ABSTRACT
MicroRNA 155 (miR-155) plays important roles in the regulation of the development and functions of a variety of immune cells. We previously revealed a vital role of miR-155 in regulating the function of dendritic cells (DCs) in breast cancer. miR-155 deficiency in DCs impaired their maturation, migration, cytokine production, and ability to activate T cells. In the current study, to exploit the therapeutic value of miR-155 for breast cancer, we examined the impact of overexpression of miR-155 on antitumor responses generated by DC vaccines. We boosted miR-155 expression in DCs by generating a miR-155 transgenic mouse strain (miR-155tg) or using lentivirus transduction. DCs overexpressing miR-155 exhibited enhanced functions in response to tumor antigens. Using miR-155 overexpressing DCs, we generated a DC vaccine and found that the vaccine resulted in enhanced antitumor immunity against established breast cancers in mice, demonstrated by increased effector T cells in the mice, suppressed tumor growth, and drastically reduced lung metastasis. Our current study suggests that in future DC vaccine development for breast cancer or other solid tumors, introducing forced miR155 overexpression in DCs via various approaches such as viral transduction or nanoparticle delivery, as well as including other adjuvant agents such as TLR ligands or immune stimulating cytokines, may unleash the full therapeutic potential of the DC vaccines.
Introduction
Cancer immunotherapy attempts to harness the power of the immune system to target tumors. Effective antitumor immunity requires the generation and persistence of functional tumor-specific T cell responses. Dendritic cells (DCs) are professional antigen-presenting cells (APCs) that drive T cell-mediated immune responses. They capture and process antigens, migrate into lymphoid organs, present antigens, express lymphocyte costimulatory molecules, and secrete cytokines that initiate immune responses. Thus, DCs are the major link between the innate and adaptive immune responses. Recent reports show that DCs pulsed with tumor lysates in vitro and in vivo drive increased therapeutic antitumor immune responses after vaccination.Citation1-Citation3 Although the use of DCs in immunotherapy holds promise for cancer treatment, there are still obstacles that need to be overcome, such as tumor microenvironment-mediated inhibition of DC maturation leading to tumor escape from immune surveillance.Citation4
MicroRNAs (miRNAs) are endogenous, small, noncoding RNAs that regulate protein expression post-transcriptionally. microRNA-155 (miR-155) was one of the first miRNAs shown to play regulatory roles in the development and function of multiple immune cells.Citation5,Citation6 It is derived from the noncoding transcript of B-cell integration cluster (bic) and is essential for normal B cell differentiation and antibody production.Citation5-Citation7 miR-155 also regulates the differentiation of CD4+ T cells through Th1, Th2, and Th17 pathwaysCitation8-Citation10 and affects the development of regulatory T (Treg) cells.Citation11 Furthermore, miR-155 is required for CD8+ T cell responses to acute viral and bacterial challenges.Citation12-Citation15
Our previous studies and those from other groups have shown that miR-155 deficiency in DCs inhibits DC maturation, migration, subsequent T cell activation, and cytokine production by directly targeting c-fos, Arg-2, SOCS-1 and Jarid2 in DCs.Citation6,Citation16-Citation18 We found that miR-155 expression is increased during DC activation during the initiation of the anti-tumor immune response against breast cancer; however, cytokines, such as IL-10 and IL-6 in the tumor microenvironment impair DC activation and thus blunt anti-tumor immunity.Citation16 In this study, we examine the impact of miR-155 overexpression on DC vaccine-induced immune activation and test the feasibility of miR-155 overexpression as a strategy to improve the antitumor potency of DC vaccines. We show that miR-155 overexpressing DCs are highly effective in promoting functional activation of T cells and antitumor activity against breast cancer.
Results
miR-155-overexpressing bone marrow cells reduce tumor growth and lung metastasis
We previously showed that host miR-155 deficiency promotes breast cancer growth by impairing dendritic cell functions.Citation16 In order to examine the impact of miR-155 overexpression on immune cell functions, we generated the first whole body miR-155 transgenic (miR-155tg) mouse model (Fig. S1). There is no obvious phenotype associated with miR-155 transgenic expression in healthy transgenic mice compared with wild type (WT) mice, including growth curve, body weight, and organ weights (data not shown). Compared to WT mice, miR-155tg mice have more CD3+/CD4+ and CD3+/CD8+ T cells and fewer CD19+ B cells in the spleen; and while the transgenic mice have comparable total CD11c+ dendritic cells and F4/80+ macrophages in the spleen, their splenic macrophages express higher levels of MHCII (Fig. S2). To examine whether enhanced miR-155 expression in immune cells could lead to improved anti-tumor immunity in tumor-bearing mice, we performed a bone marrow transplantation (BMT) study. Lethally irradiated WT C57BL/6 mice were reconstituted with either WT or miR-155tg bone marrow cells. After 4 weeks, WT and miR-155tg chimeric mice (referred as WT-BMT and miR-155tg-BMT hereafter, respectively) were inoculated with EO771 breast cancer cells ()). At the endpoint of the experiment, miR-155 expression in bone marrow cells was determined by qPCR, and there was an average ~10-fold higher level of miR-155 expression in the bone marrow cells from miR-155tg-BMT mice than those from WT-BMT mice, confirming the successful bone marrow reconstitution (). Mice transplanted with miR-155tg bone marrow cells exhibited significantly attenuated tumor growth () and reduced lung metastasis ().
Figure 1. miR-155-overexpressing bone marrow cells reduce tumor growth and lung metastasis. (a) Timeline of this experiment. Seven-week-old, female C57BL/6 mice received oral antibiotics for 2 weeks both prior to and after lethal irradiation and bone marrow transplantation with either WT or miR-155tg bone marrow. 4 weeks after BMT, 2 × 105 EO771 tumor cells were implanted into each of the 4th pair of mammary fat pads, tumor growth was monitored and the mice were sacrificed 35 days after tumor inoculation. (b) miR-155 expression in bone marrow (BM) of all mice at the conclusion of the study to confirm successful bone marrow transplant. Tumor growth over 35 days (c) and tumor weights (d) at sacrifice. Manual counting of lung surface metastases (e) and measured average size determined using serial lung sections (f). Results are shown as means ± SEM (n = 10). *p < .05, **p < .01, ***p < .005
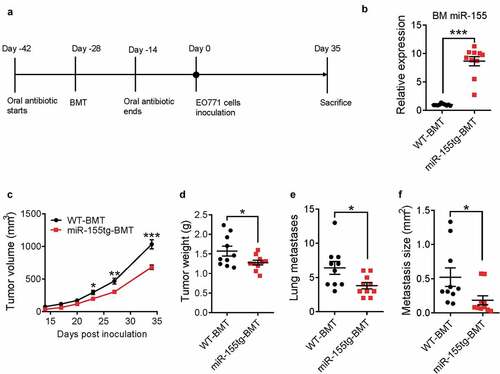
miR-155-overexpressing bone marrow cells elicit enhanced antitumor response
To investigate whether miR-155 overexpression in bone marrow cells affects antitumor immune responses, we analyzed immune cells in the blood, spleen, and tumor-draining lymph nodes from the mice in the aforementioned experiment by flow cytometry at the endpoint (35 days after tumor cell inoculation). In the blood, there was no difference in total T cells (), but there were more CD8+ T cells and less CD4+ T cells in the miR-155tg-BMT mice (). Furthermore, we found that both spleens and tumor-draining lymph nodes contained significantly more cells in the miR-155tg-BMT mice; particularly, compared to WT-BMT mice, miR-155tg-BMT mice had significantly more CD8+ T cells in the spleen (), indicating that there was likely a more robust anti-tumor immune response in the miR-155tg-BMT mice, both locally and, to some degree, systemically. More importantly, we found that the numbers of IFNγ-expressing CD8+ T cells were significantly increased in the spleens and tumor-draining lymph nodes of miR-155tg-BMT mice (; Fig. S3), suggesting that an increase of cytotoxic T cells may be involved in the observed suppression of EO771 tumor growth and metastasis.
Figure 2. miR155 overexpressing bone marrow cells elicit enhanced antitumor response. Total circulating T cells (a) and CD8+ (b) and CD4+ (c) T cells of WT or miR-155tg BMT mice carrying EO771 breast tumor. (n = 5). Total cells, and CD8+ or CD4+ T cells in spleens (d) and tumor-draining lymph nodes (e). (n = 10). Absolute numbers of active cytotoxic T cells (CD8+IFNγ+) in spleens (f) and tumor draining lymph nodes (g). (n = 5). Results are shown as means ± SEM (n = 5 or 10). *p < .05, **p < .01, ***p < .005
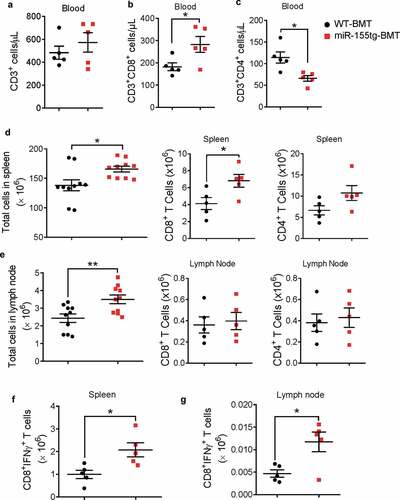
miR-155 overexpression promotes dendritic cell maturation and migration
DCs are central regulators of the adaptive immune response and are necessary for T-cell-mediated cancer immunity. The DC-mediated antitumor immunity relies on mature DCs that provide both tumor antigens and costimulatory signals to activate T cells.Citation4 We have previously discovered that miR-155 deficiency in DCs impairs their maturation, migration, cytokine production, and their ability to activate T cells.Citation16 Here we determined how elevated miR-155 expression affects DC maturation. In the bone marrow reconstitution model described above, we found that there were significantly more CD11c+ DCs in the tumors and tumor draining lymph nodes, while the CD11c+ cells in the spleen exhibited a similar trend but the increase did not reach statistical significance ()) in miR-155tg-BMT mice. These DCs also expressed much higher levels of maturation markers (MHCII, CD40, CD80, and CD86) in the spleen, tumor-draining lymph nodes, and tumors (). To further determine whether miR-155 overexpression directly promotes DC maturation, we generated bone marrow-derived DCs (BMDCs) from WT and miR-155tg mice (Fig. S4) and measured MHC-II and costimulatory markers after pulsing these DCs with tumor antigen (EO771 cell lysate and EO771-conditioned medium, TuAg). Consistent with the in vivo results, we found higher expression of MHC-II, CD80, and CD86 on miR-155tg BMDCs than WT BMDCs ().
Figure 3. miR155 overexpression increases dendritic cell frequency, maturation, and migration. (a) DC frequencies in the spleens, tumors and lymph nodes of tumor bearing mice. (n = 5). (b, c) DC maturation of splenic, tumor and lymph node DCs was determined by MHCII, CD40, CD80, and CD86 staining. (n = 5). (d) miR155tg and WT BMDCs were pulsed with tumor antigen (TuAg) and stained for maturation markers (MHCII, CD80, and CD86), and analyzed with flow cytometry. (n = 3). (e) Frequencies of CCR7 highly expressed DCs (CCR7hi) in tumor and lymph node of WT or miR-155tg BMT mice. (n = 5). (f) CCR7 expression on WT and miR155tg BMDCs with or without TuAg treatment. (n = 3). (g) WT and miR155tg DCs were pulsed with tumor material, then exposed to a CCL19 gradient. Migration in response to CCL19 through a trans-well insert was quantified (left) and representative images were shown (right). (n = 3). Results are shown as means ± SEM. *p < .05, **p < .01, ***p < .005
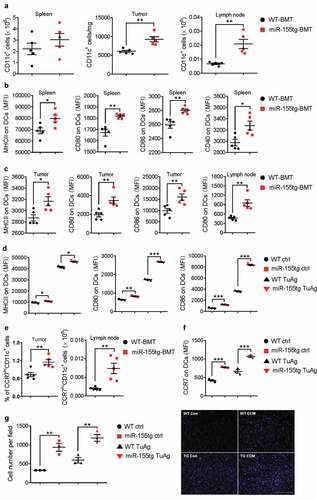
The binding of C-C chemokine receptor type 7 (CCR7) to its ligands C-C motif chemokine ligands 19 and 21 (CCL19 and CCL21) is the driving force for DC migration toward draining lymph nodes, which is an essential step for eliciting T cell activation and expansion.Citation19 We have previously reported that miR-155 can manipulate CCR7 expression at the epigenetic level.Citation16 As expected, CCR7 expression was found to be much higher on DCs in the tumor and tumor-draining lymph nodes of miR-155tg-BMT mice (). Consistently, CCR7 expression is drastically increased in both tumor antigen-pulsed and naïve miR-155tg BMDCs in vitro (). We next performed a trans-well migration assay, and found that miR-155 overexpression promoted BMDC migration in response to a gradient of CCL19 (). Overall, these results suggest that overexpression of miR-155 in DCs challenged by tumor antigens promotes their maturation and migration to the draining lymph nodes where they encounter and activate T cells.
miR-155 overexpression enhances the capability of DCs to activate T cells
To evaluate if miR-155 overexpression in DCs increases their ability to stimulate T cell activation and proliferation, we co-cultured tumor antigen-inexperienced splenic T cells from healthy, WT mice with WT or miR-155tg BMDCs pulsed with tumor antigens. miR-155tg BMDCs induced much stronger activation of CD4+ and CD8+ T cells in terms of CD69 expression and intracellular IFN-γ production than WT BMDCs (). Also, consistent with this result, miR-155 overexpression enhanced the capacity of DCs to induce T cell expansion as demonstrated by a CFSE proliferation assay (); Fig. S5).
Figure 4. miR155 overexpression enhances the capability of DCs to activate T cells. WT and miR155tg DCs were pulsed with tumor antigen, then co-cultured with tumor antigen-inexperienced WT T cells. (a) After 48 hours of co-culture, T cells were stained for CD3, CD4, CD8, CD69, and IFNγ, then analyzed using flow cytometry. (n = 3). (b) Some tumor antigen-inexperienced T cells were stained with CFSE prior to co-culture; after 120 hours of co-culture, T cell CFSE fluorescence was analyzed using flow cytometry. The “Proliferation” tool in FlowJo was used to analyze the samples and assign the Proliferation Index. (n = 3). (c–e) Tumor-bearing WT mice were s.c. injected twice with tumor antigen-pulsed WT or miR155tg DCs. Tumors, spleens, and tumor draining lymph nodes were then harvested, and dissociated to a single cell suspension. Tumor-draining lymph node and spleen cellularity after tumor antigen pulsed DCs s.c. injections. (n = 6–8) (c). Activation of CD4+ (d) or CD8+ (e) T cells was analyzed using CD45, CD3, CD4, CD8, and CD69 antibodies and flow cytometry. Results are shown as means ± SEM. (n = 3–8). *p < .05, **p < .01, ***p < .005
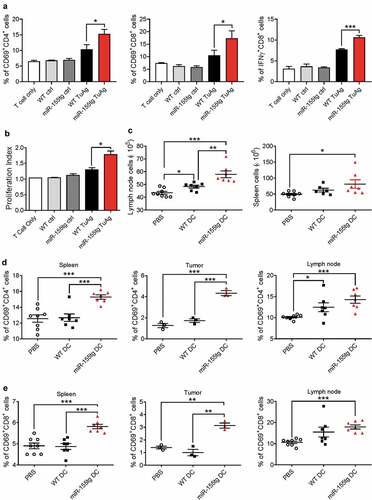
We further examined the capability of miR-155-overexpressing DCs to activate T cells in vivo. Mice were immunized with tumor-pulsed miR-155tg and WT BMDCs 1 day and 5 days after tumor cell implantation. Seven days after tumor cell implantation, mice were sacrificed and tumors, spleens, and tumor-draining lymph nodes were evaluated for T cell activation. It was noted that the cellularity of tumor-draining lymph nodes and spleens was increased in mice that had received miR-155tg BMDCs (). When compared to mice immunized with WT BMDCs, mice immunized with miR-155tg BMDCs exhibited significantly more robust activation of CD4+ and CD8+ T cells in tumors, spleens, and tumor-draining lymph nodes (). Given the increased T cell activation in vivo and in vitro, this change in the numbers of lymph node and spleen cells is likely due to an augmented anti-tumor immune response initiated by the introduction of mature miR-155tg DCs. Interestingly, both WT and miR-155tg BMDC injections reduced total macrophages (CD11b+F4/80+) and MDSCs (CD11b+Gr1+) in the tumors to a similar level (Fig. S6).
In order to further confirm the findings that miR-155-overexpressing DCs effectively enhance anti-tumor immune responses, we also augmented miR-155 expression in DCs by lentivirus-mediated overexpression. miR-155-overexpressing BMDCs were generated by transduction using mouse bic lentiviruses previously generated in our laboratory.Citation20 The transduction efficiency was 20% ~ 40% and the overall miR-155 expression level was increased by ~1.9-fold (Fig. S7). It was found that miR-155 lentivirus-transduced BMDCs (LV-Bic DCs) exhibited higher expression levels of DC maturation markers than control lentivirus-transduced BMDCs (LV-pWPI DCs) after being pulsed with tumor lysates and conditioned medium (); Fig. S8). When these LV-Bic DCs were administered to EO771 tumor cell inoculated mice after the tumor cells were injected for 24 h, larger tumor-draining lymph nodes () and improved T cell activation () were observed 48 h after LV-Bic DC administration compared to those in LV-pWPI DC-injected mice. These results again indicate that a stronger early anti-tumor immune response was elicited by miR-155-overexpressing DCs, demonstrating enhanced effectiveness of this DC-based vaccine even in the immune-suppressive microenvironment present in the tumor-bearing mice.
Figure 5. Lentivirus-mediated miR155 overexpression enhances the capability of DCs to activate T cells. (a) BMDCs infected with LV-pWPI or LV-Bic were pulsed with tumor material, stained for maturation markers (MHCII, CD80, and CD86), and analyzed with flow cytometry. (n = 3). (b) Tumor-bearing WT mice were s.c. injected tumor-pulsed LV-pWPI or LV-Bic DCs. Tumor draining lymph nodes were weighed and total cells were counted using a glass slide hemocytometer. (n = 6). (c) Tumor draining lymph nodes were then harvested, dissociated to a single cell suspension, and stained with CD3, CD4, CD8, CD69 or IFNγ antibodies. Stained cells were analyzed by flow cytometry. (n = 5–6). Results are shown as means ± SEM. *p < .05, **p < .01, ***p < .005
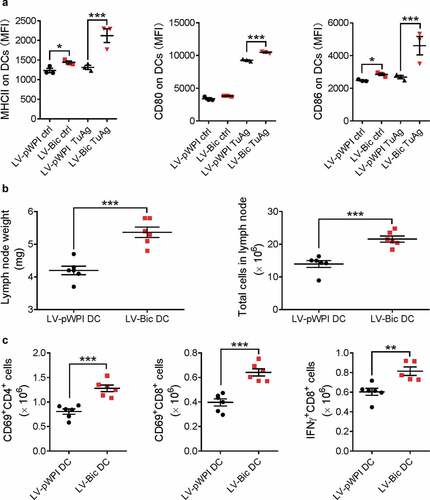
miR-155 overexpression in DCs boosts antigen-specific activation of T cells
To confirm that overexpression of miR-155 can enhance DC antigen presentation and antigen-specific T cell activation, we used ovalbumin (OVA) as a surrogate antigen to perform an ELISpot assay. BMDCs from age-matched female WT C57Bl/6 or miR-155tg mice were treated with ovalbumin or/and EO771 tumor antigen (TuAg, a combination of EO771 cell conditioned medium and cell lysate) for 24 h and were analyzed for DC maturation/activation. It was found that miR-155tg BMDCs were more mature/activated than WT BMDCs under either OVA or TuAg alone or in combination (). Indeed, when the treated DCs were co-cultured with OT-1 T cells, miR-155tg DCs presented OVA-derived antigens more effectively to OT-1 T cells and increased the frequency of activated CD8+ T cells (CD69+, or CD44+/CD62L−) (); Fig. S9), indicating miR-155tg DCs can process and present both OVA-derived antigens and tumor antigens more effectively to T cells than WT DCs. It is understandable that OVA-treated DCs activate OT-1 T cells much more effectively than tumor antigens-treated DCs. Furthermore, ELISpot assay showed that OVA treated or TuAg + OVA treated miR-155tg DCs significantly enhanced IFNγ+ T cells compared to OVA treated or TuAg + OVA treated WT DCs, respectively (). In addition, TuAg + OVA treated DCs are more effective in activating OT-1 T cells than OVA-treated DCs, suggesting that tumor cell conditioned medium and lysate indeed enhanced DC function.
Figure 6. miR-155-overexpression in DCs boosts antigen-specific activation of T cells. (a) Day 8 WT and miR155tg BMDCs were pulsed with OVA whole protein (500 µg/ml) with or without tumor antigen (TuAg, Conditioned medium + cell lysate) for 24 h, the maturation status of DC was determined by MHCII, CD40, CD80, and CD86 expression using flow cytometry. (b) Above mentioned BMDCs treated by OVA and/or TuAg were co-cultured with T cells isolated from the spleen of OT-I mouse for 24 h. T cells were harvested and antigen specific T cell activation was analyzed using flow cytometry by staining of CD69, CD44 and CD62L. (c) 1 × 104 antigen experienced T cells were transferred into IFNγ mAb pre-coated ELISpot plate for another 24 h, ELISpot assay was performed and IFNγ spots were counted under a dissection microscope. Results are shown as means ± SEM. (n = 3). *p < .05, **p < .01, ***p < .005
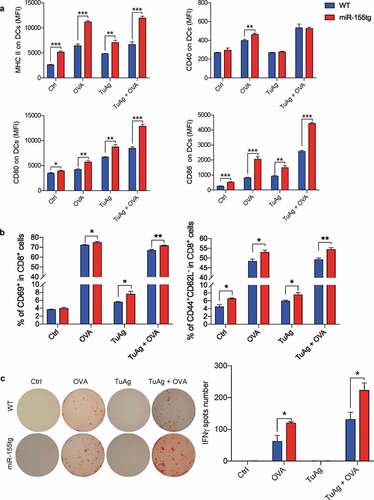
miR-155 overexpression increases the efficacy of a DC-based vaccine for breast cancer
To exploit miR-155 overexpression as a strategy to improve the efficacy of DC-based vaccines against breast tumors, we injected tumor-antigen pulsed BMDCs from miR-155tg or WT mice into mice bearing EO771 breast tumors twice a week for 3 weeks as an anti-tumor DC vaccine (). A third control group received only PBS injections of the same volume as the DC-containing injections. Vaccine therapy with miR-155tg BMDCs significantly inhibited tumor growth (). To provide more insights into the enhanced antitumor response, we examined effector T cells in mice receiving vaccine therapies. The percentage of IFNγ-expressing CD8+ T cells in the spleens and the tumors as well as the percentages of CD69+ cells in CD8+ or CD4+ T cells in the tumors of miR-155tg BMDC-immunized mice were significantly increased when compared to WT BMDC-immunized mice (), pointing to an increased systemic immune response.
Figure 7. miR155 overexpression enhances the efficacy of DC vaccine. (a) Timeline of the experiment of EO771 orthotopic breast cancer model. Seven-week-old, female C57Bl/6 mice were inoculated with 2 × 105 EO771 cells in each of the 4th pair of mammary fat pads. Beginning on the following day for 3 weeks, mice received twice-weekly s.c. injections of tumor-pulsed WT or miR155tg DCs; and mice were sacrificed 38 days after tumor implantation. (b) Tumor growth and tumor weight at sacrifice. (n = 8–17). (c) T cell activation in spleens or tumors was determined by staining for CD3, CD4, CD8, CD69 and IFNγ on single cell suspensions and performing flow cytometry. (n = 8–13). (d) Timeline of the experiment of 4T1 orthotopic breast cancer model. Balb/c mice were inoculated with 2 × 105 4T1 cells and treated with DC vaccine with or without forced miR-155 expression by lentiviral transduction at indicated time points. (e) Tumor growth was monitored till Day 40 post-tumor inoculation. (n = 23–30). (f) Tumor pulmonary metastatic nodules were counted at the experimental end-point. (n = 13–15). (g) At the end-point, tumor draining lymph nodes from the surviving mice were analyzed for activated T cells; the absolute numbers of activated T cells are shown. (n = 13–15). Results are shown as means ± SEM. *p < .05, **p < .01, ***p < .005
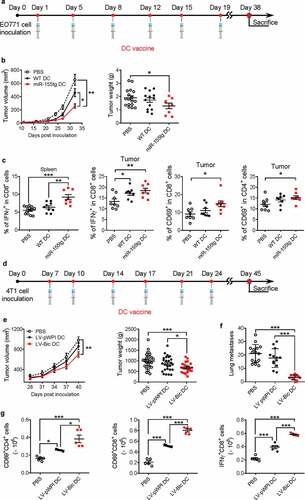
In another set of experiments, 4T1 breast cancer cells and syngeneic Balb/c mice were used. Lentiviral transduction of Balb/c BMDCs with either LV-pWPI or LV-Bic resulted in 41% and 35% transduction efficiency, respectively (Fig. S10A). Expression levels of MHCII and CD86 were significantly increased in BMDCs upon treatment with 4T1 cell conditioned medium and lysates (Fig. S10B). To further test this result in vivo, eight-week-old female Balb/c mice were inoculated with 4T1 cells. To test the efficacy of the DC vaccine in a more therapeutic setting, we started the vaccine treatment when the tumors were established. On days 7, 10, 14, 17, 21 and 24 post-inoculation, tumor-pulsed BMDCs transduced with either LV-pWPI or LV-bic were injected into the mice as DC vaccines with a third group receiving PBS injections as a control (). Compared to PBS, the miR155 lentivirus-transduced DC vaccine significantly delayed tumor growth () and more drastically reduced tumor pulmonary metastasis (), while the control lentivirus-transduced DC vaccine did not have a significant impact. Moreover, as assessed by flow cytometry, there were significantly more activated CD4+ and CD8+ T cells in the tumor draining lymph nodes of miR155-overexpressing DC-vaccinated mice than in those of PBS or control DC-vaccinated mice ().
Discussion
Immunization with DCs loaded with tumor antigens can prime a tumor specific cytotoxic T lymphocyte (CTL) response and induce antitumor immunity in experimental animals.Citation21-Citation23 DC immunotherapy has already been introduced clinically and has been proven feasible, safe, and effective in some patients.Citation24-Citation26 Efficient induction of active CTLs is critical for successful anti-tumor immunotherapy.Citation27 However, further efforts are required to improve the immune response elicited by DC-based vaccines.
miR-155 is a key regulator of the immune response against solid tumors; it influences a variety of immune cells involved in anti-tumor responses, including T cells, natural killer cells, and myeloid cells, such as DCs and MDSCs.Citation14,Citation28-Citation32 We and others have previously shown that expression of miR-155 in murine DCs is important for both their maturation and function, as demonstrated by the observation that miR-155-deficient mice display impaired immune responses to pathogens and tumors.Citation5,Citation17 This is due in part to reduced expression of CD40 and CD86 following TLR activation or exposure to the TME. The DCs isolated from miR-155-deficient mice also have an impaired ability to activate antigen-specific T cells. Dunand-Sauthier et al. found that miR-155-induced repression of Arg2 expression is critical for DCs to drive T cell activation by controlling arginine availability in the extracellular environment.Citation33 Our previous results revealed that miR-155 can upregulate CCR7 expression to promote DC migration.Citation16
To examine if increased miR-155 expression can enhance DC functions and improve the efficacy of DC-based vaccines for breast cancer, we developed a miR-155 transgenic mouse model. Bone marrow transplantation followed by orthotopic tumor implantation allowed for the comparison of the responses of several immune cell types between WT and miR-155tg immune systems. The results largely pointed to an increase in systemic immune activation, especially DC activation, as well as increased CD8+ T cell activity. It is clear that miR-155 overexpression in bone marrow progenitors drives DC differentiation, T cell activation, and cytokine production by several cell types when challenged with tumor antigens. In vitro assays showed that the expression of costimulatory factors in DCs was increased upon exposure to tumor materials. CCR7 expression was also increased in DCs in agreement with our previous results obtained using miR-155−/- animals. This increase in CCR7 expression yielded an increase in the migratory response of DCs to CCL19, a key cytokine produced by high endothelial venules and T cell zones in lymph nodes to attract antigen-loaded DCs. DC-induced T cell activation and proliferation were significantly increased by DC overexpression of miR-155. The injection of miR-155tg BMDCs into EO771 tumor-bearing mice resulted in a more pronounced decrease in systemic tumor-induced immune suppression than the introduction of WT BMDCs. To confirm that overexpression of miR-155 can enhance DC antigen presentation and antigen-specific T cell activation, we used ovalbumin (OVA) as a surrogate antigen to perform an ELISpot assay, our results demonstrated that miR-155tg DCs can process and present both OVA-derived antigens and tumor antigens to T cells and antigen specifically activate OT-1 T cells more effectively than WT DCs. Finally, implementation of a common DC vaccination regimen in two orthotopic models of murine breast cancer showed that DC miR-155 overexpression was able to improve the vaccine’s capacity to slow primary tumor growth and maintain anti-tumor immunity long after the last vaccine injection had been administered. Our DC vaccine regimen does not employ any additional maturation stimulants, such as TLR4 ligands or TNF-α, because our objective here was not to implement the maximally matured DC vaccine, but was instead to study the effects of miR-155 overexpression on DC maturation and resulting anti-tumor immunity. In further vaccine development, such additional stimulatory agents could also be added. Taken together, these data show that miR-155 overexpression enhances DC maturation and migration, and that miR-155 overexpressed DC-based vaccines induce a stronger systemic immune response and more effectively suppress tumor growth and metastasis in a murine model of breast cancer.
The murine breast cancer cell lines that we have chosen to use are both considered representative models of TNBC. We have chosen to investigate our immune therapy in the context of TNBC largely for 2 reasons. First, TNBC is the molecular subtype with the least number of treatment options, and the highest mortality rate. Second, it has been shown that TNBC may actually be more immunogenic than other subtypes, rendering it more responsive to immune therapies.Citation34
Although DC-based vaccines hold a great promise as potent immune therapies for solid tumors including TNBC, only one has been approved for clinical use, which is Sipuleucel-T for prostate cancer.Citation35 The reasons for the lower-than-expectation outcome for these DC vaccines are multifold; a major reason is that tumor-derived factors may disable the injected DCs. For example, our previous study showed that cytokines such as IL-10 and IL-6 produced and secreted by tumor cells impair DC activation and functions by suppressing miR-155 expression, while miR-155 plays a vital role in DC functions by directly targeting c-fos, Arg-2, SOCS-1 and Jarid2.Citation16 Our current study suggests that in future DC vaccine development for breast cancer or other solid tumors, introducing forced miR155 overexpression in DCs via various approaches such as viral transduction or nanoparticle delivery, as well as including other adjuvant agents such as TLR ligands or immune stimulating cytokines, may unleash the full therapeutic potential of the DC vaccines.
Materials and methods
Mice
All animal experiments were approved by the Institutional Animal Care and Use Committee (IACUC) at the University of South Carolina. C57BL/6 and Balb/c mice, and OT-1 (C57BL/6-Tg(TcraTcrb)1100Mjb/J) mice were obtained from Jackson Laboratories (Bar Harbor, Maine). A DNA fragment consisting of a CMV promoter, the mouse Bic sequence, and an SV40 late polyadenylation signal sequence was used for generation of miR-155 transgenic mice on C57Bl/6 background by microinjection. Mice were maintained in pathogen-free conditions at the University of South Carolina according to National Institutes of Health guidelines.
Bone marrow transplantation
BMT was conducted as described previously.Citation36 Briefly, 20 recipient female WT mice were lethally irradiated (900 rad). Bone marrow cells were harvested from WT and miR-155tg donor mice by flushing the femurs and tibias with PBS supplemented with 2% FBS. The flushed bone marrow cells were resuspended in PBS, and 1 × 106 nucleated WT or miR-155tg cells were injected retro-orbitally into 10 irradiated WT mice within 6 h after irradiation, respectively.
Cell culture, tumor-conditioned medium, and tumor cell lysate
EO771 tumor cells were expanded in high-glucose Dulbecco’s modified eagle medium (DMEM, Gibco) supplemented with 10% fetal bovine serum (FBS, Gibco), 100 U/mL penicillin (Sigma-Aldrich Corp), and 100 µg/mL streptomycin (Sigma-Aldrich Corp) in a humidified, 5% CO2 incubator at 37°C.
EO771 cells were seeded at 5 × 106 cells per 75 cm2 bottle and cultured to 70% confluence. All cells for all experiments performed were counted using a glass slide hemocytometer. The medium was then removed, plates were rinsed once with PBS, and serum-free DMEM was added. Cells were further cultured for 48 h in this medium, the medium was then collected, filtered through 0.45-μm filters, and further concentrated 25-fold using Centrifugal Filters with a 3-kD molecular weight cutoff (Millipore, UFC900324).
Tumor cell lysate was obtained by culturing EO771 tumor cells for 48 h in serum-free DMEM, rinsing once with PBS, and then disrupting the cells with four freeze-thaw cycles in liquid nitrogen and a 37°C water bath. The solution was then centrifuged at 10,000 × g for 10 min to remove insoluble cell fragments. The supernatant was referred to as cell lysate and used as a source of soluble tumor antigens.
Orthotopic breast cancer model
A mouse orthotopic breast cancer model was established as previously described.Citation37 Briefly, 2 × 105 EO771 or 4T1 cells suspended in 20 μL PBS were implanted into each side of the 4th pair of mammary fat pads of mice. The tumor size was monitored by caliper measurement on indicated days. Tumor volume was calculated according to the following formula: (short axis)2 × (long axis)/2 ≈ volume. The volumes or weights of left and right tumors from each mouse were averaged to present as one biological replicate. At the experimental end point, mice were sacrificed, and lungs, tumors, tumor-draining lymph nodes, and spleens were removed, weighed, and processed for FACS or other analyses.
Cell isolation from tissues
Cells from lymph nodes and spleens were isolated by mechanical disruption. Tumor tissue was weighed then chopped into < 1 mm fragments and enzymatically digested in the following mixture: 5 mL of RPMI 1640 medium supplemented with 10% FBS, 20 mg Collagenase type IV, 10 µg Hyaluronidase, and 100 µg DNase I. Digestion took place for 1 h at 37°C. Cells were thoroughly rinsed with ice-cold PBS, then erythrocytes were lysed using red blood cell lysing buffer (Sigma, St. Louis, MO) per manufacturer’s instructions. Cell suspensions were passed through 70-μm cell strainers then suspended in PBS.
For DC and T cell purification, 1 × 108 cells isolated as described above were sequentially incubated with 20 μL PE-conjugated CD11c or CD3 antibody, 100 μL PE selection cocktail, and 50 μL magnetic nanoparticles (EasySep™ Mouse PE Positive Selection Kit), and then were separated using magnet following manufacturer’s instructions. All cells for all experiments performed were counted using a glass slide hemocytometer. In all samples, a purity of > 95% was achieved as monitored by flow cytometry.
Generation of BMDCs and tumor antigen pulsing
Bone marrow cells were rinsed from mouse femurs and tibiae in a laminar flow hood. Erythrocytes were depleted using red blood cell lysing buffer (Sigma-Aldrich Corp.) per manufacturer’s instructions. Bone fragments were filtered from cells using 70-µm cell strainers. Resulting cells were then cultured at a density of 1 × 106 cells/mL (10 mL in 10-cm plates) in DC medium (RPMI 1640 medium supplemented with 10% FBS, penicillin/streptomycin, 50 μM β-mercaptoethanol, 10–20 ng/mL recombinant GM-CSF (rGM-CSF) and 10–20 ng/mL rIL-4 (BioAbChem Inc. Ladson, SC). 10 mL of fresh DC medium was added on Day 3. After 8 days of culture, loosely adherent cells were harvested by gentle pipetting. Each preparation was confirmed to be > 90% positive for CD11c. Resulting cells were suspended in control medium (RPMI 1640 medium with 10% FBS and penicillin/streptomycin) or treatment medium (Control medium with 20% (v/v) concentrated EO771 conditioned medium (ECM) and 100 μg/mL of tumor lysate) at 0.5 × 106 cells/mL and cultured for 48 h. After pulsation, DCs were collected in PBS with 3 mM EDTA for 10 min at RT.
In vitro T cell activation and proliferation assays
Day 8 BMDCs were seeded at a density of 1 × 105 cells in 0.5 mL of either control or treatment medium in 24-well plates for 48 h. Supernatant was then removed, and RPMI 1640 complete medium containing 1 × 106 MACS-purified CD3+ T cells was added into each well. T cell proliferation was determined by staining tumor antigen-inexperienced splenic T cells with carboxyfluorescein succinimidyl ester (CFSE), then co-culturing these cells with matured DCs for 5 days in complete RPMI-1640 medium supplemented with 20 U/mL recombinant mouse IL-2 (Biolegend). CFSE dilution through division was determined by flow cytometry. CFSE-stained samples of T cells alone and unstained T cells were used as representative negative and positive proliferation controls, respectively. The “Proliferation” tool in FlowJo was used to analyze the samples and assign the Proliferation Index.
To assess DC-induced T cell activation, DCs were gently rinsed twice with PBS to remove residual treatment medium. 1–2 × 106 tumor antigen-inexperienced splenic T cells were then added in 1 mL complete RPMI-1640 medium. The co-culture was maintained in a 37°C, 5% CO2, humidified incubator for 48 hours. T cells were then collected by rinsing the wells and stained to determine cell surface expression of CD69 on all T cells and intracellular expression of IFNγ on CD8+ T cells for flow cytometry.
In vivo T cell activation
Twenty-four hours after tumor implantation, EO771 tumor-conditioned medium and lysate-matured WT or miR-155tg BMDCs were collected and 1 × 106 cells were injected subcutaneously into the groins of tumor-bearing mice (5 × 105 DCs each injected into the left and right groin area). A second DC injection was administered in the same manner four days later. Mice were sacrificed seven days after tumor cell implantation. Spleens, lymph nodes and tumors were collected and processed for flow cytometric analysis of T cell activation and other immunologically relevant parameters.
Flow cytometry
Flow cytometry was performed as previously described.Citation16 Briefly, single cell suspensions from various tissues were depleted of erythrocytes. Surface staining was performed by first blocking Fc receptors using anti-CD16/32 (Biolegend), then incubating with fluorescein-conjugated antibodies (Table S1) in staining buffer (PBS 2% FBS) for 30 min at 4°C in the dark. Internal staining was performed for IFNγ using eBioscience Cell Stimulation Cocktail (Thermo Fisher Scientific) followed by BD fixation and permeabilization treatment following manufacturer protocols. All samples were rinsed with PBS twice before being analyzed using a BD FACS Aria II flow cytometer and CXP software version 2.2 (BD Biosciences). Data were collected for 1 × 104 to 1 × 106 cells per sample.
Quantitative real-time PCR (qPCR) for mRNA and miR-155 expression
Tissue/cells were lysed in 700 μL Qiazol lysis reagent (Qiagen), and tissue samples were homogenized. RNA was extracted using Qiagen miRNeasy Mini Kits to allow for the collection of microRNAs. cDNA was then synthesized using 1 μg RNA using Qiagen miScript II RT kits. qPCR was performed in a Bio-Rad CFX96 thermocycler using iQ™ SYBR® Green Supermix (Bio-Rad). All primers used for qPCR analysis of genes were synthesized by Integrated DNA Technologies (Coralville, IA). The primer sequences are listed in Supplemental Table S2. The relative amount of target mRNA was determined using the comparative threshold (Ct) method by normalizing target mRNA Ct values to those of 18S RNA. qPCR run conditions were 95°C for 15 min followed by a repeated cycling of 94°C for 15 s, 55°C for 30 sec, and 70°C for 30 s. Samples were run in duplicate. miR-155 expression was measured using Qiagen miScript SYBR Green PCR kits according to the manufacturer’s instructions. Qiagen miScript Primers were purchased for miR-155 and U6, for normalization.
In vitro DC migration
CCL19 (100 ng/mL, Biolegend) in serum-free RPMI-1640 medium was added to 24 well plates and allowed to equilibrate overnight in a 37°C humidified, 5% CO2 incubator. 0.2 × 106 control and tumor antigen-pulsed DCs were seeded into 8-μm pore size polystyrene trans-well inserts (Corning) in serum-free RPMI-1640 and allowed to migrate for 3 h at 37°C. Trans-well inserts were then removed from the culture plates and fixed in 4% paraformaldehyde for 20 min on ice. Cells on the upper surface of the inserts were wiped away with cotton swabs. Cells that had migrated to the underside of the insert were stained with DAPI and counted. Each sample was run in triplicate, and 10 fields were collected at 10× magnification for each membrane using an EVOS FL Auto 2 System. DAPI-stained cells were counted in each field using Image-Pro Plus analysis software (Media Cybernetics, Rockville, MD).
Antigen specific T cell activation and IFNγ ELISpot assay
Day 8 BMDCs were seeded at a density of 2 × 104 cells in 96-well plate, with or without treatment using 500 μg/ml of OVA (Ovalbumin, Sigma, A5503) and tumor antigen (ECM + lysate). After 24 h, treatment media were removed and cells were washed with PBS for 3 times. 1 × 105 MACS-purified OT-I T cells from OT-1 mice in 200 μl of RPMI 1640 complete medium were added and co-cultured with DCs (DC:T cell = 1:5) for 24 h, then 1 × 104 T cells were transferred into IFNγ mAb pre-coated ELISpot plate for another 24 h. IFNγ producing T cells were detected using ELISpot kit according to the manufacturer’s instructions (BD Biosciences, 551083). Some OVA and tumor antigen treated DCs and DC-cocultured T cells were analyzed by flow cytometry.
Lentiviral vector construction and lentiviral transduction
Briefly, a 419-bp DNA fragment containing mouse pre-miR-155 stem-loop (bic gene) was amplified by PCR from mouse genomic cDNA. This fragment was then sub-cloned into the bi-cistronic lentiviral vector pWPI; the resulting vector mediates expression of both miR-155 and GFP. Verification of the miR-155 overexpression construct was performed via DNA sequencing. The mouse miR-155 overexpression plasmid, the packaging plasmid pCMVΔR8.91, and the envelope plasmid pMD2.G were co-transfected into HEK293T cells using ProFection® mammalian transfection system (Promega) per the manufacturer’s instructions for viral production. The RetroNectin-bound Virus Infection method was used to transfect BMDCs. This involved the preloading of lentiviral solutions (MOI = 30) onto RetroNectin-coated (60 µg/mL) plates. 5 × 105 cells in 1 mL were seeded onto lentivirus pre-coated 24-well plates, which were then centrifuged at 2,000 × g for 1 h at 25°C and incubated at 37oC for 72 h.
DC vaccination
EO771 or 4T1 breast tumor cells (2 × 105 in 20 µL PBS) were implanted into the 4th pair of mammary fat pads of 8 week-old female C57BL/6 (total 41) or Balb/c (total 34) mice, respectively. Corresponding tumor-conditioned medium and lysate-matured bone marrow-derived DCs were removed from culture dishes by incubating in PBS with 3 mM EDTA for 10 min at RT. These DCs were then resuspended in PBS and 1 × 106 cells were injected subcutaneously into the groins of tumor-bearing mice (5 × 105 DCs each injected into the left and right groin area). This DC harvesting and injection procedure was repeated every three to four days for a total of six DC injections. Tumor volume was monitored until sacrifice. At the endpoint of experiment, mice were sacrificed; tumors, tumor-draining lymph nodes, spleens, and lungs were collected for various analyses.
Statistics
Data were shown as mean ± standard error of mean (SEM) whenever the mean was the primary value representative of a sample group’s behavior. Multiple group comparison of means is accomplished using One-way ANOVA followed by Tukey multiple comparison tests, while two group comparison was accomplished using a two-tailed Student’s t test. All such comparisons are performed using GraphPad Prism software (Graphpad Software Inc.). Differences between proportions were determined using a two tailed N-1 Chi-Square test. p ≤ 0.05 was considered statistically significant for all tests.
Abbreviations
CSFE | = | Carboxyfluorescein diacetate succinimidyl ester |
DC | = | Dendritic cell |
miR-155 | = | microRNA-155 |
miR-155−/- | = | bic/miR-155 knockout |
miR-155tg | = | microRNA-155 transgenic |
qPCR | = | Quantitative real-time RT-PCR |
TCM | = | Tumor cell conditioned media |
TME | = | Tumor microenvironment |
Author contribution
DF and JW designed the study; JH, FW, QL, FS, YW and US performed the experiments; FW, JW, JH and DF wrote the manuscript draft; WA, HC, and ML helped in interpretation of the data and manuscript writing.
Disclosure of potential conflicts of interest
No potential conflicts of interest were disclosed.
Supplemental Material
Download ()Supplementary material
Supplemental data for this article can be accessed on the publisher’s website.
Additional information
Funding
References
- Lim DS, Kim JH, Lee DS, Yoon CH, Bae YS. DC immunotherapy is highly effective for the inhibition of tumor metastasis or recurrence, although it is not efficient for the eradication of established solid tumors. Cancer Immunol Immunother. 2007;56(11):1817–14. doi:10.1007/s00262-007-0325-0.
- Lee WC, Wang, HC, Hung, CF, Huang, PF, Lia, CR, Chen, MF. Vaccination of advanced hepatocellular carcinoma patients with tumor lysate-pulsed dendritic cells: a clinical trial. J Immunother. 2005;28(5):496–504. doi:10.1097/01.cji.0000171291.72039.e2.
- Yu JS, Liu G, Ying H, Yong WH, Black KL, Wheeler CJ. Vaccination with tumor lysate-pulsed dendritic cells elicits antigen-specific, cytotoxic T-cells in patients with malignant glioma. Cancer Res. 2004;64(14):4973–4979. doi:10.1158/0008-5472.CAN-03-3505.
- Gardner A, Ruffell B. Dendritic cells and cancer immunity. Trends Immunol. 2016;37(12):855–865. doi:10.1016/j.it.2016.09.006.
- Rodriguez A, Vigorito E, Clare S, Warren MV, Couttet P, Soond DR, van Dongen S, Grocock RJ, Das PP, Miska EA, et al. Requirement of bic/microRNA-155 for normal immune function. Science. 2007;316(5824):608–611. doi:10.1126/science.1139253.
- Thai T-H, Calado DP, Casola S, Ansel KM, Xiao C, Xue Y, Murphy A, Frendewey D, Valenzuela D, Kutok JL, et al. Regulation of the germinal center response by microRNA-155. Science. 2007;316(5824):604–608. doi:10.1126/science.1141229.
- Vigorito E, Perks KL, Abreu-Goodger C, Bunting S, Xiang Z, Kohlhaas S, Das PP, Miska EA, Rodriguez A, Bradley A, et al. microRNA-155 regulates the generation of immunoglobulin class-switched plasma cells. Immunity. 2007;27(6):847–859. doi:10.1016/j.immuni.2007.10.009.
- O’Connell RM, Kahn D, Gibson WSJ, Round JL, Scholz RL, Chaudhuri AA, Kahn ME, Rao DS, Baltimore D. MicroRNA-155 promotes autoimmune inflammation by enhancing inflammatory T cell development. Immunity. 2010;33(4):607–619. doi:10.1016/j.immuni.2010.09.009.
- Singh UP, Murphy AE, Enos RT, Shamran HA, Singh NP, Guan H, Hegde VL, Fan D, Price RL, Taub DD, et al. miR-155 deficiency protects mice from experimental colitis by reducing T helper type 1/type 17 responses. Immunology. 2014;143(3):478–489. doi:10.1111/imm.2014.143.issue-3.
- Escobar T, Yu C-R, Muljo SA, Egwuagu CE. STAT3 activates miR-155 in Th17 cells and acts in concert to promote experimental autoimmune uveitis. Invest Ophthalmol Vis Sci. 2013;54(6):4017–4025. doi:10.1167/iovs.13-11937.
- Yao R, Ma Y-L, Liang W, Li -H-H, Ma Z-J, Yu X, Liao Y-H. MicroRNA-155 modulates Treg and Th17 cells differentiation and Th17 cell function by targeting SOCS1. PLoS One. 2012;7(10):e46082. doi:10.1371/journal.pone.0046082.
- Tsai C-Y, Allie SR, Zhang W, Usherwood EJ. MicroRNA miR-155 affects antiviral effector and effector memory CD8 T cell differentiation. J Virol. 2013;87(4):2348–2351. doi:10.1128/JVI.01742-12.
- Lind EF, Elford AR, Ohashi PS. Micro-RNA 155 is required for optimal CD8+ T cell responses to acute viral and intracellular bacterial challenges. J Immunol. 2013;190(3):1210–1216. doi:10.4049/jimmunol.1202700.
- Dudda JC, Salaun B, Ji Y, Palmer D, Monnot G, Merck E, Boudousquie C, Utzschneider D, Escobar T, Perret R, et al. MicroRNA-155 is required for effector CD8+ T cell responses to virus infection and cancer. Immunity. 2013;38(4):742–753. doi:10.1016/j.immuni.2012.12.006.
- Gracias DT, Stelekati E, Hope JL, Boesteanu AC, Doering TA, Norton J, Mueller YM, Fraietta JA, Wherry EJ, Turner M, et al. The microRNA miR-155 controls CD8+ T cell responses by regulating interferon signaling. Nat Immunol. 2013;14(6):593–602. doi:10.1038/ni.2576.
- Wang J, Iwanowycz S, Yu F, Jia X, Leng S, Wang Y, Li W, Huang S, Ai W, Fan D, et al. microRNA-155 deficiency impairs dendritic cell function in breast cancer. Oncoimmunology. 2016;5(11):e1232223. doi:10.1080/2162402X.2016.1232223.
- Dunand-Sauthier I, Santiago-Raber M-L, Capponi L, Vejnar CE, Schaad O, Irla M, Seguín-Estévez Q, Descombes P, Zdobnov EM, Acha-Orbea H, et al. Silencing of c-fos expression by microRNA-155 is critical for dendritic cell maturation and function. Blood. 2011;117(17):4490–4500. doi:10.1182/blood-2010-09-308064.
- Lu C, Huang X, Zhang X, Roensch K, Cao Q, Nakayama KI, Blazar BR, Zeng Y, Zhou X. miR-221 and miR-155 regulate human dendritic cell development, apoptosis, and IL-12 production through targeting of p27kip1, KPC1, and SOCS-1. Blood. 2011;117(16):4293–4303. doi:10.1182/blood-2010-12-322503.
- Forster R, Davalos-Misslitz AC, Rot A. CCR7 and its ligands: balancing immunity and tolerance. Nat Rev Immunol. 2008;8(5):362–371. doi:10.1038/nri2297.
- Du F, Yu F, Wang Y, Hui Y, Carnevale K, Fu M, Lu H, Fan D. MicroRNA-155 deficiency results in decreased macrophage inflammation and attenuated atherogenesis in apolipoprotein E-deficient mice. Arterioscler Thromb Vasc Biol. 2014;34(4):759–767. doi:10.1161/ATVBAHA.113.302701.
- Fields RC, Shimizu K, Mule JJ. Murine dendritic cells pulsed with whole tumor lysates mediate potent antitumor immune responses in vitro and in vivo. Proc Natl Acad Sci U S A. 1998;95(16):9482–9487. doi:10.1073/pnas.95.16.9482.
- Mayordomo JI, Zorina T, Storkus WJ, Zitvogel L, Celluzzi C, Falo LD, Melief CJ, Ildstad ST, Martin Kast W, Deleo AB, et al. Bone marrow-derived dendritic cells pulsed with synthetic tumour peptides elicit protective and therapeutic antitumour immunity. Nat Med. 1995;1(12):1297–1302. doi:10.1038/nm1295-1297.
- Paglia P, Chiodoni C, Rodolfo M, Colombo MP. Murine dendritic cells loaded in vitro with soluble protein prime cytotoxic T lymphocytes against tumor antigen in vivo. J Exp Med. 1996;183(1):317–322. doi:10.1084/jem.183.1.317.
- Banchereau J, Palucka AK, Dhodapkar M, Burkeholder S, Taquet N, Rolland A, Taquet S, Coquery S, Wittkowski KM, Bhardwaj N, et al. Immune and clinical responses in patients with metastatic melanoma to CD34(+) progenitor-derived dendritic cell vaccine. Cancer Res. 2001;61(17):6451–6458.
- Schuler-Thurner B, Schultz ES, Berger TG, Weinlich G, Ebner S, Woerl P, Bender A, Feuerstein B, Fritsch PO, Romani N, et al. Rapid induction of tumor-specific type 1 T helper cells in metastatic melanoma patients by vaccination with mature, cryopreserved, peptide-loaded monocyte-derived dendritic cells. J Exp Med. 2002;195(10):1279–1288. doi:10.1084/jem.20012100.
- de Vries IJ, Lesterhuis WJ, Scharenborg NM, Engelen LPH, Ruiter DJ, Gerritsen MJP, Croockewit S, Britten CM, Torensma R, Adema GJ, et al. Maturation of dendritic cells is a prerequisite for inducing immune responses in advanced melanoma patients. Clini Cancer Res. 2003;9(14):5091–5100.
- Coulie PG, Van den Eynde BJ, van der Bruggen P, Boon T. Tumour antigens recognized by T lymphocytes: at the core of cancer immunotherapy. Nat Rev Cancer. 2014;14(2):135–146. doi:10.1038/nrc3670.
- Trotta R, Chen L, Ciarlariello D, Josyula S, Mao C, Costinean S, Yu L, Butchar JP, Tridandapani S, Croce CM, et al. miR-155 regulates IFN-gamma production in natural killer cells. Blood. 2012;119(15):3478–3485. doi:10.1182/blood-2011-12-398099.
- Zonari E, Pucci F, Saini M, Mazzieri R, Politi LS, Gentner B, Naldini L. A role for miR-155 in enabling tumor-infiltrating innate immune cells to mount effective antitumor responses in mice. Blood. 2013;122(2):243–252. doi:10.1182/blood-2012-08-449306.
- Li L, Zhang J, Diao W, Wang D, Wei Y, Zhang C-Y, Zen K. MicroRNA-155 and MicroRNA-21 promote the expansion of functional myeloid-derived suppressor cells. J Immunol. 2014;192(3):1034–1043. doi:10.4049/jimmunol.1301309.
- Chen S, Wang L, Fan J, Ye C, Dominguez D, Zhang Y, Curiel TJ, Fang D, Kuzel TM, Zhang B, et al. Host miR155 promotes tumor growth through a myeloid-derived suppressor cell-dependent mechanism. Cancer Res. 2015;75(3):519–531. doi:10.1158/0008-5472.CAN-14-2331.
- Wang J, Yu F, Jia X, Iwanowycz S, Wang Y, Huang S, Ai W, Fan D. MicroRNA-155 deficiency enhances the recruitment and functions of myeloid-derived suppressor cells in tumor microenvironment and promotes solid tumor growth. Int J Cancer. 2015;136(6):E602–13. doi:10.1002/ijc.29151.
- Dunand-Sauthier I, Irla M, Carnesecchi S, Seguín-Estévez Q, Vejnar CE, Zdobnov EM, Santiago-Raber M-L, Reith W. Repression of arginase-2 expression in dendritic cells by microRNA-155 is critical for promoting T cell proliferation. J Immunol. 2014;193(4):1690–1700. doi:10.4049/jimmunol.1301913.
- Liu Z, Li M, Jiang Z, Wang X. A comprehensive immunologic portrait of triple-negative breast cancer. Trans Oncol. 2018;11(2):311–329. doi:10.1016/j.tranon.2018.01.011.
- Vacchelli E, Vitale I, Eggermont A, Fridman WH, Fučíková J, Cremer I, Galon J, Tartour E, Zitvogel L, Kroemer G, et al. Trial watch: dendritic cell-based interventions for cancer therapy. Oncoimmunology. 2013;2(10):e25771. doi:10.4161/onci.25771.
- Yancey PG, Ding Y, Fan D, Blakemore JL, Zhang Y, Ding L, Zhang J, Linton MF, Fazio S. Low-density lipoprotein receptor-related protein 1 prevents early atherosclerosis by limiting lesional apoptosis and inflammatory Ly-6Chigh monocytosis: evidence that the effects are not apolipoprotein E dependent. Circulation. 2011;124(4):454–464. doi:10.1161/CIRCULATIONAHA.111.032268.
- Jia X, Yu F, Wang J, Iwanowycz S, Saaoud F, Wang Y, Hu J, Wang Q, Fan D. Emodin suppresses pulmonary metastasis of breast cancer accompanied with decreased macrophage recruitment and M2 polarization in the lungs. Breast Cancer Res Treat. 2014;148(2):291–302. doi:10.1007/s10549-014-3164-7.