, Agan BK, Armstrong A, Fraser S, et al. Incidence and risk factors for the occurrence of non-AIDS-defining cancers among human immunodeficiency virus-infected individuals. Cancer. 2005;104(7):1505–11. doi:10.1002/cncr.21334. PMID:16104038.
- Biggar RJ, Chaturvedi AK, Goedert JJ, Engels EA, Study HACM. AIDS-related cancer and severity of immunosuppression in persons with AIDS. J Natl Cancer Inst. 2007;99(12):962–72. doi:10.1093/jnci/djm010. PMID:17565153.
- Vajdic CM, McDonald SP, McCredie MR, van Leeuwen MT, Stewart JH, Law M, Chapman JR, Webster AC, Kaldor JM, Grulich AE. Cancer incidence before and after kidney transplantation. JAMA. 2006;296(23):2823–31. doi:10.1001/jama.296.23.2823. PMID:17179459.
- Senovilla L, Vitale I, Martins I, Tailler M, Pailleret C, Michaud M, Galluzzi L, Adjemian S, Kepp O, Niso-Santano M, et al. An immunosurveillance mechanism controls cancer cell ploidy. Science. 2012;337(6102):1678–84. doi:10.1126/science.1224922. PMID:23019653.
- Davoli T, Uno H, Wooten EC, Elledge SJ. Tumor aneuploidy correlates with markers of immune evasion and with reduced response to immunotherapy. Science. 2017;355(6322):eaaf8399. doi:10.1126/science.aaf8399. PMID:28104840.
- Blumenthal DT, Raizer JJ, Rosenblum MK, Bilsky MH, Hariharan S, Abrey LE. Primary intracranial neoplasms in patients with HIV. Neurology. 1999;52(8):1648–51. doi:10.1212/WNL.52.8.1648. PMID:10331693.
- Schiff D, O'Neill B, Wijdicks E, Antin JH, Wen PY. Gliomas arising in organ transplant recipients: an unrecognized complication of transplantation? Neurology. 2001;57(8):1486–8. doi:10.1212/WNL.57.8.1486. PMID:11673595.
- Lei L, Sonabend AM, Guarnieri P, Soderquist C, Ludwig T, Rosenfeld S, Bruce JN, Canoll P. Glioblastoma models reveal the connection between adult glial progenitors and the proneural phenotype. PLoS One. 2011;6(5):e20041. doi:10.1371/journal.pone.0020041. PMID:21625383.
- Tran Thang NN, Derouazi M, Philippin G, Arcidiaco S, Di Berardino-Besson W, Masson F, Hoepner S, Riccadonna C, Burkhardt K, Guha A, et al. Immune infiltration of spontaneous mouse astrocytomas is dominated by immunosuppressive cells from early stages of tumor development. Cancer Res. 2010;70(12):4829–39. doi:10.1158/0008-5472.CAN-09-3074. PMID:20501837.
- Sonabend AM, Bansal M, Guarnieri P, Lei L4 Amendolara B, Soderquist C, Leung R, Yun J, Kennedy B, Sisti J, et al. The transcriptional regulatory network of proneural glioma determines the genetic alterations selected during tumor progression. Cancer Res. 2014;74(5):1440–51. doi:10.1158/0008-5472.CAN-13-2150. PMID:24390738.
- MacKie RM, Reid R, Junor B. Fatal melanoma transferred in a donated kidney 16 years after melanoma surgery. N Engl J Med. 2003;348(6):567–8. doi:10.1056/NEJM200302063480620. PMID:12571271.
- Morath C, Rohmeiss P, Schwenger V, Waldherr R, Ritz E, Zeier M, Fallsehr C, Kloor M, von Knebel Doeberitz M, Andrassy J, et al. Transmission of donor-derived small-cell carcinoma cells by a nontumor-bearing allograft. Transplantation. 2005;80(4):540–2. doi:10.1097/01.tp.0000168489.71242.fd. PMID:16123733.
- Detry O, De Roover A, de Leval L, Herens C, Delwaide J, Honoré P, Meurisse M. Transmission of an undiagnosed sarcoma to recipients of kidney and liver grafts procured in a non-heart beating donor. Liver Transpl. 2005;11(6):696–9. doi:10.1002/lt.20457. PMID:15915495.
- Jonas S, Bechstein WO, Lemmens HP, Neuhaus R, Thalmann U, Neuhaus P. Liver graft-transmitted glioblastoma multiforme. A case report and experience with 13 multiorgan donors suffering from primary cerebral neoplasia. Transpl Int. 1996;9(4):426–9. doi:10.1111/j.1432-2277.1996.tb00903.x. PMID:8819282.
- Chen H, Shah AS, Girgis RE, Grossman SA. Transmission of glioblastoma multiforme after bilateral lung transplantation. J Clin Oncol. 2008;26(19):3284–5. doi:10.1200/JCO.2008.16.3543. PMID:18591565.
- Jimsheleishvili S, Alshareef AT, Papadimitriou K, Bregy A, Shah AH, Graham RM, Ferraro N, Komotar RJ. Extracranial glioblastoma in transplant recipients. J Cancer Res Clin Oncol. 2014;140(5):801–7. doi:10.1007/s00432-014-1625-3. PMID:24595597.
- Müller C, Holtschmidt J, Auer M, Heitzer E, Lamszus K, Schulte A, Matschke J, Langer-Freitag S, Gasch C, Stoupiec M, et al. Hematogenous dissemination of glioblastoma multiforme. Sci Transl Med. 2014;6(247):247ra101. doi:10.1126/scitranslmed.3009095. PMID:25080476.
- Kapoor S, Kurland G, Jakacki R. Recurrent medulloblastoma following pediatric double-lung transplant. J Heart Lung Transplant. 2000;19(10):1011–3. doi:10.1016/S1053-2498(00)00149-2. PMID:11044697.
- Koebel CM, Vermi W, Swann JB, Zerafa N, Rodig SJ, Old LJ, Smyth MJ, Schreiber RD. Adaptive immunity maintains occult cancer in an equilibrium state. Nature. 2007;450(7171):903–7. doi:10.1038/nature06309. PMID:18026089.
- Romero I, Garrido C, Algarra I, Collado A, Garrido F, Garcia-Lora AM. T lymphocytes restrain spontaneous metastases in permanent dormancy. Cancer Res. 2014;74(7):1958–68. doi:10.1158/0008-5472.CAN-13-2084. PMID:24531750.
- Khong HT, Restifo NP. Natural selection of tumor variants in the generation of “tumor escape” phenotypes. Nat Immunol. 2002;3(11):999–1005. doi:10.1038/ni1102-999. PMID:12407407.
- Takeda K, Nakayama M, Hayakawa Y, Kojima Y, Ikeda H, Imai N, Ogasawara K, Okumura K, Thomas DM, Smyth MJ, et al. IFN-γ is required for cytotoxic T cell-dependent cancer genome immunoediting. Nat Commun. 2017;8:14607. doi:10.1038/ncomms14607. PMID:28233863.
- Aptsiauri N, Cabrera T, Garcia-Lora A, Lopez-Nevot MA, Ruiz-Cabello F, Garrido F. MHC class I antigens and immune surveillance in transformed cells. Int Rev Cytol. 2007;256:139–89. doi:10.1016/S0074-7696(07)56005-5. PMID:17241907.
- Garcia-Lora A, Algarra I, Garrido F. MHC class I antigens, immune surveillance, and tumor immune escape. J Cell Physiol. 2003;195(3):346–55. doi:10.1002/jcp.10290. PMID:12704644.
- Carretero R, Romero JM, Ruiz-Cabello F, Maleno I, Rodriguez F, Camacho FM, Real LM, Garrido F, Cabrera T. Analysis of HLA class I expression in progressing and regressing metastatic melanoma lesions after immunotherapy. Immunogenetics. 2008;60(8):439–47. doi:10.1007/s00251-008-0303-5. PMID:18545995.
- del Campo AB, Kyte JA, Carretero J, Zinchencko S, Méndez R, González-Aseguinolaza G, Ruiz-Cabello F, Aamdal S, Gaudernack G, Garrido F, et al. Immune escape of cancer cells with beta2-microglobulin loss over the course of metastatic melanoma. Int J Cancer. 2014;134(1):102–13. doi:10.1002/ijc.28338. PMID:23784959.
- Shukla SA, Rooney MS, Rajasagi M, Tiao G, Dixon PM, Lawrence MS, Stevens J, Lane WJ, Dellagatta JL, Steelman S, et al. Comprehensive analysis of cancer-associated somatic mutations in class I HLA genes. Nat Biotechnol. 2015;33(11):1152–8. doi:10.1038/nbt.3344. PMID:26372948.
- Rooney MS, Shukla SA, Wu CJ, Getz G, Hacohen N. Molecular and genetic properties of tumors associated with local immune cytolytic activity. Cell. 2015;160(1-2):48–61. doi:10.1016/j.cell.2014.12.033. PMID:25594174.
- Facoetti A, Nano R, Zelini P, Morbini P, Benericetti E, Ceroni M, Campoli M, Ferrone S. Human leukocyte antigen and antigen processing machinery component defects in astrocytic tumors. Clin Cancer Res. 2005;11(23):8304–11. doi:10.1158/1078-0432.CCR-04-2588. PMID:16322289.
- Zagzag D, Salnikow K, Chiriboga L, Yee H, Lan L, Ali MA, Garcia R, Demaria S, Newcomb EW. Downregulation of major histocompatibility complex antigens in invading glioma cells: stealth invasion of the brain. Lab Invest. 2005;85(3):328–41. doi:10.1038/labinvest.3700233. PMID:15716863.
- Pollack BP, Sapkota B, Cartee TV. Epidermal growth factor receptor inhibition augments the expression of MHC class I and II genes. Clin Cancer Res. 2011;17(13):4400–13. doi:10.1158/1078-0432.CCR-10-3283. PMID:21586626.
- Rutledge WC, Kong J, Gao J, Gutman DA, Cooper LA, Appin C, Park Y, Scarpace L, Mikkelsen T, Cohen ML, et al. Tumor-infiltrating lymphocytes in glioblastoma are associated with specific genomic alterations and related to transcriptional class. Clin Cancer Res. 2013;19(18):4951–60. doi:10.1158/1078-0432.CCR-13-0551. PMID:23864165.
- Mehling M, Simon P, Mittelbronn M, Meyermann R, Ferrone S, Weller M, Wiendl H. WHO grade associated downregulation of MHC class I antigen-processing machinery components in human astrocytomas: does it reflect a potential immune escape mechanism? Acta Neuropathol. 2007;114(2):111–9. doi:10.1007/s00401-007-0231-8. PMID:17541610.
- Larkin J, Chiarion-Sileni V, Gonzalez R. Combined Nivolumab and Ipilimumab or Monotherapy in Untreated Melanoma. N Engl J Med. 2015;373(1):23–34. doi:10.1056/NEJMoa1504030. PMID:26027431.
- Motzer RJ, Rini BI, McDermott DF, Redman BG, Kuzel TM, Harrison MR, Vaishampayan UN, Drabkin HA, George S, Logan TF, et al. Nivolumab for Metastatic Renal Cell Carcinoma: Results of a Randomized Phase II Trial. J Clin Oncol. 2015;33(13):1430–7. doi:10.1200/JCO.2014.59.0703. PMID:25452452.
- Sharma P, Callahan MK, Bono P, Kim J, Spiliopoulou P, Calvo E, Pillai RN, Ott PA, de Braud F, Morse M, et al. Nivolumab monotherapy in recurrent metastatic urothelial carcinoma (CheckMate 032): a multicentre, open-label, two-stage, multi-arm, phase 1/2 trial. Lancet Oncol. 2016;17(11):1590–8. doi:10.1016/S1470-2045(16)30496-X. PMID:27733243.
- Antonia SJ, López-Martin JA, Bendell J, Ott PA, Taylor M, Eder JP, Jäger D, Pietanza MC, Le DT, de Braud F, et al. Nivolumab alone and nivolumab plus ipilimumab in recurrent small-cell lung cancer (CheckMate 032): a multicentre, open-label, phase 1/2 trial. Lancet Oncol. 2016;17(7):883–95. doi:10.1016/S1470-2045(16)30098-5. PMID:27269741.
- Snyder A, Makarov V, Merghoub T, Yuan J, Zaretsky JM, Desrichard A, Walsh LA, Postow MA, Wong P, Ho TS, et al. Genetic basis for clinical response to CTLA-4 blockade in melanoma. N Engl J Med. 2014;371(23):2189–99. doi:10.1056/NEJMoa1406498. PMID:25409260.
- Rizvi NA, Hellmann MD, Snyder A, Kvistborg P, Makarov V, Havel JJ, Lee W, Yuan J, Wong P, Ho TS, et al. Cancer immunology. Mutational landscape determines sensitivity to PD-1 blockade in non-small cell lung cancer. Science. 2015;348(6230):124–8. doi:10.1126/science.aaa1348. PMID:25765070.
- Rosenberg JE, Hoffman-Censits J, Powles T, van der Heijden MS, Balar AV, Necchi A, Dawson N, O'Donnell PH, Balmanoukian A, Loriot Y, et al. Atezolizumab in patients with locally advanced and metastatic urothelial carcinoma who have progressed following treatment with platinum-based chemotherapy: a single-arm, multicentre, phase 2 trial. Lancet. 2016;387(10031):1909–20. doi:10.1016/S0140-6736(16)00561-4. PMID:26952546.
- Le DT, Durham JN, Smith KN, Wang H, Bartlett BR, Aulakh LK, Lu S, Kemberling H, Wilt C, Luber BS, et al. Mismatch repair deficiency predicts response of solid tumors to PD-1 blockade. Science. 2017;357(6349):409–13. doi:10.1126/science.aan6733. PMID:28596308.
- Bouffet E, Larouche V, Campbell BB, Merico D, de Borja R, Aronson M, Durno C, Krueger J, Cabric V, Ramaswamy V, et al. Immune Checkpoint Inhibition for Hypermutant Glioblastoma Multiforme Resulting From Germline Biallelic Mismatch Repair Deficiency. J Clin Oncol. 2016;34(19):2206–11. doi:10.1200/JCO.2016.66.6552. PMID:27001570.
- Hunter C, Smith R, Cahill DP, Stephens P, Stevens C, Teague J, Greenman C, Edkins S, Bignell G, Davies H, et al. A hypermutation phenotype and somatic MSH6 mutations in recurrent human malignant gliomas after alkylator chemotherapy. Cancer Res. 2006;66(8):3987–91. doi:10.1158/0008-5472.CAN-06-0127. PMID:16618716.
- Cahill DP, Levine KK, Betensky RA, Codd PJ, Romany CA, Reavie LB, Batchelor TT, Futreal PA, Stratton MR, Curry WT, et al. Loss of the mismatch repair protein MSH6 in human glioblastomas is associated with tumor progression during temozolomide treatment. Clin Cancer Res. 2007;13(7):2038–45. doi:10.1158/1078-0432.CCR-06-2149. PMID:17404084.
- Yip S, Miao J, Cahill DP, Iafrate AJ, Aldape K, Nutt CL, Louis DN. MSH6 mutations arise in glioblastomas during temozolomide therapy and mediate temozolomide resistance. Clin Cancer Res. 2009;15(14):4622–9. doi:10.1158/1078-0432.CCR-08-3012. PMID:19584161.
- Wang J, Cazzato E, Ladewig E, Frattini V, Rosenbloom DI, Zairis S, Abate F, Liu Z, Elliott O, Shin YJ, et al. Clonal evolution of glioblastoma under therapy. Nat Genet. 2016;48(7):768–76. doi:10.1038/ng.3590. PMID:27270107.
- Lawrence MS, Stojanov P, Polak P, Kryukov GV, Cibulskis K, Sivachenko A, Carter SL, Stewart C, Mermel CH, Roberts SA, et al. Mutational heterogeneity in cancer and the search for new cancer-associated genes. Nature. 2013;499(7457):214–8. doi:10.1038/nature12213. PMID:23770567.
- Hodges TR, Ott M, Xiu J, Gatalica Z, Swensen J, Zhou S, Huse JT, de Groot J, Li S, Overwijk WW, et al. Mutational burden, immune checkpoint expression, and mismatch repair in glioma: implications for immune checkpoint immunotherapy. Neuro Oncol. 2017;19(8):1047–1057. Oxford University Press. doi:10.1093/neuonc/nox026. PMID:28371827.
- Matsushita H, Vesely MD, Koboldt DC, Rickert CG, Uppaluri R, Magrini VJ, Arthur CD, White JM, Chen YS, Shea LK, et al. Cancer exome analysis reveals a T-cell-dependent mechanism of cancer immunoediting. Nature. 2012;482(7385):400–4. doi:10.1038/nature10755. PMID:22318521.
- DuPage M, Mazumdar C, Schmidt LM, Cheung AF, Jacks T. Expression of tumour-specific antigens underlies cancer immunoediting. Nature. 2012;482(7385):405–9. doi:10.1038/nature10803. PMID:22318517.
- Sampson JH, Heimberger AB, Archer GE, Aldape KD, Friedman AH, Friedman HS, Gilbert MR, Herndon JE 2nd, McLendon RE, Mitchell DA, et al. Immunologic escape after prolonged progression-free survival with epidermal growth factor receptor variant III peptide vaccination in patients with newly diagnosed glioblastoma. J Clin Oncol. 2010;28(31):4722–9. doi:10.1200/JCO.2010.28.6963. PMID:20921459.
- Brown CE, Alizadeh D, Starr R, Weng L, Wagner JR, Naranjo A, Ostberg JR, Blanchard MS, Kilpatrick J, Simpson J, et al. Regression of Glioblastoma after Chimeric Antigen Receptor T-Cell Therapy. N Engl J Med. 2016;375(26):2561–9. doi:10.1056/NEJMoa1610497. PMID:28029927.
- Hegde M, Mukherjee M, Grada Z, Pignata A, Landi D, Navai SA, Wakefield A, Fousek K, Bielamowicz K, Chow KK, et al. Tandem CAR T cells targeting HER2 and IL13Rα2 mitigate tumor antigen escape. J Clin Invest. 2016;126(8):3036–52. doi:10.1172/JCI83416. PMID:27427982.
- Bielamowicz K, Fousek K, Byrd TT, Samaha H, Mukherjee M, Aware N, Wu MF, Orange JS, Sumazin P, Man TK, et al. Trivalent CAR T-cells Overcome Interpatient Antigenic Variability in Glioblastoma. Neuro Oncol. 2017:1–13. Oxford University. doi:10.1093/neuonc/nox182. PMID:29016929.
- Dutoit V, Herold-Mende C, Hilf N, Schoor O, Beckhove P, Bucher J, Dorsch K, Flohr S, Fritsche J, Lewandrowski P, et al. Exploiting the glioblastoma peptidome to discover novel tumour-associated antigens for immunotherapy. Brain. 2012;135(Pt 4):1042–54. doi:10.1093/brain/aws042. PMID:22418738.