ABSTRACT
Systemic anticancer immunity can be reinstated via the induction of immunogenic cell death (ICD) in malignant cells. Thus, certain classes of cytotoxic compounds, for example, anthracyclines, oxaliplatin and taxanes are endowed with the capacity to act on cancer cells to ignite premortem stress pathways that lead to the surface exposure of calreticulin (CALR) and the cellular release of adenosine triphosphate, annexin A1, high mobility group B1 and type-1 interferons. Altogether, these alterations constitute the hallmarks of ICD. Here we report the design of a discovery pipeline for the identification of novel ICD inducers by means of a phenotypic screening platform. The use of fluorescent biosensors as proxies for the manifestation of ICD hallmarks has enabled the exploration of large collections of chemical compounds by automatized screening routines. Imaging-based assessment and phenotypic selection led to the identification of potential ICD inducers that could be validated further in vitro and in vivo, confirming that bona fide ICD inducers possess the capacity to induce immunological long-term memory and to confer resistance against rechallenge with syngeneic tumors. Machine learning algorithms analyzing the physicochemical properties of ICD inducers can assist in the preselection of compounds with potential ICD-stimulatory properties, further accelerating the screening efforts designed to develop new immunotherapeutic agents.
Introduction
Immunogenic cell death (ICD) can be triggered under a variety of cellular stress conditions such as the exposure to oncolytic compounds, ionizing irradiation and specific chemotherapeutics.Citation1−Citation9 The pattern of premortem stress responses evoked by these interventions mimics the cellular defense against viral infection and sets off an immune response against tumor-associated antigens.Citation10,Citation11 In this context, chemotherapeutic agents like anthracyclines, cyclophosphamide, oxaliplatin and taxanes mediate at least part of their antineoplastic effects through anticancer immune responses.Citation12 These cytotoxicants cause premortem stress responses, stimulating the cancer cells to emit signals that render them detectable for the immune system.Citation13-Citation16 Such immunogenic cell death hallmarks include an autophagic response that facilitates the cellular release of adenosine triphosphate (ATP),Citation17,Citation18an endoplasmic reticulum (ER) stress response causing the exposure of calreticulin (CALR) on the cell surface,Citation13 the cellular demise-related exodus of the nuclear protein high mobility group box 1 (HMGB1),Citation19,Citation20 as well as a type-1 interferon response.Citation21 ATP acts as a chemoattractant for dendritic cell (DC) precursors,Citation22 while CALR functions as an ‘eat me’ signal to facilitate the uptake of tumor-associated antigen by DC, Citation23 and liberated HMGB1 activates Toll-like receptor-4 (TLR4) to stimulate DC maturation.Citation24,Citation25 Cell death is also accompanied by the release of cytoplasmic annexin A1 (ANXA1), which acts as an essential chemotactic factor for DC homing and immunological synapse formation through its action on formyl peptide receptor 1 (FPR1).Citation26
Clinical evidence supports the importance of ICD for long-term therapeutic effects and the necessity for each of the aforementioned ligands and receptors for its onset. Thus, patients bearing tumors that lack features of ICD-related premortem stress (such as autophagy or ER stress) or immunogenic factors (such as ANXA1, CALR and HMGB1) have a poor prognosis.Citation27,Citation28 Moreover, patients harboring deficient receptors for HMGB1 (TLR4) or ANXA1 (FPR1) exhibit a reduced progression-free or overall survival post-chemotherapy.Citation13,Citation26 Measures that improve ICD inductionCitation13,Citation29,Citation30 increase the efficacy of ICD inducers in preclinical models, as well as in cancer patients.Citation31 Moreover, in mouse models, pretreatment with ICD inducers sensitizes cancers to subsequent treatment with immune checkpoint blockers.Citation32 Altogether, these preclinical and clinical results support the active search for optimal ICD inducers.
A screening platform for the discovery of ICD inducers
Given the immunological and oncological significance of ICD, we decided to identify novel pharmacological agents that are capable of inducing ICD and hence triggering anticancer immunity in preclinical models. To this aim, we generated an ICD fingerprinting platform that allows for the simultaneous assessment of ICD-associated features by means of automated epifluorescence microscopy-based assays applied to human cancer cells stably transduced with fluorescent biosensors constructs ( and ).Citation34
Figure 1. Discovery pipeline. The discovery pipeline for novel immunogenic cell death inducers consists of several building blocks. Machine learning algorithms relate the physicochemical properties of molecules from chemical compound repositories with the likelihood of a compound to induce ICD thus allowing to pre-select a fraction of drugs with the highest probability to induce ICD. In the next step pre-selected drugs are assessed for their potency to induce ICD-associated features in fluorescent biosensors cells by means of high content screening. Confirmed hits are further validated by additional methodology in human and murine cells different from the initial screening approach. Lead compounds are identified in vivo, by assessing compound-induced immunological long-term memory and to confer resistance against rechallenge with syngeneic tumors.Citation33 Finally, lead compounds are tested as monoimmunotherapy and/or are combined with the subsequent application of immune checkpoint blockade in order to boost T cell responses. The lower panel depicts a schematic representation of the main biosensors employed for the detection of immunogenic cell death hallmarks (such as calreticulin (CALR) exposure, high mobility group box 1 (HMGB1) exodus and adenosine triphosphate (ATP) secretion) in HCS.
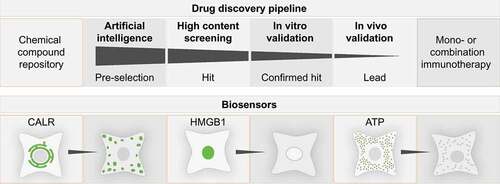
Figure 2. Graphical description of the cell biology screening platform in its present configuration. The cell biology screening platform entails an array of automates that allow for semi-automated cell culture, compound management, barcoded sample tracking and multiplex readout. Automated workflows for image-based high content chemical compound screening coupled to sophisticated image analysis and data mining routines allow for the identification of immunogenic cell death surrogate markers by means of fluorescent biosensors.
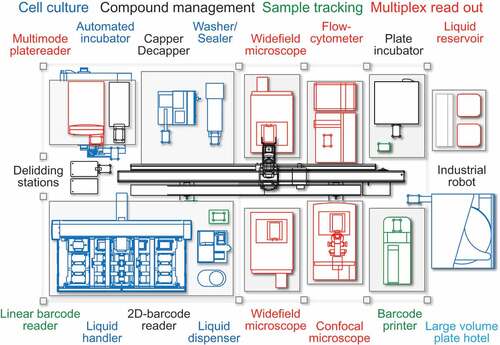
As a first approach, we quantified ICD hallmarks such as ATP liberation, CALR exposure and HMGB1 exodus, which occur before or during deathCitation13,Citation35,Citation36 in cancer cells exposed to compound libraries to identify possible hits. Transgene-encoded fluorescent biosensors allowing for the subcellular localization of HMGB1 and CALR were stably expressed in human osteosarcoma U2OS cells that were counterstained with quinacrine (which allows for the visualization of intracellular ATP) and 4ʹ,6-diamidino-2-phenylindole (DAPI, which labels chromatin). These cells were exposed to chemical libraries () comprising currently used anticancer drugs (Oncology drugs set, National Cancer Institute, Development Therapeutic Program; NCI DTP, Bethesda, MD, US) and agents that have reached clinical trial stage or have obtained approval by the FDA (US drug library, MicroSource discovery systems, Gaylordsville, CT, US), one by one, over different concentration ranges and time points, followed by several rounds of high-content data analysis.
Table 1. Screening campaigns for the discovery of novel ICD inducers
This approach led to the identification of a list of ICD-inducing compounds that fell into the group of cardiac glycosides (GC).Citation31 As a result, a range of cardiac glycosides were further validated for their in vitro effects on biomarkers of ICD, followed by the confirmation that they were able to stimulate anticancer immune responses and to generate immunological memory in vivo (in mice) when combined with moderately successful chemotherapeutics such as cisplatin or mitomycin C (both of which on their own are not able to induce ICD). Retrospective clinical analysis confirmed an increased overall survival for patients that received GC during anticancer treatments.
Following a very similar approach, yet focusing on a subset of experimental agents from the National Cancer Institute (NCI) Mechanistic Diversity Set (i.e. agents that were selected due to their capacity to limit cellular proliferation within the NCI-60 panel of cell lines), we identified septacidin as a novel ICD inducer. Indeed, septacidin was able to cause anticancer immune responses and control tumor growth in vivo in a T cell-dependent fashion.Citation37
Recently, we screened the Public Chemogenomic Set for Protein Kinases covering more than 500 compoundsCitation40 and an in-house collection of major FDA-approved tyrosine kinase inhibitors for ICD induction. In this screening campaign, we were able to show that crizotinib acts off-target to induce all ICD hallmarks in human and mouse cancer cell lines if this tyrosine kinase inhibitor was used at a high-dose (10 µM) that trespasses the specific inhibition of its usual target kinases (ALK and ROS1) and hence induces ‘off-target’ effects on other tyrosine kinases, converging crizotinib de facto into a multikinase inhibitor. High-dose crizotinib induced the redistribution of microtubule-associated protein 1A/1B light chain 3B (hereafter referred to as LC3) fused to green fluorescent protein (GFP) to cytoplasmic dots (LC3-GFP puncta) as a sign of autophagyCitation39 and stimulated the phosphorylation of eukaryotic translation initiation factor 2α (eIF2α) by the ER stress kinase eIF2α kinase 3 (EIF2AK3).Citation39 As a correlate of these premortem stress responses, crizotinib stimulated the release of ATP from cancer cells (downstream of autophagy), as well as the surface exposure of CALR (downstream of ER stress). Moreover, crizotinib was highly effective in stimulating the release of HMGB1 and annexin A1 (as a result of cell death) and the activation of a transcriptional program involving the upregulation of mRNAs coding for type-1 interferons and programmed death – ligand 1 (PD-L1).Citation39 These in vitro effects were particularly strong when crizotinib was combined with non-ICD inducing chemotherapeutics (in particular cisplatin and mitomycin C). Indeed, crizotinib could be advantageously combined with cisplatin in vivo, in preclinical mouse models of non-small cell lung cancer (NSLCL) to induce therapeutic anticancer immune responses. Importantly, such a combination therapy (crizotinib + cisplatin) efficiently sensitized orthotopic NSCLCs to subsequent immunotherapy targeting programmed death-1 (PD-1), the receptor of PD-L1. This sensitization effect could be mechanistically explained by the capacity of crizotinib/cisplatin co-treatment to increase tumor infiltration by cytotoxic T lymphocytes.Citation39
In summary, we have been using the ICD fingerprint platform for the discovery of several novel immunogenic cell death inducers (), all of which were pre-clinically validated.
Machine learning for identifying the molecular properties of ICD inducers
We used machine learning algorithms to determine the most important biomarkers that predict the induction of ICD. This approach revealed that the pathognomonic biomarker of ICD is the phosphorylation of eIF2α and its downstream correlates including the surface exposure of CALR, the formation of stress granules and the induction of autophagy (which to some extent depends on eIF2α phosphorylation).Citation38 At the same time, it appears that prototypic ICD inducers do not stimulate the activation of ER stress pathways other than eIF2α phosphorylation, meaning that they do not cause the activation of the transcription factors ATF4, ATF6 and XBP1.Citation38,Citation41,Citation42 Thus, bona fide ICD inducers elicit a restricted pattern of cellular stress responses, conferring them with a sort of ‘specificity’. Importantly, this information on the selected stress pathway elicited by validated ICD inducers can be used to design algorithms that relate the physicochemical properties of these molecules with their biological activity. Using this approach, we can now use these algorithms to predict the likelihood of a compound to induce ICD, simply by feeding information on its physicochemical and structural properties into the computer. This strategy can be used to pre-filter large compound libraries to concentrate subsequent screening efforts on a fraction of the drug collection with the highest probability to induce ICD. This approach harbors the promise of reducing costs and accelerating the speed of drug discovery in the immuno-oncology space.
Concluding remarks
In summary, we assembled a multistep discovery pipeline for the biosensor-based identification of new ICD inducers. This discovery pipeline () includes an artificial intelligence-driven pre-selection process that narrows down the compounds of interest, selecting the those with the highest likelihood based on their physicochemical and structural properties. Subsequently, an automated medium-throughput screening campaign can be performed for high-content analyses of the drug effects on cancer cells expressing fluorescent biosensors that measure different ICD-related effects (). This leads to the first round of phenotypic hit identification, still with the general caveat of false positive (and also false negative results), thus requiring a second round of in vitro validation, usually involving several distinct cancer cell lines. Confirmed leads then undergo a final in vivo validation that usually involves two steps. First, cancer cells are killed in vitro with the ICD inducing drug candidate and then injected subcutaneously into immunocompetent mice to evaluate their capacity to induce a protective anticancer immune response precluding the growth of live cancer cells that are inoculated into the mice one week later. Second, the efficacy of ICD inducers is comparatively evaluated in immunocompetent and immunodeficient mice bearing established tumors with the expectation that tumor growth reduction is improved in the presence of an intact immune system (). Using this discovery pipeline, we are identifying new ICD inducers that should enter clinical trials, initially as single agents and then in combination with immune checkpoint blockade.
Abbreviations
DC dendritic cell
ER endoplasmic reticulum
GC cardiac glycoside
ICD immunogenic cell death
NCI DTP National Cancer Institute, Development Therapeutic Program
Disclosure of Potential Conflicts of Interest
The authors declare no conflict of interest.
Acknowledgments
LB is supported by BMS. GK is supported by the Ligue contre le Cancer (équipes labelisées); Agence National de la Recherche (ANR) – Projets blancs; ANR under the frame of E-Rare-2, the ERA-Net for Research on Rare Diseases; Association pour la recherche sur le cancer (ARC); Cancéropôle Ile-de-France; Institut National du Cancer (INCa); Fondation Bettencourt-Schueller; Fondation de France; Fondation pour la Recherche Médicale (FRM); the European Commission (ArtForce); the European Research Council (ERC); the LabEx Immuno-Oncology; the SIRIC Stratified Oncology Cell DNA Repair and Tumor Immune Elimination (SOCRATE); the SIRIC Cancer Research and Personalized Medicine (CARPEM); the Seerave foundation; the Swiss Bridge Foundation, ISREC and the Paris Alliance of Cancer Research Institutes (PACRI).
References
- Yatim N, Cullen S, Albert ML. Dying cells actively regulate adaptive immune responses. Nat Rev Immunol. 2017;17(4):262–275. doi:10.1038/nri.2017.9.
- Wennerberg E, Vanpouille-Box C, Bornstein S, Yamazaki T, Demaria S, Galluzzi L. Immune recognition of irradiated cancer cells. Immunol Rev. 2017;280(1):220–230. doi:10.1111/imr.12568.
- Casares N, Pequignot MO, Tesniere A, Ghiringhelli F, Roux S, Chaput N, Schmitt E, Hamai A, Hervas-Stubbs S, Obeid M, et al. Caspase-dependent immunogenicity of doxorubicin-induced tumor cell death. J Exp Med. 2005;202(12):1691–1701. doi:10.1084/jem.20050915.
- Kepp O, Senovilla L, Vitale I, Vacchelli E, Adjemian S, Agostinis P, Apetoh L, Aranda F, Barnaba V, Bloy N, et al. Consensus guidelines for the detection of immunogenic cell death. Oncoimmunology. 2014;3(9):e955691. doi:10.4161/21624011.2014.955691.
- Garg AD, More S, Rufo N, Mece O, Sassano ML, Agostinis P, Zitvogel L, Kroemer G, Galluzzi L. Trial watch: immunogenic cell death induction by anticancer chemotherapeutics. Oncoimmunology. 2017;6(12):e1386829. doi:10.1080/2162402X.2017.1386829.
- Galluzzi L, Vitale I, Aaronson SA, Abrams JM, Adam D, Agostinis P, Alnemri ES, Altucci L, Amelio I, Andrews DW, et al. Molecular mechanisms of cell death: recommendations of the nomenclature committee on cell death 2018. Cell Death Differ. 2018;25(3):486–541. doi:10.1038/s41418-017-0012-4.
- Garg AD, Agostinis P. Cell death and immunity in cancer: from danger signals to mimicry of pathogen defense responses. Immunol Rev. 2017;280(1):126–148. doi:10.1111/imr.12574.
- Garg AD, Dudek-Peric AM, Romano E, Agostinis P. Immunogenic cell death. Int J Dev Biol. 2015;59(1–3):131–140. doi:10.1387/ijdb.150061pa.
- Garg AD, Krysko DV, Vandenabeele P, Agostinis P. Hypericin-based photodynamic therapy induces surface exposure of damage-associated molecular patterns like HSP70 and calreticulin. Cancer Immunol Immunother. 2012;61(2):215–221. doi:10.1007/s00262-011-1184-2.
- Pol J, Vacchelli E, Aranda F, Castoldi F, Eggermont A, Cremer I, Sautès-Fridman C, Fucikova J, Galon J, Spisek R, et al. Trial watch: immunogenic cell death inducers for anticancer chemotherapy. Oncoimmunology. 2015;4(4):e1008866. doi:10.1080/2162402X.2015.1008371.
- Adkins I, Fucikova J, Garg AD, Agostinis P, Spisek R. Physical modalities inducing immunogenic tumor cell death for cancer immunotherapy. Oncoimmunology. 2014;3(12):e968434. doi:10.4161/21624011.2014.968434.
- Galluzzi L, Buque A, Kepp O, Zitvogel L, Kroemer G. Immunological effects of conventional chemotherapy and targeted anticancer agents. Cancer Cell. 2015;28(6):690–714. doi:10.1016/j.ccell.2015.10.012.
- Galluzzi L, Buque A, Kepp O, Zitvogel L, Kroemer G. Immunogenic cell death in cancer and infectious disease. Nat Rev Immunol. 2017;17(2):97–111. doi:10.1038/nri.2016.107.
- Kepp O, Galluzzi L, Martins I, Schlemmer F, Adjemian S, Michaud M, Sukkurwala AQ, Menger L, Zitvogel L, Kroemer G. Molecular determinants of immunogenic cell death elicited by anticancer chemotherapy. Cancer Metastasis Rev. 2011;30(1):61–69. doi:10.1007/s10555-011-9273-4.
- Tesniere A, Schlemmer F, Boige V, Kepp O, Martins I, Ghiringhelli F, Aymeric L, Michaud M, Apetoh L, Barault L, et al. Immunogenic death of colon cancer cells treated with oxaliplatin. Oncogene. 2010;29(4):482–491. doi:10.1038/onc.2009.356.
- Senovilla L, Vitale I, Martins I, Tailler M, Pailleret C, Michaud M, Galluzzi L, Adjemian S, Kepp O, Niso-Santano M, et al. An immunosurveillance mechanism controls cancer cell ploidy. Science. 2012;337(6102):1678–1684. doi:10.1126/science.1224922.
- Michaud M, Martins I, Sukkurwala AQ, Adjemian S, Ma Y, Pellegatti P, Shen S, Kepp O, Scoazec M, Mignot G, et al. Autophagy-dependent anticancer immune responses induced by chemotherapeutic agents in mice. Science. 2011;334(6062):1573–1577. doi:10.1126/science.1208347.
- Garg AD, Krysko DV, Vandenabeele P, Agostinis P. Extracellular ATP and P(2)X(7) receptor exert context-specific immunogenic effects after immunogenic cancer cell death. Cell Death Dis. 2016;7:e2097. doi:10.1038/cddis.2015.411.
- Liu P, Zhao L, Loos F, Iribarren K, Lachkar S, Zhou H, Gomes-da-Silva LC, Chen G, Bezu L, Boncompain G, et al. Identification of pharmacological agents that induce HMGB1 release. Sci Rep. 2017;7(1):14915. doi:10.1038/s41598-017-14848-1.
- Liu P, Zhao L, Loos F, Iribarren K, Kepp O, Kroemer G. Epigenetic anticancer agents cause HMGB1 release in vivo. Oncoimmunology. 2018;7(6):e1431090. doi:10.1080/2162402X.2018.1490854.
- Sistigu A, Yamazaki T, Vacchelli E, Chaba K, Enot DP, Adam J, Vitale I, Goubar A, Baracco EE, Remédios C, et al. Cancer cell-autonomous contribution of type I interferon signaling to the efficacy of chemotherapy. Nat Med. 2014;20(11):1301–1309. doi:10.1038/nm.3708.
- Ma Y, Adjemian S, Mattarollo SR, Yamazaki T, Aymeric L, Yang H, Portela Catani JP, Hannani D, Duret H, Steegh K, et al. Anticancer chemotherapy-induced intratumoral recruitment and differentiation of antigen-presenting cells. Immunity. 2013;38(4):729–741. doi:10.1016/j.immuni.2013.03.003.
- Stoll G, Iribarren K, Michels J, Leary A, Zitvogel L, Cremer I, Kroemer G. Calreticulin expression: interaction with the immune infiltrate and impact on survival in patients with ovarian and non-small cell lung cancer. Oncoimmunology. 2016;5(7):e1177692. doi:10.1080/2162402X.2016.1177692.
- Apetoh L, Ghiringhelli F, Tesniere A, Criollo A, Ortiz C, Lidereau R, Mariette C, Chaput N, Mira J-P, Delaloge S, et al. The interaction between HMGB1 and TLR4 dictates the outcome of anticancer chemotherapy and radiotherapy. Immunol Rev. 2007;220:47–59. doi:10.1111/j.1600-065X.2007.00573.x.
- Vanpouille-Box C, Galluzzi L. CD103(+) cells at the forefront of anticancer immunity. Oncoimmunology. 2017;6(11):e1356154. doi:10.1080/2162402X.2017.1356154.
- Vacchelli E, Ma Y, Baracco EE, Sistigu A, Enot DP, Pietrocola F, Yang H, Adjemian S, Chaba K, Semeraro M, et al. Chemotherapy-induced antitumor immunity requires formyl peptide receptor 1. Science. 2015;350(6263):972–978. doi:10.1126/science.aad0779.
- Ladoire S, Enot D, Andre F, Zitvogel L, Kroemer G. Immunogenic cell death-related biomarkers: impact on the survival of breast cancer patients after adjuvant chemotherapy. Oncoimmunology. 2016;5(2):e1082706. doi:10.1080/2162402X.2015.1082706.
- Fucikova J, Truxova I, Hensler M, Becht E, Kasikova L, Moserova I, Vosahlikova S, Klouckova J, Church SE, Cremer I, et al. Calreticulin exposure by malignant blasts correlates with robust anticancer immunity and improved clinical outcome in AML patients. Blood. 2016;128(26):3113–3124. doi:10.1182/blood-2016-08-731737.
- Wang YJ, Fletcher R, Yu J, Zhang L. Immunogenic effects of chemotherapy-induced tumor cell death. Genes Dis. 2018;5(3):194–203. doi:10.1016/j.gendis.2018.05.003.
- Yamazaki T, Galluzzi L. TREX1 cuts down on cancer immunogenicity. Trends Cell Biol. 2017;27(8):543–545. doi:10.1016/j.tcb.2017.06.001.
- Menger L, Vacchelli E, Adjemian S, Martins I, Ma Y, Shen S, Yamazaki T, Sukkurwala AQ, Michaud M, Mignot G, et al. Cardiac glycosides exert anticancer effects by inducing immunogenic cell death. Sci Transl Med. 2012;4(143):143ra99. doi:10.1126/scitranslmed.3003807.
- Pfirschke C, Engblom C, Rickelt S, Cortez-Retamozo V, Garris C, Pucci F, Yamazaki T, Poirier-Colame V, Newton A, Redouane Y, et al. Immunogenic chemotherapy sensitizes tumors to checkpoint blockade therapy. Immunity. 2016;44(2):343–354. doi:10.1016/j.immuni.2015.11.024.
- Humeau J, Levesque S, Kroemer G, Pol JG. Gold standard assessment of immunogenic cell death in oncological mouse models. Methods Mol Biol. 2019;1884:297–315. doi:10.1007/978-1-4939-8885-3_21.
- Kepp O, Galluzzi L, Lipinski M, Yuan J, Kroemer G. Cell death assays for drug discovery. Nat Rev Drug Discov. 2011;10(3):221–237. doi:10.1038/nrd3373.
- Panaretakis T, Kepp O, Brockmeier U, Tesniere A, Bjorklund AC, Chapman DC, Durchschlag M, Joza N, Pierron G, van Endert P, et al. Mechanisms of pre-apoptotic calreticulin exposure in immunogenic cell death. Embo J. 2009;28(5):578–590. doi:10.1038/emboj.2009.1.
- Ghiringhelli F, Apetoh L, Tesniere A, Aymeric L, Ma Y, Ortiz C, Vermaelen K, Panaretakis T, Mignot G, Ullrich E, et al. Activation of the NLRP3 inflammasome in dendritic cells induces IL-1beta-dependent adaptive immunity against tumors. Nat Med. 2009;15(10):1170–1178. doi:10.1038/nm.2028.
- Sukkurwala AQ, Adjemian S, Senovilla L, Michaud M, Spaggiari S, Vacchelli E, Baracco EE, Galluzzi L, Zitvogel L, Kepp O, et al. Screening of novel immunogenic cell death inducers within the NCI mechanistic diversity set. Oncoimmunology. 2014;3:e28473. doi:10.4161/onci.28473.
- Bezu L, Sauvat A, Humeau J, Gomes-da-Silva LC, Iribarren K, Forveille S, Garcia P, Zhao L, Liu P, Zitvogel L, et al. eIF2alpha phosphorylation is pathognomonic for immunogenic cell death. Cell Death Differ. 2018;25(8):1375–1393. doi:10.1038/s41418-017-0044-9.
- Liu P, Zhao L, Pol J, Levesque S, Petrazzuolo A, Pfirschke C, Engblom C, Rickelt S, Yamazaki T, Iribarren K, et al. Crizotinib-induced immunogenic cell death in non-small cell lung cancer. Nat Commun. 2019;10(1):1486. doi:10.1038/s41467-019-09415-3.
- Drewry DH, Wells CI, Andrews DM, Angell R, Al-Ali H, Axtman AD, Capuzzi SJ, Elkins JM, Ettmayer P, Frederiksen M, et al. Progress towards a public chemogenomic set for protein kinases and a call for contributions. PLoS One. 2017;12(8):e0181585. doi:10.1371/journal.pone.0181585.
- Giglio P, Gagliardi M, Tumino N, Antunes F, Smaili S, Cotella D, Hoadley KA, Print C, Knowlton N, Black MA, et al. PKR and GCN2 stress kinases promote an ER stress-independent eIF2alpha phosphorylation responsible for calreticulin exposure in melanoma cells. Oncoimmunology. 2018;7(8):e1466765. doi:10.1080/2162402X.2018.1490854.
- van Vliet AR, Martin S, Garg AD, Agostinis P. The PERKs of damage-associated molecular patterns mediating cancer immunogenicity: from sensor to the plasma membrane and beyond. Semin Cancer Biol. 2015;33:74–85. doi:10.1016/j.semcancer.2015.03.010.