ABSTRACT
CD19 immunotherapies based on T cells opened new avenues in the treatment of pediatric B-cell precursor acute lymphoblastic leukemia (BCP-ALL). However, Fc engineered CD19 antibodies may also bear great potential. In light of recent preclinical and clinical data, perspectives of such antibodies designed for improved effectiveness in BCP-ALL are presented.
CD19 targeting with Fc engineered antibodies optimized for effector cell recruitment
The CD19 antigen is among the front line target structures in clinical trials for antibody-based therapy of B-lineage lymphomas and leukemias due to its restricted expression.Citation1 Breakthroughs were achieved by the bispecific T cell engager (BiTE) antibody blinatumomab and chimeric antigen receptors for genetic engineering of T cells (CAR T cells).Citation2 Promising results were also obtained with novel CD19 antibodies that were optimized by Fc engineering to enhance their therapeutic efficacy in recent years.Citation3,Citation4 These antibody modifications aimed to improve the antibody's affinity to activating Fcγ receptors (FcγR) on various effector cells and thereby boost its ability to elicit effector cell-mediated killing of leukemia cells.Citation5 Fc engineered antibodies were obtained either by introducing distinct amino acid substitutions within the FcγR binding site of the constant heavy chain region (CH) 2 or by modifying the Fc-linked N-glycan by reducing the content of core fucose.Citation3,Citation4,Citation6 Thereby, fit-for-purpose CD19 antibodies such as MOR208 (formerly XmAb5574) carrying the amino acid exchanges S239D/I332E or inebilizumab (MEDI-551) harboring an afucosylated Fc domain were generated. Such antibodies have shown enhanced potency in mediating antibody-dependent cell-mediated cytotoxicity (ADCC) with NK cells and in inducing antibody-dependent cellular phagocytosis (ADCP) of lymphoma and leukemia cells by macrophages in vitro and in animal models.Citation3,Citation4,Citation7 The corresponding native CD19 IgG1 antibodies triggered these effector functions inefficiently. Importantly, Fc engineered CD19 antibodies have demonstrated efficacy and safety in patients with B cell malignancies in early phase clinical trials and applications.Citation8,Citation9 Thus, Fc engineered CD19 antibodies with enhanced FcγR binding have a high potential to extend the current armamentarium of CD19-directed therapies ().
Figure 1. Design of CD19 antibody constructs and CD19 targeting strategies. (A) CD19 antibody constructs include the clinically approved [CD19 × CD3] bispecific T cell engager (BiTE) molecule blinatumomab consisting of two single-chain fragments variable (scFv) fused with a small linker peptide and chimeric antigen receptors (CAR) for T cell engineering containing an extracellular CD19 scFv as well as intracellular signaling domains from CD3ζ and co-stimulatory 4-1BB (TM, transmembrane domain). Fc engineered CD19 antibodies in advanced clinical trials include MOR208, whose Fc domain was engineered by introducing amino acid substitutions S239D/I332), and the nonfucosylated, glyco-engineered antibody inebilizumab (MEDI-551). In addition, CD19 antibody drug conjugates (ADC), in which CD19 antibodies are coupled to cytotoxic agents like monomethyl auristatin F (MMAF) as in denintuzumab mafodotin, (SGN-D19A), are in different phases of clinical development. (B) Blinatumomab redirects T cell cytotoxicity to CD19 by engagement of CD3 on T cells. CAR T cells, which are generated by transduction with CAR expression constructs, recognize CD19-positive leukemia cells via their artificial antigen receptor. In contrast, Fc engineered CD19 antibodies (Ab) activate effector cells including NK cells and macrophages by engagement of activating Fcγ receptors (FcγR) and trigger enhanced antibody-dependent cell-mediated cytotoxicity (ADCC) or phagocytosis (ADCP). In ADCs, the antibody functions as a vehicle to transport the cytotoxic drug to tumor cells. Upon binding, the ADC-antigen complex is internalized and the cytotoxic moiety is released, thereby triggering programmed cell death (PCD).
![Figure 1. Design of CD19 antibody constructs and CD19 targeting strategies. (A) CD19 antibody constructs include the clinically approved [CD19 × CD3] bispecific T cell engager (BiTE) molecule blinatumomab consisting of two single-chain fragments variable (scFv) fused with a small linker peptide and chimeric antigen receptors (CAR) for T cell engineering containing an extracellular CD19 scFv as well as intracellular signaling domains from CD3ζ and co-stimulatory 4-1BB (TM, transmembrane domain). Fc engineered CD19 antibodies in advanced clinical trials include MOR208, whose Fc domain was engineered by introducing amino acid substitutions S239D/I332), and the nonfucosylated, glyco-engineered antibody inebilizumab (MEDI-551). In addition, CD19 antibody drug conjugates (ADC), in which CD19 antibodies are coupled to cytotoxic agents like monomethyl auristatin F (MMAF) as in denintuzumab mafodotin, (SGN-D19A), are in different phases of clinical development. (B) Blinatumomab redirects T cell cytotoxicity to CD19 by engagement of CD3 on T cells. CAR T cells, which are generated by transduction with CAR expression constructs, recognize CD19-positive leukemia cells via their artificial antigen receptor. In contrast, Fc engineered CD19 antibodies (Ab) activate effector cells including NK cells and macrophages by engagement of activating Fcγ receptors (FcγR) and trigger enhanced antibody-dependent cell-mediated cytotoxicity (ADCC) or phagocytosis (ADCP). In ADCs, the antibody functions as a vehicle to transport the cytotoxic drug to tumor cells. Upon binding, the ADC-antigen complex is internalized and the cytotoxic moiety is released, thereby triggering programmed cell death (PCD).](/cms/asset/23c2770b-df38-486e-9d18-92ba06aaa072/koni_a_1448331_f0001_oc.jpg)
Potential of Fc engineered CD19 antibodies in pediatric BCP-ALL
Although about 90% of children can be cured by chemotherapy alone,Citation10 Fc engineered CD19 antibodies may provide an additional tool with limited side effects for some patients.Citation8,Citation9 We have recently evaluated the efficacy of Fc engineered CD19 antibodies in xenograft models of pediatric BCP-ALL,Citation11 the most common malignancy in childhood. Novel treatment modalities are needed in these patients as relapse occurs in ∼15% – 20%, making ALL a leading cause of cancer-related deaths in children. Importantly, certain pediatric patient subgroups such as BCP-ALL involving mixed lineage leukemia (MLL) gene fusions in infants have a particularly poor prognosis.Citation12-Citation14 Novel therapeutic options are also required in the relapsed and refractory setting and in situations in which treatment toxicity has to be reduced, e. g. due to underlying comorbities. An important approach for that purpose is the use of immunotherapies, and therapy with Fc engineered CD19 antibodies may represent a powerful option with few side effects.Citation8,Citation9,Citation15,Citation16
To experimentally address the efficacy and the feasibility of a therapy with an Fc engineered CD19 antibody in pediatric BCP-ALL, we generated antibody CD19-DE using MOR208 V-regions and an Fc domain, which was engineered by introducing the S239D/I332E modifications in the antibody's CH2 domain for improved FcγR binding.Citation3,Citation11 The antibody was tested in patient-derived xenograft (PDX) models of pediatric MLL-rearranged BCP-ALL patients in NSG mice. In an experimental minimal residual disease (MRD) setting, in which very low numbers of ALL cells were transplanted, the antibody demonstrated high efficacy and significantly prolonged the survival of treated animals. A substantial number of PDX mice bearing leukemia cells from different patients were even cured by the therapy, as assessed by PCR-MRD negativity in the bone marrow upon termination of the experiment. Importantly, therapeutic efficacy was lost upon depletion of macrophages in vivo, suggesting that recruitment of macrophages was an important underlying mechanism of action of CD19-DE. This was also confirmed in in vitro ADPC experiments using patient BCP-ALL samples and macrophages from healthy human donors.
To get a more comprehensive picture of the potency of the antibody, CD19-DE was tested in a randomized pre-clinical phase II-like xenograft trial using 13 BCP-ALL patient samples. This experimental design better reflects the diversity and heterogeneity of a patient population and raises the quality of pre-clinical in vivo data.Citation17 Indeed, the experiment revealed the antibody`s efficacy over a broad panel of PDX from different MLL-rearranged BCP-ALL patients, which may have important implications for the applicability of our data to the clinical setting.Citation11 Antibody CD19-DE was also tested in a situation of overt leukemia, in which engraftment of leukemia cells was awaited before initiation of antibody therapy.Citation11 To simulate a clinical situation, the antibody was also combined with chemotherapy. Mimicking an ALL induction regimen consisting of dexamethasone, vincristine and PEG-asparaginase, neither CD19-DE nor chemotherapy alone were able to cure the mice in this overt leukemia situation. However, the leukemia was eradicated in 70% of the PDX mice when CD19-DE and chemotherapy were combined. These data suggest that CD19-DE required concomitant cytoreduction by chemotherapy to achieve its full therapeutic efficacy.
Our experiments reveal the importance of efficient effector cell activation by CD19 antibodies and provide profound in vivo evidence for a broad activity of CD19-DE in MLL-rearranged BCP-ALL.Citation11 These findings may encourage testing of Fc engineered CD19 antibodies in pediatric patients with MLL-rearrangement or other suitable situations. However, this study also delineates limitations of Fc engineered antibody therapy in overt leukemia. In this situation, a higher leukemic burden and unfavorable effector-to-target cell ratios may preclude a cure with the Fc engineered CD19 antibody alone.Citation11 Therefore, Fc engineered CD19 antibody therapy may be particularly effective in situations of low leukemia burden, either in the MRD situation after intensive chemotherapy or upon recurrence of MRD after hematopoietic stem cell transplantation. Furthermore, this type of therapy may be particularly valuable in protocols applying chemotherapy and immunotherapy sequentially.
The role of Fc engineered antibodies in the portfolio of CD19 immunotherapies
Whereas Fc engineered CD19 antibodies such as CD19-DE, MOR208 or inebilizumab recruit FcγR expressing effector cells,Citation3,Citation4,Citation11 CD19 directed immunotherapies currently rely on T cell functions employing either bispecific antibody constructs or applying CAR technologies (, ).Citation2 In addition, CD19 antibody drug conjugates (ADC) are tested in clinical trials.Citation18-Citation20 In our opinion, Fc engineered antibodies may offer certain advantages over blinatumomab or CD19 CAR T cells because Fc engineered antibodies are easy to apply and show only limited toxicity.
The BiTE molecule blinatumomab consists of two single-chain fragment variable (scFv) antibodies fused via a short peptide linker ().Citation2 The first scFv is specific for CD19 while the second one engages the CD3 trigger molecule on T cells. Blinatumomab proved highly efficient in adult BCP-ALL and B-cell lymphoma patients as well as in pediatric BCP-ALL.Citation2,Citation21,Citation22 It has been approved by the FDA for the treatment of relapsed or refractory adult and pediatric ALL patients and current trials are in planning employing blinatumomab in the upfront non-relapsed setting in pediatric ALL also. Likewise, impressive effects in both adults and children were achieved by administration of genetically modified T cells which express a CD19 specific CAR consisting typically of a CD19 scFv fused to intracellular signal transduction domain of CD3ζ for activation and at least one costimulatory domain, e.g. from CD28 or 4-1BB (CD137) ().Citation2,Citation23-Citation24 For generation of autologous CAR T cells, T cells are isolated, expanded ex vivo, and transduced with the CD19 specific CAR construct using viral transduction methods, and re-infused into the patient. Costimulatory domains included in the CAR were shown to be necessary to achieve CAR T cell expansion in the patient and for long persistence, which provides ongoing control of the disease.Citation2 Impressively, in a phase I study by Lee and colleagues in children and younger adults with BCP-ALL CD19 CAR T cell therapy achieved a complete response in 70% of patients.Citation23 In another pilot trial in relapsed/refractory childhood ALL, CR was observed in 94% of the patients and the OS rate was 78%.Citation25 Two CD19 CAR T cell therapies have recently been approved by the FDA. Tisagenlecleucel (CTL019) for the treatment of children and young adults with BCP-ALL that is refractory or in second or later relapse and axicabtagene ciloleucel for the treatment of adult patients with relapsed or refractory large B-cell lymphoma after two or more lines of systemic therapy.Citation24-Citation27 It is important to note that also CD22 specific CAR T cells have recently proven effective in BCP-ALL and may, in the future, provide another valid treatment option for infants and children.Citation28
However, despite the remarkable success and response rates even in patients with high leukemic burden, CAR T cell therapies and, to a lesser extent, blinatumomab are associated with toxicities of T-cell recruitment.Citation2,Citation21,Citation24,Citation27 These toxicities include cytokine release syndrome (CRS), seizures and encephalopathy. In addition, CD19 CAR T cell therapy may be associated with long-term B cell aplasia.Citation2,Citation24 Blinatumomab has the disadvantage that its molecule size is only 55 kDa, which results in unfavorable pharmacokinetic properties with a fast clearance from the blood and the body and an elimination half-life of approximately 2 hours.Citation2,Citation29 Thus, blinatumomab treatment requires continuous infusion over several weeks in each cycle requiring special equipment and training of patients and health professionals. Also, both blinatumomab and CAR T cell therapy are enormously cost intensive. CAR T cells are logistically demanding and can exclusively be applied in specialized centers. In contrast, Fc engineered CD19 antibodies have long plasma retention times and are easy to apply every two or three weeks on an outpatient basis. Their use in humans is associated only with mild adverse events, making this type of therapy particular attractive for infants and children.Citation8,Citation9 Finally, production and usage of Fc engineered antibodies are comparatively easy and may also be suitable for a broad usage even in low and middle income countries.
Future challenges in CD19 therapies in BCP-ALL
CD19 therapies are associated with antigen escape and outgrowth of CD19-negative leukemic sub-clones.Citation23,Citation25,Citation30 CD19-negative relapses were observed both during blinatumomab and CAR T cell treatment. In the majority of cases CD19 antigen loss was attributed to a selection of leukemic cells expressing either CD19 isoforms that lack the cognate epitope for the CD19 CAR construct or CD19 isoforms that were not shuttled to the cell surface.Citation31 More rarely, CD19-directed therapy may promote the emergence of CD19-negative lineage switch variants by shifting leukemic progenitor differentiation towards a myeloid immunophenotype.Citation32-Citation34 This may be important to consider in the treatment of MLL-rearranged BCP-ALL, which is derived from very early progenitor cells that may show an enhanced propensity to lineage switch. Whether CD19-negative relapse will occur during therapy with Fc engineered antibodies or ADCs has not been studied yet. In our preclinical study, outgrowth of CD19-negative subclones in PDX animals was not observed and such a phenomenon was not reported in early clinical studies of similar constructs in patients.Citation9,Citation11,Citation15 CD22 specific immunotherapies (e.g. the ADC inotuzumab ozogamicinCitation35 and CD22 CAR T cellsCitation28) or antibodies against myeloid targets may represent valid treatment options in that situation. A promising future concept for the therapy with Fc engineered antibodies is the combination of two antibodies of different specificities for simultaneous targeting of two antigens, which should hamper tumor escape by loss of one of the targets.
Naked antibodies as single agents are often not efficient in situations other than MRD, which was also observed in our own PDX study with CD19-DE in the overt leukemia setting.Citation11 On the basis of our findings we propose to enhance the efficacy of such antibodies by stimulating effector cells, in particular macrophages (). These cells played a crucial role our animal study. Of note, increasing evidence suggests that next to natural killer cells, macrophages and other myeloid effector cells play an important role in mediating the cytotoxic effects of antibody therapy.Citation36 Macrophages are almost universally present, and although promotion of cancer growth cannot be completely neglected, they were also shown to exert vigorous anti-tumor responses upon antibody treatment. CD19-DE and the antibodies MOR208 and inebilizumab efficiently triggered ADCP of BCP-ALL cells by human macrophages in vitro.Citation3,Citation4,Citation11 It could recently be shown that rational combinations of antibodies with chemotherapeutic regimens may remodel the tumor microenvironment to overcome resistance of leukemia cells to macrophage-mediated killing in the bone marrow.Citation37 Whether such effects also contributed to the observed benefits achieved by combination of the antibody with vincristine, PEG-asparaginase and dexamethasone should be a matter of future studies.
Figure 2. Enhancing effector cell-mediated antibody functions. Potential strategies to further improve antibody-dependent cell-mediated phagocytosis (ADCP) by Fc engineered CD19 antibodies include combination of the antibody with chemotherapy regimen that modulate the tumor microenvironment in the bone marrow (e. g. by induced secretion of cytokines such as TNFα). Also, ADCP may be promoted by blocking interactions between the inhibitory SIRPα receptor on macrophages and the ‘don't eat me’ signal CD47 on ALL blasts. Another strategy may be to co-stimulate NK cells by combining CD19 antibodies with immune modulatory antibodies targeting for example the co-stimulatory CD137 receptor, which alter the NK cell activation status and boost antibody-dependent cell-mediated cytotoxicity (ADCC) in combination with a tumor targeting antibody. Moreover, CD19 antibodies may be combined with immunomodulatory cytokines (e. g. GM-CSF, IFN-γ) or drugs (e. g. lenalidomide) for sustained activation of lymphocytes or myeloid effector cells.
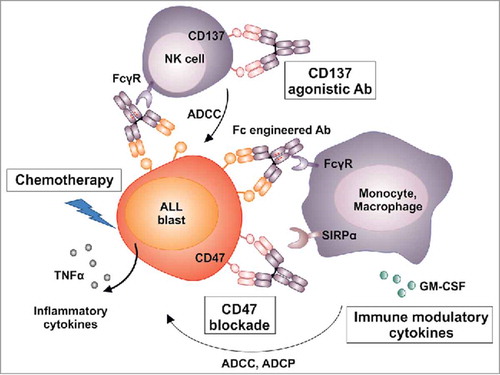
In addition, the phagocytic uptake of ALL cells by macrophages may be enhanced by blocking interactions between the inhibitory receptor signal-regulatory-protein (SIRP) α on myeloid effector cells and the ‘don't eat me’ signal CD47 on target cells.Citation38 This may be achieved by monoclonal CD47 antibodies or blocking peptides. Another strategy may be to co-stimulate myeloid effector cells and NK cells, which are absent in our NSG PDX model, but are expected to contribute to antibody-mediated effects in patients. Combining CD19 antibodies with agonistic CD137 antibodies, which were shown to alter the NK cell activation status leading to enhanced ADCCCitation39 may be warranted. Moreover, Fc engineered antibodies may be combined with immunomodulatory cytokines (e. g. GM-CSF, IFN-γ) or drugs (e. g. lenalidomide) to promote activation of lymphocytes and/or myeloid effector cells ().Citation40
Conclusions
CD19 directed immunotherapies using blinatumomab or CAR T cells have been approved as valid options in the treatment of relapse or refractory childhood BCP-ALL. Fc engineered CD19 antibodies may further expand the therapeutic options. Fc engineered CD19 antibodies are able to kill leukemia cells by engaging different effector cell populations, leading to induction of cytotoxicity and phagocytosis. Such constructs have a long blood retention time compared to blinatumomab and could be applied more conveniently. Despite improved FcγR affinity, this type of antibodies show only few side effects when applied in patients,Citation9,Citation15 and, importantly, side effects of T-cell activation such as CRS and encephalopathy may be avoided. Despite such expected advantages compared to blinatumomab or CAR T cells, it remains to be determined whether Fc engineered antibodies will be equally efficient in the clinic. It will be particularly important to determine whether long-lasting tumor control can be achieved and which are the best combination strategies with other therapies.
Disclosure of conflicts of interest
The authors declare no competing financial interests.
Acknowledgments
M. P. is supported by the Mildred-Scheel professorship program by the Deutsche Krebshilfe e. V. C. K., M. P. and D. M. S. are supported by research grants by the Wilhelm Sander Foundation. C. K. and D. M. S. are supported by a research grant by the Deutsche José Carreras Leukämie-Stiftung.
References
- Strohl WR. Current progress in innovative engineered antibodies. Protein Cell. 2018;9(1):86–120. doi:10.1007/s13238-017-0457-8. PMID:28822103.
- Aldoss I, Bargou RC, Nagorsen D, Friberg GR, Baeuerle PA, Forman SJ. Redirecting T cells to eradicate B-cell acute lymphoblastic leukemia: Bispecific T-cell engagers and chimeric antigen receptors. Leukemia. 2017;31(4):777–87. doi:10.1038/leu.2016.391. PMID:28028314.
- Horton HM, Bernett MJ, Pong E, Peipp M, Karki S, Chu SY, Richards JO, Vostiar I, Joyce PF, Repp R, et al. Potent in vitro and in vivo activity of an Fc-engineered anti-CD19 monoclonal antibody against lymphoma and leukemia. Cancer Res. 2008;68(19):8049–57. doi:10.1158/0008-5472.CAN-08-2268. PMID:18829563.
- Matlawska-Wasowska K, Ward E, Stevens S, Wang Y, Herbst R, Winter SS, Wilson BS. Macrophage and NK-mediated killing of precursor-B acute lymphoblastic leukemia cells targeted with a-fucosylated anti-CD19 humanized antibodies. Leukemia. 2013;27(6):1263–74. doi:10.1038/leu.2013.5. PMID:23307031.
- Kellner C, Otte A, Cappuzzello E, Klausz K, Peipp M. Modulating Cytotoxic Effector Functions by Fc Engineering to Improve Cancer Therapy. Transfus Med Hemother. 2017;44(5):327–36. doi:10.1159/000479980. PMID:29070978.
- Pfeiffer M, Stanojevic S, Feuchtinger T, Greil J, Handgretinger R, Barbin K, Jung G, Martin D, Niethammer D, Lang P. Rituximab mediates in vitro antileukemic activity in pediatric patients after allogeneic transplantation. Bone Marrow Transplant. 2005;36(2):91–97. doi:10.1038/sj.bmt.1705014. PMID:15908973.
- Kellner C, Zhukovsky EA, Potzke A, Bruggemann M, Schrauder A, Schrappe M, Kneba M, Repp R, Humpe A, Gramatzki M, et al. The Fc-engineered CD19 antibody MOR208 (XmAb5574) induces natural killer cell-mediated lysis of acute lymphoblastic leukemia cells from pediatric and adult patients. Leukemia. 2013;27(7):1595–8. doi:10.1038/leu.2012.373. PMID:23277329.
- Woyach JA, Awan F, Flinn IW, Berdeja JG, Wiley E, Mansoor S, Huang Y, Lozanski G, Foster PA, Byrd JC. A phase 1 trial of the Fc-engineered CD19 antibody XmAb5574 (MOR00208) demonstrates safety and preliminary efficacy in relapsed CLL. Blood. 2014;124(24):3553–60. doi:10.1182/blood-2014-08-593269. PMID:25301708.
- Seidel UJ, Schlegel P, Grosse-Hovest L, Hofmann M, Aulwurm S, Pyz E, Schuster FR, Meisel R, Ebinger M, Feuchtinger T, et al. Reduction of Minimal Residual Disease in Pediatric B-lineage Acute Lymphoblastic Leukemia by an Fc-optimized CD19 Antibody. Mol Ther. 2016;24(9):1634–43. doi:10.1038/mt.2016.141. PMID:27380762.
- Pui CH, Yang JJ, Hunger SP, Pieters R, Schrappe M, Biondi A, Vora A, Baruchel A, Silverman LB, Schmiegelow K, et al. Childhood Acute Lymphoblastic Leukemia: Progress Through Collaboration. J Clin Oncol. 2015;33(27):2938–48. doi:10.1200/JCO.2014.59.1636. PMID:26304874.
- Schewe DM, Alsadeq A, Sattler C, Lenk L, Vogiatzi F, Cario G, Vieth S, Valerius T, Rosskopf S, Meyersieck F, et al. An Fc-engineered CD19 antibody eradicates MRD in patient-derived MLL-rearranged acute lymphoblastic leukemia xenografts. Blood. 2017;130(13):1543–52. doi:10.1182/blood-2017-01-764316. PMID:28698205.
- Locatelli F, Schrappe M, Bernardo ME, Rutella S. How I treat relapsed childhood acute lymphoblastic leukemia. Blood. 2012;120(14):2807–16. doi:10.1182/blood-2012-02-265884. PMID:22896001.
- Brown P. Treatment of infant leukemias: challenge and promise. Hematology Am Soc Hematol Educ Program. 2013;2013:596–600. doi:10.1182/asheducation-2013.1.596. PMID:24319237.
- Pieters R, Schrappe M, De Lorenzo P, Hann I, De Rossi G, Felice M, Hovi L, LeBlanc T, Szczepanski T, Ferster A, et al. A treatment protocol for infants younger than 1 year with acute lymphoblastic leukaemia (Interfant-99): An observational study and a multicentre randomised trial. Lancet. 2007;370(9583):240–50. doi:10.1016/S0140-6736(07)61126-X. PMID:17658395.
- Jurczak W, Zinzani PL, Gaidano G, Goy A, Provencio M, Nagy Z, Robak T, Maddocks KJ, Buske C, Korolkiewicz RP, et al. Phase IIa Study of Single-Agent MOR208 in Patients with Relapsed or Refractory B-Cell Non-Hodgkin's Lymphoma. Blood. 2015;126(23):1528. PMID:26059948
- Forero-Torres A, Hamadani M, Fanale MA, Bello CM, J. KT, Offner F, Verhoef G, Federico M, Gregory SA, Sonet A, et al. Safety Profile and Clinical Response To MEDI-551, a Humanized Monoclonal Anti-CD19, In a Phase 1/2 Study In Adults With Relapsed Or Refractory Advanced B-Cell Malignancies. Blood. 2013;122(21):1810.
- Townsend EC, Murakami MA, Christodoulou A, Christie AL, Koster J, DeSouza TA, Morgan EA, Kallgren SP, Liu H, Wu SC, et al. The Public Repository of Xenografts Enables Discovery and Randomized Phase II-like Trials in Mice. Cancer cell. 2016;29(4):574–86. doi:10.1016/j.ccell.2016.03.008. PMID:27070704.
- Ryan MC, Palanca-Wessels MC, Schimpf B, Gordon KA, Kostner H, Meyer B, Yu C, Van Epps HA, Benjamin D. Therapeutic potential of SGN-CD19B, a PBD-based anti-CD19 drug conjugate, for treatment of B-cell malignancies. Blood. 2017;130(18):2018–26. doi:10.1182/blood-2017-04-779389. PMID:28903943.
- Fathi AT, Borate U, DeAngelo DJ, O'Brien MM, Trippett T, Shah BD, Hale GA, Foran JM, Silverman LB, Tibes R, et al. A Phase 1 Study of Denintuzumab Mafodotin (SGN-CD19A) in Adults with Relapsed or Refractory B-Lineage Acute Leukemia (B-ALL) and Highly Aggressive Lymphoma. Blood. 2015;126(23):1328.
- Kantarjian HM, Lioure B, Kim SK, Atallah E, Leguay T, Kelly K, Marolleau JP, Escoffre-Barbe M, Thomas XG, Cortes J, et al. A Phase II Study of Coltuximab Ravtansine (SAR3419) Monotherapy in Patients With Relapsed or Refractory Acute Lymphoblastic Leukemia. Clin Lymphoma Myeloma Leuk. 2016;16(3):139–45. doi:10.1016/j.clml.2015.12.004. PMID:26775883.
- von Stackelberg A, Locatelli F, Zugmaier G, Handgretinger R, Trippett TM, Rizzari C, Bader P, O'Brien MM, Brethon B, Bhojwani D, et al. Phase I/Phase II Study of Blinatumomab in Pediatric Patients With Relapsed/Refractory Acute Lymphoblastic Leukemia. J Clin Oncol. 2016;34(36):4381–9. doi:10.1200/JCO.2016.67.3301. PMID:27998223.
- Topp MS, Gokbuget N, Stein AS, Zugmaier G, O'Brien S, Bargou RC, Dombret H, Fielding AK, Heffner L, Larson RA, et al. Safety and activity of blinatumomab for adult patients with relapsed or refractory B-precursor acute lymphoblastic leukaemia: a multicentre, single-arm, phase 2 study. Lancet Oncol. 2015;16(1):57–66. doi:10.1016/S1470-2045(14)71170-2. PMID:25524800
- Lee DW, Kochenderfer JN, Stetler-Stevenson M, Cui YK, Delbrook C, Feldman SA, Fry TJ, Orentas R, Sabatino M, Shah NN, et al. T cells expressing CD19 chimeric antigen receptors for acute lymphoblastic leukaemia in children and young adults: a phase 1 dose-escalation trial. Lancet. 2015;385(9967):517–28. doi:10.1016/S0140-6736(14)61403-3. PMID:25319501.
- Maude SL, Frey N, Shaw PA, Aplenc R, Barrett DM, Bunin NJ, Chew A, Gonzalez VE, Zheng Z, Lacey SF, et al. Chimeric antigen receptor T cells for sustained remissions in leukemia. N Engl J Med. 2014;371(16):1507–17. doi:10.1056/NEJMoa1407222. PMID:25317870.
- Grupp SA MS, Shaw PA, Aplenc R, Barrett DR, Callahan C, et al. . Durable Remissions in Children with Relapsed/Refractory ALL Treated with T Cells Engineered with a CD19-Targeted Chimeric Antigen Receptor (CTL019). Blood. 2015;126(23):681.
- Neelapu SS, Locke FL, Bartlett NL, Lekakis LJ, Miklos DB, Jacobson CA, Braunschweig I, Oluwole OO, Siddiqi T, Lin Y, et al. Axicabtagene Ciloleucel CAR T-Cell Therapy in Refractory Large B-Cell Lymphoma. N Engl J Med. 2017;377(26):2531–44. doi:10.1056/NEJMoa1707447. PMID:29226797
- Maude SL, Laetsch TW, Buechner J, Rives S, Boyer M, Bittencourt H, Bader P, Verneris MR, Stefanski HE, Myers GD, et al. Tisagenlecleucel in Children and Young Adults with B-Cell Lymphoblastic Leukemia. N Engl J Med. 2018;378(5):439–48. doi:10.1056/NEJMoa1709866. PMID:29385370.
- Fry TJ, Shah NN, Orentas RJ, Stetler-Stevenson M, Yuan CM, Ramakrishna S, Wolters P, Martin S, Delbrook C, Yates B, et al. CD22-targeted CAR T cells induce remission in B-ALL that is naive or resistant to CD19-targeted CAR immunotherapy. Nat Med. 2018;24(1):20–28. doi:10.1038/nm.4441. PMID:29155426.
- Zhu M, Wu B, Brandl C, Johnson J, Wolf A, Chow A, Doshi S. Blinatumomab, a Bispecific T-cell Engager (BiTE((R))) for CD-19 Targeted Cancer Immunotherapy: Clinical Pharmacology and Its Implications. Clin Pharmacokinet. 2016;55(10):1271–88. doi:10.1007/s40262-016-0405-4. PMID:27209293.
- Topp MS, Gokbuget N, Zugmaier G, Degenhard E, Goebeler ME, Klinger M, Neumann SA, Horst HA, Raff T, Viardot A, et al. Long-term follow-up of hematologic relapse-free survival in a phase 2 study of blinatumomab in patients with MRD in B-lineage ALL. Blood. 2012;120(26):5185–7. doi:10.1182/blood-2012-07-441030. PMID:23024237.
- Sotillo E, Barrett DM, Black KL, Bagashev A, Oldridge D, Wu G, Sussman R, Lanauze C, Ruella M, Gazzara MR, et al. Convergence of Acquired Mutations and Alternative Splicing of CD19 Enables Resistance to CART-19 Immunotherapy. Cancer Discov. 2015;5(12):1282–95. doi:10.1158/2159-8290.CD-15-1020. PMID:26516065.
- Jacoby E, Nguyen SM, Fountaine TJ, Welp K, Gryder B, Qin H, Yang Y, Chien CD, Seif AE, Lei H, et al. CD19 CAR immune pressure induces B-precursor acute lymphoblastic leukaemia lineage switch exposing inherent leukaemic plasticity. Nat Commun. 2016;7:12320. doi:10.1038/ncomms12320. PMID:27460500.
- Rayes A, McMasters RL, O'Brien MM. Lineage Switch in MLL-Rearranged Infant Leukemia Following CD19-Directed Therapy. Pediatric blood & cancer. 2016;63(6):1113–5. doi:10.1002/pbc.25953. PMID:26914337.
- Gardner R, Wu D, Cherian S, Fang M, Hanafi LA, Finney O, Smithers H, Jensen MC, Riddell SR, Maloney DG, et al. Acquisition of a CD19-negative myeloid phenotype allows immune escape of MLL-rearranged B-ALL from CD19 CAR-T-cell therapy. Blood. 2016;127(20):2406–10. doi:10.1182/blood-2015-08-665547. PMID:26907630.
- Kantarjian HM, DeAngelo DJ, Stelljes M, Martinelli G, Liedtke M, Stock W, Gokbuget N, O'Brien S, Wang K, Wang T, et al. Inotuzumab Ozogamicin versus Standard Therapy for Acute Lymphoblastic Leukemia. N Engl J Med. 2016;375(8):740–53. doi:10.1056/NEJMoa1509277. PMID:27292104.
- Weiskopf K, Weissman IL. Macrophages are critical effectors of antibody therapies for cancer. MAbs. 2015;7(2):303–10. doi:10.1080/19420862.2015.1011450. PMID:25667985.
- Pallasch CP, Leskov I, Braun CJ, Vorholt D, Drake A, Soto-Feliciano YM, Bent EH, Schwamb J, Iliopoulou B, Kutsch N, et al. Sensitizing protective tumor microenvironments to antibody-mediated therapy. Cell. 2014;156(3):590–602. doi:10.1016/j.cell.2013.12.041. PMID:24485462.
- Chao MP, Alizadeh AA, Tang C, Jan M, Weissman-Tsukamoto R, Zhao F, Park CY, Weissman IL, Majeti R. Therapeutic antibody targeting of CD47 eliminates human acute lymphoblastic leukemia. Cancer Res. 2011;71(4):1374–84. doi:10.1158/0008-5472.CAN-10-2238. PMID:21177380.
- Kohrt HE, Houot R, Goldstein MJ, Weiskopf K, Alizadeh AA, Brody J, Muller A, Pachynski R, Czerwinski D, Coutre S, et al. CD137 stimulation enhances the antilymphoma activity of anti-CD20 antibodies. Blood. 2011;117(8):2423–32. doi:10.1182/blood-2010-08-301945. PMID:21193697.
- Houot R, Kohrt H, Goldstein MJ, Levy R. Immunomodulating antibodies and drugs for the treatment of hematological malignancies. Cancer Metastasis Rev. 2011;30(1):97–109. doi:10.1007/s10555-011-9274-3. PMID:21271352.