ABSTRACT
The choice of chemo- or immuno-therapy for muscle-invasive bladder cancer (MIBC) patients remains contentious. Podoplanin is newly identified as an immune checkpoint which intrigues us to explore the clinical significance and immunoregulatory role of tumor-infiltrating podoplanin+ cells (PDPN+ cells) in MIBC. A retrospective analysis of 259 MIBC patients from Zhongshan Hospital (n = 141) and Shanghai Cancer Center (n = 118) was conducted. A total of 406 MIBC patients from TCGA database were enrolled to investigate the relationship between PDPN and molecular characterization. We found that tumor-infiltrating PDPN+ cell abundance indicated an inferior overall survival and recurrence-free survival. pT2 MIBC patients with PDPN+ cell low infiltration could benefit more from adjuvant chemotherapy (ACT). Increased PDPN+ cell infiltration was associated with diminished GZMB and TNF-α expression while correlated with expanded PD-1, PD-L1, LAG-3 and TIM-3 expression and tumor-promoting regulatory T cell and M2 macrophage infiltration. Tumors with high PDPN mRNA expression mainly presented luminal-infiltrated and basal-squamous subtypes (2017 TCGA classification) or stroma-rich and Ba/Sq subtypes (consensus classification). Elevated PDPN mRNA expression was associated with less FGFR3 activation signature and more T-cell-inflamed signature and EGFR activation signature. In conclusion, tumor-infiltrating PDPN+ cells could be applied as an independent prognosticator for clinical outcome and a predictive biomarker for suboptimal ACT responsiveness, which was also associated with immunosuppressive contexture infiltration. Intratumoral PDPN expression had a correlation with MIBC molecular classification and therapy-related signatures. The novel immune checkpoint PDPN should be considered as a possible immunotherapeutic target for MIBC.
Introduction
Bladder cancer is a prevalent disease with substantial mortality rate and recurrence risk. Roughly 25% of patients are diagnosed as muscle-invasive or metastatic disease with inferior prognosis.Citation1 Adjuvant cisplatin-based chemotherapy is applied for postoperative muscle-invasive bladder cancer (MIBC) patients to improve survival outcomes.Citation2 However, several meta-analyses illustrated that adjuvant chemotherapy (ACT) could only reduce a quarter risk of death.Citation3 Chemotherapeutic resistance limited the clinical application of ACT. Further classification of MIBC that can predict survival outcomes and chemotherapeutic responsiveness is eagerly needed.
Beyond cytotoxic chemotherapy, an expanded range of effective therapies for MIBC are evolving in recent years.Citation4,Citation5 Targeted therapies are exhibiting promising results in MIBC management. BLC2001 trial showed an impressive tumor response rate with the use of erdafitinib, a tyrosine kinase inhibitor of fibroblast growth factor receptor (FGFR) 1–4.Citation6 Targeting epidermal growth factor receptor (EGFR) showed no significant improvements in outcome, which need further exploration with suitable biomarkers or mechanisms.Citation7 Meanwhile, immune checkpoint (ICK) blockade therapy such as nivolumab (anti-PD-1) and atezolizumab (anti-PD-L1) promotes MIBC treatment significantly as shown in CheckMate 275 trial and IMvigor 210 trial.Citation8,Citation9 Advancing molecular pathology has reclassified MIBC subgroups to potentially support the choice of ACT, targeted therapy or ICK blockade therapy.Citation10,Citation11 Predictive biomarkers for individualized cancer therapies are still being pursued.
Podoplanin (PDPN) is a mucin-type transmembrane glycoprotein, which is a specific marker for lymphatic endothelial cellsCitation12 and also expressed on various immune cells.Citation13 Previous studies uncovered that PDPN+ macrophages promoted lymphangiogenesis and lymphoinvasion in breast cancerCitation14 while PDPN+ myeloid cells suppressed T cell infiltration in glioma.Citation15 Moreover, PDPN serves as a marker for nonpathogenic Th17 subset, negatively regulating Th17-mediated inflammation.Citation16 Recently, PDPN has been identified as a novel ICK expressing on CD8+ T cells and CD4+ T cells, which limits the survival of T cells and orchestrates an exhausted T cell phenotype.Citation17 As a newly-discovered ICK, PDPN is a potential immunotherapeutic target. However, the clinical significance of PDPN+ cells infiltrating in tumors still remains ambiguous. The role of PDPN in regulating the tumor immune microenvironment requires more elucidation.
Herein, our results indicated that tumor-infiltrating PDPN+ cell abundance identified a poor prognosis subset of MIBC patients with suboptimal ACT responsiveness and immunosuppressive contexture infiltration. Intratumoral PDPN expression was associated with MIBC molecular classification and signatures of PD-L1 blockade immunotherapy and FGFR- and EGFR-targeted therapy. Targeting PDPN could be a potential therapeutic approach for MIBC.
Materials and methods
Ethics approval and consent to participate
Written informed consent was obtained from each patient enrolled in this study. The study was conducted and performed after approved by the Clinical Research Ethics Committee of Zhongshan Hospital and the Ethics Committee of Fudan University Shanghai Cancer Center.
Study population
A total of 393 bladder cancer patients were enrolled who underwent radical cystectomy at Zhongshan Hospital of Fudan University from 2002 to 2014 (n = 215, Zhongshan Cohort) and Fudan University Shanghai Cancer Center from 2008 to 2012 (n = 178, FUSCC Cohort). The tissue samples of Zhongshan Cohort and FUSCC Cohort were formalin-fixed and paraffin-embedded. The enrolled cases conformed the following inclusion criteria: (a) informed consent; (b) performed radical cystectomy for bladder cancer; (c) available for paraffin-embedded tumor tissues; (d) confirmed postoperative histopathology diagnosis. A total of 134 patients were excluded based on the following exclusion criteria: (a) incomplete clinical data; (b) incomplete follow-up information; (c) diagnosed as non-urothelial carcinoma; (d) diagnosed as non-muscle invasive bladder cancer; (e) with other malignancies; (f) received neoadjuvant chemotherapy or radiotherapy; (g) tissue detachment during immunohistochemistry (IHC) staining process. The detailed flowchart for patients' filtration of both cohorts is presented in Supplementary Figure 1. Finally, Zhongshan Cohort and FUSCC Cohort were comprised of 141 and 118 MIBC patients for analysis, respectively. Cisplatin-based combination adjuvant chemotherapy was applied for 119 patients of the 2 cohorts which lasted at one therapeutic cycle at least. The follow-up protocol was based on the European Association of Urology (EAU) guidelines for MIBC. The follow-up visits comprised medical history, physical examination and laboratory measurement every 3 months in the first year, every 6 months in the second year and once per year routinely afterward. The Cancer Genome Atlas (TCGA) data set was obtained from http://www.cbioportal.org in Feb. 2018. In total, 406 MIBC patients were feasible for analysis with available clinical data and follow-up information.
Assay methods
IHC staining was performed on formalin-fixed, paraffin-embedded tumor microarrays (TMA) as described previously.Citation18 To ensure an objective comparison of samples for evaluation, we performed IHC staining on an entire set of TMA each time. The IHC antibodies for molecules and immune cells staining are listed in Supplementary Table 1 and Supplementary Table 2. TMA slides of PDPN and PD-L1 IHC staining were scanned with Image-Pro Plus 6.0. TMA slides of other cells and molecules staining were scanned with NanoZoomer-XR (Hamamatsu). A PDPN+ lymphatic vessel was defined as a cluster of endothelial cells or a single endothelial cell positive for PDPN staining.Citation19 Natural killer cells (NK cells) were quantified as the percentage of stained-positive cells in each section. Tumor-infiltrating PDPN+ cell counts, PD-L1+ immune cell counts, other stained-positive cell counts and intratumoral PDPN+ lymphatic vessel counts were calculated as the mean value of 3 randomized high power magnification fields (HPF, ×200 magnification) of each section by Dr. Chen and Dr. Zhang who were blind to follow-up and clinical data. A semi-quantitative approach was applied to assign the IHC score of tumor necrosis factor-alpha (TNF-α) expression. In brief, the staining intensity was graded as 0 (negative staining), 1 (weak staining), 2 (moderate staining) and 3 (strong staining), while the proportion was scored as the percentage of positive cells (0 - 100%). The IHC score ranging from 0 to 300 was generated with the multiplication of staining intensity and proportion. The representative images of molecules and immune cells are illustrated in Supplementary Figure 2 and Supplementary Figure 3, and the representative image of intratumoral PDPN+ lymphatic vessels is illustrated in Supplementary Figure 4A. The cutoff value for classifying tumor-infiltrating PDPN+ cells high/low groups was 18 cells/HPF, which was determined by the median value of tumor-infiltrating PDPN+ cells in Zhongshan Cohort. This cutoff value was then applied in FUSCC Cohort for consistency.
Statistical methods
Overall survival (OS) was calculated from the date of operation until the date of death or last follow-up. Patients alive at last follow-up were censored for OS. Recurrence-free survival (RFS) was calculated from the date of operation until the date of first recurrence or last follow-up. Patients alive and without recurrence were censored for RFS.
ICK score was defined as the mean expression value of PD1, PD-L1, CTLA-4, LAG-3 and TIM-3. Effector score was defined as the mean expression value of granzyme B (GZMB), interferon-γ (IFN-γ) and perforin (PRF1) while TNF-α was excluded because of its semi-quantitative evaluation approach. The cutoff value for PDPN mRNA expression in TCGA was determined by the median value. The gene expression signature scores for luminal signature, basal signature, p53-like signature and cell cycle signature were obtained from the previous study.Citation10 The gene expression scores for FGFR3 activation signature,Citation20 T-cell-inflamed signature,Citation21 EGFR activation signature (gene members of BIOCARTA_EGF_PATHWAY from gene set enrichment analysis)Citation22 and proliferation signatureCitation23 were defined by gene set variation analysis.Citation24 Gene set signature list is presented in Supplementary Table 3.
SPSS 22.0 (SPSS Inc., Chicago, IL, USA) was utilized for statistical analyses. Student t-test and Chi-square test were conducted in patient characteristics analysis. Mann–Whitney U test, Spearman correlation analysis and Kruskal–Wallis H test were applied for statistical p values in the analyses of immune infiltration and molecular characterization. Survival outcomes were analyzed by Kaplan–Meier curves, log-rank test and univariate/multivariate Cox regression analysis. P values less than 0.05 were considered statistically significant. Data were shown as mean ± SD in this study.
Results
Tumor-infiltrating PDPN+ cell abundance indicates inferior survival outcomes
Tumor-infiltrating PDPN+ cells were quantified in MIBC (representative images are presented in ). Patient characteristics of both cohorts are listed in . PDPN+ cell infiltration of both cohorts significantly correlated with clinicopathological features (pT stage, pN stage and AJCC stage). Meanwhile, intratumoral PDPN+ lymphatic vessels were evaluated and the positive rate was 55.2% in 259 MIBC patients (representative images are presented in Supplementary Figure 4a comparing with tumor-infiltrating PDPN+ cells). There is no correlation between tumor-infiltrating PDPN+ cells and intratumoral PDPN+ lymphatic vessels (Supplementary Figure 4B). The clinical significance of tumor-infiltrating PDPN+ cells was next explored. Median follow-up was 74.5 months (Zhongshan Cohort) and 44.5 months (FUSCC Cohort). Kaplan-Meier analysis and log-rank test were applied for comparing OS and RFS. PDPN+ cells high infiltration was associated with significantly inferior OS in both cohorts (p < .001 and p < .001; ). The 5-y OS was estimated at 41.0% and 28.9% in patients with PDPN+ cell high infiltration compared with 80.6% and 76.0% in patients with PDPN+ cell low infiltration in both cohorts, respectively. Patients with PDPN+ cell high infiltration also had worse RFS in both cohorts (p < .001 and p < .001; ). The 5-y RFS was estimated at 32.5% and 36.3% in patients with PDPN+ cell high infiltration compared with 65.9% and 77.6% in patients with PDPN+ cell low infiltration in both cohorts, respectively. We found that PDPN+ cell infiltration was an independent adverse prognostic factor for OS and RFS according to univariate (Supplementary Table 4) and multivariate (Supplementary Table 5) Cox regression analyses.
Table 1. Patient characteristics of Zhongshan cohort and FUSCC cohort
Figure 1. Prognostic significance of tumor-infiltrating PDPN+ cell abundance. Representative images of PDPN immunohistochemistry staining showing tumor-infiltrating PDPN+ cells high (a) and low (b) infiltration in MIBC. Kaplan–Meier curves for OS with PDPN+ cell infiltration strata in Zhongshan Cohort (c) and FUSCC Cohort (d). Kaplan–Meier curves for RFS with PDPN+ cell infiltration strata in Zhongshan Cohort (e) and FUSCC Cohort (f). Log-rank p values were shown
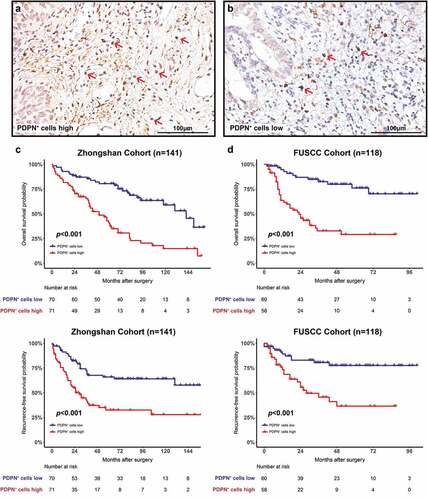
Tumor-infiltrating PDPN+ cell abundance yields poor adjuvant chemotherapeutic response
The association between PDPN+ cell infiltration and therapeutic responsiveness of cisplatin-based ACT was further investigated. We combined the two cohorts for exploration. ACT application did not improve OS of pT2 MIBC patients (p = .111; ) or PDPN+ cells high subgroup (p = .927; ). However, ACT application led to a superior OS in PDPN+ cells low subgroup (p = .022; ). The RFS showed no improvement with ACT application in pT2 MIBC patients (p = .248; ) or PDPN+ cells high subgroup (p = .521; ). Nevertheless, there was an improved RFS in PDPN+ cells low subgroup with ACT application (p = .020; ). Univariate cox regression analysis was performed to assess the relationship between PDPN+ cell infiltration and ACT benefit, which suggested that pT2 MIBC patients with PDPN+ cell low infiltration could receive more benefit from adjuvant chemotherapy (OS: HR, 0.290, 95% CI, 0.095 ~ 0.884, p = .030, RFS: HR, 0.262, 95% CI, 0.077 ~ 0.887, p = .031) while no ACT benefit was observed in patients with pT3 + 4 MIBC or its subgroups according to PDPN+ cell stratification (Supplementary Table 6).
Figure 2. Predictive value of tumor-infiltrating PDPN+ cell abundance for suboptimal adjuvant cisplatin-based chemotherapy responsiveness. Kaplan–Meier curves for OS comparing ACT applied versus not applied in all pT2 patients (a), PDPN+ cells high subgroup (b) and PDPN+ cells low subgroup (c). Kaplan–Meier curves for RFS comparing ACT applied versus not applied in all pT2 patients (d), PDPN+ cells high subgroup (e) and PDPN+ cells low subgroup (f). Log-rank p values were shown
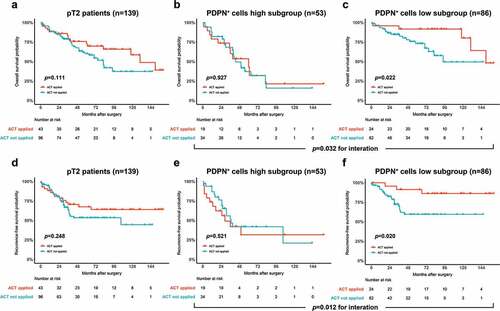
Tumor-infiltrating PDPN+ cell abundance correlates with immunoevasive contexture
We investigated whether tumor-infiltrating PDPN+ cells were associated with the tumor immune microenvironment which closely related to prognosis, adjuvant treatment responsiveness and other parameters.Citation25 Through evaluating PDPN+ cell infiltration with the effector score and effector molecules (IFN-γ, GZMB, PRF1 and TNF-α; ), we found that the effector score decreased significantly in PDPN+ cell high infiltration group (p = .036; ) indicating an impaired anti-tumor function. PDPN+ cell infiltration adversely correlated with GZMB and TNF-α expression (Spearman’s R: −0.190 and −0.236, p = .024 and p = .005; ). The association between PDPN+ cell infiltration and other ICK molecules (PD-1, PD-L1, CTLA-4, TIM-3 and LAG-3) was next evaluated (). The ICK score was higher in PDPN+ cell high infiltration group than PDPN+ cell low infiltration group (p = .005; ). PDPN+ cell infiltration strongly correlated with PD-1 and PD-L1 expression (Spearman’s R: 0.288 and 0.351, p = .001 and p < .001; ) and was also associated with LAG-3 and TIM-3 expression (Spearman’s R: 0.241 and 0.255, p = .004 and p = .002, Supplementary Figure 5B). Previously, we identified an immune cell infiltration classification (immunotype A/B) based on the infiltration level of CD8+ T cells, NK cells, regulatory T cells (Tregs), macrophages and mast cells, which could be applied for predicting survival outcome and ACT benefit.Citation26 We sought to find the association between PDPN+ cell infiltration and immune contexture (). PDPN+ cells infiltrated more in immunotype B tumors compared with immunotype A tumors (p = .005; ). Moreover, increased PDPN+ cell infiltration was significantly associated with more Tregs and M2 macrophage infiltration (Spearman’s R: 0.227 and 0.192, p = .007 and p = .023; ). The comprehensive correlation analyses between PDPN+ cells and molecules or immune cells were demonstrated in Supplementary Figure 5A-D.
Figure 3. Characterization of the immunoevasive microenvironment with tumor-infiltrating PDPN+ cell abundance. (a) Heatmap showing PDPN+ cell infiltration and effector molecules (IFN-γ, GZMB, PRF1 and TNF-α) expression in Zhongshan Cohort (n = 141). (b) Evaluation of effector score in PDPN+ cells high/low infiltration groups (n = 141). (c) Correlation between PDPN+ cell infiltration and GZMB or TNF-α expression (n = 141). (d) Heatmap showing PDPN+ cell infiltration and immune checkpoint (ICK) molecules (PD-1, PD-L1, CTLA-4, TIM-3 and LAG-3) expression in Zhongshan Cohort (n = 141). (e) Evaluation of ICK score in PDPN+ cells high/low infiltration groups (n = 141). (f) Correlation between PDPN+ cell infiltration and PD-1 or PD-L1 expression (n = 141). (g) Heatmap showing PDPN+ cell infiltration, immunotype A/B distribution, immune cells involved in immunotype A/B definition (CD8+ T cells, NK cells, Tregs, macrophages and mast cells) and other anti-tumor or pro-tumor immune cells (Th1 cells, B cells, M1 macrophages, Th2 cells, M2 macrophages and neutrophils) infiltration (n = 141). (h) Distribution of PDPN+ cell infiltration in immunotype A/B groups (n = 141). (i) Correlation between PDPN+ cell infiltration and Tregs or M2 macrophage infiltration (n = 141). Data were analyzed by Mann–Whitney U test and Spearman’s rank correlation test
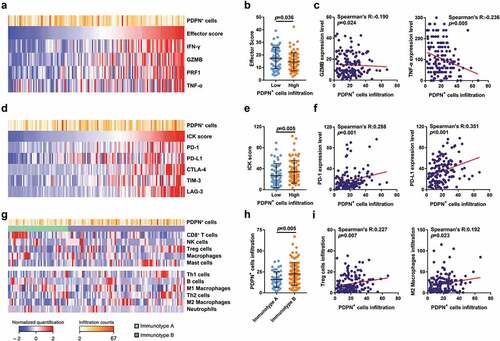
Molecular correlates in intratumoral PDPN abundant TCGA cohort patients
We found that PDPN mRNA expression in TCGA showed difference across molecular subtypes. PDPN mRNA expression accumulated in luminal-infiltrated and basal-squamous subtypes compared with luminal-papillary subtype by 2017 TCGA classification (p < .001; ).Citation10 Meanwhile, there was a higher PDPN mRNA expression in stroma-rich and Ba/Sq subtypes than LumP and LumU subtypes by the consensus classification (p < .001; ).Citation11 Next, we applied the molecular subtype signature to further investigate the association between PDPN mRNA expression and molecular characteristics. Tumors with high PDPN mRNA expression showed a low level of luminal signature (p < .001) and a high level of basal signature and p53-like signature (p < .001 and p < .001; ). Previous studies illustrated that molecular classification may stratify patients for personalized anti-tumor therapies.Citation4 FGFR3 activation signature decreased (p < .001) while T-cell-inflamed signature and EGFR activation signature elevated in tumors with high PDPN mRNA expression (p < .001 and p < .001; ). However, PDPN mRNA expression showed no correlation with cell cycle signature and proliferation signature (p = .368 and p = .292; ).
Figure 4. Characterization of PDPN mRNA expression across molecular subtypes. Comparison of the PDPN mRNA expression across 2017 TCGA classification (a) and consensus classification (b) in TCGA cohort (n = 406). (c) Comparison of the expression of molecular subtype signatures (luminal signature, basal signature and p53-like signature) between high and low PDPN mRNA expression groups. (d) Comparison of the expression of therapy-associated signatures (FGFR3 activation signature, T-cell-inflamed signature and EGFR activation signature) between high and low PDPN mRNA expression groups. (e) Comparison of the expression of cell cycle signature and proliferation signature between high and low PDPN mRNA expression groups. Data were analyzed by Kruskal–Wallis H test (a, b) and Mann–Whitney U test (c–e)
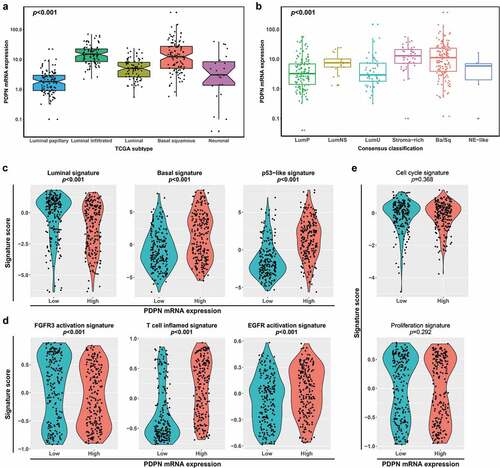
Discussion
The low response rate of ACT for MIBC patients remains an intractable clinical issue.Citation3 Stratification of patients who are resistant or sensitive to first-line chemotherapy has a direct clinical significance for proper therapies. It was previously unveiled that bladder tumors with high MSH2 proteinCitation27 or somatic ERCC2 mutationCitation28,Citation29 received superior response from platinum-based chemotherapy. There is an expanding focus on the involvement of immune system in the cytotoxic chemotherapy process.Citation30 Our previous studies concentrated on the contribution of immune contexture to the beneficial of ACT in MIBC including tumor-infiltrating mast cells,Citation31 neutrophils,Citation32 B cellsCitation33 and an immunotype A/B classification.Citation26 As a brand-new ICK,Citation17 PDPN was first identified as a prognosticator and a predictive biomarker for adjuvant cisplatin-based chemotherapy responsiveness in MIBC patients. The intrinsic relationship between tumor-infiltrating PDPN+ cells and ACT resistance is worth investigating. Moreover, targeting PDPN synergizes with adjuvant chemotherapy may be considered in the future.
Originally, PDPN is applied as a lymphatic vessel marker in multiple cancer types including bladder cancer.Citation34 Beyond its ability to mark lymphatic endothelial cells, we identified a poor prognosis and immunoevasive subtype MIBC with tumor-infiltrating PDPN+ cell abundance. The study of PDPN being a T cell ICK elucidates that PDPN deficiency in CD8+ T cells results in an enhancement of TNF-α production,Citation17 which is partly consistent with our finding that PDPN+ cell infiltration correlated with diminished TNF-α expression in tumor microenvironment. Furthermore, we revealed that PDPN+ cell infiltration correlated with other ICK expression including PD-1, PD-L1, LAG-3 and TIM-3. Previous studies demonstrated that the frequency of Foxp3+ Tregs was significantly elevated in mice with T-cell-specific overexpression of PDPN compared with the wild type.Citation35 It was also reported that PDPN-deficient macrophages impaired the expression of Arg1, a phenotype marker for M2 macrophages.Citation15 Through comparing the immune contexture between PDPN+ cells high and low infiltration groups, we found that PDPN+ cell abundance identified the immune contexture with increased Tregs and M2 macrophage infiltration in MIBC which were both associated with immunosuppression leading to poor prognosis.Citation25,Citation30
There is a growing recognition that molecular classification may predict tumor progression and provide information on effective therapeutic responsiveness, including ACT, ICK blockade therapies and targeted therapies.Citation4,Citation5 We found that tumors with high PDPN expression could present a basal/squamous-like characterization, which was associated with poor prognosis, high EGFR and its ligand expression and high ICK expression.Citation10,Citation11 High T-cell-inflamed signature was also observed in high PDPN expression group, which was a marker for favorable response to anti-PD-L1 immunotherapy.Citation21 Thus, we inferred that PD-L1 blockade immunotherapy or EGFR-targeted therapy may potentially take effect in these tumors. We also revealed that PDPN expression correlated with p53-like signature, which is enriched in luminal-infiltrated and stroma-rich subtypesCitation10,Citation11 and previously reported as a marker for predicting resistance to neoadjuvant chemotherapy.Citation36 Meanwhile, low PDPN expression was detected in tumors with luminal-papillary or LumP subtype featuring with good prognosis and FGFR3 mutation.Citation10,Citation11 FGFR3-targeted therapy may be an encouraging choice for low PDPN expression tumors. Reclassification of bladder cancer by molecular heterogeneity provided an innovative approach to improving the therapeutic effectiveness. PDPN expression possessed the potential to classify MIBC subgroups and guide personalized anti-tumor therapies. The therapy-related predictive value of PDPN and the detailed mechanisms between PDPN and certain therapies need more investigation through in vitro or in vivo experiments.
In summary, tumor-infiltrating PDPN+ cells were identified as an independent prognosticator for survival outcome and a predictive biomarker for poor ACT responsiveness, highlighting its clinical significance in MIBC. Tumors with PDPN+ cell abundance infiltrated intense immunosuppressive contexture. Intratumoral PDPN expression has a relationship with MIBC molecular classification and therapy-related signatures. PDPN could be a potential immunotherapeutic target for treating MIBC.
Disclosure of potential conflicts of interest
No potential conflicts of interest were disclosed.
Consent for publication
All authors provide their consent for publication of the manuscript.
Author contributions
Q. Zhou, Z. Wang, H. Zeng and H. Zhang for acquisition of data, analysis and interpretation of data, statistical analysis and drafting of the manuscript; Z. Liu, Q. Huang, J. Wang, Y. Chang, Q. Bai, L. Liu, Y. Zhu, L. Xu, B. Dai and J. Guo for technical and material support; Y. Xia, Y. Wang and J. Xu for study concept and design, analysis and interpretation of data, drafting of the manuscript, obtained funding and study supervision. All authors read and approved the final manuscript.
Supplemental Material
Download ()Acknowledgments
We thank Dr. Lingli Chen (Department of Pathology, Zhongshan Hospital, Fudan University, Shanghai, China) and Dr. Peipei Zhang (Department of Pathology, Ruijin Hospital, Shanghai Jiao Tong University School of Medicine, Shanghai, China) for their excellent pathological technology help.
Availability of data and materials
All data generated that are relevant to the results presented in this article are included in this article. Other data that were not relevant for the results presented here are available from the corresponding author Dr. Xu upon reasonable request.
Supplementary material
Supplemental data for this article can be accessed on the publisher’s website.
Additional information
Funding
References
- Kamat AM, Hahn NM, Efstathiou JA, Lerner SP, Malmstrom PU, Choi W, Guo CC, Lotan Y, Kassouf W. Bladder cancer. Lancet. [Journal Article; Review]. 2016;388(10061):2796–9. doi:10.1016/S0140-6736(16)30512-8.
- Alfred Witjes J, Lebret T, Compérat EM, Cowan NC, De Santis M, Bruins HM, Hernández V, Espinós EL, Dunn J, Rouanne M, et al. Updated 2016 EAU guidelines on muscle-invasive and metastatic bladder cancer. Eur Urol. 2017;71(3):462–475. doi:10.1016/j.eururo.2016.06.020.
- Leow JJ, Martin-Doyle W, Rajagopal PS, Patel CG, Anderson EM, Rothman AT, Cote RJ, Urun Y, Chang SL, Choueiri TK, et al. Adjuvant chemotherapy for invasive bladder cancer: a 2013 updated systematic review and meta-analysis of randomized trials. Eur Urol. 2014;66(1):42–54. doi:10.1016/j.eururo.2013.08.033.
- Alifrangis C, McGovern U, Freeman A, Powles T, Linch M. Molecular and histopathology directed therapy for advanced bladder cancer. Nat Rev Urol. [Journal Article; Review]. 2019;16(8):465–483. doi:10.1038/s41585-019-0208-0.
- Felsenstein KM, Theodorescu D. Precision medicine for urothelial bladder cancer: update on tumour genomics and immunotherapy. Nat Rev Urol. 2018;15(2):92–111. doi:10.1038/nrurol.2017.179.
- Loriot Y, Necchi A, Park SH, Garcia-Donas J, Huddart R, Burgess E, Fleming M, Rezazadeh A, Mellado B, Varlamov S, et al. Erdafitinib in locally advanced or metastatic urothelial carcinoma. N Engl J Med. [Clinical Trial, Phase II; Journal Article; Multicenter Study; Randomized Controlled Trial; Research Support, Non-U.S. Gov’t]. 2019 Jul 25;381(4):338–348. doi:10.1056/NEJMoa1817323.
- Powles T, Huddart RA, Elliott T, Sarker S, Ackerman C, Jones R, Hussain S, Crabb S, Jagdev S, Chester J, et al. Phase III, double-blind, randomized trial that compared maintenance lapatinib versus placebo after first-line chemotherapy in patients with human epidermal growth factor receptor 1/2–positive metastatic bladder cancer. J Clin Oncol. 2017;35(1):48–55. doi:10.1200/JCO.2015.66.3468.
- Sharma P, Retz M, Siefker-Radtke A, Baron A, Necchi A, Bedke J, Plimack ER, Vaena D, Grimm M-O, Bracarda S et al. Nivolumab in metastatic urothelial carcinoma after platinum therapy (CheckMate 275): a multicentre, single-arm, phase 2 trial. Lancet Oncol. 2017 Jan 01;18(3):312–322. doi:10.1016/S1470-2045(17)30065-7.
- Balar AV, Galsky MD, Rosenberg JE, Powles T, Petrylak DP, Bellmunt J, Loriot Y, Necchi A, Hoffman-Censits J, Perez-Gracia JL, et al. Atezolizumab as first-line treatment in cisplatin-ineligible patients with locally advanced and metastatic urothelial carcinoma: a single-arm, multicentre, phase 2 trial. Lancet. 2017;389(10064):67–76. doi:10.1016/S0140-6736(16)32455-2.
- Robertson AG, Kim J, Al-Ahmadie H, Bellmunt J, Guo G, Cherniack AD, Hinoue T, Laird PW, Hoadley KA, Akbani R, et al. Comprehensive molecular characterization of muscle-invasive bladder cancer. Cell. [Journal Article]. 2017 oct 19;171(3):540–556. doi:10.1016/j.cell.2017.09.007.
- Kamoun A, de Reynies A, Allory Y, Sjodahl G, Robertson AG, Seiler R, Hoadley KA, Groeneveld CS, Al-Ahmadie H, Choi W, et al. A consensus molecular classification of muscle-invasive bladder cancer. Eur Urol. [Journal Article]. 2020 Apr 01;77(4):420–433. doi:10.1016/j.eururo.2019.09.006.
- Pan Y, Yago T, Fu J, Herzog B, McDaniel JM, Mehta-D’Souza P, Cai X, Ruan C, McEver RP, West C, et al. Podoplanin requires sialylated O-glycans for stable expression on lymphatic endothelial cells and for interaction with platelets. Blood. [Journal Article; Research Support, N.I.H., Extramural; Research Support, Non-U.S. Gov’t; Research Support, U.S. Gov’t, Non-P.H.S.]. 2014 Dec 04;124(24):3656–3665. doi:10.1182/blood-2014-04-572107.
- Astarita JL, Acton SE, Turley SJ. Podoplanin: emerging functions in development, the immune system, and cancer. Front Immunol. 2012;3:283. doi:10.3389/fimmu.2012.00283.
- Bieniasz-Krzywiec P, Martín-Pérez R, Ehling M, García-Caballero M, Pinioti S, Pretto S, Kroes R, Aldeni C, Di Matteo M, Prenen H, et al. Podoplanin-expressing macrophages promote lymphangiogenesis and lymphoinvasion in breast cancer. Cell Metab. 2019;30(5):917–936. doi:10.1016/j.cmet.2019.07.015.
- Eisemann T, Costa B, Peterziel H, Angel P. Podoplanin positive myeloid cells promote glioma development by immune suppression. Front Oncol. 2019 Jan 20;9:187. doi:10.3389/fonc.2019.00187.
- Nylander AN, Ponath GD, Axisa P, Mubarak M, Tomayko M, Kuchroo VK, Pitt D, Hafler DA. Podoplanin is a negative regulator of Th17 inflammation. JCI Insight. 2017 Jan 01;2(17). doi:10.1172/jci.insight.92321.
- Chihara N, Madi A, Kondo T, Zhang H, Acharya N, Singer M, Nyman J, Marjanovic ND, Kowalczyk MS, Wang C, et al. Induction and transcriptional regulation of the co-inhibitory gene module in T cells. NATURE. 2018;558(7710):454–459. doi:10.1038/s41586-018-0206-z.
- Fu Q, Xu L, Wang Y, Jiang Q, Liu Z, Zhang J, Zhou Q, Zeng H, Tong S, Wang T, et al. Tumor-associated macrophage-derived interleukin-23 interlinks kidney cancer glutamine addiction with immune evasion. Eur Urol. 2019;75(5):752–763. doi:10.1016/j.eururo.2018.09.030.
- Afonso J, Santos LL, Amaro T, Lobo F, Longatto-Filho A. The aggressiveness of urothelial carcinoma depends to a large extent on lymphovascular invasion–the prognostic contribution of related molecular markers. Histopathology. [Journal Article; Research Support, Non-U.S. Gov’t]. 2009;55(5):514–524. doi:10.1111/j.1365-2559.2009.03425.x.
- Sjodahl G, Lauss M, Lovgren K, Chebil G, Gudjonsson S, Veerla S, Patschan O, Aine M, Ferno M, Ringner M et al. A molecular taxonomy for urothelial carcinoma. Clin Cancer Res. 2012 Jun 15;18(12):3377–3386. doi:10.1158/1078-0432.CCR-12-0077-T.
- Ayers M, Lunceford J, Nebozhyn M, Murphy E, Loboda A, Kaufman DR, Albright A, Cheng JD, Kang SP, Shankaran V, et al. IFN-gamma-related mRNA profile predicts clinical response to PD-1 blockade. J Clin Invest. [Clinical Trial, Phase I; Journal Article; Randomized Controlled Trial]. 2017 Aug 01;127(8):2930–2940. doi:10.1172/JCI91190.
- Subramanian A, Tamayo P, Mootha VK, Mukherjee S, Ebert BL, Gillette MA, Paulovich A, Pomeroy SL, Golub TR, Lander ES et al. Gene set enrichment analysis: a knowledge-based approach for interpreting genome-wide expression profiles. Proc Natl Acad Sci U S A. 2005 Oct 25;102(43):15545–15550. doi:10.1073/pnas.0506580102.
- Tan TZ, Rouanne M, Tan KT, Huang RY, Thiery JP. molecular subtypes of urothelial bladder cancer: results from a meta-cohort analysis of 2411 tumors. Eur Urol. [Journal Article; Meta-Analysis; Research Support, Non-U.S. Gov’t]. 2019 Mar 01;75(3):423–432.
- Hanzelmann S, Castelo R, Guinney J. GSVA: gene set variation analysis for microarray and RNA-seq data. BMC Bioinf. [Journal Article; Research Support, N.I.H., Extramural; Research Support, Non-U.S. Gov’t]. 2013;14:7. doi:10.1186/1471-2105-14-7.
- Fridman WH, Zitvogel L, Sautès Fridman C, Kroemer G. The immune contexture in cancer prognosis and treatment. Nat Rev Clin Oncol. 2017;14(12):717–734. doi:10.1038/nrclinonc.2017.101.
- Fu H, Zhu Y, Wang Y, Liu Z, Zhang J, Xie H, Fu Q, Dai B, Ye D, Xu J et al. Identification and validation of stromal immunotype predict survival and benefit from adjuvant chemotherapy in patients with muscle-invasive bladder cancer. Clin Cancer Res. 2018 Jul 01;24(13):3069–3078. doi:10.1158/1078-0432.CCR-17-2687.
- Henrique R, Nunes SP, Jeronimo C. MSH2 Expression and Resistance to Cisplatin in Muscle-invasive Bladder Cancer: A Mix of Progress and Challenges. EUR UROL. [Editorial; Comment]. 2019 Feb 01;75(2):251–252. doi:10.1016/j.eururo.2018.11.014.
- Van Allen EM, Mouw KW, Kim P, Iyer G, Wagle N, Al-Ahmadie H, Zhu C, Ostrovnaya I, Kryukov GV, O’Connor KW et al. Somatic ERCC2 mutations correlate with cisplatin sensitivity in muscle-invasive urothelial carcinoma. Cancer Discov. 2014 Oct 01;4(10):1140–1153. doi:10.1158/2159-8290.CD-14-0623.
- Liu D, Plimack ER, Hoffman-Censits J, Garraway LA, Bellmunt J, Van Allen E, Rosenberg JE. Clinical validation of chemotherapy response biomarker ERCC2 in muscle-invasive urothelial bladder carcinoma. JAMA Oncol. [Letter; Validation Studies]. 2016;2(8):1094–1096. doi:10.1001/jamaoncol.2016.1056.
- Schneider AK, Chevalier MF, Derré L. The multifaceted immune regulation of bladder cancer. Nature reviews. Urology. 2019 Jan 01;16(10):613–630. doi:10.1038/s41585-019-0226-y.
- Liu Z, Zhu Y, Xu L, Zhang J, Xie H, Fu H, Zhou Q, Chang Y, Dai B, Xu J, et al. Tumor stroma-infiltrating mast cells predict prognosis and adjuvant chemotherapeutic benefits in patients with muscle invasive bladder cancer. ONCOIMMUNOLOGY. [Journal Article]. 2018 Jan 20;7(9):e1474317. doi:10.1080/2162402X.2018.1474317.
- Zhou L, Xu L, Chen L, Fu Q, Liu Z, Chang Y, Lin Z, Xu J. Tumor-infiltrating neutrophils predict benefit from adjuvant chemotherapy in patients with muscle invasive bladder cancer. ONCOIMMUNOLOGY. 2017 Jan 01;6(4):e1293211. doi:10.1080/2162402X.2017.1293211.
- Jiang Q, Fu Q, Chang Y, Liu Z, Zhang J, Xu L, Zhu Y, Wang Y, Zhang W, Xu J, et al. CD19+ tumor-infiltrating B-cells prime CD4+ T-cell immunity and predict platinum-based chemotherapy efficacy in muscle-invasive bladder cancer. Cancer Immunol Immunother. 2019;68(1):45–56. doi:10.1007/s00262-018-2250-9.
- Mathieu R, Lucca I, Roupret M, Briganti A, Shariat SF. The prognostic role of lymphovascular invasion in urothelial carcinoma of the bladder. Nat Rev Urol. [Journal Article; Review]. 2016;13(8):471–479. doi:10.1038/nrurol.2016.126.
- Peters A, Burkett PR, Sobel RA, Buckley CD, Watson SP, Bettelli E, Kuchroo VK. Podoplanin negatively regulates CD4+ effector T cell responses. J Clin Invest. [Journal Article; Research Support, N.I.H., Extramural; Research Support, Non-U.S. Gov’t]. 2015;125(1):129–140. doi:10.1172/JCI74685.
- Choi W, Porten S, Kim S, Willis D, Plimack ER, Hoffman-Censits J, Roth B, Cheng T, Tran M, Lee I-L et al. Identification of distinct basal and luminal subtypes of muscle-invasive bladder cancer with different sensitivities to frontline chemotherapy. Cancer Cell. 2014 Jan 01;25(2):152–165. doi:10.1016/j.ccr.2014.01.009.