ABSTRACT
Nearly half of melanoma patients develop brain metastases during the course of their disease. Despite advances in both localized radiation and systemic immunotherapy, brain metastases remain difficult to treat, with most patients surviving less than 5 months from the time of diagnosis. While both treatment regimens have individually shown considerable promise in treating metastatic melanoma, there is interest in combining these strategies to take advantage of potential synergy. In order to study the ability of local radiation and anti-PD-1 immunotherapy to induce beneficial anti-tumor immune responses against distant, unirradiated tumors, we used two mouse models of metastatic melanoma in the brain, representing BRAF mutant and non-mutant tumors. Combination treatments produced a stronger systemic anti-tumor immune response than either treatment alone. This resulted in reduced tumor growth and larger numbers of activated, cytotoxic CD8+ T cells, even in the unirradiated tumor, indicative of an abscopal effect. The immune-mediated effects were present regardless of BRAF status. These data suggest that irradiation of brain metastases and anti-PD-1 immunotherapy together can induce abscopal anti-tumor responses that control both local and distant disease.
Introduction
Metastatic melanoma is a highly aggressive disease that is associated with poor survival.Citation1,Citation2 Brain metastases (BM) are common, with 20% of patients presenting and 50% developing them during the course of disease.Citation3-Citation5 Radiotherapy is a major treatment modality of melanoma, with successful control of local lesions often occurring in up to 80% of patients.Citation4,Citation6,Citation7 Despite successful control of localized disease, however, patients frequently develop metastases, including in the brain, that result in overall treatment failure.
Immunotherapies that boost the patients’ own anti-tumor immune responses have revolutionized the management of melanoma and show promise against both intra and extracranial melanoma.Citation8-Citation10 These findings are part of an evolving understanding of the brain as an immune-accessible tissue.Citation11,Citation12 Immune-checkpoint inhibitors, including antibody blockade of programmed death-1 (PD-1) signaling are now widely used against melanoma due to their efficacy in recent clinical trials.Citation13-Citation15 Anti-PD-1 treatment can result in dramatic successes in melanoma patients, with response rates from 25 to 45%.Citation16-Citation22 Anti-PD-1 treatment has also demonstrated efficacy in other forms of cancer including bladder, colorectal, and certain hematopoietic malignancies.Citation23-Citation26 PD-1 expression is induced on T cells in response to signals in the tumor microenvironment including chronic antigen exposure. Signaling through PD-1 delivers negative signals to T cells, which reduces cytotoxic function and contributes to immune tolerance.Citation27 Blockade of PD-1, therefore, prevents T cell inhibition and supports anti-tumor immune responses.Citation28,Citation29
In the current study, we used two models of mouse melanoma representing BRAF wildtype (wt) and mutant tumors to model the clinical management of cancer patients with brain metastases of these two major types of melanoma. One of the tumor models utilized B16-F10 cells, which develop aggressive tumors in vivo that are well-studied in pre-clinical models of immunotherapy, and which express wild-type BRAF.Citation30,Citation31 The other model made use of D4M cells, which were derived from a spontaneous melanoma induced in a transgenic mouse expressing mutant BRAF (V600E) combined with genetic knock-out of the tumor suppressor gene Pten.Citation32,Citation33 This actively transforming BRAFV600E mutation is a common feature of nearly 50% of human melanomas, and drives disease progression, highlighting the clinical relevance of this model.Citation34-Citation36
We used these cell lines in two models of metastatic melanoma. In one model, two contralateral flank tumors were established subcutaneously in mice. In subsequent experiments, tumor cells were injected into the brain and flanks of mice to model brain metastasis and extracranial disease. Mice were then treated with anti-PD-1 combined with localized radiation to the head (to target the brain tumor) or to one of the flank tumors (for the bilateral flank tumor model).Citation37-Citation40 In the bilateral flank tumor model, we found that the combination of irradiation and anti-PD-1 treatment resulted in the greatest survival of mice, and that even growth of the unirradiated flank tumor was significantly delayed in the group receiving combined irradiation and anti-PD-1. Strikingly, similar results were observed in models of brain metastasis using both B16-F10 and D4M tumor lines, in which the brain was irradiated during PD-1 treatment. To our knowledge, this is the first time that such response has been demonstrated in this kind of model.
We hypothesized that this delay was due to the enhancement of a systemic anti-tumor immune response which induced an abscopal effect on the flank tumor. Indeed, we found that the tumor tissue in the unirradiated flanks of anti-PD-1 treated mice, in both the head irradiation- and flank irradiation models, had significantly greater T cell infiltrates and expression of markers associated with cytotoxic immunity. These data demonstrate that anti-PD-1 therapy combined with irradiation of only a single metastatic lesion can result in a beneficial immune response that can affect unirradiated distal tumors. Collectively, our novel findings indicate that combined radiation and anti-PD-1 checkpoint blockade therapy can have beneficial effects on stimulating systemic anti-tumor immune responses to induce regression of distant tumors.
Results
Combination radiation and anti-PD-1 therapy induces a systemic anti-tumor response
The abscopal effect is a phenomenon in which the combination of systemic immunotherapy and irradiation of localized lesions causes a systemic anti-tumor immune response that affects the growth of distal, non-irradiated tumors. Though a rare but real occurrence in the clinic, this effect has been observed in a number of pre-clinical mouse models of melanoma.Citation41,Citation42 To determine the immunologic changes underlying this process, we began by performing similar studies with contralateral flank tumors to observe whether similar responses could be induced with both our D4M and B16 models of melanoma. In , B16 tumors were established in each flank of mice. Mice were then treated with anti-PD-1, and one of the tumors was irradiated (8 Gy/4 fractions). We chose this dosing schedule because previously published studies have indicated that fractionated radiation dosages induce greater immunity than single doses, and maximize abscopal effects.Citation43 Anti-PD-1 treatment continued once every 5 days for the duration of the experiment. As expected, the irradiated tumor exhibited decreased growth. Likewise, while anti-PD-1 and irradiation each resulted in a significant delay in growth, the combination of the two treatments resulted in the greatest effect in both the directly irradiated and distant, unirradiated tumors. In order to determine whether significantly delayed growth of non-irradiated, contralateral tumors was due to anti-tumor immune responses, tumor tissue was harvested from mice at the conclusion of the experiment, dissociated by digestion with collagenase and DNase, and stained for T cells and quantified by flow cytometry (). These studies indicated that delayed tumor growth was associated with an increased CD8+ T cell infiltrate, and correspondingly enhanced anti-tumor immune responses.Citation44,Citation45
Figure 1. Combination radiation and anti-PD-1 therapy induces an abscopal effect in contralateral flank tumors. A. 1.5 × 105 B16-F10 tumor cells were injected in to each flank of wildtype C57BL/6 mice. Nine days later, mice received 150 µg anti-PD-1 antibody or IgG via intraperitoneal injection. After five days, indicated mice received 8 Gy in 4 fractions to one flank tumor. Growth of the irradiated (left) and non-irradiated contralateral tumor (right) was followed for 20 days. B. At the conclusion of the experiment, tumor tissues were harvested and digested to a single cell suspension with collagenase/hyaluronidase. T cell phenotype and frequency was determined using staining for readout by flow cytometry. Indicated study is representative of the independent experiments. *P < 0.05 for the indicated comparisons at day 20 in A. and between anti-PD-1 and anti-PD-1 + head irradiation groups in B.
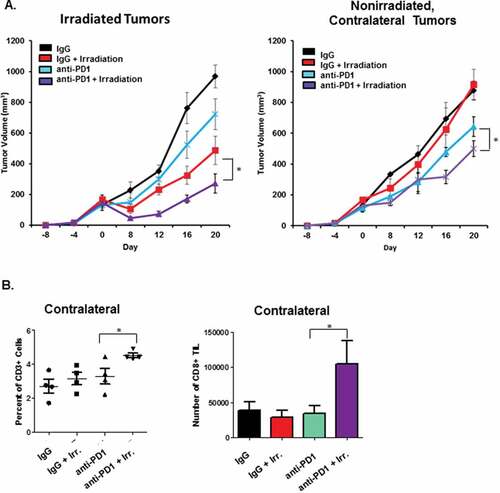
Combination anti-PD-1 and radiation induced an abscopal effect in a brain metastasis model of melanoma
Radiotherapy is frequently used for treatment of brain metastases. Given the ability of radiation to induce and enhance anti-tumor immune responses, we sought to combine radiation with immunotherapy to enhance systemic anti-tumor immune responses. D4M melanoma cells were injected both intracranially and in the flank of immune competent syngeneic mice. Fourteen days post-injection, mice were irradiated to the head (8 Gy in 4 fractions) after verifying by bioluminescence imaging that they had equally sized luminescent tumors. For mice receiving anti-PD-1, antibody treatment began five days prior to irradiation (summarized in ), as recent studies indicated that the optimal timing of checkpoint blockade is just prior to irradiation, presumably so that the antibody is present in the tissues at the time of therapy.Citation46-Citation48 Survival was followed over the next 25 days and is illustrated in . Animals were removed from the study when tumor growth or neurological complications exceeded veterinary guidelines. These data indicate that while radiation and anti-PD-1 were slightly beneficial alone, the combination had the greatest impact on animal survival with about 20% of the mice still being alive at day 25. To observe the effect of radiation and anti-PD-1 treatment on individual, we assessed their growth for 10 days following radiation using calipers (for flank tumors) or by bioluminescence imaging (for flanks and brain). Interestingly, the flank tumors on mice treated with anti-PD-1 and irradiation to the head were most growth inhibited, and progressed much less than the tumors on mice given either treatment alone, suggesting that the beneficial effects of combined therapy can be seen soon after radiation treatment ends (). We then followed growth of the flank tumor in all four treatment groups out over a longer period of time (20 days post radiation), and found that the group treated with both radiation and anti-PD-1 antibody continued to have a significant delay over either treatment alone. Of note, we found that head irradiation alone was not sufficient to have a long-term effect on growth of the flank tumor, which suggests that head irradiation served to enhance the effect of the anti-PD-1 treatment on the non-irradiated flank tumor ( and ). Similar results were observed using bioluminescence imaging of the flank tumors on Day 20. At this time point, we also observed that both radiation and anti-PD-1 treatment (and the combination) were sufficient to reduce the BM tumor to a near-undetectable levels when measured by bioluminescence imaging. ( and ).
Figure 2. Irradiation and PD-1 blockade therapy induce an additive effect in a model of metastatic melanoma of the brain. A. Graphical representation of the experimental setup and treatment schedule for head irradiation experiments. B. Mice receiving concomitant D4M tumors implanted intracranial and in the flank were treated with IgG or anti-PD-1 antibody followed by four doses of 2 Gy irradiation to the head according to the experimental plan in A. Mice were then followed for 25 days post irradiation, and survival is indicated by the Kaplan-Meier plot. C. Growth in flank tumor size as measured by volume for the indicated groups is represented. On day 20, IVIS bioluminescence imaging of the animals was performed and graphs represent the intensity of luminescence of flank tumors (D.) and brain tumors (E.). Representative bioluminescence images at this time point. (F.) *p < 0.05 and **p < 0.01 for comparisons between the indicated groups.
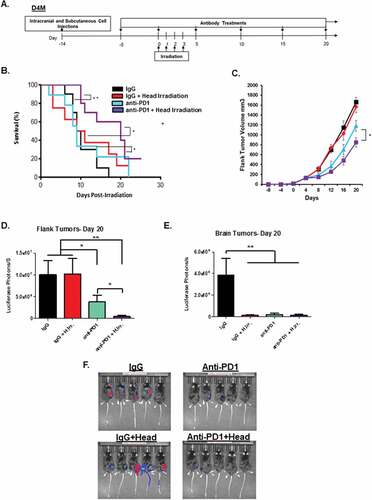
BM irradiation and anti-PD-1 therapy enhance anti-tumor immunity
Having observed that the combination of radiation and anti-PD-1 antibody could combine to significantly delay tumor growth in distant, non-irradiated D4M tumors we next sought to determine whether the tumor delay was associated with increased immune infiltrate into the tumor tissues. Immunofluorescence assays of brain tumors harvested 15 days after irradiation revealed significantly greater numbers of both CD8+ and CD4+ T cells in PD-1 treated groups and a significant increase in CD8+ T cells in anti-PD-1 plus irradiation treated mice over anti-PD-1 alone ( and ). At the conclusion of the experiment, flank tumors were harvested, dissociated by collagenase digestion and assayed for immune cell infiltrate by flow cytometry. These studies revealed that the number and percentage of CD4+ T cells was largely unchanged by either radiation or anti-PD-1 treatment or the combination (–). In contrast, we found significantly more CD8+ T cells in both frequency and number in the combination PD-1 antibody and irradiation treated group, and a modest increase in the anti-PD-1 alone group (–). We also assessed Ki-67 expression on tumor-resident T cells and found significantly higher expression on both CD4+ and CD8+ T cells in the combination treatment group (). When CD8+ TIL were re-stimulated ex vivo using anti-CD3/CD28, we found that T cells from anti-PD-1 alone treated tumors produced more interferon-γ (IFNγ) than either control IgG or radiation alone, but CD8+ T cells from combination-treated tumors produced dramatically more. Together these data indicate that the optimal delay in flank tumor growth observed in animals treated with both head irradiation and anti-PD-1 antibody we associated with an enhanced anti-tumor immune response that was mediated by CD8+ cytotoxic T cells (). In addition to increased IFNγ, we also found significantly greater t-bet expression in anti-PD-1 combination groups as compared to IgG alone, and a trend toward greater granzyme B expression in these T cells (). Lastly, we assessed the effects of radiation, anti-PD-1 antibody treatments or the combination on other common regulatory immune cells in the tumor microenvironment. The tumor microenvironment has been demonstrated to contain significantly high numbers of cells like CD4+ regulatory T cells (Treg) and myeloid-derived suppressor cells (MDSC), and their presence is associated with immunosuppression and poor anti-tumor immune responses.Citation49,Citation50 In our model of irradiation plus anti-PD-1 therapy of BM, we found that while anti-PD-1 treatment alone was able to slightly decrease then percentage of CD4+ Treg in the flank tumor, the combination of PD-1 plus head irradiation resulted in the greatest, most significant decrease. () This decrease was also observed in the number of these cells (). We then assessed the ratio of the number of CD4+ Treg and CD8+ T cells in each treatment group and found that the combination therapy group had significantly larger ratio of CD8+ T cells to Treg (). Lastly, we assessed the percentage of GR1+ CD11b+ MDSC among CD45+ cells in the tumor and found that while both anti-PD-1 alone and combination treatment resulted in a slight increase in MDSC, these differences were not statistically significant from IgG treated groups (). This result suggests that anti-PD-1 treatment alone may not affect MDSC cell frequency, since these cells express the ligand of PD-1, PD-1L. Overall, these data suggest that the reduced tumor growth effects of combination anti-PD-1 therapy and irradiation reduce tumor growth by inducing a stronger CD8+ cytotoxic T cell response directed against the non-irradiated tumor.
Figure 3. Abscopal effects induced by radiation and anti-PD1 combination therapy are associated with increased anti-tumor immunity. A. At the conclusion of the previous experiment, brain tissue was sectioned and T cell markers stained for immunofluorescence microscopy. B. Quantification of the number of cells in at least four independent fields for each treatment group. C. Flank tumor tissue was harvested and dissociated by collagenase/hyaluronidase digestion and immune cell markers were stained for readout and quantification by flow cytometry. Ki-67 expression was assessed on the indicated T cell subsets by permeabilization and staining followed by readout by flow cytometry. D-I. T cell subset percentages in non-irradiated flank tumor tissue were compared to total cell recovery to determine total number of T cells and ratio of CD8T cells to regulatory CD4T cells (Treg). CD4+/CD8+ percentages in D. and G. are of total CD45+ cells. J. CD8+ T cells from non-irradiated flank tumors were restimluated ex vivo with plate-bound anti-CD3 and anti-CD28 antibodies followed by intracellular cytokine staining for interferon-gamma (IFNγ) staining. K. Expression of the indicated markers of cytotoxic T cell function on CD8+ T cells from non-irradiated flank tumor tissue were stained following permeabilization and read-out by flow cytometry. *p ≤ 0.5 and **p ≤ 0.01 for indicated comparisons between anti-PD-1 and anti-PD-1 plus head irradiation treatment groups according to a student’s t test.
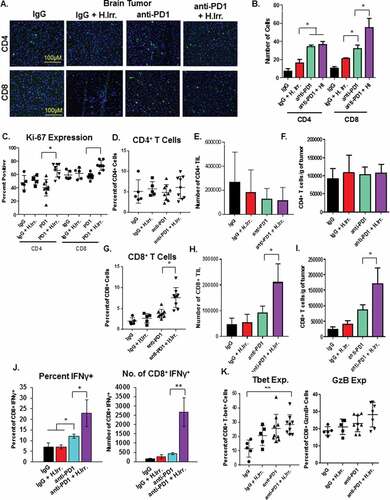
Figure 4. Effect of combination BM irradiation and PD-1 treatment on regulatory immune cell populations in distant flank tumors. A. Tissue from flank D4M tumors were harvested at the conclusion of the experiment described in , dissociated by collagenase/hyaluronidase digestion, and stained for regulatory T cell markers before being quantified by flow cytometry. Regulatory T cells were defined as CD25hi Foxp3+ cells among CD3+ CD4+ T cells. B. Based on the percentages of the flow stain, the numbers of Treg per tumor and the ratio of these cells to the number of CD8+ T cells was determined (B, C). D. The percentage of myeloid derived suppressor cells (MDSCs) was determined in the tumors by anti-body staining and flow cytometry. Cells were gated on CD45+ Gr1+ CD11b+ populations. *p ≤ 0.5 for the indicated comparisons to the anti-PD-1 plus irradiation group.
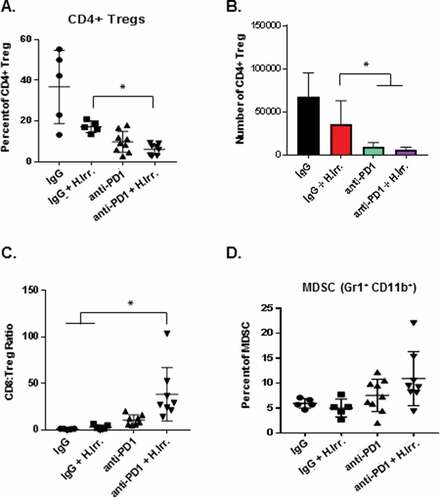
Combination therapy induces an abscopal effect in multiple melanoma models
We next extended our model of immunotherapy of metastatic melanoma of the brain and flank with using the B16 model of melanoma which expresses wildtype BRAF. Tumors were established intracranially and subcutaneously in the flank similar to those using D4M cells. Likewise, anti-PD-1 antibody or IgG control treatment began five days before radiotherapy, which followed the same dosing schedule used previously (4 fractions of 2 Gy each). Following brain tumor growth by bioluminescence imaging, we found that, over the course of the first 10 days following treatment, that radiation or anti-PD-1 or the combination was able to modestly slow tumor growth as compared to IgG alone control, with only slight differences between anti-PD-1 and combination groups (). When we assessed growth of the flank tumors by bioluminescence imaging over the course of the first 10 days post-radiation, we found that the combination treatment had the greatest effect on tumor growth, which was significantly less than both the anti-PD-1 alone and radiation plus IgG control groups; a similar finding to what we observed using the D4M model. Consistent with the D4M model, the effects of combination treatment on flank tumor growth were visible over the duration of the experiment ( and ). These data from 2 different mouse models suggest that the benefit of combination therapy is independent of BRAF mutational status and may be generalizable. Lastly, to verify that the delay in tumor growth observed in treatment groups was due to enhanced anti-tumor immune responses, we assessed the frequency and number of CD8+ T cells present in the flank tumors at the conclusion of the experiment by dissociation in collagenase followed by flow cytometry. Similar to our previous D4M studies, anti-PD-1 treated groups had dramatically greater frequency and numbers of CD8+ T cells present in the tumor tissue, while the combination head irradiation and anti-PD-1 treatment group had significantly greater T cell infiltrate than anti-PD-1 alone (). Together these data confirm our observation that the radiation of a distant melanoma tumor in the brain in combination with systemic anti-PD-1 therapy can enhance a systemic anti-tumor T cell response that can have beneficial effects on other metastatic tumors in the same animal.
Figure 5. Anti-PD-1 treatment and irradiation induces an abscopal effect in B16 tumors. B16 tumors were established intracranially and subcutaneously in the flank similar to those using D4M cells. Brain (A.) and flank (B.) tumor growth was followed by bioluminescence imaging and graphs represent percent in growth. C. D. At the conclusion of the experiment, tumors were harvested and dissociated by collagenase/hyaluronidase digestion followed by staining for CD8+ T cell markers. Cells were then read-out and quantified by flow cytometry. *p < 0.5 and **p < 0.01 for the indicated comparisons between treatment groups. For the tumor growth graph. *p < 0.05 for the combination group vs. others at the final time point using ANOVA analysis.
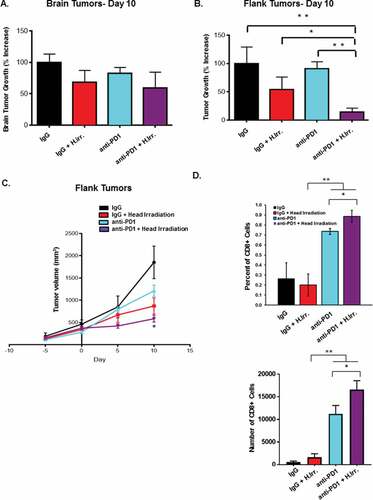
Discussion
In the present study we report that anti-PD-1 antibody therapy in combination with radiation of mouse intracranial brain melanoma tumors can induce an abscopal effect slowing the growth of a non-irradiated flank tumor in the same animal that is greater than the use of the antibody alone. Consistent with clinical observations, anti-PD-1 therapy alone can significantly inhibit tumor growth in our model, but when combined with radiotherapy of the intracranial tumor, anti-PD-1 dramatically enhances the anti-tumor immune response against the unirradiated flank tumor as well. Likewise, we found that radiation alone was unable to induce any measurable change in the size or immune composition of non-irradiated flank tumors; however the combined radiation and anti-PD-1 therapies induced systemic immune responses in nearly every animal. These results are similar to those reported for other, recent preclinical studies that used anti-PD-1 (as well as other checkpoint blockade antibodies), though ours is the first to include irradiation of melanoma brain tumors specifically.Citation43,Citation51-Citation53
The phenomenon of the radiation-induced abscopal effect was first described by Mole in 1953, but this rarely occurs in the clinic when using only routine radiotherapy regimens.Citation54 Increasing appreciation that these occasional tumor regressions were likely immune-mediated, together with recent insights into how irradiation actually enhances the immunogenicity of tumor tissue, has renewed interest in using immunotherapies to amplify these responses. In fact, immune involvement is increasingly appreciated as a major mechanism of radiotherapy efficacy.Citation55 For instance, radiation-induced cell death has been shown to release proteins such as HMGB1 that can act as immunological danger signals that can enhance anti-tumor T cell responses.Citation56 Indeed, clinical studies have found a positive correlation between serum levels of these danger signal proteins and overall survival.Citation57
The brain and central nervous system have traditionally been considered immune-privileges sites based on the ability of the blood brain barrier to largely inhibit the influx of both immune effectors and antigen presenting cellsCitation58. More recent studies, however, indicate that both antigen-presenting cells and activated T cells are more adept at infiltrating central nervous tissues than previously thought.Citation11,Citation12,Citation59-Citation61 Further, BM were shown to contain large numbers of T cells and the extent of T cell infiltration correlates with survival prognosis.Citation62,Citation63 Pre-clinical animal data have also demonstrated that radiation can increase the permeability of the blood-brain barrier.Citation64 Further, immunoglobulins such as antibodies administered as part of immunotherapy were found to penetrate the blood brain barrier into nerve and brain tissue, where they exert their beneficial effects on tissue-resident T cells, particularly after radiotherapy.Citation65 These observations influenced our decision to initiate anti-PD-1 administration prior to radiation treatment, and suggest that optimal clinical use of these treatments should follow similar timing schedules.
The use of radiation for the treatment of brain metastases in melanoma is an increasingly common procedure with stereotactic techniques that have high rates of local control up to 80% and have fewer complications compared to whole-brain irradiation.Citation66,Citation67 Despite these local successes, disease progression often occurs in distant metastases, which underscores the need for combinatorial strategies that have systemic effects, including immunotherapy. Recent, promising retrospective studies found that the combination of radiosurgery with ipilimumab (anti-CTLA-4, a related immune checkpoint signaling protein) raised the median survival of metastatic melanoma patients with brain metastases from 4.9 to 21 months, though these studies could only speculate about immune involvement in this phenomenon.Citation68-Citation70 Whether the positive benefits of combining radiation of brain metastases with immunotherapy were true synergistic effects or worked separately was not addressed by these studies. The animal model data in our current study indicate that these two treatment strategies work together.
Lastly, the applicability of this treatment strategy to both BRAF mutant and wildtype forms of melanoma is also notable in that non-mutated BRAF tumors have fewer options for targeted therapy (due to the absence of mutant BRAF), making anti-PD-1 and radiation combination therapy an important strategy to treat both kinds of melanoma together. In conclusion, our findings highlight the benefit of combining immune checkpoint blockade of the PD-1/PDL-1 signaling axis with radiotherapy of BM for treating not just localized tumors in the brain, but also of distant metastases via induction of an abscopal effect. Our data further suggest that these two increasingly common treatment modalities for metastatic melanoma would have synergistic effects in the clinic, and provides the basis for the use of other combinations of radiation and immunotherapy in future treatment strategies.
Materials and methods
Cell lines
The B16-F10 tumor cell line has been previously described and was obtained from the American Type Culture Collection. D4M melanoma cells have been previously described and were a generous gift of Dr. Molly Jenkins (Dartmouth University).Citation32 Both cell lines were transduced to express firefly luciferase as previously described.Citation71 All cells were maintained in RPMI with 10% Fetal Bovine Serum, 10 mmol/L HEPES, 1 mmol/L sodium pyruvate, 2 mmol/L nonessential amino acids and 100 u/ml penicillin/streptomycin in an incubator containing 10% CO2. 1 μg/ml puromycin was used to select for transduced cells.
Mouse tumor implantation, irradiation, and TIL isolation
C57BL/6 mice were obtained from Jackson Laboratories (Bar Harbor, ME). For tumor growth experiments, 150,000 B16 or D4M tumor cells were implanted subcutaneously in 100 µl sterile saline and tumor growth was monitored by measuring tumor diameter with calipers every third day for the duration of the experiment. For intracranial tumors 2 × 104 tumor cells were injected as previously described.Citation40 Briefly, cells were implanted into the right frontal lobes of wildtype C57BL/6 mice. For bioluminescence imaging, mice were given d-luciferase (Promega) via i.p. injection before imaging using an IVIS imaging system. Animals were immobilized with isoflurane during this procedure. For antibody-treatment, anti-PD-1 antibody or an IgG control were injected at 150 μg/dose i.p. starting five days before irradiation and continuing every fifth day for the duration of the experiment. For irradiation, animals were immobilized via a single injection of ketamine and xylazine and irradiation was delivered to the mouse head or flank tumor using a Pantak X-ray irradiator. Lead shielding was used to limit radiation exposure to other areas of the body. Four 2 Gy doses were administered daily for a total radiation dose of 8 Gy. Tumor-infiltrating lymphocytes were isolated by disruption of tumor tissue first by mincing with crossed scalpels under sterile conditions followed by enzymatic digestion as described above. Live cells were isolated from debris by centrifugation over a Ficoll gradient prior to staining for flow cytometry or cryopreservation for further analysis. All animals were housed in the Biological Resources Unit of the Cleveland Clinic Lerner Research Institute according to Office of Laboratory Animal Welfare guidelines and experiments were conducted under an approved Institutional Animal Care and Use Committee protocol.
Antibodies, immunofluorescence, and flow cytometry
Fluorescently conjugated, anti-mouse antibodies were purchased from Biolegend (San Diego, CA) (CD3-PerCP, CD8 FITC, CD8 APC, PD-1 FITC, CD45 AlexaFluor700, Ki-67 APC or PerCP Cy5.5, CD25 FITC, Foxp3 PE, Gr1 APC, CD11b AlexaFlour 647, Interferon-γ PE, Granzyme B APC, Tbet APC). Prior to staining, all cells were treated with anti-FcγIII/CD16 antibody from Biolegend according to the manufacturer’s recommended protocol (human/mouse TrueStain FCX). Antibody staining was performed in phosphate-buffered saline with 0.1% fetal bovine serum or bovine serum albumin. Data were collected on FACS Calibur or LSR II instruments and analyzed with the FlowJo data analysis software (FlowJo Inc, Salem OR). Anti-PD-1 antibody (mDX400) used in vivo was and the IgG control were provided by Merck & Company via a Materials Transfer Agreement and has been described previously.Citation72 Immunofluorescent staining of cells and tissues sections was performed as described.Citation73 Quantification of immunofluorescence images was performed using ImageJ software (https://imagej.nih.gov/ij/).
Statistics
Means of all groups were compared for statistical differences by Student’s t test or a One-Way Analysis of Variance (ANOVA). A Bonferroni t test was used, following the ANOVA, to understand the statistical difference between two groups, when more than two groups were compared. Data was presented as means ±SD. Significance levels were set to p < 0.05.
Disclosure statement
Merck & Co., Inc., Kenilworth, NJ USA provided anti-PD-1 antibody and financial support for the study.
References
- Spagnolo F, Picasso V, Lambertini M, Ottaviano V, Dozin B, Queirolo P. Survival of patients with metastatic melanoma and brain metastases in the era of MAP-kinase inhibitors and immunologic checkpoint blockade antibodies: A systematic review. Cancer Treat Rev. 2016;45:38–45. doi:10.1016/j.ctrv.2016.03.003.
- Davies MA, Liu P, McIntyre S, Kim KB, Papadopoulos N, Hwu W-J, Hwu P, Bedikian A. Prognostic factors for survival in melanoma patients with brain metastases. Cancer. 2011;117:1687–1696. doi:10.1002/cncr.25634.
- Bafaloukos D, Gogas H. The treatment of brain metastases in melanoma patients. Cancer Treat Rev. 2004;30:515–520. doi:10.1016/j.ctrv.2004.05.001.
- Ahmed KA, Stallworth DG, Kim Y, Johnstone PA, Harrison LB, Caudell JJ, Yu HHM, Etame AB, Weber JS, Gibney GT. Clinical outcomes of melanoma brain metastases treated with stereotactic radiation and anti-PD-1 therapy. Ann Oncol. 2016;27:434–441. doi:10.1093/annonc/mdv622.
- Gibney GT, Forsyth PA, Sondak VK. Melanoma in the brain: biology and therapeutic options. Melanoma Res. 2012;22:177–183. doi:10.1097/CMR.0b013e328352dbef.
- Yu C, Chen JCT, Apuzzo MLJ, O’Day S, Giannotta SL, Weber JS, Petrovich Z. Metastatic melanoma to the brain: prognostic factors after gamma knife radiosurgery. Int J Radiat Oncol Biol Phys. 2002;52:1277–1287.
- Ramakrishna N, Margolin KA. Multidisciplinary approach to brain metastasis from melanoma; local therapies for central nervous system metastases. Am Soc Clin Oncol Educ Book. 2013;33:399–403. doi:10.1200/EdBook_AM.2013.33.399.
- O’Reilly A, Larkin J. Checkpoint inhibitors in advanced melanoma: effect on the field of immunotherapy. Expert Rev Anticancer Ther. 2017;7:1–9.
- Roth P, Preusser M, Weller M. Immunotherapy of brain cancer. Oncol Res Treat. 2016;39:326–334. doi:10.1159/000446338.
- Emens LA, Ascierto PA, Darcy PK, Demaria S, Eggermont AMM, Redmond WL, Seliger B, Marincola FM. Cancer immunotherapy: opportunities and challenges in the rapidly evolving clinical landscape. Eur J Cancer. 2017;81:116–129. doi:10.1016/j.ejca.2017.01.035.
- Prins RM, Vo DD, Khan-Farooqi H, Yang M-Y, Soto H, Economou JS, Liau LM, Ribas A. NK and CD4 cells collaborate to protect against melanoma tumor formation in the brain. J Immunol. 2006;177:8448–8455.
- Anandasabapathy N, Victora GD, Meredith M, Feder R, Dong B, Kluger C, Yao K, Dustin ML, Nussenzweig MC, Steinman RM, et al. Flt3L controls the development of radiosensitive dendritic cells in the meninges and choroid plexus of the steady-state mouse brain. J Exp Med. 2011;208:1695–1705. doi:10.1084/jem.20102657.
- Ribas A, Puzanov I, Dummer R, Schadendorf D, Hamid O, Robert C, Hodi FS, Schachter J, Pavlick AC, Lewis KD, et al. Pembrolizumab versus investigator-choice chemotherapy for ipilimumab-refractory melanoma (KEYNOTE-002): a randomised, controlled, phase 2 trial. Lancet Oncol. 2015;16:908–918. doi:10.1016/S1470-2045(15)00083-2.
- Specenier P. Pembrolizumab use for the treatment of advanced melanoma. Expert Opin Biol Ther. 2017;17:765–780. doi:10.1080/14712598.2017.1309388.
- Betof AS, Nipp RD, Giobbie-Hurder A, Johnpulle RAN, Rubin K, Rubinstein SM, Flaherty KT, Lawrence DP, Johnson DB, Sullivan RJ. Impact of age on outcomes with immunotherapy for patients with melanoma. Oncologist. 2017;22:1–8. doi:10.1634/theoncologist.2017-0001.
- Topalian SL, Hodi FS, Brahmer JR, Gettinger SN, Smith DC, McDermott DF, Powderly JD, Carvajal RD, Sosman JA, Atkins MB, et al. Safety, activity, and immune correlates of anti-PD-1 antibody in cancer. N Engl J Med. 2012;366:2443–2454. doi:10.1056/NEJMoa1200690.
- Vaillant F, Merino D, Lee L, Breslin K, Pal B, Ritchie ME, Smyth GK, Christie M, Phillipson LJ, Burns CJ, et al. Targeting BCL-2 with the BH3 mimetic ABT-199 in estrogen receptor-positive breast cancer. Cancer Cell. 2013;24:120–129. doi:10.1016/j.ccr.2013.06.002.
- Zou W, Wolchok JD, Chen L. PD-L1 (B7-H1) and PD-1 pathway blockade for cancer therapy: mechanisms, response biomarkers, and combinations. Sci Transl Med. 2016;8:328rv4. doi:10.1126/scitranslmed.aaf0746.
- Larkin J, Chiarion-Sileni V, Gonzalez R, Grob JJ, Cowey CL, Lao CD, Schadendorf D, Dummer R, Smylie M, Rutkowski P, et al. Combined nivolumab and ipilimumab or monotherapy in untreated melanoma. N Engl J Med. 2015;373:23–34. doi:10.1056/NEJMoa1504030.
- Robert C, Long GV, Brady B, Dutriaux C, Maio M, Mortier L, Hassel JC, Rutkowski P, McNeil C, Kalinka-Warzocha E, et al. Nivolumab in previously untreated melanoma without BRAF mutation. N Engl J Med. 2015;372:320–330. doi:10.1056/NEJMoa1412082.
- Topalian SL, Sznol M, McDermott DF, Kluger HM, Carvajal RD, Sharfman WH, Brahmer JR, Lawrence DP, Atkins MB, Powderly JD, et al. Survival, durable tumor remission, and long-term safety in patients with advanced melanoma receiving nivolumab. J Clin Oncol. 2014;32:1020–1030. doi:10.1200/JCO.2013.53.0105.
- Weber JS, D’Angelo SP, Minor D, Hodi FS, Gutzmer R, Neyns B, Hoeller C, Khushalani NI, Miller WH, Lao CD, et al. Nivolumab versus chemotherapy in patients with advanced melanoma who progressed after anti-CTLA-4 treatment (CheckMate 037): a randomised, controlled, open-label, phase 3 trial. Lancet Oncol. 2015;16:375–384. doi:10.1016/S1470-2045(15)70076-8.
- Powles T, Eder JP, Fine GD, Braiteh FS, Loriot Y, Cruz C, Bellmunt J, Burris HA, Petrylak DP, Teng S-L, et al. MPDL3280A (anti-PD-L1) treatment leads to clinical activity in metastatic bladder cancer. Nature. 2014;515:558–562. doi:10.1038/nature13904.
- Le DT, Uram JN, Wang H, Bartlett BR, Kemberling H, Eyring AD, Skora AD, Luber BS, Azad NS, Laheru D, et al. PD-1 blockade in tumors with mismatch-repair deficiency. N Engl J Med. 2015;372:2509–2520. doi:10.1056/NEJMoa1500596.
- Ansell SM, Lesokhin AM, Borrello I, Halwani A, Scott EC, Gutierrez M, Schuster SJ, Millenson MM, Cattry D, Freeman GJ, et al. PD-1 blockade with nivolumab in relapsed or refractory Hodgkin’s lymphoma. N Engl J Med. 2015;372:311–319. doi:10.1056/NEJMoa1411087.
- Armand P, Nagler A, Weller EA, Devine SM, Avigan DE, Chen Y-B, Kaminski MS, Holland HK, Winter JN, Mason JR, et al. Disabling immune tolerance by programmed death-1 blockade with pidilizumab after autologous hematopoietic stem-cell transplantation for diffuse large B-cell lymphoma: results of an international phase II trial. J Clin Oncol. 2013;31:4199–4206. doi:10.1200/JCO.2012.48.3685.
- Pardoll DM. The blockade of immune checkpoints in cancer immunotherapy. Nat Rev Cancer. 2012;12:252–264. doi:10.1038/nrc3239.
- Zou W, Chen L. Inhibitory B7-family molecules in the tumour microenvironment. Nat Rev Immunol. 2008;8:467–477. doi:10.1038/nri2326.
- Tumeh PC, Harview CL, Yearley JH, Shintaku IP, Taylor EJM, Robert L, Chmielowski B, Spasic M, Henry G, Ciobanu V, et al. PD-1 blockade induces responses by inhibiting adaptive immune resistance. Nature. 2014;515:568–571. doi:10.1038/nature13954.
- Klebanoff CA, Gattinoni L, Palmer DC, Muranski P, Ji Y, Hinrichs CS, Borman ZA, Kerkar SP, Scott CD, Finkelstein SE, et al. Determinants of successful CD8+ T-cell adoptive immunotherapy for large established tumors in mice. Clin Cancer Res. 2011;17:5343–5352. doi:10.1158/1078-0432.CCR-11-0503.
- Overwijk WW, Schluns KS. Functions of gammaC cytokines in immune homeostasis: current and potential clinical applications. Clin Immunol. 2009;132:153–165. doi:10.1016/j.clim.2009.03.512.
- Jenkins MH, Steinberg SM, Alexander MP, Fisher JL, Ernstoff MS, Turk MJ, Mullins DW, Brinckerhoff CE. Multiple murine BRaf(V600E) melanoma cell lines with sensitivity to PLX4032. Pigment Cell Melanoma Res. 2014;27:495–501. doi:10.1111/pcmr.12220.
- Dankort D, Curley DP, Cartlidge RA, Nelson B, Karnezis AN, Damsky WE, Jr., You MJ, DePinho RA, McMahon M, Bosenberg M. Braf(V600E) cooperates with Pten loss to induce metastatic melanoma. Nat Genet. 2009;41:544–552. doi:10.1038/ng.356.
- Rubinstein JC, Sznol M, Pavlick AC, Ariyan S, Cheng E, Bacchiocchi A, Kluger HM, Narayan D, Halaban R. Incidence of the V600K mutation among melanoma patients with BRAF mutations, and potential therapeutic response to the specific BRAF inhibitor PLX4032. J Transl Med. 2010;8:67. doi:10.1186/1479-5876-8-72.
- Long GV, Menzies AM, Nagrial AM, Haydu LE, Hamilton AL, Mann GJ, Hughes TM, Thompson JF, Scolyer RA, Kefford RF. Prognostic and clinicopathologic associations of oncogenic BRAF in metastatic melanoma. J Clin Oncol. 2011;29:1239–1246. doi:10.1200/JCO.2010.32.4327.
- Lee JH, Choi JW, Kim YS. Frequencies of BRAF and NRAS mutations are different in histological types and sites of origin of cutaneous melanoma: a meta-analysis. Br J Dermatol. 2011;164:776–784. doi:10.1111/j.1365-2133.2010.10185.x.
- Baumann BC, Dorsey JF, Benci JL, Joh DY, Kao GD. Stereotactic intracranial implantation and in vivo bioluminescent imaging of tumor xenografts in a mouse model system of glioblastoma multiforme. J Vis Exp. 2012;67:4089.
- Clark AJ, Fakurnejad S, Ma Q, Hashizume R. Bioluminescence imaging of an immunocompetent animal model for glioblastoma. J Vis Exp. 2016;107:e53287.
- Man J, Shoemake JD, Ma T, Rizzo AE, Godley AR, Wu Q, Mohammadi AM, Bao S, Rich JN, Yu JS. Hyperthermia sensitizes glioma stem-like cells to radiation by inhibiting AKT signaling. Cancer Res. 2015;75:1760–1769. doi:10.1158/0008-5472.CAN-14-3621.
- Man J, Shoemake J, Zhou W, Fang X, Wu Q, Rizzo A, Prayson R, Bao S, Rich JN, Yu JS. Sema3C promotes the survival and tumorigenicity of glioma stem cells through Rac1 activation. Cell Rep. 2014;9:1812–1826. doi:10.1016/j.celrep.2014.10.055.
- Ribeiro Gomes J, Schmerling RA, Haddad CK, Racy DJ, Ferrigno R, Gil E, Zanuncio P, Buzaid AC. Analysis of the abscopal effect with anti-PD1 therapy in patients with metastatic solid tumors. J Immunother. 2016;39:367–372. doi:10.1097/CJI.0000000000000141.
- Park SS, Dong H, Liu X, Harrington SM, Krco CJ, Grams MP, Mansfield AS, Furutani KM, Olivier KR, Kwon ED. PD-1 restrains radiotherapy-induced abscopal effect. Cancer Immunol Res. 2015;3:610–619. doi:10.1158/2326-6066.CIR-14-0138.
- Dewan MZ, Galloway AE, Kawashima N, Dewyngaert JK, Babb JS, Formenti SC, Demaria S. Fractionated but not single-dose radiotherapy induces an immune-mediated abscopal effect when combined with anti-CTLA-4 antibody. Clin Cancer Res. 2009;15:5379–5388. doi:10.1158/1078-0432.CCR-09-0265.
- Han S, Zhang C, Li Q, Dong J, Liu Y, Huang Y, Jiang T, Wu A. Tumour-infiltrating CD4(+) and CD8(+) lymphocytes as predictors of clinical outcome in glioma. Br J Cancer. 2014;110:2560–2568. doi:10.1038/bjc.2014.162.
- Yu JS, Lee PK, Ehtesham M, Samoto K, Black KL, Wheeler CJ. Intratumoral T cell subset ratios and Fas ligand expression on brain tumor endothelium. J Neurooncol. 2003;64:55–61.
- Young KH, Baird JR, Savage T, Cottam B, Friedman D, Bambina S, Messenheimer DJ, Fox B, Newell P, Bahjat KS, et al. Optimizing timing of immunotherapy improves control of tumors by hypofractionated radiation therapy. PLoS One. 2016;11:e0157164. doi:10.1371/journal.pone.0157164.
- Postow MA, Callahan MK, Barker CA, Yamada Y, Yuan J, Kitano S, Mu Z, Rasalan T, Adamow M, Ritter E, et al. Immunologic correlates of the abscopal effect in a patient with melanoma. N Engl J Med. 2012;366:925–931. doi:10.1056/NEJMoa1112824.
- Barker CA, Postow MA, Khan SA, Beal K, Parhar PK, Yamada Y, Lee NY, Wolchok JD. Concurrent radiotherapy and ipilimumab immunotherapy for patients with melanoma. Cancer Immunol Res. 2013;1:92–98. doi:10.1158/2326-6066.CIR-13-0082.
- Tanaka A, Sakaguchi S. Regulatory T cells in cancer immunotherapy. Cell Res. 2017;27:109–118. doi:10.1038/cr.2016.151.
- Kodumudi KN, Weber A, Sarnaik AA, Pilon-Thomas S. Blockade of myeloid-derived suppressor cells after induction of lymphopenia improves adoptive T cell therapy in a murine model of melanoma. J Immunol. 2012;189:5147–5154. doi:10.4049/jimmunol.1200274.
- Deng L, Liang H, Burnette B, Beckett M, Darga T, Weichselbaum RR, Fu Y-X. Irradiation and anti-PD-L1 treatment synergistically promote antitumor immunity in mice. J Clin Invest. 2014;124:687–695. doi:10.1172/JCI67313.
- Shiraishi K, Ishiwata Y, Nakagawa K, Yokochi S, Taruki C, Akuta T, Ohtomo K, Matsushima K, Tamatani T, Kanegasaki S. Enhancement of antitumor radiation efficacy and consistent induction of the abscopal effect in mice by ECI301, an active variant of macrophage inflammatory protein-1alpha. Clin Cancer Res. 2008;14:1159–1166. doi:10.1158/1078-0432.CCR-07-4485.
- Strigari L, Mancuso M, Ubertini V, Soriani A, Giardullo P, Benassi M, D’Alessio D, Leonardi S, Soddu S, Bossi G. Abscopal effect of radiation therapy: interplay between radiation dose and p53 status. Int J Radiat Biol. 2014;90:248–255. doi:10.3109/09553002.2014.874608.
- Reynders K, Illidge T, Siva S, Chang JY, de Ruysscher D. The abscopal effect of local radiotherapy: using immunotherapy to make a rare event clinically relevant. Cancer Treat Rev. 2015;41:503–510. doi:10.1016/j.ctrv.2015.03.011.
- Park B, Yee C, Lee K-M. The effect of radiation on the immune response to cancers. Int J Mol Sci. 2014;15:927–943. doi:10.3390/ijms15010927.
- Apetoh L, Ghiringhelli F, Tesniere A, Obeid M, Ortiz C, Criollo A, Mignot G, Maiuri MC, Ullrich E, Saulnier P, et al. Toll-like receptor 4-dependent contribution of the immune system to anticancer chemotherapy and radiotherapy. Nat Med. 2007;13:1050–1059. doi:10.1038/nm1622.
- Suzuki Y, Mimura K, Yoshimoto Y, Watanabe M, Ohkubo Y, Izawa S, Murata K, Fujii H, Nakano T, Kono K. Immunogenic tumor cell death induced by chemoradiotherapy in patients with esophageal squamous cell carcinoma. Cancer Res. 2012;72:3967–3976. doi:10.1158/0008-5472.CAN-12-0851.
- Korn T, Kallies A. T cell responses in the central nervous system. Nat Rev Immunol. 2017;17:179–194. doi:10.1038/nri.2016.144.
- Rothhammer V, Muschaweckh A, Gasteiger G, Petermann F, Heink S, Busch DH, Heikenwälder M, Hemmer B, Drexler I, Korn T. alpha4-integrins control viral meningoencephalitis through differential recruitment of T helper cell subsets. Acta Neuropathol Commun. 2014;2:27. doi:10.1186/2051-5960-2-27.
- Ifergan I, Kébir H, Bernard M, Wosik K, Dodelet-Devillers A, Cayrol R, Arbour N, Prat A. The blood-brain barrier induces differentiation of migrating monocytes into Th17-polarizing dendritic cells. Brain. 2008;131:785–799. doi:10.1093/brain/awm295.
- Ji Q, Castelli L, Goverman JM. MHC class I-restricted myelin epitopes are cross-presented by Tip-DCs that promote determinant spreading to CD8(+) T cells. Nat Immunol. 2013;14:254–261. doi:10.1038/ni.2513.
- Galon J, Mlecnik B, Bindea G, Angell HK, Berger A, Lagorce C, Lugli A, Zlobec I, Hartmann A, Bifulco C, et al. Towards the introduction of the ‘Immunoscore’ in the classification of malignant tumours. J Pathol. 2014;232:199–209. doi:10.1002/path.4287.
- Berghoff AS, Fuchs E, Ricken G, Mlecnik B, Bindea G, Spanberger T, Hackl M, Widhalm G, Dieckmann K, Prayer D, et al. Density of tumor-infiltrating lymphocytes correlates with extent of brain edema and overall survival time in patients with brain metastases. Oncoimmunology. 2016;5:e1057388. doi:10.1080/2162402X.2015.1057388.
- Cao Y, Tsien CI, Shen Z, Tatro DS, Ten Haken R, Kessler ML, Chenevert TL, Lawrence TS. Use of magnetic resonance imaging to assess blood-brain/blood-glioma barrier opening during conformal radiotherapy. J Clin Oncol. 2005;23:4127–4136. doi:10.1200/JCO.2005.07.144.
- Jiang X, Engelbach JA, Yuan L, Cates J, Gao F, Drzymala RE, Hallahan DE, Rich KM, Schmidt RE, Ackerman JJH, et al. Anti-VEGF antibodies mitigate the development of radiation necrosis in mouse brain. Clin Cancer Res. 2014;20:2695–2702. doi:10.1158/1078-0432.CCR-13-1941.
- Choong ES, Lo S, Drummond M, Fogarty GB, Menzies AM, Guminski A, Shivalingam B, Clarke K, Long GV, Hong AM. Survival of patients with melanoma brain metastasis treated with stereotactic radiosurgery and active systemic drug therapies. Eur J Cancer. 2017;75:169–178. doi:10.1016/j.ejca.2017.01.007.
- Liew DN, Kano H, Kondziolka D, Mathieu D, Niranjan A, Flickinger JC, Kirkwood JM, Tarhini A, Moschos S, Lunsford LD. Outcome predictors of Gamma Knife surgery for melanoma brain metastases. Clinical article. J Neurosurg. 2011;114:769–779. doi:10.3171/2010.5.JNS1014.
- Knisely JP, Yu JB, Flanigan J, Sznol M, Kluger HM, Chiang VL. Radiosurgery for melanoma brain metastases in the ipilimumab era and the possibility of longer survival. J Neurosurg. 2012;117:227–233. doi:10.3171/2012.5.JNS111929.
- Kiess AP, Wolchok JD, Barker CA, Postow MA, Tabar V, Huse JT, Chan TA, Yamada Y, Beal K. Stereotactic radiosurgery for melanoma brain metastases in patients receiving ipilimumab: safety profile and efficacy of combined treatment. Int J Radiat Oncol Biol Phys. 2015;92:368–375. doi:10.1016/j.ijrobp.2015.01.004.
- Cohen JV, Kluger HM. Systemic immunotherapy for the treatment of brain metastases. Front Oncol. 2016;6:49. doi:10.3389/fonc.2016.00049.
- Eyler CE, Wu Q, Yan K, MacSwords JM, Chandler-Militello D, Misuraca KL, Lathia JD, Forrester MT, Lee J, Stamler JS, et al. Glioma stem cell proliferation and tumor growth are promoted by nitric oxide synthase-2. Cell. 2011;146:53–66. doi:10.1016/j.cell.2011.06.006.
- Darr D, Clark KS, Phillips JH, Pinheiro E, Sriram V, Xiong J, Jordan J, Sharpless NE, Perou C, Moschos S. Abstract 5024: mDX400, the murine analog against the anti-PD1 antibody MK-347 is active in immunocompetent, autochthonous murine models of melanoma and breast cancer. Cancer Res. 2014;74:5024. doi:10.1158/0008-5472.CAN-13-3514.
- Demelash A, Pfannenstiel LW, Tannenbaum CS, Li X, Kalady MF, DeVecchio J, Gastman BR. Structure-function analysis of the Mcl-1 protein identifies a novel senescence-regulating domain. J Biol Chem. 2015;290:21962–21975. doi:10.1074/jbc.M115.663898.