ABSTRACT
Objectives: The interaction between the quorum sensing (QS) molecules of gut microbiota and the immunity of colorectal cancer (CRC) has not been investigated before.
Methods: We measured the concentration of autoinducer-2 (AI-2) in samples of stool, colorectal tissue, saliva and serum of CRC patients, and compared this to AI-2 levels in colorectal adenoma (AD) and normal colon mucosa (NC). To explore the activated signaling pathways involved, we utilized AI-2 extracted from Fusobacterium nucleatum to stimulate macrophages and validated these in vitro findings in human CRC tissues.
Results: The AI-2 concentration in both colorectal tissue and stool of CRC patients was significantly higher when compared to that in AD and NC (all P values < .01). The AI-2 concentration along with the progression of CRC in both tissues and stools was significantly increased (P= .045,P= .0003, respectively). After AI-2 stimulation, TNFSF9 was the most significantly increased protein in macrophage cells (P < .01). TNFSF9 expression was significantly higher in CRC tissues when compared to NCs (P< .0001), which was mainly derived from macrophages in the tumor microenvironment. Moreover, AI-2 level was positively associated with CD3 + T cell numbers (P= .0462), and negatively associated with CD4/CD8 ratio (P= .0113) within CRC tissues.
Conclusions: We demonstrated for the first time that AI-2 may serve as a novel marker for screening CRC in the clinic. AI-2 was associated with tumor immunity in CRCs through tumor-associated macrophages and CD4/CD8 ratio in a TNFSF9-dependent manner.
Introduction
Mammals have coevolved with microbiota for millions of years, therefore, microbiota profoundly influence the homeostasis of host physiology.Citation1 In the gut of humans, roughly 100 billion microorganisms reside,Citation2 which play a key role in establishing gut mucosal immunity.Citation3 The imbalance of intestinal bacteria has been linked to various human diseases,Citation4 including obesity,Citation5 diabetes,Citation6 atherosclerotic cardiovascular disease,Citation7 neurogenic diseases like Alzheimer’s disease,Citation8 and colorectal cancer (CRC).Citation9
CRC has emerged as the third most common cancer worldwide.Citation10 A growing body of literature has recognized the importance of microbiota in the development and progress of CRC.Citation11 For example, Fusobacterium nucleatum (F. nucleatum) has recently been proposed to be associated with CRC,Citation12 through two types of virulence mechanisms: colonization and induction of the host immune response.Citation13 The dysbiosis of gut microbiota is closely related to CRC.Citation10 However, most studies up to now have not fully elucidated the exact mechanism involved in the progress of dysbiosis of gut bacteria that contributes to CRC. The host immunity has received considerable attention in the development of CRC; however, the detailed mechanism of interaction between gut microbiota and mucosal immunity remains to be elucidated.Citation14
The quorum sensing (QS) system, which is widely employed by bacteria, is an important mechanism of intercellular communication among bacterial species.Citation15 The QS system enables bacteria to act as a group rather than an individual cell.Citation16 Autoinducer-2 (AI-2), a major type of QS molecules that mediates the communication among interspecies,Citation15 has the ability to regulate gene expression, virulence and biofilm formation of bacteria.Citation1,Citation17 The colon mucosal biofilm has been shown to be associated with an increased risk for sporadic CRC.Citation18 Recent studies have revealed that AI-2 could potentially affect cytokine secretion of the host cells.Citation19 Therefore, it is likely that AI-2 plays a role in the development and progression of CRC.
Coevolved with resident microbiota over millennia, the immune system of the gut may have developed the ability to respond to QS molecules, such as AI-2 of inhabited bacteria.Citation20 Macrophages are immune cells that are vital for host defense, tissue repair, and inflammation.Citation21 It is generally recognized that macrophages are responsible for detecting microbial signals, thereby playing a critical role in microbial sensing.Citation22 Moreover, macrophages are the most abundant immune cells in the tumor microenvironment.Citation23 Recent discoveries have shown that gut microbiota plays an essential role in the carcinogenesis of CRC, and macrophages play an important role in this progress.Citation23 However, the exact role of macrophages in the interaction between gut microbiota and the immunity of CRC needs to be further investigated. Moreover, tumor-infiltrating lymphocytes (TILs) are important players in tumor immune surveillance, and each subpopulation of TILs possess a unique antitumor role.Citation24 CD4 + T cells and CD8 + T cells have been demonstrated to play an important role in antitumor immunity.Citation25,Citation26 In addition, CD4/CD8 ratio is a significant predictor of overall survival in patients with CRC.Citation27 Thus, macrophages or CD4/CD8 ratio in the tumor environment appear to be important in the anticancer immune response.
We hypothesize that AI-2 produced by gut microbiota may play a role in the carcinogenesis of CRC through immune cells of the gut mucosa. To test our hypothesis, we measured the concentration of AI-2 in clinical samples (stool, colorectal tissues, saliva, and serum) of CRC patients, and compared this to colorectal adenoma (AD) and normal colon mucosa (NC). Next, we utilized AI-2 that was produced by F. nucleatum to stimulate human macrophage cell line U-937, to explore the main AI-2-activated signaling pathway. Finally, we validated our in vitro findings in human CRC tissues, focusing on tumor-infiltrating macrophages and TILs. To our knowledge, this is the first time in which the association of AI-2 with the immunity of CRC was explored.
Materials and methods
Sample collection and preparation
All samples were collected from patients who underwent colonoscopy or surgery in the Affiliated Hospital of North Sichuan Medical College (Nanchong, China) and the Affiliated Hospital of Southwest Medical University (Luzhou, China). A total of 312 samples (stool, tissue, saliva and serum samples) were collected and processed as described in previous studies.Citation28,Citation29 Clinicopathological data were acquired according to hospital records. Informed consent was obtained from all participants, and the study was approved by the review board of the Affiliated Hospital of North Sichuan Medical College (Nanchong, China).
Bacterial strain and culture conditions
Vibrio harveyi (V. harveyi) strain BB170 was purchased from the Guangdong culture collection center and used to detect AI-2. Autoinducer bioassay (AB) media was prepared for culturing of cells and used for bioluminescence assays as described by Nilesh et al.Citation28 F. nucleatum strains F01 were isolated from CRC tissues and confirmed in our previous study.Citation30
Analysis of physiological samples
Standard solution of dihydroxy-2, 3-pentanedione (DPD) (Omm Scientific Inc. CAS: 710374–30-4, Dallas, TX, USA), the precursor of AI-2, was prepared as previously described.Citation31 Ten microliters of each of the processed samples was added in triplicate to 90 µl of diluted V. harveyi BB170 cultures in 96-well polystyrene microtiter plates (Costar, corning, NY, USA). The plates were incubated at 30°C and 160rpm in an orbital shaker, and bioluminescence was recorded by a TriStar LB 941 microplate reader (Berthold, Wildbad, Germany) and shown as a relative light unit (RLU). The concentration of AI-2 in each sample was calculated according to their bioluminescence value.Citation28
Purification of F. nucleatum (F01) AI-2
Overnight cultured F. nucleatum (F01) was diluted with fresh medium at a ratio of 1:10 and cultured until OD660 nm = 0.7, then the supernatant was collected by centrifugation at 13000 rpm for 10 min and 4°C. The supernatant was filtered through a 0.2-μm pore-size membrane filter and subsequently a Centricon YM-3 3-kDa exclusion filter. The filtrate was then chromatographed on a C18 Sep-Pak reverse-phase column (Waters Co, Milford, MA, USA) according to the manufacturer’s instructions.
Cell culture and treatment
U937 monocytes were purchased from BeNa culture collection (BNCC100498, Beijing, China), and RPMI medium 1640 supplemented with 10% FBS was used for cell culture purposes. For differentiation, U937 monocytes were cultured in 100 ng/mL phorbol 12-myristate 13-acetate (PMA) for 48 h. Then, U937-derived macrophages were challenged with AI-2 at a concentration of 400 μmol/L for 6 h and 37°C. Differentiated cells without AI-2 treatment were used as controls. Three experimental replicates were performed for each condition. Then, the cells were harvested and stored at −80°C for further use.
Quantitative proteomics analysis
Proteins were isolated and digested from AI-2-treated cells and control. After digestion, peptides were desalted and reconstituted in 0.5 M triethylammonium bicarbonate (TEAB) and processed using 6-plex TMT kit (Thermo Fisher Scientific, Waltham, MA, USA) according to the manufacturer’s protocol. Subsequently, the tryptic peptides were fractionated and analyzed as previously described.Citation32
Immunofluorescence analysis
Colorectal tissue samples were embedded in paraffin and sectioned at 5-μm thickness. Sections were blocked for 30 min and 37°C by 10% goat serum and incubated with primary antibodies: CD3 (1:100, R&D Systems, MN, USA); CD4 (1:100, Santa Cruz, CA, USA); CD8-α (1:100, Santa Cruz, CA, USA); CD68 (1:100, Santa Cruz, CA, USA); TNFSF9 (1:100, Bioworld, MN, USA) for 18 h at 4°C. Subsequently, each section was incubated with appropriate secondary antibodies for 1 h at 37°C. DAPI (Beyotime, China) was used for nuclear counterstain at room temperature for 10 min. Images were captured using a microscope (BX53; Olympus, Tokyo, Japan).
Quantification of immune cells
Immunofluorescence for immune cell markers (CD3, CD4, CD8, and CD68) was determined from captured pictures. As described in our previous study, the cell density of T cells and macrophages was determined by CD3+ or CD68+, respectively.Citation33 Subpopulations of T cells were determined by CD4+ or CD8+. A total of five random fields at 400× magnification were captured for each sample, and positive cells were presented as an average number of positive cells per square millimeter.Citation34
Statistical analysis
Data are presented as the mean ± standard deviation for continuous variables and proportions for categorical variables. Data were analyzed using one-way analysis of variance (ANOVA with Bonferroni correction) and unpaired t-test for parametric variables, and Kruskal–Wallis for nonparametric variables, as appropriate. For categorical variables, differences were determined by χ2 or Fisher exact tests. Pearson and Spearman correlation analysis were used for correlational analyses. All statistical calculations were performed using SPSS software Version 13.0 (SPSS Inc., Chicago, IL, USA). Differences were considered significant if P < .05.
Results
The AI-2 concentration in stools and colorectal tissues of patients with CRC was significantly higher when compared to that in adenomas and healthy controls
First, we measured the concentration of AI-2 in colorectal tissue, stool, saliva, and serum samples of patients with CRC, and compared this to AD and NC. The clinicopathological findings of patients are presented in Supplementary Table S1-S4. Among the three histological types, there were no significant differences in patient gender or age. The AI-2 concentration in both colorectal tissue and stool of CRC patients was significantly higher than that in ADs and NCs (all P< .01) (). In addition, the AI-2 concentration in stool samples of AD patients was significantly higher when compared to that of NCs (P < .01) (). In saliva samples, no significant differences were observed among CRC, AD and NC groups (P > .05) (). The AI-2 concentration in saliva of CRC patients was higher compared to that of ADs and NCs but did not reach statistical significance (P> .05). In serum samples, the concentration of AI-2 did not reach the minimum detection limit of the whole-cell biosensing system.
Figure 1. Comparison of AI-2 concentration in colorectal tissue, stool, and saliva samples of patients with colorectal cancer, colorectal adenoma, and healthy controls. (a) AI-2 concentration in colorectal tissues of CRC patients (n = 45) was significantly higher when compared to that of AD (n = 31) and NC (n = 20) (P< .0001, P< .0001, respectively). (b) The AI-2 concentration in stool samples from CRC patients (n = 53) was significantly higher when compared to that from AD (n = 31) and NC (n = 32) (P< .0001, P< .0001, respectively). Moreover, the AI-2 concentration in stool samples of AD patients was significantly higher when compared to that from NC (P< .01). (c) The AI-2 concentration in saliva samples showed no significant differences among CRC (n = 21), AD (n = 21) and NC (n = 21) (P =.8083). ****P <.0001, **P< .01, one-way ANOVA. NC, normal colon; AD, adenoma; CRC, colorectal cancer.
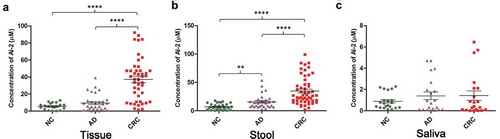
The AI-2 levels were increased with the progression of CRC staging in tumor tissues and stool samples
In this study, we investigated whether the AI-2 concentration was associated with CRC TNM staging. The clinicopathological findings of patients with different stages of CRC are presented in Supplementary Table S5, S6. In tissues and stool samples, no significant difference was found in patient age, sex, and tumor differentiation among different stages of CRC. The AI-2 concentration was significantly increased along with the progression of CRC in both tumor tissues and stool samples (P = .045,P = .0003, respectively) (). In addition, when compared to proximal CRC, the AI-2 levels were significantly higher in distal CRC in both tumor tissues and stool samples (P < .0001, P =.0101, respectively) ().
Figure 2. The level of AI-2 increased along with the progression of colorectal cancer in tumor tissues and stool samples and differed between proximal and distal colorectal cancer. (a) AI-2 concentration increased along with the progression of CRC in tumor tissues (P= .045). Stage I (n = 10), stage II (n = 12), stage III (n = 13), stage IV (n = 10). (b) AI-2 concentration increased along with the progress of CRC in stool samples (P= .0003). Stage I (n = 12), stage II (n = 14), stage III (n = 13), stage IV (n = 14). (c) The AI-2 concentration was significantly higher in distal CRC (n = 29) when compared to proximal CRC (n = 16) in tumor tissues (P <.0001). (d) AI-2 concentration was significantly higher in distal CRC (n = 32) when compared to proximal CRC (n = 21) in stool samples (P =.0101). ★ P< .05 compared to CRC stage I, # P< .05 compared to CRC stage II, * P< .05 compared to CRC stage III, Kruskal–Wallis test. ****P <.0001, *P< .05, unpaired t-test. CRC, colorectal cancer.
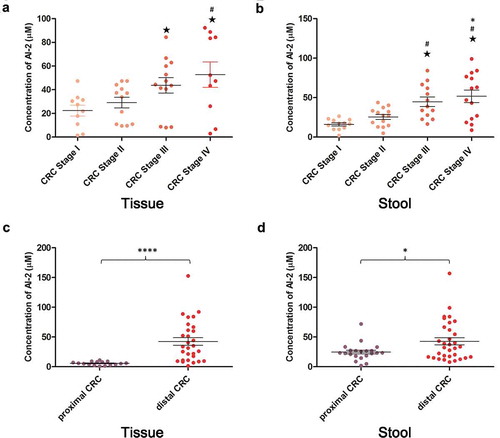
AI-2 treatment greatly increased TNFSF9 expression in macrophages in vitro
Macrophages in the gut mucosa play an important role in resisting bacterial pathogens.Citation22 Therefore, we wondered whether macrophages would respond to bacterial AI-2. After AI-2 treatment of U973-derived macrophages, a total of 5317 proteins were identified and quantified by comparing AI-2-treated U973-derived macrophages with non-treated controls using quantitative proteomics. In this study, a total of nine proteins showed an abundance change of at least twofold in AI-2 treated U973-derived macrophages when compared with controls: nine proteins were over-expressed whereas no proteins were down-regulated (). The results showed that TNFSF9 was the most significantly increased protein after AI-2 stimulation when compared to controls (P < .01). The findings suggested that TNFSF9 signaling may possibly be the main pathway in the response of macrophages to bacterial AI-2.
Table 1. Proteins with at least twofold change in abundance in AI-2 treated U973-derived macrophages compared with control.
TNFSF9 expression positively associated with AI-2 levels and human CRC progression
Next, we explored whether the expression of TNFSF9 was upregulated in human CRC tissues. TCGA and GTEx projects provided a large number of RNA sequencing data from tumor samples and normal tissues.Citation35 GEPIA (Gene Expression Profiling Interactive Analysis) is a visualization online website based on TCGA and GTEx data. We first explored the mRNA expression of TNFSF9 in CRC patients by using the GEPIA database, and found that the mRNA expression of TNFSF9 was significantly higher in CRC samples (n = 275) when compared to NCs (n = 41) (P < .05, ). Immunofluorescence analysis showed that TNFSF9 protein expression was significantly higher in CRC tissues when compared to NCs (P < .0001) (). Moreover, the expression of TNFSF9 increased with the progression of CRC (P < .0001) (). In addition, a positive correlation was found between TNFSF9 expression and AI-2 concentration within CRC tissues (Spearman correlation coefficient: 0.4218, 95% confidence interval [CI]: 0.0613 to 0.6849, P = .0203) ().
Figure 3. The TNFSF9 expression increased along with the progression of colorectal cancer and positively associated with the AI-2 concentration in tumor tissues. (a) Representative images of TNFSF9+ cells in CRC and normal tissues. Magnification, 400×; scale bars, 20 μm. (b) Using the GEPIA database, the mRNA expression of TNFSF9 was significantly higher in CRC samples (n = 275) when compared to normal samples (n = 41) (P< .05). (c) The density of TNFSF9+ cells was significantly higher in CRC tissues (n = 30) when compared to normal mucosa (n = 15) (P< .0001, unpaired t-test). (d) Representative images of TNFSF9+ cells in different stages of CRC. Magnification, 400×; scale bars, 20 μm. (e) The density of TNFSF9+ cells in tumors increased along with the progression of CRC. ★ P < .05 compared to CRC stage I. (f) Positive correlation between TNFSF9 expression and AI-2 concentration in CRC tissues (n = 30) (r = 0.4218, P= .0203, Spearman correlation analysis).
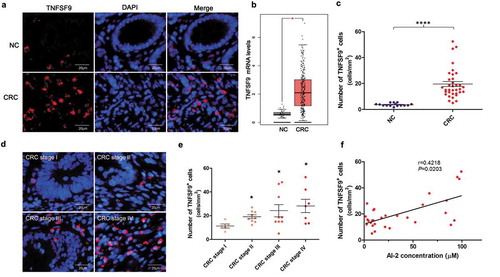
TNFSF9 was mainly derived from macrophages in the human CRC microenvironment
To delineate the cell source of high abundance of TNFSF9 in CRC microenvironment, multi-immunofluorescence was performed to analyze the co-staining of immune cells (CD3, CD68) and TNFSF9 in 30 CRCs and 15 paired NCs (). The co-staining of immune cells (CD68+ macrophages, CD3+ T cells) and TNFSF9 protein in CRC tissues were significantly higher when compared to that in NCs (P< .0001, P< .0001, respectively) (). In addition, the frequency of co-staining for CD68+ macrophages and TNFSF9 protein in CRCs was significantly higher when compared to that for CD3+ T cells and TNFSF9 (P = .0002) (). Together, these findings suggested that increased TNFSF9 expression in human CRC tissues was mainly derived from macrophages in the tumor microenvironment.
Figure 4. TNFSF9 was mainly derived from macrophages in the colorectal microenvironment. (a) The frequency of immunofluorescence co-staining for immune cells (CD3+, CD68+) and TNFSF9 in CRC tissues (n = 30) was significantly higher when compared to normal colorectal tissues (n = 15) (P< .0001, P< .0001, respectively). In CRC tissue, the frequency of immunofluorescence co-staining for macrophages (CD68+) and TNFSF9 was significantly higher when compared to that for CD3 + T cells and TNFSF9 (P< .05). (b, c) Representative images of co-staining for immune cells (CD3+, CD68+) and TNFSF9 in NC and CRC tissues. Magnification, 400×; scale bars, 20 μm. **** P <.0001, * P< .05, unpaired t-test. CRC, colorectal cancer; NC, normal colon.
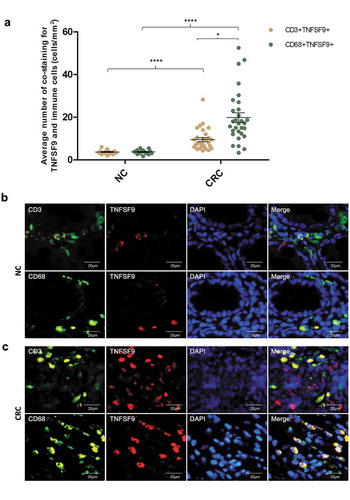
AI-2 concentration and TNFSF9 expression negatively associated with the CD4/CD8 ratio within the human CRC microenvironment
We further analyzed the T cell subpopulations (CD4+ T cells, CD8+ T cells) of TILs correlating to AI-2 concentration and TNFSF9 expression. We found a significantly positive correlation between CD3 + T cell numbers and AI-2 concentration (P= .0462), and between CD3+, CD8 + T cell numbers and TNFSF9 expression in CRCs (P= .0159, P= .0025, respectively) (). TILs in CRCs had a reversed CD4/CD8 ratio when compared to NCs (P< .0001) (). Moreover, the CD4/CD8 ratio negatively associated with AI-2 concentration (Spearman correlation coefficient: −0.4561, 95% CI: −0.7068 to −0.1036, P= .0113) and TNFSF9 expression (Spearman correlation coefficient: −0.5672, 95% CI: −0.7746 to −0.2495, P= .0011) within CRC tissues (). Combined, these results suggested that AI-2 and TNFSF9 may be associated with increased T cell numbers and decreased CD4/CD8 ratio within the CRC microenvironment.
Figure 5. The CD4/CD8 ratio was decreased within the human CRC microenvironment, and negatively associated with AI-2 concentration and TNFSF9 expression. (a) Representative images of subpopulations of tumor infiltration lymphocytes (CD4+ T cells and CD8+ T cells) in NC and CRC tissues. (b) Positive correlation between CD3 + T cell numbers and AI-2 concentration in CRCs (r = 0.3668, P= .0462, Pearson correlation analysis). (c) Positive correlation between CD8 + T cell numbers and TNFSF9 expression in CRCs (r = 0.5310, P= .0025, Spearman correlation analysis). (d) A reversed CD4/CD8 ratio was found in CRC when compared to NC (P< .0001, unpaired t-test). (e) Negative correlation between CD4/CD8 ratio and AI-2 concentration in CRC tissues (n = 30) (r = −0.4561, P= .0113, Spearman correlation analysis). (f) Negative correlation between CD4/CD8 ratio and TNFSF9 expression in CRC tissues (n = 30) (r = −0.5672, P= .0011, Spearman correlation analysis). CRC, colorectal cancer; NC, normal colon.
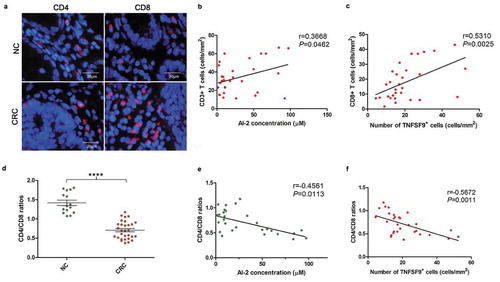
Discussion
In a number of studies, it has been suggested that gut microbiota plays a critical role in regulating host immunity and metabolism,Citation36 while the imbalance of gut microbiota was believed to lead to intestinal disorders by disturbing the immune system of the mucosa.Citation37 In a previous study, it was shown that in the mammalian gastrointestinal tract, AI-2 mediated communication among bacteria, thereby shaping the structure of the microbial community.Citation38 We demonstrated, for the first time, that the AI-2 concentration was significantly increased in cancer tissues and stool samples of CRC patients when compared to ADs and NCs. Another interesting finding was that the AI-2 concentration increased along with the progression of CRC. These findings indicated that AI-2 may play a role in the development and progression of CRC, possibly through influencing the microbial community in the human gut.
In addition, the concentration of AI-2 in distal CRC was significantly higher when compared to proximal CRC. This discrepancy may result from the different structures of microbiota in different subsites of the colon in CRC.Citation39 Importantly, these findings suggested that AI-2 may be a novel marker for screening CRC, especially for distal CRC. AI-2, as a QS molecule of gut microbiota, may serve as a prospective marker for screening CRC from a new visual angle that is different from the traditional method, which is based on the biological behavior of the tumor itself. Moreover, the detection of AI-2 in stool samples of patients would be a simple and feasible method for screening of CRC in the clinic.
Macrophages play a critical role in microbial sensing.Citation22 We hypothesized that AI-2 may be sensed and responded to by the gut immune system, especially macrophages in the gut mucosa. Using quantitative proteomics analysis, the most significant increase of TNFSF9 was found in macrophages that were treated with AI-2 extracts from F. nucleatum. In previous studies, we demonstrated that F. nucleatum plays an essential role in the progression of CRC.Citation33,Citation40–Citation42 Furthermore, we observed that the expression of TNFSF9 protein was significantly higher in human CRC tissue when compared to adjacent normal tissue, and a positive correlation was found between AI-2 concentration and TNFSF9 expression. Our findings were consistent with previous studies, in which was revealed that TNFSF9 expression was upregulated in CRC tissues.Citation43,Citation44 Our results demonstrated that AI-2 may be associated with the development and progression of CRC through macrophages involving TNFSF9 signaling.
Interestingly, it has recently been reported that mammalian epithelial cells of the tumor produced AI-2 mimics, which have AI-2 activity.Citation1 Communication between host cells and bacteria occurs by generating AI-2 mimics that affect gene expression of bacteria through the AI-2 pathway in return.Citation1 Thus, AI-2, a small molecule generated by both bacteria and the host, may act as a hub for communication between gut microbiota and immunity of host colon mucosa. Moreover, AI-2 produced by gut microbiota could be sensed by the gut immune system, thereby contributing to the development of microbiota-related diseases, such as CRC.
TNFSF9 expression was mainly found on several antigen-presenting cells, such as macrophages.Citation45 In our study, TNFSF9 in the microenvironment of CRC was mainly derived from tumor-associated macrophages (TAMs). It is reported that high macrophage infiltration correlated with improved survival for CRC patients.Citation46 Furthermore, TNFSF9 signaling has been proven to have potential effects on T cells, including both CD4+ and CD8+ T cells.Citation47 Infiltration of CD8 + T-cells within cancer tissues has been demonstrated to contribute to a better survival of CRC patients.Citation25,Citation48 Moreover, CRC patients with low CD4/CD8 ratios had favorable overall survival.Citation27,Citation49 In this study, we found a positive correlation between CD3 + T cell numbers and AI-2/TNFSF9 levels, and between CD8 + T cell numbers and TNFSF9 expression in CRCs, suggesting that AI-2/TNFSF9 levels were associated with anti-tumor immunity in CRC patients. Moreover, our results showed that the CD4/CD8 ratio negatively associated with AI-2 concentration within CRC tissues. The association of AI-2 level in CRC patients with overall survival needs to be investigated in future studies. Taken together, these findings demonstrated that AI-2 produced by gut microbiota was associated with tumor immunity in CRC patients through TAMs and CD4/CD8 ratio in a TNFSF9-dependent manner.
Disclosure of interest
The authors report no conflict of interest.
Supplemental Material
Download Zip (46.8 KB)Supplementary material
Supplemental data for this article can be accessed on the publisher’s website.
Additional information
Funding
References
- Ismail AS, Valastyan JS, Bassler BL. A host-produced autoinducer-2 mimic activates bacterial quorum sensing. Cell Host Microbe. 2016;19(4):470–480. doi:10.1016/j.chom.2016.02.020.
- Larroya-Garcia A, Navas-Carrillo D, Orenes-Pinero E. Impact of gut microbiota on neurological diseases: diet composition and novel treatments. Crit Rev Food Sci Nutr. 2018:1–15. doi:10.1080/10408398.2018.1484340.
- Belkaid Y, Hand TW. Role of the microbiota in immunity and inflammation. Cell. 2014;157(1):121–141. doi:10.1016/j.cell.2014.03.011.
- Hall AB, Tolonen AC, Xavier RJ. Human genetic variation and the gut microbiome in disease. Nat Rev Genet. 2017;18(11):690–699. doi:10.1038/nrg.2017.63.
- Maruvada P, Leone V, Kaplan LM, Chang EB. The human microbiome and obesity: moving beyond associations. Cell Host Microbe. 2017;22(5):589–599. doi:10.1016/j.chom.2017.10.005.
- Forslund K, Hildebrand F, Nielsen T, Falony G, Le Chatelier E, Sunagawa S, Prifti E, Vieira-Silva S, Gudmundsdottir V, Pedersen HK, et al. Disentangling type 2 diabetes and metformin treatment signatures in the human gut microbiota. Nature. 2015;528(7581):262–266. doi:10.1038/nature15766.
- Jie Z, Xia H, Zhong SL, Feng Q, Li S, Liang S, Zhong H, Liu Z, Gao Y, Zhao H, et al. The gut microbiome in atherosclerotic cardiovascular disease. Nat Commun. 2017;8(1):845. doi:10.1038/s41467-017-00900-1.
- Wu SC, Cao ZS, Chang KM, Juang JL. Intestinal microbial dysbiosis aggravates the progression of Alzheimer’s disease in Drosophila. Nat Commun. 2017;8(1):24. doi:10.1038/s41467-017-00040-6.
- Scott TA, Quintaneiro LM, Norvaisas P, Lui PP, Wilson MP, Leung KY, Herrera-Dominguez L, Sudiwala S, Pessia A, Clayton PT, et al. Host-microbe co-metabolism dictates cancer drug efficacy in C. elegans. Cell. 2017;169(3):442–56e18. doi:10.1016/j.cell.2017.03.040.
- Kwong TNY, Wang X, Nakatsu G, Chow TC, Tipoe T, Dai RZW, Tsoi KKK, Wong MCS, Tse G, Chan MTV, et al. Association between bacteremia from specific microbes and subsequent diagnosis of colorectal cancer. Gastroenterology. 2018;155(2):383–90 e8. doi:10.1053/j.gastro.2018.04.028.
- Ahn J, Sinha R, Pei Z, Dominianni C, Wu J, Shi J, Goedert JJ, Hayes RB, Yang L. Human gut microbiome and risk for colorectal cancer. J Natl Cancer Inst. 2013;105(24):1907–1911. doi:10.1093/jnci/djt300.
- Sears CL. The who, where and how of fusobacteria and colon cancer. eLife. 2018:7. doi:10.7554/eLife.28434.
- Han YW. Fusobacterium nucleatum: a commensal-turned pathogen. Curr Opin Microbiol. 2015:23141–23147. doi:10.1016/j.mib.2014.11.013.
- Hooper LV, Littman DR, Macpherson AJ. Interactions between the microbiota and the immune system. Science. 2012;336(6086):1268–1273. doi:10.1126/science.1223490.
- Galloway WR, Hodgkinson JT, Bowden SD, Welch M, Spring DR. Quorum sensing in Gram-negative bacteria: small-molecule modulation of AHL and AI-2 quorum sensing pathways. Chem Rev. 2011;111(1):28–67. doi:10.1021/cr100109t.
- Kaper JB, Sperandio V. Bacterial cell-to-cell signaling in the gastrointestinal tract. Infect Immun. 2005;73(6):3197–3209. doi:10.1128/IAI.73.6.3197-3209.2005.
- Federle MJ. Autoinducer-2-based chemical communication in bacteria: complexities of interspecies signaling. Contrib Microbiol. 2009:1618–1632. doi:10.1159/000219371.
- Yu J, Chen Y, Fu X, Zhou X, Peng Y, Shi L, Chen T, Wu Y. Invasive Fusobacterium nucleatum may play a role in the carcinogenesis of proximal colon cancer through the serrated neoplasia pathway. Int J Cancer. 2016;139(6):1318–1326. doi:10.1002/ijc.30168.
- Zargar A, Quan DN, Carter KK, Guo M, Sintim HO, Payne GF, Bentley WE. Bacterial secretions of nonpathogenic Escherichia coli elicit inflammatory pathways: a closer investigation of interkingdom signaling. mBio. 2015;6(2):e00025. doi:10.1128/mBio.00025-15.
- Scheres N, Lamont RJ, Crielaard W, Krom BP. LuxS signaling in Porphyromonas gingivalis-host interactions. Anaerobe. 2015;35(Pt A):3–9. doi:10.1016/j.anaerobe.2014.11.011.
- Murray PJ, Wynn TA. Protective and pathogenic functions of macrophage subsets. Nat Rev Immun. 2011;11(11):723–737. doi:10.1038/nri3073.
- Mortha A, Chudnovskiy A, Hashimoto D, Bogunovic M, Spencer SP, Belkaid Y, Merad M. Microbiota-dependent crosstalk between macrophages and ILC3 promotes intestinal homeostasis. Science. 2014;343(6178):1249288. doi:10.1126/science.1249288.
- Bader JE, Enos RT, Velazquez KT, Carson MS, Nagarkatti M, Nagarkatti PS, Chatzistamou I, Davis JM, Carson JA, Robinson CM, et al. Macrophage depletion using clodronate liposomes decreases tumorigenesis and alters gut microbiota in the AOM/DSS mouse model of colon cancer. Am J Physiol Gastrointest Liver Physiol. 2018;314(1):G22–G31. doi:10.1152/ajpgi.00229.2017.
- Umansky V, Schirrmacher V, Rocha M. New insights into tumor-host interactions in lymphoma metastasis. J Mol Med. 1996;74:353–363.
- Naito Y, Saito K, Shiiba K, Ohuchi A, Saigenji K, Nagura H, Ohtani H. CD8+ T cells infiltrated within cancer cell nests as a prognostic factor in human colorectal cancer. Cancer Res. 1998;58:3491–3494.
- Dudley ME, Wunderlich JR, Robbins PF, Yang JC, Hwu P, Schwartzentruber DJ, Topalian SL, Sherry R, Restifo NP, Hubicki AM, et al. Cancer regression and autoimmunity in patients after clonal repopulation with antitumor lymphocytes. Science. 2002;298(5594):850–854. doi:10.1126/science.1076514.
- Diederichsen AC, Hjelmborg J, Christensen PB, Zeuthen J, Fenger C. Prognostic value of the CD4+/CD8+ ratio of tumour infiltrating lymphocytes in colorectal cancer and HLA-DR expression on tumour cells. Cancer Immunol Immunother. 2003;52(7):423–428. doi:10.1007/s00262-003-0388-5.
- Raut N, Pasini P, Daunert S. Deciphering bacterial universal language by detecting the quorum sensing signal, autoinducer-2, with a whole-cell sensing system. Anal Chem. 2013;85(20):9604–9609. doi:10.1021/ac401776k.
- Raut N, Joel S, Pasini P, Daunert S. Bacterial autoinducer-2 detection via an engineered quorum sensing protein. Anal Chem. 2015;87(5):2608–2614. doi:10.1021/ac504172f.
- Chen Y, Peng Y, Yu J, Chen T, Wu Y, Shi L, Li Q, Wu J, Fu X. Invasive Fusobacterium nucleatum activates beta-catenin signaling in colorectal cancer via a TLR4/P-PAK1 cascade. Oncotarget. 2017;8(19):31802–31814. doi:10.18632/oncotarget.15992.
- Song J, Qin Q, Li T, Ren F, Zhang H, Xie Y, Jin J. Impact of carbohydrates on autoinducer-2 secretion of Bifidobacterium longum subsp. longum BBMN68. Lett Appl Microbiol. 2018;66(4):340–346. doi:10.1111/lam.12854.
- Li L, Shi L, Yang S, Yan R, Zhang D, Yang J, He L, Li W, Yi X, Sun L, et al. SIRT7 is a histone desuccinylase that functionally links to chromatin compaction and genome stability. Nat Commun. 2016;712235. doi:10.1038/ncomms12235.
- Chen T, Li Q, Wu J, Wu Y, Peng W, Li H, Wang J, Tang X, Peng Y, Fu X. Fusobacterium nucleatum promotes M2 polarization of macrophages in the microenvironment of colorectal tumours via a TLR4-dependent mechanism. Cancer Immunol Immunother. 2018;67(10):1635–1646. doi:10.1007/s00262-018-2233-x.
- Nosho K, Baba Y, Tanaka N, Shima K, Hayashi M, Meyerhardt JA, Giovannucci E, Dranoff G, Fuchs CS, Ogino S. Tumour-infiltrating T-cell subsets, molecular changes in colorectal cancer, and prognosis: cohort study and literature review. J Pathol. 2010;222(4):350–366. doi:10.1002/path.2774.
- Tang Z, Li C, Kang B, Gao G, Li C, Zhang Z. GEPIA: a web server for cancer and normal gene expression profiling and interactive analyses. Nucleic Acids Res. 2017;45(W1):W98–W102. doi:10.1093/nar/gkx247.
- Wu H, Tremaroli V, Backhed F. Linking microbiota to human diseases: a systems biology perspective. Trends Endocrinol Metab. 2015;26(12):758–770. doi:10.1016/j.tem.2015.09.011.
- Dzutsev A, Badger JH, Perez-Chanona E, Roy S, Salcedo R, Smith CK, Trinchieri G. Microbes and Cancer. Annu Rev Immunol. 2017:35199–35228. doi:10.1146/annurev-immunol-051116-052133.
- Thompson JA, Oliveira RA, Djukovic A, Ubeda C, Xavier KB. Manipulation of the quorum sensing signal AI-2 affects the antibiotic-treated gut microbiota. Cell Rep. 2015;10(11):1861–1871. doi:10.1016/j.celrep.2015.02.049.
- Flemer B, Lynch DB, Brown JM, Jeffery IB, Ryan FJ, Claesson MJ, O’Riordain M, Shanahan F, O’Toole PW. Tumour-associated and non-tumour-associated microbiota in colorectal cancer. Gut. 2017;66(4):633–643. doi:10.1136/gutjnl-2015-309595.
- Chen T, Li Q, Zhang X, Long R, Wu Y, Wu J, Fu X. TOX expression decreases with progression of colorectal cancers and is associated with CD4 T-cell density and Fusobacterium nucleatum infection. Hum Pathol. 2018: 7993–101. doi:10.1016/j.humpath.2018.05.008.
- Wu Y, Wu J, Chen T, Li Q, Peng W, Li H, Tang X, Fu X. Fusobacterium nucleatum potentiates intestinal tumorigenesis in mice via a toll-like receptor 4/p21-activated kinase 1 cascade. Dig Dis Sci. 2018;63(5):1210–1218. doi:10.1007/s10620-018-4999-2.
- Dejea CM, Fathi P, Craig JM, Boleij A, Taddese R, Geis AL, Wu X, DeStefano Shields CE, Hechenbleikner EM, Huso DL, et al. Patients with familial adenomatous polyposis harbor colonic biofilms containing tumorigenic bacteria. Science. 2018;359(6375):592–597. doi:10.1126/science.aah3648.
- Fujimoto H, Saito Y, Ohuchida K, Kawakami E, Fujiki S, Watanabe T, Ono R, Kaneko A, Takagi S, Najima Y, et al. Deregulated Mucosal Immune Surveillance through Gut-Associated Regulatory T Cells and PD-1(+) T Cells in Human Colorectal Cancer. J Immunol. 2018;200(9):3291–3303. doi:10.4049/jimmunol.1701222.
- Akiyama Y, Kondou R, Iizuka A, Ohshima K, Urakami K, Nagashima T, Shimoda Y, Tanabe T, Ohnami S, Ohnami S, et al. Immune response-associated gene analysis of 1,000 cancer patients using whole-exome sequencing and gene expression profiling-Project HOPE. Biomed Res. 2016;37(4):233–242. doi:10.2220/biomedres.37.233.
- Wang Q, Zhang P, Zhang Q, Wang X, Li J, Ma C, Sun W, Zhang L. Analysis of CD137 and CD137L expression in human primary tumor tissues. Croat Med J. 2008;49(2):192–200. doi:10.3325/cmj.2008.2.192.
- Forssell J, Oberg A, Henriksson ML, Stenling R, Jung A, Palmqvist R. High macrophage infiltration along the tumor front correlates with improved survival in colon cancer. Clin Cancer Res. 2007;13(5):1472–1479. doi:10.1158/1078-0432.CCR-06-2073.
- Kudo-Saito C, Hodge JW, Kwak H, Kim-Schulze S, Schlom J, Kaufman HL. 4-1BB ligand enhances tumor-specific immunity of poxvirus vaccines. Vaccine. 2006;24(23):4975–4986. doi:10.1016/j.vaccine.2006.03.042.
- Pages F, Mlecnik B, Marliot F, Bindea G, Ou FS, Bifulco C, Lugli A, Zlobec I, Rau TT, Berger MD, et al. International validation of the consensus Immunoscore for the classification of colon cancer: a prognostic and accuracy study. Lancet. 2018;391(10135):2128–2139. doi:10.1016/S0140-6736(18)30789-X.
- Lavotshkin S, Jalas JR, Torisu-Itakura H, Ozao-Choy J, Lee JH, Sim MS, Stojadinovic A, Wainberg Z, Bifulco CB, Fox BA, et al. Immunoprofiling for prognostic assessment of colon cancer: a novel complement to ultrastaging. J Gastrointest Surg. 2015;19(6):999–1006. doi:10.1007/s11605-015-2759-6.