ABSTRACT
Despite increasing evidence for a protective role of invariant (i) NKT cells in the control of graft-versus-host disease (GVHD), the mechanisms underpinning regulation of the allogeneic immune response in humans are not known. In this study, we evaluated the distinct effects of human in vitro expanded and flow-sorted human CD4+ and CD4− iNKT subsets on human T cell activation in a pre-clinical humanized NSG mouse model of xenogeneic GVHD. We demonstrate that human CD4− but not CD4+ iNKT cells could control xenogeneic GVHD, allowing significantly prolonged overall survival and reduced pathological GVHD scores without impairing human T cell engraftment. Human CD4− iNKT cells reduced the activation of human T cells and their Th1 and Th17 differentiation in vivo. CD4− and CD4+ iNKT cells had distinct effects upon DC maturation and survival. Compared to their CD4+ counterparts, in co-culture experiments in vitro, human CD4− iNKT cells had a higher ability to make contacts and degranulate in the presence of mouse bone marrow-derived DCs, inducing their apoptosis. In vivo we observed that infusion of PBMC and CD4− iNKT cells was associated with decreased numbers of splenic mouse CD11c+ DCs. Similar differential effects of the iNKT cell subsets were observed on the maturation and in the induction of apoptosis of human monocyte-derived dendritic cells in vitro. These results highlight the increased immunosuppressive functions of CD4− versus CD4+ human iNKT cells in the context of alloreactivity, and provide a rationale for CD4− iNKT selective expansion or transfer to prevent GVHD in clinical trials.
Introduction
Acute graft-versus-host disease (GVHD) occurs in up to 50% of patients who receive an allogeneic HLA-matched hematopoietic stem cell transplantation (allo-HSCT) and still represents a major cause of post-transplant morbidity and mortality.Citation1 Thus, reducing aGVHD while preserving its beneficial anti-leukemic (GVL) effect remains the main challenge of allo-SCT.
The pathophysiology of aGVHD, has been described as a multi-step process involving host antigen presenting cells (APC) as the main initiators and donor T cells as the major effectors of the allogeneic immune response.Citation2 Acute GVHD has been mainly associated with a Th1 and Th17 T cell profile Citation3 and can be down regulated by several subsets of immunoregulatory cells of potential therapeutic interest; among these, CD4+CD25highFoxP3+regulatory T cells (Tregs) have been shown to prevent aGVHD following haplo-identical SCT,Citation4 but there are some concerns about the their potential impairment of the GVL effect.Citation5,Citation6
Invariant NKT cells (iNKT) represent another immunoregulatory T cell subset characterized by a CD1d-restricted invariant TCR that recognizes glycolipids presented by the non-classical MHC class Ib-like molecule CD1d.Citation7 The hallmark of iNKT cells is a rapid production of a wide spectrum of cytokines and chemokines, as well as cytotoxic capabilities that enable them to regulate the immune response.Citation8 Several groups have shown the ability of recipient or donor mouse iNKT cells to control GVHD, without affecting the GVL effect.Citation9–Citation14 In humans, our group and others previously reported that the graft content of iNKT cells and their post-transplant reconstitution are associated with a reduced risk of aGVHD whereas GVL effects were preserved.Citation15–Citation18
Although iNKT cells represent an evolutionary conserved CD1d-restricted subset of T cells, murine and human iNKT cells display major functional differences. There is also a very high discrepancy of proportions and differentiation stage of circulating iNKT cells between mice and humans. In peripheral human blood, iNKT represent a highly variable proportion of CD3+ T cells (0.001 to 1%) with a homogeneous late (stage 3) maturation state.Citation19,Citation20 In mice, they reach 0,2–1% of circulating CD3+ T cells, in which the three maturation stages are heterogeneously represented.Citation21 Most importantly, while in mice, both CD4+ or CD4− subsets can produce Th1 and Th2 cytokines,Citation22 human iNKT cells are separated in two functionally distinct subsets: the CD4+ subset producing Th1 and Th2 cytokines (IL-4, IL-13, IFN-γ) and the CD4− subset producing a more restricted Th1 (IFN-γ) profile.Citation23,Citation24 In mice, the protective effect of iNKT cells upon GVHD has been related to IL-4 Citation14,Citation25 and the induced expansion of Tregs.Citation26,Citation27 In humans, correlations have been described between the occurrence of acute GVHD and the graft content or the expansion capacities of iNKT cells Citation16,Citation28 but their direct implication in the control of GVHD and their mechanisms of action had not been yet established. In addition, while in mice the CD4+ iNKT cell subset has been shown to control GVHD, the correlations made in humans between graft iNKT cells and the occurrence of aGVHD suggest a role for the CD4− iNKT cell subset.Citation16,Citation28 Because CD4− iNKT cells represent the predominant iNKT subtype in human grafts and in the recipient’s peripheral blood after transplantation, their potential interest compared to their CD4+ counterparts in the control of alloreactivity needed to be explored.
In this study, we therefore compared the potential role and mechanisms of action of human CD4+ versus CD4− iNKT subsets in the NSG mouse model of xenogeneic GVHD.
Results
Human CD4− but not CD4+ iNKT cells reduce GVHD in a xenogeneic model of human cell transplantation
Irradiated NSG mice were transplanted with iNKT-depleted human PBMCs (PBMC alone group) or PBMC supplemented with either autologous ex vivo-expanded CD4− iNKT (PBMC+iNKT4neg group) or CD4+ iNKT cells (PBMC+iNKT4pos group) at an iNKT/T cell ratio of 1:6. Co-administration of CD4− iNKT cells and human PBMCs prolonged the overall survival () and reduced weight loss (), clinical GVHD () and liver pathological scores () in comparison to mice transplanted with human PBMCs alone. In contrast, addition of CD4+ iNKT cells to PBMCs had no effect. As described in this model after low doses of PBMC injection,Citation29 skin and gut clinical symptoms were invariably absent at 4 weeks after transplantation and pathological GVHD scores in these organs were very limited (Fig S1).
Figure 1. Human iNKT4−, but not iNKT4+ cells can control the development of xeno-GVHD in irradiated NSG mice
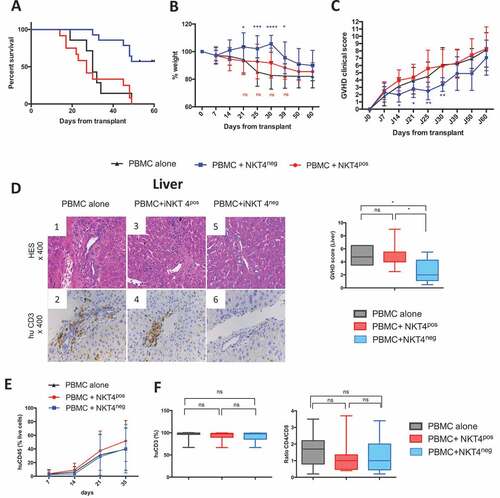
The reduction of xenogeneic GVHD in mice receiving human CD4− iNKT cells was not due to impaired engraftment of human T cells, since kinetics and rates of CD45+ human cell engraftment in mouse peripheral blood were similar between all groups of mice (). At day 35, percentages of circulating human CD3+ T cells and CD4/CD8 T cell ratios were similar in all groups (). Percentages of human T cells were also similar between all groups in the spleen and bone marrow (Fig S2). Of note, no circulating human iNKT cells were observed in any of the groups after day 21 of human cell transplantation.
Human CD4− and CD4+ iNKT cells differentially modulate human t cell activation and cytokine responses in the context of xenogeneic activation in vivo
We observed that co-administration of iNKT cell subsets did not inhibit human T cell proliferation in vivo as evaluated by incorporation of BrdU (). However, in the PBMC+iNKT4neg group, there was reduced expression of the activation markers, CD25 and CD69, on circulating human CD4+ T and CD8+ T cells in comparison to the PBMC+iNKT4pos group (, ). We did not observe any significant differences in the proportion of FoxP3+ CD4+ T cells among total human CD4+ T cells in the blood, spleen and bone marrow between the 3 groups of mice (). Measurement of plasma levels of human cytokines in the plasma of the BMT recipients showed reduced levels of IL-17, IFN-γ, TNF-α and but increased concentrations of IL-4 at day 21 in the PBMC+iNKT4neg group in comparison to mice transplanted with PBMCs alone (). Mice in the PBMC+iNKT4pos group also had reduced IFN-γ levels and higher IL-4; however, IL-17 and TNF-α levels were similar to mice transplanted with PBMCs alone. Plasma levels of human IL-2, IL-6, IL-10, IL2Ra, IL1Ra, MIP1a, IL-12 and IL-7 were similar in all groups of mice (Fig S4). In summary, the balance between Th1+Th17 versus Th2 cytokines was shifted in favor of a more Th2-like profile in PBMC+iNKT4neg group in comparison to the other groups (). Intracellular staining of human CD4+ T cells isolated from the spleen of BMT recipients showed significantly lower percentages of IL-17+ and RORγt+ cells in the PBMC+iNKT4neg group compared to the other groups ().
Figure 2. In vivo assessment of mouse serum levels of human cytokine and T cell polarization after human PBMC + iNKT CD4− or CD4+ injection
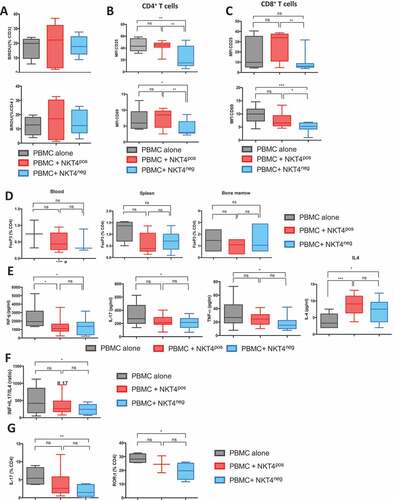
Human iNKT cell subsets have differential effects on mouse DCs in vitro and in vivo
Because antigenic presentation is a primary event in the T cell activation and cytokine response, we further analyzed the effects of CD4+ and CD4− iNKT cells on mouse DCs in the model of xenogenic GVHD. At day 28 after BMT, we observed reduced frequencies of CD11c+ murine DCs in the spleens of recipients in the PBMC+iNKT4neg group in comparison to the other groups (). To determine the maturation status of the CD11c+ population, we measured the expression of several markers associated with DC differentiation. We found that the expression of CD40, CD80 and CD86 were increased upon murine CD11c+ DCs in the PBMC+iNKT4pos group compared to human PBMCs alone (). In contrast, murine DC expression of CD40 and CD86 in the PBMC+iNKT4neg group was similar to mice transplanted human PBMCs alone ().
Figure 3. In vivo and in vitro assessment of human iNKT CD4− or CD4+ cell effects on the survival and maturation mouse bone marrow derived dendritic cells (moBMDC)
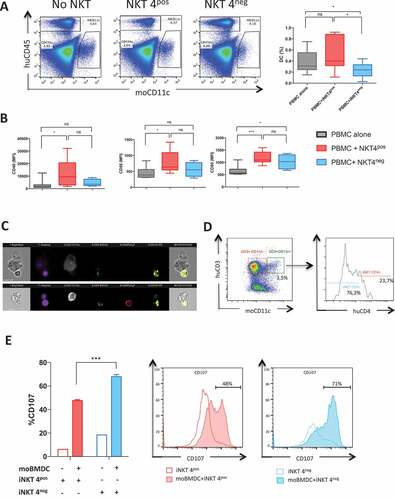
As human iNKT have been reported to recognize murine CD1d,Citation30,Citation31 we further analyzed whether human CD4− or CD4+ iNKT cells could interact with NSG mouse (mo) BMDCs in vitro. Image Stream analysis of co-cultures of human CD4− and CD4+ iNKT cells with murine BM-derived DCs at a 2:2:1 ratio, showed that both CD4− and CD4+ human iNKT subsets could form contacts with the murine cells (). However, human CD4− iNKT cells made more than 3-fold more contacts than CD4+ iNKT cells (76.3 versus 23.7%, p < 0.0001) (), suggesting that the former population could outcompete the latter for contacts with DC. To test whether increased contact formation was associated led to cytotoxic molecule degranulation, we determined the CD107a externalization. As shown in , CD107a externalization was significantly higher in CD4− compared to CD4+ iNKT cells, suggesting a higher degranulation capacity of iNKT CD4− cells in contact with murine DC.
Human iNKT cell subsets have opposite effects on human monocyte-derived DCs in vitro
Based on the distinct effects of human iNKT subsets observed on mouse BM-derived and splenic DCs, we further explored the effects of human iNKT subsets on the survival and maturation of human monocyte-derived DCs (moDCs) in vitro. Human CD4− iNKT cells have been reported to be capable of killing human mature moDC at a high iNKT:DC ratios.Citation16 We comparatively analyzed the capacity of both human CD4+ and CD4− iNKT cells to induce the apoptosis or necrosis of mature moDCs at different iNKT:DC ratios in vitro. We found that both iNKT cell subsets could kill mature MoDCs in a dose dependent manner from iNKT:DC ratios of 1:1 to 10:1, mainly by inducing the apoptosis of moDCs (). At iNKT:T ratios above 2:1, CD4− iNKT cells were consistently more efficient than CD4+ iNKT cells at inducing early (Annexin V+ PI−) and late (Annexin V+ PI+) apoptosis of moDCs (). The induction of apoptosis of human MoDCs by both iNKT cell subsets was contact dependent ().
Figure 4. In vitro effects of human CD4+ and CD4− iNKT cell subsets on the maturation and survival of human monocyte-derived DCs
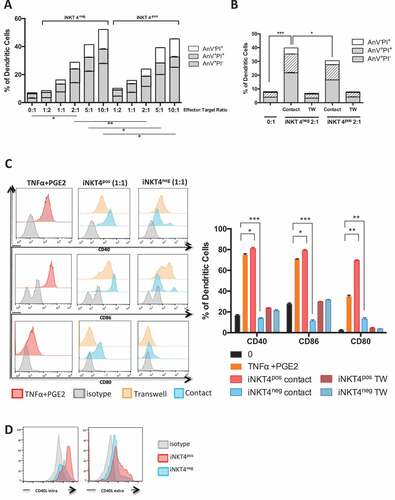
In addition, to a previous description of differential effects of human CD4− and CD4+ iNKT cells on human DC maturation and function,Citation32 we observed that human CD4+ iNKT cells induce the maturation of moDCs in a contact-dependent manner even in the absence of additional maturation cytokines, whereas human CD4− iNKT cells had no effect (). The differential effect of CD4+ and CD4− iNKT cells on the maturation of immature MoDCs might be explained by higher expression of CD40-L on CD4+ in comparison to CD4− iNKT cells ().
Taken together, these data show that unlike their CD4+ counterparts, human CD4− iNKT cells show greater proficiency for inducing the apoptosis of mature DCs and do not induce the maturation of immature DCs.
Discussion
There in an increasing interest in using iNKT cells as a cellular therapy for GVHD control.Citation33,Citation34 In this study we aimed to identify which subtype of human iNKT might prevent GVHD and its mechanism of action in a xenogeneic GVHD model. We show that human CD4− iNKT cells and not their CD4+ counterpart protect xenografted mice from GVHD by interfering with recipient APCs and human T cell responses. Human CD4− iNKT cell subset has the unique potency to reduce the numbers and maturation levels of recipient APCs and therefore to reduce human allo- and xenogeneic T cell activation as well as Th1 and Th17 T cell cytokine production without inhibiting human T cell engraftment.
These results are in line with our and others’ recent reported data showing that CD4− iNKT cell graft content and/or expansion capacities represent major predictive factors of the occurrence of acute GVHD in allografted patients.Citation15,Citation16,Citation28 Nevertheless, the results obtained in humans appear in apparent contradiction with the description of a protective effect of murine CD4+ iNKT cells Citation26,Citation35 or IL-4 producing murine iNKT cells in mouse GVHD models,Citation9,Citation11,Citation14,Citation25 since IL-4 is mainly produced by CD4+ human iNKT cells. However, to our knowledge, no study has compared the differential effects of murine CD4− versus CD4+ iNKT cell subsets, which seems puzzling as both mouse CD4+ and CD4− iNKT cells can produce IL-4.Citation22 The protective effect of human CD4− iNKT cells, which are functionally characterized by the rapid production of high amounts of INF-γ, in the context of GVHD can also be conciliated with the described benefic effect of INF-γ when administered early after allo-SCT.Citation36 Moreover, in a mouse model of GVHD, infusion of murine type II CD8+ NKT cells secreting high amounts of INF-γ has been reported to delay the occurrence of GVHD.Citation37,Citation38
Another apparent discrepancy between murine and human iNKT cells in the regulation of GVHD is the description of interactions between iNKT cells and Tregs in mouse models of GVHD Citation25,Citation27,Citation35 that has not been observed in our mouse model. This might be explained by the relatively limited numbers of Tregs injected within the 3 × 106 human PBMC graft and their absence in NSG mice. This might also be due to intrinsic limits of the xenogeneic GVHD model, which in the absence of production of human cytokines, such as IL-2, might be associated with impaired long-term survival of human Treg and iNKT cells. In favor of this hypothesis, we could not detect either Treg or iNKT in the peripheral blood, spleen or bone marrow of transplanted mice after engraftment of human T cells; we cannot, however, exclude their earlier migration to other tissues.
Mice receiving CD4− iNKT cells had reduced expression of human T cell activation markers and of Th1 and Th17 cytokines in comparison to mice transplanted with or without CD4+ iNKT cells. Moreover, human iNKT have been reported to switch human DCs towards a tolerogenic phenotype.Citation32 These facts led us to hypothesize differential modulatory effects of human CD4+ and CD4− iNKT cell subsets on APCs during the allogeneic immune response. Whereas both subsets induced the apoptosis of mature monocyte-derived DCs, the human CD4− iNKT subset had higher cytotoxic capacities. This observation is in agreement with reported higher expression of cytotoxic molecules such as NKG2D or perforin/granzyme by CD4− in comparison to CD4+ iNKT cells.Citation16,Citation32 In vitro, by contrast with their CD4− counterparts, CD4+ iNKT cells induce the maturation of monocyte-derived DCs without any additional cytokines, in a contact-dependent manner, possibly related to their higher expression of CD40-L than CD4− iNKT cells.Citation32 As human CD1d recognition by iNKT are largely conserved between speciesCitation30,Citation31, we hypothesized that human iNKT could modulate mouse DC maturation and survival and explain by this way the modulation of xenogeneic GVHD. We indeed showed that human iNKT cells can interact with mouse DCs and that CD4− iNKT cells are more likely to make contacts with mouse DCs and to kill murine DCs in vitro and in vivo than CD4+ iNKT cells. By contrast and compared to their CD4− counterparts, CD4+ iNKT cells induce the maturation of immature DCs without impairing the numbers of splenic DCs in vivo. These opposite effects of CD4+ and CD4− iNKT cells on DC maturation and survival can certainly explain the increased T cell activation and absence of protection from GVHD observed in the presence of CD4+ in comparison to CD4− iNKT cells.
In comparison to Tregs,Citation4,Citation39,Citation40 iNKT cells could have the potential advantage that they will preserve the proliferation of conventional T cells, a property that could be critical to the development of anti-infectious and anti-tumoral responses; the latter effect is suggested by the preserved or enhanced GVL effect described in patients with enhanced post-transplant iNKT reconstitution.Citation15,Citation17
In conclusion, our data demonstrate that human CD4− iNKT cells better control the in vivo human allogeneic T cell responses compared to their CD4+ counterparts. These data are of major importance to guide expansions protocols and cellular target therapy in the prevention of human acute GVHD.
Material and methods
Human iNKT expansion
Human iNKT were expanded from peripheral blood mononuclear cells (PBMC) from healthy volunteers (Etablissement Français du Sang (EFS), agreement N°14/EFS/018) cultured with alpha-galactosylceramide (α-GalCer) 100 ng/mL, (KRN7000, Avanti Polar Lipids, Alabaster, AL, USA) and rhIL-2 (845 IU/mL, ImmunoTools, Friesoythe, Germany) for 15 days as described elsewhere.Citation28
Mice and xenogeneic mouse model of GVHD
NOD.Cg-PrkdcscidIl2rgtm1WjI/SzJ (NSG) mice, aged from 8 to 12 weeks, provided by Lucienne Chatenoud (INSERM U1013, Paris, France) were irradiated sub-lethally using 200-cGy total body irradiation by x-ray (RS 2000, Rad Source) on day−1, followed on day 0, by the intravenous infusion of 3 × 106 PBS57 tetramer negative PBMCs. Some mice were supplemented with ex vivo expanded and flow sorted 2.5 × 105 CD4+ or CD4− iNKT cells from the same donor.
Mice were evaluated in a blinded fashion, twice a week, for survival and GVHD clinical score as reported elsewhere.Citation41 According to our local Ethical Committee, mice were euthanized when achieving critical signs (weight loss above 20% and/or critical clinical signs).
In some experiments, 100 μL of peripheral blood was drawn weekly from the mandibular vein to assess engraftment of human cells. Plasma was stored at −80°C until analysis. All protocols were approved by the local Ethical Committee (CEEA34.0AP.018.11).
Histology
Pathological GVHD assessment was performed 4 weeks following human PBMC injection. Liver, small intestine and skin tissues were placed in 10% formalin, embedded in paraffin, sectioned and stained with hematoxylin–eosin–safran (HES). Anti-hCD3 (eBioscience, Paris, France) staining was performed when appropriate. GVHD pathological scoring was performed under blinded conditions as described elsewhere.Citation41 Photographs were taken using Å ~ 400 magnification with a Leica DF295 camera mounted on a Leica DMLB microscope.
Generation of mouse bone marrow derived dendritic cells (MoBMDCs)
Bone marrow cells of NSG mice were plated at a density of 1 × 10e6/mL in complete IMDM medium (10% FBS 50 IU/mL penicillin, 50 IU/mL streptomycin, 1% HEPES, 5 × 10−5M β-mercaptoethanol) supplemented with GM-CSF obtained from J558 hybridoma. After 3 days of culture, 75% of the media and non-adherent cells were removed and complete IMDM media supplemented with GM-CSF was added back. At day 7, all the media was gently removed, and adherent MoBMDCs were dislodged out of the culture plate by up and down pipetting with frozen 3mM EDTA in PBS. MoBMDC were assessed by FACS for their mCD11chigh expression.
Image-stream flow cytometry (AMNIS) and degranulation assay
MoBMDCs (50 000 per well) stained with anti-mCD11c mAb were cultured with CD4− iNKT or CD4+ iNKT sorted cells or a mixture of the two subsets (50% CD4+ and 50% CD4− iNKT) at a iNKT4+:iNKT4−:DC ratio of 2:2:1 in 100 μL of complete RPMI medium supplemented with anti-hCD107a-PE mAb (eBiosciences, Paris, France) or the corresponding IgG1 isotype control and Monensin A. Cultures were performed in a 96 round bottom well plate. After 4 hours of culture, cells were fixed by ethanol free 4% PFA, washed and stained with Hoechst 33342 (Invitrogen, Life Technologies, Villebon sur Yvette, France). Cells were run on an Imagestream ISX mkII (Amnis Corp. Millipore, Seattle, WA, USA) and a 60X magnification was used for all acquisitions. Data were acquired using the INSPIRE software (Amnis Corp) and analyzed using the IDEAS™ software (version 6.2 Amnis Corp). Approximately 10,000 events were collected in all experiments.
Cytokine concentrations
The concentrations of human cytokines in mouse plasma were determined using a customized Bio-Plex Pro Human Cytokine 17-plex Assay (Bio-Rad Laboratories Inc, France). Results were analyzed on a Bio-Plex200 Instrument (Luminex xMap Technology).
Flow cytometry and cell sorting
For ex vivo mouse DC analysis, splenocytes were obtained by pressing the spleen through a 100μm strainer with 120UI/mL DNase I treatment (Sigma-Aldrich, Saint-Quentin Fallavier, France). All ex vivo obtained cells were pre-incubated with CD16/CD32 mAb (clone 2.4G2) to block Fcγ receptors (eBioscience, Paris, France). Mouse CD11c+ DCs were analyzed in the human CD45− gate. For ex vivo intracellular cytokine staining, mice blood samples were first incubated in 1X Red cells Lysis buffer (BD Bioscience, Le Pont de Claix, France), and cells were stimulated for 4 h in complete RPMI medium (RPMI-1640 medium, 10% fetal bovine serum (FBS) 100 IU/mL penicillin, 100 IU/mL streptomycin, 200 mmol L-Glutamine, 10 mMol HEPES, all from Life Technologies, Villebon-sur-Yvette, France) in the presence of 50 ng/ml phorbol 12-myristate 13-acetate (PMA), 500 ng/ml ionomycin and 10 μg brefeldin A (all from Sigma-Aldrich, Saint-Quentin Fallavier, France). Intracellular staining was performed on fixed/permeabilized cells (Fix/Perm solution, eBioscience, Paris, France) according to manufacturer’s instructions. Human cells were gated according to human CD45+ staining.
Apoptosis was assessed using Propidium Iodide (PI) and Annexin V staining in 1X Annexin V buffer (eBioscience, Paris, France). BrdU staining was done on spleen cells according to manufacturer’s instructions (BrdU staining proliferation kit, BD Bioscience, Le Pont de Claix, France).
Monoclonal antibodies used for cell staining are listed in Table S1. Cells were analyzed on a FACS Canto II (BD Biosciences, Le Pont de Claix, France), coupled with FlowJo software vX.0.7 (Tree Star Inc. San Diego, CA, USA). Cell sorting was performed on BD FACS Aria (BD Bioscience, Le Pont de Claix, France).
Generation of human monocyte-derived DC
Monocytes were isolated from human PBMC by CD14 positive magnetic cell sorting (Miltenyi Biotec Monocyte Isolation Kit I, Miltenyi Biotec SAS, Paris, France). Immature DC were obtained by culturing monocytes in supplemented complete RPMI medium in the presence of GM-CSF (1000 IU/ml) and IL-4 (500 IU/ml, all from RD Systems, Lille, France) for 6 days. Mature DC were obtained by addition of 10 ng/mL prostaglandine E2 (Sigma-Aldrich, Saint-Quentin Fallavier, France) + 1000 UI/mL TNFα (RD Systems, Lille, France) for 24 hours.
iNKT-DC cultures
In the maturation assays, unrelated CD4+ or CD4− iNKT were cultured at 1:1 ratio with immature DCs, in contact or in transwell chambers (Costar, NY). In the apoptosis assays, unrelated iNKT CD4+ or CD4− were added to mature DCs at various iNKT/DC ratios. PI and Annexin V staining was performed after 4 to 24 hours of co-culture according to manufacturer’s instructions (eBioscience, Paris, France). DCs were analyzed in the PBS57 tetramer negative gate.
Statistical analysis
Comparison tests were performed using analysis of variance for repeated measurements followed by Student’s t test or Fisher’s exact test when appropriate. Survival curves were compared by log-rank test. The results are expressed as the mean ± SEM. The p-values were identified as follows: *, P < 0.05; **, P < 0.01; ***, P < 0.001. Statistical analyses were performed using Prism version 5.01 (GraphPad software)
Authorship contributions
TC, MTR, OH designed the experiments, interpreted the data and wrote the manuscript.
TC, JR and MD performed the experiments.
MD performed the confocal analyses.
BF performed the histopathology analyses.
RR and MB provided technical support.
JB, FC and IM provided a critical review of the data and manuscript.
All authors approved the manuscript.
Disclosures of Conflicts of interests
The authors declare no financial conflict of interest.
Supplementary matierials for this article can be accessed here
Supplementary_files.zip
Download Zip (345.4 KB)Acknowledgments
We are grateful to the NIH Tetramer Core Facility for providing CD1d tetramer reagents. We thank Lucienne Chatenoud (INSERM U1013, Paris, France) for providing NSG mice and Ronjon Chakraverty (Institute of Immunity and Transplantation and Cancer Institute, London, UK) for his critical review of the manuscript.
Additional information
Funding
References
- Ferrara JLM, Levine JE, Reddy P, Holler E. Graft-versus-host disease. Lancet Lond Engl. 2009;373(9674):1550–1561. doi:10.1016/S0140-6736(09)60237-3.
- Ferrara JLM, Reddy P. Pathophysiology of graft-versus-host disease. Semin Hematol. 2006;43(1):3–10. doi:10.1053/j.seminhematol.2005.09.001.
- Henden AS, Hill GR. Cytokines in graft-versus-host disease. J Immunol Baltim Md 1950. 2015;194(10):4604–4612.
- Di Ianni M, Falzetti F, Carotti A, Terenzi A, Castellino F, Bonifacio E, Del Papa B, Zei T, Ostini RI, Cecchini D, et al. Tregs prevent GVHD and promote immune reconstitution in HLA-haploidentical transplantation. Blood. 2011;117(14):3921–3928. doi:10.1182/blood-2010-10-311894.
- Hicheri Y, Bouchekioua A, Hamel Y, Henry A, Rouard H, Pautas C, Beaumont JL, Kuentz M, Cordonnier C, Cohen JL, et al. Donor regulatory T cells identified by FoxP3 expression but also by the membranous CD4+CD127low/neg phenotype influence graft-versus-tumor effect after donor lymphocyte infusion. J Immunother Hagerstown Md 1997. 2008;31(9):806–811. doi:10.1097/CJI.0b013e318184908d
- Maury S, Lemoine FM, Hicheri Y, Rosenzwajg M, Badoual C, Cherai M, Beaumont J-L, Azar N, Dhedin N, Sirvent A, et al. CD4+CD25+ regulatory T cell depletion improves the graft-versus-tumor effect of donor lymphocytes after allogeneic hematopoietic stem cell transplantation. Sci Transl Med. 2010;2(41):41ra52. doi:10.1126/scitranslmed.3001302.
- Bendelac A, Savage PB, Teyton L. The biology of NKT cells. Annu Rev Immunol. 2007;25:297–336. doi:10.1146/annurev.immunol.25.022106.141711.
- Matsuda JL, Mallevaey T, Scott-Browne J, Gapin L. CD1d-restricted iNKT cells, the “Swiss-Army knife” of the immune system. Curr Opin Immunol. 2008;20(3):358–368. doi:10.1016/j.coi.2008.03.018.
- Kuwatani M, Ikarashi Y, Iizuka A, Kawakami C, Quinn G, Heike Y, Yoshida M, Asaka M, Takaue Y, Wakasugi H. Modulation of acute graft-versus-host disease and chimerism after adoptive transfer of in vitro-expanded invariant Vα14 natural killer T cells. Immunol Lett. 2006;106(1):82–90. doi:10.1016/j.imlet.2006.05.001.
- Morris ES, MacDonald KPA, Rowe V, Banovic T, Kuns RD, Don AL, Bofinger HM, Burman AC, Olver SD, Kienzle N, et al. NKT cell-dependent leukemia eradication following stem cell mobilization with potent G-CSF analogs. J Clin Invest. 2005;115(11):3093–3103. doi:10.1172/JCI25249. Epub 2005 Oct 13.
- Yang J, Gao L, Liu Y, Ren Y, Xie R, Fan H, Qian K. Adoptive therapy by transfusing expanded donor murine natural killer T cells can suppress acute graft-versus-host disease in allogeneic bone marrow transplantation. Transfusion (Paris). 2010;50(2):407–417. doi:10.1111/trf.2010.50.issue-2.
- Pillai AB, George TI, Dutt S, Teo P, Strober S. Host NKT cells can prevent graft-versus-host disease and permit graft antitumor activity after bone marrow transplantation. J Immunol Baltim Md 1950. 2007;178(10):6242–6251.
- Kuns RD, Morris ES, Macdonald KPA, Markey KA, Morris HM, Raffelt NC, Banovic T, Don ALJ, Rowe V, Burman AC, et al. Invariant natural killer T cell-natural killer cell interactions dictate transplantation outcome after -galactosylceramide administration. Blood. 2009;113(23):5999–6010. doi:10.1182/blood-2008-10-183335.
- Leveson-Gower DB, Olson JA, Sega EI, Luong RH, Baker J, Zeiser R, Negrin RS. Low doses of natural killer T cells provide protection from acute graft-versus-host disease via an IL-4-dependent mechanism. Blood. 2011;117(11):3220–3229. doi:10.1182/blood-2010-08-303008. Epub 2011 Jan 21.
- Rubio M-T, Moreira-Teixeira L, Bachy E, Bouillie M, Milpied P, Coman T, Suarez F, Marcais A, Sibon D, Buzyn A, et al. Early posttransplantation donor-derived invariant natural killer T-cell recovery predicts the occurrence of acute graft-versus-host disease and overall survival. Blood. 2012;120(10):2144–2154. doi:10.1182/blood-2012-01-404673.
- Chaidos A, Patterson S, Szydlo R, Chaudhry MS, Dazzi F, Kanfer E, McDonald D, Marin D, Milojkovic D, Pavlu J, et al. Graft invariant natural killer T-cell dose predicts risk of acute graft-versus-host disease in allogeneic hematopoietic stem cell transplantation. Blood. 2012;119(21):5030–5036. doi:10.1182/blood-2011-11-389304.
- de Lalla C, Rinaldi A, Montagna D, Azzimonti L, Bernardo ME, Sangalli LM, Paganoni AM, Maccario R, Di Cesare-Merlone A, et al. Invariant NKT cell reconstitution in pediatric leukemia patients given HLA-haploidentical stem cell transplantation defines distinct CD4+ and CD4- subset dynamics and correlates with remission state. J Immunol Baltim Md 1950. 2011;186(7):4490–4499. doi:10.4049/jimmunol.1003748. Epub 2011 Feb 25.
- Beziat V, Nguyen S, Exley M, Achour A, Simon T, Chevallier P, Sirvent A, Vigouroux S, Debré P, Rio B, et al. Shaping of iNKT cell repertoire after unrelated cord blood transplantation. Clin Immunol Orlando Fla. 2010;135(3):364–373. doi:10.1016/j.clim.2010.01.010.
- Chan AC, Leeansyah E, Cochrane A, D’ Udekem D’ Acoz Y, Mittag D, Harrison LC, Godfrey DI, Berzins SP. Ex-vivo analysis of human Natural Killer T cells demonstrates heterogeneity between tissues and within established CD4+ and CD4− subsets. Clin Exp Immunol. 2013;172(1):129–137. doi:10.1111/cei.2013.172.issue-1.
- Berzins SP, Cochrane AD, Pellicci DG, Smyth MJ, Godfrey DI. Limited correlation between human thymus and blood NKT cell content revealed by an ontogeny study of paired tissue samples. Eur J Immunol. 2005;35(5):1399–1407. doi:10.1002/eji.200425958.
- Matsuda JL, Gapin L, Sidobre S, Kieper WC, Tan JT, Ceredig R, Surh CD, Kronenberg M. Homeostasis of Vα14i NKT cells. Nat Immunol. 2002;3(10):966–974. doi:10.1038/ni837.
- Coquet JM, Chakravarti S, Kyparissoudis K, McNab FW, Pitt LA, McKenzie BS, Berzins SP, Smyth MJ, Godfrey DI. Diverse cytokine production by NKT cell subsets and identification of an IL-17-producing CD4-NK1.1- NKT cell population. Proc Natl Acad Sci U S A. 2008;105(32):11287–11292. doi:10.1073/pnas.0801631105.
- Gumperz JE, Miyake S, Yamamura T, Brenner MB. Functionally distinct subsets of CD1d-restricted natural killer T cells revealed by CD1d tetramer staining. J Exp Med. 2002;195(5):625–636. doi:10.1084/jem.20011786.
- Lee PT, Benlagha K, Teyton L, Bendelac A. Distinct functional lineages of human Vα24 natural killer T cells. J Exp Med. 2002;195(5):637–641. doi:10.1084/jem.20011908.
- Pillai AB, George TI, Dutt S, Strober S. Host natural killer T cells induce an interleukin-4-dependent expansion of donor CD4+CD25+Foxp3+ T regulatory cells that protects against graft-versus-host disease. Blood. 2009;113(18):4458–4467. doi:10.1182/blood-2008-06-165506.
- Schneidawind D, Baker J, Pierini A, Buechele C, Luong RH, Meyer EH, Negrin RS. Third-party CD4+ invariant natural killer T cells protect from murine GVHD lethality. Blood. 2015;125(22):3491–3500. doi:10.1182/blood-2014-11-612762.
- Du J, Paz K, Thangavelu G, Schneidawind D, Baker J, Flynn R, Duramad O, Feser C, Panoskaltsis-Mortari A, Negrin RS, et al. Invariant natural killer T cells ameliorate murine chronic GVHD by expanding donor regulatory T cells. Blood. 2017;129(23):3121–3125. doi:10.1182/blood-2016-11-752444.
- Rubio M-T, Bouillie M, Bouazza N, Coman T, Trebeden-Nègre H, Gomez A, Suarez F, Sibon D, Brignier A, Paubelle E, et al. Pre-transplant donor CD4- invariant NKT cell expansion capacity predicts the occurrence of acute graft-versus-host disease. Leukemia. 2017 Apr;31(4):903–912. doi:10.1038/leu.2016.281. Epub 2016 Oct 14.
- Ito R, Katano I, Kawai K, Hirata H, Ogura T, Kamisako T, Eto T, Ito M. Highly sensitive model for xenogenic GVHD using severe immunodeficient NOG mice. Transplantation. 2009;87(11):1654–1658. doi:10.1097/TP.0b013e3181a5cb07.
- Brossay L, Kronenberg M. Highly conserved antigen-presenting function of CD1d molecules. Immunogenetics. 1999;50(3–4):146–151. doi:10.1007/s002510050590.
- Brossay L, Chioda M, Burdin N, Koezuka Y, Casorati G, Dellabona P, Kronenberg M. CD1d-mediated recognition of an α-galactosylceramide by natural killer T cells is highly conserved through mammalian evolution. J Exp Med. 1998;188(8):1521–1528. doi:10.1084/jem.188.8.1521.
- Liu T-Y, Uemura Y, Suzuki M, Narita Y, Hirata S, Ohyama H, Ishihara O, Matsushita S. Distinct subsets of human invariant NKT cells differentially regulate T helper responses via dendritic cells. Eur J Immunol. 2008;38(4):1012–1023. doi:10.1002/(ISSN)1521-4141.
- Chen Y-B, Efebera YA, Johnston L, Ball ED, Avigan D, Lekakis LJ, Bachier CR, Martin P, Duramad O, Ishii Y, et al. Increased Foxp3+Helios+ regulatory T cells and decreased acute graft-versus-host disease after allogeneic bone marrow transplantation in patients receiving sirolimus and RGI-2001, an activator of invariant natural killer T cells. Biol Blood Marrow Trans J Am Soc Blood Marrow Trans. 2017;23(4):625–634. doi:10.1016/j.bbmt.2017.01.069.
- Mavers M, Maas-Bauer K, Negrin RS. Invariant natural killer T cells as suppressors of graft-versus-host disease in allogeneic hematopoietic stem cell transplantation. Front Immunol. 2017;8:900. doi:10.3389/fimmu.2017.00900.
- Schneidawind D, Pierini A, Alvarez M, Pan Y, Baker J, Buechele C, Luong RH, Meyer EH, Negrin RS. CD4+ invariant natural killer T cells protect from murine GVHD lethality through expansion of donor CD4+CD25+FoxP3+ regulatory T cells. Blood. 2014;124(22):3320–3328. doi:10.1182/blood-2014-05-576017.
- Brok HP, Vossen JM, Heidt PJ. IFN-γ-mediated prevention of graft-versus-host disease: pharmacodynamic studies and influence on proliferative capacity of chimeric spleen cells. Bone Marrow Transplant. 1998;22(10):1005–1010. doi:10.1038/sj.bmt.1701478.
- Baker J, Verneris MR, Ito M, Shizuru JA, Negrin RS. Expansion of cytolytic CD8(+) natural killer T cells with limited capacity for graft-versus-host disease induction due to interferon gamma production. Blood. 2001;97(10):2923–2931. doi:10.1182/blood.V97.10.2923.
- Kim JH, Choi EY, Chung DH. Donor bone marrow type II (non-Valpha14Jalpha18 CD1d-restricted) NKT cells suppress graft-versus-host disease by producing IFN-gamma and IL-4. J Immunol Baltim Md 1950. 2007;179(10):6579–6587.
- Hannon M, Lechanteur C, Lucas S, Somja J, Seidel L, Belle L, Bruck F, Baudoux E, Giet O, Chantillon AM,et al. Infusion of clinical-grade enriched regulatory T cells delays experimental xenogeneic graft-versus-host disease. Transfusion (Paris). 2014;54(2):353–363. doi:10.1111/trf.12279. Epub 2013 Jun 17
- Hippen KL, Merkel SC, Schirm DK, Nelson C, Tennis NC, Riley JL, June CH, Miller JS, Wagner JE, Blazar BR. Generation and large-scale expansion of human inducible regulatory T cells that suppress graft-versus-host disease. Am J Transpl Off J Am Soc Transpl Am Soc Transpl Surg. 2011;11(6):1148–1157. doi:10.1111/j.1600-6143.2011.03558.x.
- D’Aveni M, Rossignol J, Coman T, et al. G-CSF mobilizes CD34+ regulatory monocytes that inhibit graft-versus-host disease. Sci Transl Med. 2015;7(281):281ra42. doi:10.1126/scitranslmed.3010435.