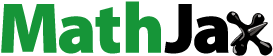
ABSTRACT
Programmed cell death-1 (PD-1) and/or cytotoxic T lymphocyte–associated antigen 4 (CTLA-4) immune checkpoint inhibitor (ICI) treatments are associated with adverse events (AEs), which may be dependent on ICI dose. Applying a model-based meta-analysis to evaluate safety data from published clinical trials from 2005 to 2018, we analyzed the dose/exposure dependence of ICI treatment-related AE (trAE) and immune-mediated AE (imAE) rates. Unlike with PD-1 inhibitor monotherapy, CTLA-4 inhibitor monotherapy exhibited a dose/exposure dependence on most AE types evaluated. Furthermore, combination therapy with PD-1 inhibitor significantly strengthened the dependence of trAE and imAE rates on CTLA-4 inhibitor dose/exposure.
Introduction
Immune checkpoint inhibitors (ICIs) have shown efficacy across various cancers and are being evaluated in multiple clinical trials.Citation1 ICIs are associated with immune-mediated adverse events (imAEs), particularly in combination therapy.Citation2 Immunological checkpoints function to prevent autoimmune reactions; therefore, their inhibition can cause immune dysregulation and autoimmune-type reactions that lead to imAEs.Citation3,Citation4 Additional AEs, more typical of reactions to chemotherapy, have also been observed with programmed cell death ligand-1 (PD-L1) and programmed cell death-1 (PD-1) inhibitors.Citation5 The management of AEs and patient safety using appropriate ICI dosing regimens is, therefore, an essential consideration in the optimization of ICI monotherapy and combination therapy.Citation3,Citation4
Multiple clinical trials and reviews of the safety of cytotoxic T lymphocyte–associated antigen 4 (CTLA-4) inhibitors, PD-1 inhibitors, and PD-L1 inhibitors, and their combinations using pooled and meta-analysis approaches have been published.Citation1–Citation35 Previously, AEs have been evaluated for various organ classes, including gastrointestinal,Citation14 dermatologic,Citation15 hepatic,Citation9 renal,Citation16 endocrine,Citation17 pulmonary,Citation18,Citation19 and other rare immune-related AEs.Citation20 It has been shown that the type, incidence rate and severity of imAEs differ for the different ICIs classes and further increase under combination treatments with CTLA-4 and PD-(L)1 blocking antibodies.Citation4,Citation6,Citation26
However, despite such a substantial amount of clinical ICI safety data generated to date, a full mechanistic understanding on the pathophysiology of imAEs and a potential relationship between imAE rates and ICI efficacy are still lacking.Citation36 To date, factors such as baseline T cell repertoire, cytokine and antibody profiles, as well as gut microbiota composition, have been found to be associated with a higher risk of imAEs in single studies.Citation37,Citation38 In terms of a relationship between AEs and ICI dose/exposure, analyses have focused on a limited number of doses, and dose dependence has not been systematically quantified for any of these organ classes. Trough levels at steady state have been used in a study of ipilimumab AE rates, based on individual-level data for 498 patients pooled from 4 phase 2 studies, where the dose-dependent effect was quantified using a logistic regression model.Citation25 However, these early modeling results have not been validated further in later studies. Therefore, in continuation of our previous work,Citation21 we aimed to apply model-based meta-analysis methodologies for the quantitative study of the relationship between ICI dose and AEs.
Materials and methods
Literature search and data collection
The Preferred Reporting Items for Systematic Reviews and Meta-Analyses (PRISMA) guidelines were followed for article search and selection.Citation39 Two investigators (BS and AO) independently searched and assessed the PubMed-Medline and Citeline TrialtroveCitation40 databases, along with the published American Society of Clinical Oncology (ASCO) and European Society for Medical Oncology (ESMO) abstracts, to identify relevant PD-1 and CTLA-4 ICI safety data published from 2005 to 2018. For the PubMed-Medline search, the following keywords were used: nivolumab OR pembrolizumab OR ipilimumab OR tremelimumab OR lambrolizumab OR ticilimumab OR (CTLA-4 OR PD-1) AND (safety OR adverse events) AND (oncology OR cancer). For the published ASCO abstracts search, the following keywords were used: nivolumab OR pembrolizumab OR ipilimumab OR tremelimumab, with a similar search performed for the published ESMO abstracts and the Citeline Trialtrove database. In addition, we also reviewed references identified within published systematic reviews and meta-analyses. The AEs grouped by organ class have not been systematically reported for PD-L1 inhibitors; these trials were not included in the current analysis.
Adverse Events
Reporting of specific imAE types may vary across trials, thereby affecting the synthesis of evidence for each specific imAE.Citation29 In the current study, subgroup analyses were conducted for grade 3/4 imAEs for 5 organ classes: gastrointestinal, skin, pulmonary, hepatic, and endocrine. Rates of total treatment-related AEs (trAEs) and total grade 3/4 trAEs were also evaluated. The majority of studies included in the analysis-classified AEs according to the Medical Dictionary for Regulatory Activities, and AE grades were defined according to CTCAE v4.03 guidance.
Immune checkpoint inhibitor dose/exposure
Two PD-1 inhibitors (nivolumab and pembrolizumab) and two CTLA-4 inhibitors (ipilimumab and tremelimumab) were evaluated in both monotherapy and combination therapy settings. To combine and compare AEs across different ICI dosing schedules, we proposed a novel methodology accounting for differences in ICI pharmacokinetics (PK) and potency (with respect to target receptor binding). We used population PK models for ICIs as published in the literature, along with documents from the United States Food and Drug Administration and the European Medicines Agency.Citation41–Citation54 Based on parameter values derived from these PK models, we simulated exposure profiles in plasma for various sets of administered dosing regimens. Concentrations were averaged when steady-state conditions were assumed to have been reached. The simulated averaged concentration was next normalized by the drug concentration at which 50% inhibition of the native target receptor is achieved (IC50). Published IC50 values have been measured in vitro. Normalization of drug exposure by the drug IC50 value allowed us to combine AE data from different ICIs acting on the same target receptor (refer to Supplemental Methods). All details of the PK models and derived parameter values are summarized in Supplemental Tables 1 and 2.
Table 1. Subgroup analysis of AE rates and ICI dose/exposure.
Statistical analysis
A meta-analysis was performed using trial-level data and based on a random-effects model. The Cochran Q test was used to detect heterogeneity across the different trials and between subgroups. Publication bias was evaluated by Funnel plots and Egger’s test. To determine the relationship between dose/exposure and AEs, we performed a subgroup analysis by splitting exposure intervals into high and low subgroups. Patients were assigned to subgroups based on the mean normalized drug exposure value calculated for each cohort. In fact, due to the reduced number of doses tested in the clinical programs considered, the ‘low exposure’ subgroup mostly included approved ICI dose levels, while the ‘high’ subgroup included cohorts with doses higher than the approved dosing and consequently closer to the MTD level. Logit-transformed AE rates were used and, when averaged AE rates for the subgroups were <5% for a particular organ class, AEs were considered to be rare. For rare AEs, the normal distribution assumption for within-trial variability is no longer valid and has been shown to lead to a bias in the estimation of the mean effect size.Citation55 Thus, for rare AEs, a normal-binomial general linear mixed model approach was used.Citation56
A meta-regression analysis was performed to derive a functional form of AE rate dependence on ICI dose/exposure to (i) quantitatively estimate parameters that would characterize dependence and (ii) evaluate the influence of patient baseline characteristics on dependence. The following models were tested to determine which model described the data with maximum likelihood:
Model 1, additive:
Model 2, supra-additive:
Model 3, supra-additive with a binominal PD-1 inhibitor effect:
Model 4, CTLA-4 inhibitor–driven, with PD-1 inhibitor-dependent modulation:
where refers to the normalized averaged steady-state concentration of an anti-CTLA-4 drug, and
corresponds to the logit-transformed probability of a given AE averaged across studies. Supra-additive refers to a cross term in model equations, characterizing synergism between PD-1 inhibitor and CTLA-4 inhibitor effects.
is set to 1 if a PD-1 inhibitor drug was given as monotherapy or in combination, and to 0 otherwise. To test sensitivity and to assess potential confounding factors, the following trial-level patient baseline characteristics were selected in a sequential (forward and backward) step-wise covariate search (see Supplemental Tables 1 and 3 for detailed explanations): line of therapy (first-line versus second-line or later therapy), cancer type (non-small cell lung cancer [NSCLC] versus melanoma versus others), ICI therapy combination + standard chemotherapy, ICIs (ipilimumab versus tremelimumab; nivolumab versus pembrolizumab), median age of patients in the study/cohort, percentage of males versus females, and percentage of PD-L1-positive patients at baseline. PD-L1 positivity was defined according to the thresholds used in the included studies: PD-L1 threshold was 1% for 30 studies, 5% for 3 studies, and 50% for 1 study. The final model was chosen based on multiple criteria, including the value of the Akaike information criterion, with correction for small sample size, confidence intervals (CIs) of the regression coefficients, and different model diagnostic plots. Details of the meta-regression modeling and study-level characteristics are presented in Supplemental Table 1–4. The R statistics package metaforCitation57 was used to evaluate the statistical significance (p < .05) for dose dependence.
Results
Study selection and characteristics
A total of 417 data sources were selected for further analysis. Of these, we selected 102 eligible articles and abstracts (Supplemental Figure 1). Given the recent trend toward ICI studies having nonrandomized designs,Citation58 single-arm studies meeting the inclusion criteria were included.Citation7,Citation11,Citation15,Citation17,Citation18 The selected articles included 153 treatment cohorts of 21,305 patients who received PD-1 or CTLA-4 ICI monotherapy or combination therapy across 80 clinical trials. A detailed description of the included studies is provided in Supplemental Table 5. Publication bias analysis revealed no significant asymmetry, indicating no obvious publication bias with respect to both total trAE and tissue-specific imAE types (Supplemental Figure 2).
AE analysis by ICI dose/exposure
The association between AE rates and dose/exposure was not statistically significant for PD-1 inhibitor monotherapy (; Supplemental Figure 3). For CTLA-4 inhibitor monotherapy, total grade 3/4 trAE rates were significantly higher in the high-dose/exposure subgroup versus the low-dose/exposure subgroup (37% versus 23%, respectively; p < .001), along with hepatic imAE rates (7.0% versus 1.1%, respectively; p < .001; and ; Supplemental ). There was a significant association between the rates of total trAEs (p < .05), total grade 3/4 trAEs (p < .001), gastrointestinal imAEs (p < .01), hepatic imAEs (p < .001), and skin imAEs (p < .05) with PD-1 inhibitor + CTLA-4 inhibitor combination therapy dose/exposure ( and ; Supplemental Figure 3).
Meta-regression analyses
To provide a more formal quantification of AE dependencies upon ICI dose/exposure, as well as the testing of the different trial-level patient baseline characteristics, statistical meta-regression analyses were conducted. There was no significant increase in AE rates with increased dose/exposure of PD-1 inhibitor monotherapy, while increases in CTLA-4 inhibitor dose/exposure led to significant increases in total grade 3/4 trAEs, hepatic imAEs, and gastrointestinal imAEs (). For PD-1 inhibitor + CTLA-4 inhibitor combination therapy, AE rates increased with a higher CTLA-4 inhibitor dose/exposure only. The model that best described the total grade 3/4 trAE rate dependence on ICI dose/exposure was model 4 (see Methods), where the total grade 3/4 trAE rate was driven by CTLA-4 inhibitor exposure with a PD-1 inhibitor-dependent modulation. According to model 4, the regression coefficient describing the CTLA-4 inhibitor effect increased from 0.0015 (95% CI, 0.001–0.002; p < .001) to 0.0124 (95% CI, 0.0095–0.0153; p < .001) in the presence of a PD-1 inhibitor, which explained the significantly higher AE rate observed with the combination treatment, despite lower CTLA-4 inhibitor doses in combination therapy versus monotherapy (). To further validate these results, we performed a similar analysis for gastrointestinal imAEs and hepatic imAEs. Similar to the total grade 3/4 trAE results, model 4 was shown to be the optimal model based on the objective function value and other diagnostic criteria ().
Additional factors influencing AE rates
Meta-regression analysis was used to determine additional characteristics that affected AE rates during ICI treatment. Only grade 3/4 trAEs were evaluated, which included, but were not limited to, imAEs. Combining a PD-1 inhibitor with standard chemotherapy resulted in additive toxicity, which increased total grade 3/4 trAE rates from 17.7% (90% prediction interval [PI], 16.2%-19.4%) in monotherapy studies to 39.0% (90% PI, 29.4%-49.5%) in chemotherapy combination studies (). Additionally, first-line ICI therapy was shown to increase the regression coefficient for the CTLA-4 inhibitor dose/exposure effect from 0.0004 (95% CI, 0.0001–0.0011; p < .001) to 0.0034 (95% CI, 0.0031–0.0037; p < .001), corresponding to an increase in total grade 3/4 trAEs in studies evaluating CTLA-4 inhibitors in the first-line setting (). Incorporation of these two additional covariates into the final model did not change the functional form of the dependence of trAE rates on CTLA-4 inhibitor and PD-1 inhibitor dose/exposure. This allowed us to prospectively predict total grade 3/4 trAE rates for treatment options that have not yet been tested in clinical trials: a triple combination of PD-1 inhibitor + CTLA-4 inhibitor + standard chemotherapy was predicted to achieve a grade 3/4 trAE rate of 50% at lower doses than doses tested in clinical trials of ICI dual combination therapy ().
There were no statistically significant differences in AE rates across different cancer types (Supplemental Table 2). This finding suggests that ICI-related AE rates across cancer types may not significantly differ and supports the practice of pooling ICI AE data across different cancer types when performing meta-analyses. We did not observe any statistically significant effect when incorporating ICI type for a given target class as a covariate, illustrating that CTLA-4 inhibitors and PD-1 inhibitors exhibit similar safety profiles within their target class (Supplemental Table 2). Within the CTLA-4 target class, AE differences between tremelimumab and ipilimumab have been reported once.Citation7 However, when the line of therapy and drug-specific normalized exposure were included as covariates in the present meta-analysis, no statistically significant differences between tremelimumab and ipilimumab were found (Supplemental Tables 2 and 3).
Discussion
It is now well understood that autoimmunity is an integral part of the immune system and self-reactivity preserving in T cell repertoire despite the clonal selection controls many aspects of lymphocyte biology.Citation59,Citation60 In the healthy state immune, autoreactive reactions are tightly controlled via multiple regulatory mechanisms, e.g., via expression of various immune checkpoint molecules.Citation61 Therefore, the phenomenon of peripheral immune tolerance is in fact assembled by the dynamic ratio of multiple co-stimulatory and co-inhibitory interactions.Citation62 These general immunological concepts formulated decades ago were supported nowadays with the results from the multiple clinical trials of CTLA-4, PD-1 and PD-L1 blocking antibodies, which all in addition to remarkable antitumor responses have specific profiles of immune-mediated autoreactive AEs.Citation4,Citation6,Citation26
This model-based meta-analysis evaluated safety data from 80 published clinical trials (representing 21,305 patients from 153 dosing cohorts), which, to our knowledge, is the largest analysis conducted to date and represents the first attempt to analyze the dose/exposure dependence of PD-1 and CTLA-4 ICI trAE and imAE rates upon the aggregation of all published AE data. Moreover, previous analyses of AE rate dose/exposure dependence for CTLA-4 inhibitors and PD-1 inhibitors have been limited to specific dosing regimens, without consideration of PK modeling and normalization by drug exposure.Citation2,Citation4,Citation7,Citation22 To analyze and compare AEs across trials, we proposed a novel methodology by deriving an average concentration at steady state as a measure of dose/exposure to the respective ICI, and further normalized average concentrations by drug-specific potencies (IC50 values). This allowed us to combine data for more than one ICI acting on the same target class (namely, PD-1 [nivolumab and pembrolizumab] or CTLA-4 [ipilimumab and tremelimumab]) and to perform meta-regression on dose for the quantitative characterization of dose/exposure dependence, while also evaluating the influence of patient baseline characteristics. Moreover, as opposed to AEs occurring within a specific organ class (e.g., colitis and diarrhea), the present study evaluated total trAE and imAE rates per organ class (e.g., gastrointestinal), which broadened the range of evaluated safety measures and showed that the effects observed (e.g., total AE measures) are in fact driven by events of immune-mediated origins.
The main results derived from our meta-analysis are in agreement with existing knowledge on AEs vs dose/exposure dependences, as observed in single drug development programs. For example, AE rates with PD-1 inhibitor monotherapy did not show dose/exposure dependence, which is probably due to the high specificity of PD-1 blocking antibodies and saturating biological effects (T-cell activation) with most doses tested in clinical trials. Thus, a 0.5 mg/kg Q3 W dose of pembrolizumab, which is nearly 10 times lower than the approved dose, shows 90% target engagement.Citation63 The absence of a dose/exposure vs efficacy dependence has also been observed in pooled analyses of Phase 1 and Phase 2 trials of pembrolizumab.Citation64 Similar results have been observed in Phase 1 trials of nivolumab, another approved PD-1 blocking antibody, with a dosing regimen of 0.3 mg/kg Q2 W resulting in saturating target engagement.Citation65
In contrast to PD-1 blocking antibodies, CTLA-4 inhibitors as monotherapies or in combination with a PD-1 inhibitor exhibited robust AE dose/exposure dependence for most of the AE types evaluated. These results, obtained on aggregated clinical data, are in good agreement with earlier studies of ipilimumabCitation22,Citation66,Citation67 and tremelimumab,Citation68 where AE rates, especially of grades 3 and 4, increased with drug exposure levels and were quantitatively described with population dose-exposure-safety modeling.Citation25 Such a robust dose-exposure safety relationship may be indicative of autoimmune reactions; for example, it has been shown that CTLA-4 blocking not only increases counts of activated CD4+ and CD8 + T cell subsets,Citation69–Citation71 but also significantly increases the number of TCR clonotypes.Citation72–Citation74 The qualitative and quantitative boosting of peripheral T cell recruitment via CTLA-4 blocking would inevitably cause a higher probability of autoimmune reactions. In support of this mechanism, it has been shown recently that the increase of the number of circulating T-cell effector clones with CTLA-4 inhibition can be a strong driver of immune-mediated toxicity.Citation75
In accordance with accumulating clinical evidence,Citation6 our analysis also indicates that combination therapy with CTLA-4 and PD-1 inhibitors may result in significantly higher AE rates. It should be noted that while AE rates with PD-1 inhibitor monotherapy did not exhibit any dose/exposure dependence, combination therapy with PD-1 and CTLA-4 inhibitors was found to significantly strengthen the dependence of trAE and imAE rates on CTLA-4 inhibitor dose/exposure. In fact, the functional form of the final meta-regression equation supports the addition of a multiplicative coefficient (β3), and thus supports the view that combination treatment with CTLA-4 and PD-1 inhibitors increases AE rates beyond additivity. This would suggest that, similarly to the beyond-additive efficacy benefits observed in melanoma, NSCLC and renal cell carcinoma, a combination of these two classes of ICIs may cause immune-mediated toxicity through different mechanisms.Citation76,Citation77 For example, the addition of a PD-1 inhibitor may increase the immune sensitivity of peripheral compartments (including tumor) to the boosted recruitment of newly primed CD4+ and CD8+ cells caused by CTLA-4 inhibition in lymphoid organs;Citation78,Citation79 this, in turn, may lead to a higher rate of imAEs. Interestingly, the corresponding mathematical equation describing the dependence of various trAE and imAE rates on PD-1 and CTLA-4 drug exposure (Model 4 in Materials and Methods) conceptually reflects autoimmune effects caused by lymphopenia-induced proliferation,Citation80 an autoreactive immune process experimentally shown to be amplified under PD-1 blockade.Citation81,Citation82
Another interesting finding from the presented meta-analysis is that AE rates increased with increasing CTLA-4 ICI doses, in patients receiving first-line vs second-line or later therapy. Similar differences in rates of pneumonitis in NSCLC patients receiving first-line vs second-line or later therapy have been reported; however, no significant differences for other AEs have been observed.Citation11 A similar effect of therapy line has also been shown for grade 3/4 trAEs in patients with advanced melanoma treated with pembrolizumab or ipilimumab, coupled with a trend toward greater efficacy in the first-line setting.Citation28 The current data would not be sufficient to derive a clear mechanistic explanation for this observation. However, since the line of treatment (similarly to PD-1 inhibition – see the previous paragraph) may increase the sensitivity to CTLA-4 drug exposure (eTable3), it can be hypothesized that this effect may be dependent on the overall state of systemic immunity, which in fact can be compromised given a patient’s treatment history, e.g., chemotherapy treatmentCitation83 or progressive disease (increased tumor or metastatic burden), which all may affect both the efficacy and AE profiles of ICIs in subsequent therapies.Citation84,Citation85 Incorporation of a line-of-treatment covariate provides an opportunity for a more accurate comparison of safety profiles across ICIs. For example, we did not observe differences in AE dose dependence between ipilimumab and tremelimumab using our model-based framework, once the line of therapy was taken into account as a covariate (Supplemental Tables 2 and 3); this is in contrast to an early safety meta-analysis of anti-CTLA-4 antibodies, which showed lower AE rates for tremelimumab but did not take into account line of treatment as a covariate.Citation7 Differences in AE rates for these two anti-CTLA-4 drugs were likely due to tremelimumab being mainly evaluated in patients receiving second-line or later therapy, while ipilimumab has been evaluated in both first-line and second-line or later settings, with AE rates being higher in the first-line vs second-line or later setting, as shown in the present analysis.
Another covariate that independently affected AE rates was “combination of ICI with chemotherapy”; such combinations resulted in significantly higher AE rates vs ICI monotherapy treatment. Based on the currently available data, it would be challenging to conclude whether these increased AE rates are additive or beyond-additive, in such a combination setting with chemotherapy.Citation86–Citation88 A recently published meta-analysis of NSCLC trials revealed that combining a PD-1 inhibitor with chemotherapy affects both total trAEs and imAEs,Citation89 which would support the mechanistic hypothesis of a link between immunogenic cell death and its impact on systemic immunity; for example, specific types of chemotherapies may stimulate immunological effects, including a decrease in regulatory T-cell activity, an enhancement of tumor antigen presentation, and an induction of PD-L1 expression on tumor cells, which may increase ICI action and subsequently lead to increases in AE rates.Citation90
One additional limitation of the present work is that AEs have not been systematically reported by organ classes for all of the published clinical trials and, in particular, for PD-L1 inhibitors; these trials could not be included in the analysis. However, several meta-analyses suggest that treatments using PD-L1 inhibitors may exhibit lower imAE rates vs PD-1 inhibitors; thus, safety data for these two related ICI classes should not be pooled.Citation91–Citation93 More detailed analyses would be warranted to confirm such differences, including one making use of the same model-based methodology presented here (normalization by drug exposure and grouping AEs by organ class) and possibly augmented with patient-level AE data. Also, no published data on single-dose ICI therapies exist; hence, this analysis focused on settings making use of multiple dose regimens.
Despite a number of limitations, the present model-based approach provides a valuable quantitative framework for a joint analysis of clinical safety data from multiple ICIs currently available on the market or as tested in clinical trials. More importantly, this quantitative approach allows to extrapolate and predict safety outcomes for alternative treatment dosing regimens. This may be of special interest toward the development of therapeutic combinations, since efficacy and safety outcomes are only partially correlated for the various ICIs. Thus, it has recently been confirmed, in a Phase 3 trial, that reduction of dose and administration frequency of ipilimumab, down to a 1 mg/kg Q6 W regimen and in combination with a 240 mg Q2 W dose of nivolumab can significantly reduce Grade 3/4 total AEs rates while not compromising on efficacy, in those responding patients.Citation94 These results are in excellent agreement with a growing number of clinical reports, which indicate that an adequate reduction in dose does not compromise disease control or overall survival outcome measures.Citation62,Citation63,Citation95-Citation97 The present meta-analysis, combined with quantitative population PKPD analyses of patient-level clinical data may provide a very robust framework for the further optimization of ICI dosing regimens, especially in combination settings.
Conclusion
AE rates for PD-1 inhibitor monotherapy were not dose/exposure dependent. Significant AE dose/exposure dependence for CTLA-4 inhibitor monotherapy, CTLA-4 inhibitor + PD-1 inhibitor combination therapy, and ICI + chemotherapy combination therapy was observed for multiple AE types. Patients receiving first-line ICI therapy had higher AE rates vs patients receiving second-line or later ICI therapy. There was no influence of patient characteristics, such as PD-L1 status, on the observed relationships between AE rates and ICI dose/exposure. This novel model-based meta-analysis methodology provides a quantitative framework for positioning ICI doses and dosing regimens with respect to specific AE rates.
Abbreviations
AE | = | Adverse event |
ASCO | = | American Society of Clinical Oncology |
CI | = | Confidence interval |
CTLA-4 | = | Cytotoxic T lymphocyte–associated antigen 4 |
ESMO | = | European Society for Medical Oncology |
IC50 | = | Half maximal inhibitory concentration |
ICI | = | Immune checkpoint inhibitor |
imAE | = | Immune-mediated adverse event |
NSCLC | = | Non-small cell lung cancer |
PD-1 | = | Programmed cell death-1 |
PD-L1 | = | Programmed cell death ligand-1 |
PI | = | Prediction interval |
PK | = | Pharmacokinetics |
PRISMA | = | Preferred Reporting Items for Systematic Reviews and Meta-Analyses |
trAE | = | Treatment-related adverse event |
Author contributions
All authors were involved in the concept and design of the study and data collection. BS, YK, AO, and KP conducted the analysis. All authors actively participated in manuscript development. All authors reviewed the manuscript drafts and approved the submission of the manuscript to this journal.
Declarations
Ethics approval and consent to participate: Not applicable
Availability of data and materials: Data underlying the findings described in this manuscript may be obtained in accordance with AstraZeneca’s data sharing policy described at https://astrazenecagrouptrials.pharmacm.com/ST/Submission/Disclosure
Supplemental Material
Download ()Acknowledgments
The authors thank Eric Masson, Kald Abdallah, and Andrea Vergara-Silva for scientific discussions on this work. We thank Artem Dolgun for help with data programming. Editorial support was provided by Liam Gillies, PhD, of Cactus Communications, and was funded by AstraZeneca.
Disclosure statement
BS, YK, AO, and KP are employees of M&S Decisions LLC, Moscow, Russia, which received funding from AstraZeneca to conduct this research and analysis; LC, GM, SA, RP, GD, GK, and GH are all employees of AstraZeneca.
Supplementary Material
Supplemental data for this article can be accessed on the publisher’s website.
Additional information
Funding
References
- Gong J, Chehrazi-Raffle A, Reddi S, Salgia R. Development of PD-1 and PD-L1 inhibitors as a form of cancer immunotherapy: a comprehensive review of registration trials and future considerations. J Immunother Cancer. 2018;6(1):8. doi:10.1186/s40425-018-0316-z.
- Marrone K, Ying W, Naidoo J. Immune-related adverse events from immune checkpoint inhibitors. Clin Pharmacol Ther. 2016;100(3):242–12. doi:10.1002/cpt.394.
- Boutros C, Tarhini A, Routier E, Lambotte O, Ladurie FL, Carbonnel F, Izzeddine H, Marabelle A, Champiat S, Berdelou A, et al. Safety profiles of anti-CTLA-4 and anti-PD-1 antibodies alone and in combination. Nat Rev Clin Oncol. 2016;13(8):473–486. doi:10.1038/nrclinonc.2016.58.
- Michot JM, Bigenwald C, Champiat S, Collins M, Carbonnel F, Postel-Vinay S, Berdelou A, Varga A, Bahleda R, Hollebecque A, et al. Immune-related adverse events with immune checkpoint blockade: a comprehensive review. Eur J Cancer. 2016;54:139–148. doi:10.1016/j.ejca.2015.11.016.
- Nishijima TF, Shachar SS, Nyrop KA, Muss HB. Safety and tolerability of PD‐1/PD‐L1 inhibitors compared with chemotherapy in patients with advanced cancer: a meta‐analysis. Oncologist. 2017;22(4):470–479. doi:10.1634/theoncologist.2016-0419.
- Hassel JC, Heinzerling L, Aberle J, Bähr O, Eigentler TK, Grimm MO, Grünwald V, Leipe J, Reinmuth N, Tietze JK, et al. Combined immune checkpoint blockade (anti-PD-1/anti-CTLA- 4): evaluation and management of adverse drug reactions. Cancer Treat Rev. 2017;57:36–49. doi:10.1016/j.ctrv.2017.05.003.
- Bertrand A, Kostine M, Barnetche T, Truchetet ME, Schaeverbeke T. Immune related adverse events associated with anti-CTLA-4 antibodies: systematic review and meta-analysis. BMC Med. 2015;13:211. doi:10.1186/s12916-015-0455-8.
- Eigentler TK, Hassel JC, Berking C, Aberle J, Bachmann O, Grünwald V, Kähler KC, Loquai C, Reinmuth N, Steins M, et al. Diagnosis, monitoring and management of immune-related adverse drug reactions of anti-PD-1 antibody therapy. Cancer Treat Rev. 2016;45:7–18. doi:10.1016/j.ctrv.2016.02.003.
- Zhang X, Ran YG, Wang KJ, Zhu YX, Li JH. Incidence and risk of hepatic toxicities with PD-1 inhibitors in cancer patients: a meta-analysis. Drug Des Devel Ther. 2016;10:3153–3161. doi:10.2147/DDDT.S115493.
- Wang PF, Chen Y, Song SY, Wang TJ, Ji WJ, Li SW, Liu N, Yan CX. Immune-related adverse events associated with anti-PD-1/PD-L1 treatment for malignancies: a meta-analysis. Front Pharmacol. 2017;8:730. doi:10.3389/fphar.2017.00730.
- Khunger M, Jain P, Rakshit S, Pasupuleti V, Hernandez AV, Stevenson J, Pennell NA, Velcheti V. Safety and efficacy of PD-1/PD-L1 inhibitors in treatment-naive and chemotherapy-refractory patients with non–small-cell lung cancer: a systematic review and meta-analysis. Clin Lung Cancer. 2018;19(3):e335–48. doi:10.1016/j.cllc.2018.01.002.
- Pillai RN, Behera M, Owonikoko TK, Kamphorst AO, Pakkala S, Belani CP, Khuri FR, Ahmed R, Ramalingam SS. Comparison of the toxicity profile of PD-1 versus PD-L1 inhibitors in non-small cell lung cancer: a systematic analysis of the literature. Cancer. 2018;124(2):271–277. doi:10.1002/cncr.31043.
- Sznol M, Ferrucci PF, Hogg D, Atkins MB, Wolter P, Guidoboni M, Lebbé C, Kirkwood JM, Schachter J, Daniels GA, et al. Pooled analysis safety profile of nivolumab and ipilimumab combination therapy in patients with advanced melanoma. J Clin Oncol. 2017;35(34):3815–3822. doi:10.1200/JCO.2016.72.1167.
- Wei W, Luo Z. Risk of gastrointestinal toxicities with PD-1 inhibitors in cancer patients: a meta-analysis of randomized clinical trials. Medicine (Baltimore). 2017;96(48):e8931. doi:10.1097/MD.0000000000008931.
- Belum VR, Benhuri B, Postow MA, Hellmann MD, Lesokhin AM, Segal NH, Motzer RJ, Wu S, Busam KJ, Wolchok JD, et al. Characterisation and management of dermatologic adverse events to agents targeting the PD-1 receptor. Eur J Cancer. 2016;60:12–25. doi:10.1016/j.ejca.2016.02.010.
- Abdel-Rahman O, Fouad M. A network meta-analysis of the risk of immune-related renal toxicity in cancer patients treated with immune checkpoint inhibitors. Immunotherapy. 2016;8(5):665–674. doi:10.2217/imt-2015-0020.
- Barroso-Sousa R, Barry WT, Garrido-Castro AC, Hodi FS, Min L, Krop IE, Tolaney SM. Incidence of endocrine dysfunction following the use of different immune checkpoint inhibitor regimens: a systematic review and meta-analysis. JAMA Oncol. 2018;4(2):173–182. doi:10.1001/jamaoncol.2017.3064.
- Khunger M, Rakshit S, Pasupuleti V, Hernandez AV, Mazzone P, Stevenson J, Pennell NA, Velcheti V. Incidence of pneumonitis with use of programmed death 1 and programmed death-ligand 1 inhibitors in non-small cell lung cancer: a systematic review and meta-analysis of trials. Chest. 2017;152(2):271–281. doi:10.1016/j.chest.2017.04.177.
- Ciccarese C, Iacovelli R, Bria E, Modena A, Massari F, Brunelli M, Fantinel E, Bimbatti D, Zamboni GA, Artibani W, et al. The incidence and relative risk of pulmonary toxicity in patients treated with anti-PD-1/PD-L1 therapy for solid tumors: a meta-analysis of current studies. Immunotherapy. 2017;9(7):579–587. doi:10.2217/imt-2017-0018.
- Hu YB, Zhang Q, Li HJ, Michot JM, Liu HB, Zhan P, Lv TF, Song Y. Evaluation of rare but severe immune related adverse effects in PD-1 and PD-L1 inhibitors in non-small cell lung cancer: a meta-analysis. Transl Lung Cancer Res. 2017;6(Suppl 1):S8–20. doi:10.21037/tlcr.2017.12.10.
- Abdallah K, Shulgin B, Peskov K, Kosinsky Y, Vergara-Silva A, Helmlinger G, Chu L, Masson E. Model-based meta-analysis of safety for immune checkpoint inhibitor combinations and monotherapy (ASCO abstract 89). J Clin Oncol. 2017;35(Suppl 7):89. doi:10.1200/JCO.2017.35.7_suppl.89.
- Ascierto PA, Del Vecchio M, Robert C, Mackiewicz A, Chiarion-Sileni V, Arance A, Lebbé C, Bastholt L, Hamid O, Rutkowski P, et al. Ipilimumab 10 mg/kg versus ipilimumab 3 mg/kg in patients with unresectable or metastatic melanoma: a randomised, double-blind, multicentre, phase 3 trial. Lancet Oncol. 2017;18(5):611–622. doi:10.1016/S1470-2045(17)30231-0.
- Beer TM, Kwon ED, Drake CG, Fizazi K, Logothetis C, Gravis G, Ganju V, Polikoff J, Saad F, Humanski P, et al. Randomized, double-blind, phase III trial of ipilimumab versus placebo in asymptomatic or minimally symptomatic patients with metastatic chemotherapy-naive castration-resistant prostate cancer. J Clin Oncol. 2017;35(1):40–47. doi:10.1200/JCO.2016.69.1584.
- Eggermont AM, Chiarion-Sileni V, Grob JJ, Dummer R, Wolchok JD, Schmidt H, Hamid O, Robert C, Ascierto PA, Richards JM, et al. Adjuvant ipilimumab versus placebo after complete resection of high-risk stage III melanoma (EORTC 18071): a randomised, double-blind, phase 3 trial. Lancet Oncol. 2015;16(5):522–530. doi:10.1016/S1470-2045(15)70122-1.
- Feng Y, Roy A, Masson E, Chen TT, Humphrey R, Weber JS. Exposure-response relationships of the efficacy and safety of ipilimumab in patients with advanced melanoma. Clin Cancer Res. 2013;19(14):3977–3986. doi:10.1158/1078-0432.CCR-12-3243.
- Khoja L, Day D, Wei-Wu Chen T, Siu LL, Hansen AR. Tumour- and class-specific patterns of immune-related adverse events of immune checkpoint inhibitors: a systematic review. Ann Oncol. 2017;28(10):2377–2385. doi:10.1093/annonc/mdx286.
- Wolchok JD, Chiarion-Sileni V, Gonzalez R, Rutkowski P, Grob JJ, Cowey CL, Lao CD, Wagstaff J, Schadendorf D, Ferrucci PF, et al. Overall survival with combined nivolumab and ipilimumab in advanced melanoma. N Engl J Med. 2017;377(14):1345–1356. doi:10.1056/NEJMoa1709684.
- Carlino MS, Long GV, Schadendorf D, Robert C, Ribas A, Richtig E, Nyakas M, Caglevic C, Tarhini A, Blank C, et al. Outcomes by line of therapy and programmed death ligand 1 expression in patients with advanced melanoma treated with pembrolizumab or ipilimumab in KEYNOTE-006: a randomised clinical trial. Eur J Cancer. 2018;101:236–243. doi:10.1016/j.ejca.2018.06.034.
- Créquit P, Chaimani A, Yavchitz A, Attiche N, Cadranel J, Trinquart L, Ravaud P. Comparative efficacy and safety of second-line treatments for advanced non-small cell lung cancer with wild-type or unknown status for epidermal growth factor receptor: a systematic review and network meta-analysis. BMC Med. 2017;15(1):193. doi:10.1186/s12916-017-0954-x.
- Kang YK, Boku N, Satoh T, Ryu MH, Chao Y, Kato K, Chung HC, Chen JS, Muro K, Kang WK, et al. Nivolumab in patients with advanced gastric or gastro-oesophageal junction cancer refractory to, or intolerant of, at least two previous chemotherapy regimens (ONO-4538-12, ATTRACTION-2): a randomised, double-blind, placebo-controlled, phase 3 trial. Lancet. 2017;390(10111):2461–2471. doi:10.1016/S0140-6736(17)31827-5.
- Owen DH, Wei L, Villalona-Calero MA, Bertino EM, He K, Shields PG, Carbone DP, Otterson GA. Impact of immune-related adverse events (irAE) on overall survival (OS) in patients treated with immunotherapy for non-small cell lung cancer (NSCLC). J Clin Oncol. 2017;35(15 suppl):9080. doi:10.1200/JCO.2017.35.15_suppl.9080.
- Wu J, Hong D, Zhang X, Lu X, Miao J. PD-1 inhibitors increase the incidence and risk of pneumonitis in cancer patients in a dose-independent manner: a meta-analysis. Sci Rep. 2017;7:44173. doi:10.1038/srep44173.
- Weber JS, Hodi FS, Wolchok JD, Topalian SL, Schadendorf D, Larkin J, Sznol M, Long GV, Li H, Waxman IM, et al. Safety profile of nivolumab monotherapy: a pooled analysis of patients with advanced melanoma. J Clin Oncol. 2017;35(7):785–792. doi:10.1200/JCO.2015.66.1389.
- Peng TR, Tsai F-P, Wu T-W. Indirect comparison between pembrolizumab and nivolumab for the treatment of non-small cell lung cancer: a meta-analysis of randomized clinical trials. Int Immunopharmacol. 2017;49:85–94. doi:10.1016/j.intimp.2017.05.019.
- Sato K, Akamatsu H, Murakami E, Sasaki S, Kanai K, Hayata A, Tokudome N, Akamatsu K, Koh Y, Ueda H, et al. Correlation between immune-related adverse events and efficacy in non-small cell lung cancer treated with nivolumab. Lung Cancer. 2018;115:71–74. doi:10.1016/j.lungcan.2017.11.019.
- Postow MA, Sidlow R, Hellmann MD. Immune-related adverse events associated with immune checkpoint blockade. N Engl J Med. 2018;378(2):158–168. doi:10.1056/NEJMra1703481.
- Palmieri DJ, Carlino MS. Immune checkpoint inhibitor toxicity. Curr Oncol Rep. 2018;20:72. doi:10.1007/s11912-018-0718-6.
- Connolly C, Bambhania K, Naidoo J. Immune-related adverse events: a case-based approach. Front Oncol. 2019;9:530. doi:10.3389/fonc.2019.00530.
- Moher D, Liberati A, Tetzlaff J, Altman DG; PRISMA Group. Preferred reporting items for systematic reviews and meta-analyses: the PRISMA statement. PLoS Med. 2009;6(7):e1000097. doi:10.1371/journal.pmed.1000097.
- TrialTrove: pharmaceutical clinical trials intelligence data. https://pharmaintelligence.informa.com/products-and-services/data-and-analysis/trialtrove [accessed 2018 Oct 10].
- Ahamadi M, Freshwater T, Prohn M, Li CH, de Alwis DP, de Greef R, Elassaiss‐Schaap J, Kondic A, Stone JA. Model-based characterization of the pharmacokinetics of pembrolizumab: a humanized anti-PD-1 monoclonal antibody in advanced solid tumors. CPT Pharmacometrics Syst Pharmacol. 2017;6(1):49–57. doi:10.1002/psp4.12139.
- EMA557664, Assessment report for yervoy (ipilimumab) EMA/CHMP/557664/2011. http://www.ema.europa.eu/docs/en_GB/document_library/EPAR_-_Public_assessment_report/human/002213/WC500109302.pdf [accessed 2018 Sep 25].
- Bajaj G, Wang X, Agrawal S, Gupta M, Roy A, Feng Y. Model-based population pharmacokinetic analysis of nivolumab in patients with solid tumors. CPT Pharmacometrics Syst Pharmacol. 2017;6(1):58–66. doi:10.1002/psp4.12143.
- Baverel PG, Dubois VFS, Jin CY, Zheng Y, Song X, Jin X, Mukhopadhyay P, Gupta A, Dennis PA, Ben Y, et al. Population pharmacokinetics of durvalumab in cancer patients and association with longitudinal biomarkers of disease status. Clin Pharmacol Ther. 2018;103(4):631–642. doi:10.1002/cpt.982.
- BLA125514, Pharmacology review: keytruda (pembrolizumab), 2014. https://www.accessdata.fda.gov/drugsatfda_docs/nda/2014/125514Orig1s000PharmR.pdf [accessed 2018 Sep 25].
- BLA761034, Clinical pharmacology review: tecentriq (atezolizumab), 2016. https://www.accessdata.fda.gov/drugsatfda_docs/nda/2016/761034Orig1s000ClinPharmR.pdf [accessed 2018 Sep 25].
- BLA761049, Multidisciplinary review and evaluation: bavencio (avelumab), 2016. https://www.accessdata.fda.gov/drugsatfda_docs/nda/2017/761049Orig1s000MultidisciplineR.pdf [accessed 2018 Sep 25].
- BLA761069, Pharmacology review: imfinzi (durvalumab), 2017. https://www.accessdata.fda.gov/drugsatfda_docs/nda/2017/761069Orig1s000PharmR.pdf [accessed 2018 Sep 25].
- Stroh M, Winter H, Marchand M, Claret L, Eppler S, Ruppel J, Abidoye O, Teng SL, Lin WT, Dayog S, et al. Clinical pharmacokinetics and pharmacodynamics of atezolizumab in metastatic urothelial carcinoma. Clin Pharmacol Ther. 2017;102(2):305–312. doi:10.1002/cpt.587.
- Shah DK, Betts AM. Antibody biodistribution coefficients: inferring tissue concentrations of monoclonal antibodies based on the plasma concentrations in several preclinical species and human. MAbs. 2013;5(2):297–305. doi:10.4161/mabs.23684.
- Kang D, Wang E, Wang D, Amantea M, Hsyu P. Population pharmacokinetics (PK) of tremelimumab in patients (pts) with melanoma. J Clin Oncol. 2009;27:3048.
- Wang C, Thudium KB, Han M, Wang XT, Huang H, Feingersh D, Garcia C, Wu Y, Kuhne M, Srinivasan M, et al. In vitro characterization of the anti-PD-1 antibody nivolumab, BMS-936558, and in vivo toxicology in non-human primates. Cancer Immunol Res. 2014;2(9):846–856. doi:10.1158/2326-6066.CIR-14-0040.
- Sheng J, Srivastava S, Sanghavi K, Lu Z, Schmidt BJ, Bello A, Gupta M. Clinical pharmacology considerations for the development of immune checkpoint inhibitors. J Clin Pharmacol. 2017;57(Suppl 10):S26–42. doi:10.1002/jcph.990.
- Tremelimumab. Drugs R D. 2010;10(2):123–132. doi:10.2165/11584530-000000000-00000.
- Hamza TH, van Houwelingen HC, Stijnen T. The binomial distribution of meta-analysis was preferred to model within-study variability. J Clin Epidemiol. 2008;61(1):41–51. doi:10.1016/j.jclinepi.2007.03.016.
- Stijnen T, Hamza TH, Özdemir P. Random effects meta-analysis of event outcome in the framework of the generalized linear mixed model with applications in sparse data. Stat Med. 2010;29(29):3046–3067. doi:10.1002/sim.4040.
- Viechtbauer W. Conducting meta-analyses in R with the metafor package. J Stat Softw. 2010;36(3). doi:10.18637/jss.v036.i03.
- Boixader LV, Curtis KK, Wahl J, Kenny N, Moss KR. Changes in numbers of randomized (RCT) versus non-randomized (NRCT) clinical trials from 2004–2016: evidence of shifting cancer drug development pathway. J Clin Oncol. 2017;35(15 suppl):2543. doi:10.1200/JCO.2017.35.15_suppl.2543.
- Grossman Z, Paul WE. Dynamic tuning of lymphocytes: physiological basis, mechanisms, and function. Annu Rev Immunol. 2015;33:677–713. doi:10.1146/annurev-immunol-032712-100027.
- Hogquist KA, Jameson SC. The self-obsession of T cells: how TCR signaling thresholds affect fate ‘decisions’ and effector function. Nat Immunol. 2014;15(9):815–823. doi:10.1038/ni.2938.
- Sinclair NR, Anderson CC. Co-stimulation and co-inhibition: equal partners in regulation. Scand J Immunol. 1996;43(6):597–603. doi:10.1046/j.1365-3083.1996.d01-267.x.
- Bakacs T, Moss RW, Kleef R, Szasz MA, Anderson CC. Exploiting autoimmunity unleashed by low-dose immune checkpoint blockade to treat advanced cancer. Scand J Immunol. 2019;90(6):e12821. doi:10.1111/sji.12821.
- Renner A, Burotto M, Rojas C. Exploiting autoimmunity unleashed by low-dose immune checkpoint blockade to treat advanced cancer. immune checkpoint inhibitor dosing: can we go lower without compromising clinical efficacy? J Glob Oncol. 2019;5:1–5. doi:10.1200/JGO.19.00142.
- Chatterjee MS, Elassaiss-Schaap J, Lindauer A, Turner DC, Sostelly A, Freshwater T, Mayawala K, Ahamadi M, Stone JA, de Greef R, et al. Population pharmacokinetic/pharmacodynamic modeling of tumor size dynamics in pembrolizumab-treated advanced melanoma. CPT Pharmacometrics Syst Pharmacol. 2017;6(1):29–39. doi:10.1002/psp4.12140.
- Agrawal S, Feng Y, Roy A, Kollia G, Lestini B. Nivolumab dose selection: challenges, opportunities, and lessons learned for cancer immunotherapy. J Immunother Cancer. 2016;4:72. doi:10.1186/s40425-016-0177-2.
- Wolchok JD, Neyns B, Linette G, Negrier S, Lutzky J, Thomas L, Waterfield W, Schadendorf D, Smylie M, Guthrie Jr T, et al. Ipilimumab monotherapy in patients with pretreated advanced melanoma: a randomised, double-blind, multicentre, phase 2, dose-ranging study. Lancet Oncol. 2010;11(2):155–164. doi:10.1016/S1470-2045(09)70334-1.
- Madan RA, Mohebtash M, Arlen PM, Vergati M, Rauckhorst M, Steinberg SM, Tsang KY, Poole DJ, Parnes HL, Wright JJ, et al. Ipilimumab and a poxviral vaccine targeting prostate-specific antigen in metastatic castration-resistant prostate cancer: a phase 1 dose-escalation trial. Lancet Oncol. 2012;13(5):501–508. doi:10.1016/S1470-2045(12)70006-2.
- Camacho LH, Antonia S, Sosman J, Kirkwood JM, Gajewski TF, Redman B, Pavlov D, Bulanhagui C, Bozon VA, Gomez-Navarro J, et al. Phase I/II trial of tremelimumab in patients with metastatic melanoma. J Clin Oncol. 2009;27(7):1075–1081. doi:10.1200/JCO.2008.19.2435.
- Weber JS, Hamid O, Chasalow SD, Wu DY, Parker SM, Galbraith S, Gnjatic S, Berman D. Ipilimumab increases activated T cells and enhances humoral immunity in patients with advanced melanoma. J Immunother. 2012;35(1):89–97. doi:10.1097/CJI.0b013e31823aa41c.
- Comin-Anduix B, Lee Y, Jalil J, Algazi A, de la Rocha P, Camacho LH. Detailed analysis of immunologic effects of the cytotoxic T lymphocyte-associated antigen 4-blocking monoclonal antibody tremelimumab in peripheral blood of patients with melanoma. J Transl Med. 2008;1(6):22. doi:10.1186/1479-5876-6-22.
- Wei SC, Anang NAS, Sharma R, Andrews MC, Reuben A, Levine JH, Cogdill AP, Mancuso JJ, Wargo JA, Pe’er D, et al. Combination anti-CTLA-4 plus anti-PD-1 checkpoint blockade utilizes cellular mechanisms partially distinct from monotherapies. Proc Natl Acad Sci U S A. 2019;116(45):22699–22709. doi:10.1073/pnas.1821218116.
- Cha E, Klinger M, Hou Y, Cummings C, Ribas A, Faham M, Fong L. Improved survival with T cell clonotype stability after anti-CTLA-4 treatment in cancer patients. Sci Transl Med. 2014;6(238):238ra70. doi:10.1126/scitranslmed.3008211.
- Robert L, Tsoi J, Wang X, Emerson R, Homet B, Chodon T, Mok S, Huang RR, Cochran AJ, Comin-Anduix B, et al. CTLA4 blockade broadens the peripheral T-cell receptor repertoire. Clin Cancer Res. 2014;20(9):2424–2432. doi:10.1158/1078-0432.CCR-13-2648.
- Kvistborg P, Philips D, Kelderman S, Hageman L, Ottensmeier C, Joseph-Pietras D, Welters MJ, van der Burg S, Kapiteijn E, Michielin O,et al. Anti–CTLA-4 therapy broadens the melanoma-reactive CD8+ T cell response. Sci Transl Med. 2014;6(254):254ra128. doi:10.1126/scitranslmed.3008918
- Subudhi SK, Aparicio A, Gao J, Zurita AJ, Araujo JC, Logothetis CJ, Tahir SA, Korivi BR, Slack RS, Vence L, et al. Clonal expansion of CD8 T cells in the systemic circulation precedes development of ipilimumab-induced toxicities. Proc Natl Acad Sci U S A. 2016;113(42):11919–11924. doi:10.1073/pnas.1611421113.
- Wei SC, Duffy CR, Allison JP. Fundamental mechanisms of immune checkpoint blockade therapy. Cancer Discov. 2018;8(9):1069–1086. doi:10.1158/2159-8290.CD-18-0367.
- Gide TN, Quek C, Menzies AM, Tasker AT, Shang P, Holst J, Madore J, Lim SY, Velickovic R, Wongchenko M, et al. Distinct immune cell populations define response to anti-PD-1 monotherapy and anti-PD-1/anti-CTLA-4 combined therapy. Cancer Cell. 2019;35(2):238–255.e6. doi:10.1016/j.ccell.2019.01.003.
- Spitzer MH, Carmi Y, Reticker-Flynn NE, Kwek SS, Madhireddy D, Martins MM, Gherardini PF, Prestwood TR, Chabon J, Bendall SC, et al. Systemic immunity is required for effective cancer immunotherapy. Cell. 2017;168(3):487–502.e15. doi:10.1016/j.cell.2016.12.022.
- Yost KE, Satpathy AT, Wells DK, Qi Y, Wang C, Kageyama R, McNamara KL, Granja JM, Sarin KY, Brown RA, et al. Clonal replacement of tumor-specific T cells following PD-1 blockade. Nat Med. 2019;25(8):1251–1259. doi:10.1038/s41591-019-0522-3.
- Ellestad KK, Anderson CC. Two strikes and you’re out? The pathogenic interplay of coinhibitor deficiency and lymphopenia-induced proliferation. J Immun. 2017;198:2534–2541. doi:10.4049/jimmunol.1601884.
- Ellestad KK, Lin J, Boon L, Anderson CC. PD-1 controls tonic signaling and lymphopenia-induced proliferation of T lymphocytes. Front Immunol. 2017;8:1289. doi:10.3389/fimmu.2017.01289.
- Ellestad KK, Thangavelu G, Haile Y, Lin J, Boon L, Anderson CC. Prior to peripheral tolerance, newly generated CD4 T cells maintain dangerous autoimmune potential: fas- and perforin-independent autoimmunity controlled by programmed death-1. Front Immunol. 2018;9:12. doi:10.3389/fimmu.2018.00012.
- Verma R, Foster RE, Horgan K, Mounsey K, Nixon H, Smalle N, Hughes TA, Carter CR. Lymphocyte depletion and repopulation after chemotherapy for primary breast cancer. Breast Cancer Res. 2016;18(1):10. doi:10.1186/s13058-015-0669-x.
- Carlino MS, Sandhu S. Safety and efficacy implications of discontinuing combination ipilimumab and nivolumab in advanced melanoma. J Clin Oncol. 2017;35(34):3792–3793. doi:10.1200/JCO.2017.75.2055.
- Wherry EJ, Kurachi M. Molecular and cellular insights into T cell exhaustion. Nat Rev Immunol. 2015;15(8):486–499. doi:10.1038/nri3862.
- Langer CJ, Gadgeel SM, Borghaei H, Papadimitrakopoulou VA, Patnaik A, Powell SF, Gentzler RD, Martins RG, Stevenson JP, Jalal SI, et al. Carboplatin and pemetrexed with or without pembrolizumab for advanced, non-squamous non-small-cell lung cancer: a randomised, phase 2 cohort of the open-label KEYNOTE-021 study. Lancet Oncol. 2016;17(11):1497–1508. doi:10.1016/S1470-2045(16)30498-3.
- Govindan R, Szczesna A, Ahn MJ, Schneider CP, Gonzalez Mella PF, Barlesi F, Han B, Ganea DE, Von Pawel J, Vladimirov V, et al. Phase III trial of ipilimumab combined with paclitaxel and carboplatin in advanced squamous non–small-cell lung cancer. J Clin Oncol. 2017;35:3449–3457. doi:10.1200/JCO.2016.71.7629.
- Gandhi L, Rodríguez‑Abreu D, Gadgeel S, Esteban E, Felip E, De Angelis F, Domine M, Clingan P, Hochmair MJ, Powell SF, et al. Pembrolizumab plus chemotherapy in metastatic non–small-cell lung cancer. N Engl J Med. 2018;378(22):2078–2092. doi:10.1056/NEJMoa1801005.
- Zhou Y, Chen C, Zhang X, Fu S, Xue C, Ma Y, Fang W, Yang Y, Hou X, Huang Y, et al. Immune-checkpoint inhibitor plus chemotherapy versus conventional chemotherapy for first-line treatment in advanced non-small cell lung carcinoma: a systematic review and meta-analysis. J Immun Cancer. 2018;6(1):155. doi:10.1186/s40425-018-0477-9.
- Galluzzi L, Buqué A, Kepp O, Zitvogel L, Kroemer G. Immunological effects of conventional chemotherapy and targeted anticancer agents. Cancer Cell. 2015;28(6):690–714. doi:10.1016/j.ccell.2015.10.012.
- Xu C, Chen YP, Du XJ, Liu JQ, Huang CL, Chen L, Zhou GQ, Li WF, Mao YP, Hsu C, et al. Comparative safety of immune checkpoint inhibitors in cancer: systematic review and network meta-analysis. BMJ. 2018;363:k4226. doi:10.1136/bmj.k4226.
- Wang Y, Zhou S, Yang F, Qi X, Wang X, Guan X, Shen C, Duma N, Aguilera JV, Chintakuntlawar A, et al. Treatment-related adverse events of PD-1 and PD-L1 inhibitors in clinical trials: a systematic review and meta-analysis. JAMA Oncol. 2019;5(7):1008–1019. doi:10.1001/jamaoncol.2019.0393.
- El Osta B, Hu F, Sadek R, Chintalapally R, Tang SC. Not all immune-checkpoint inhibitors are created equal: meta-analysis and systematic review of immune-related adverse events in cancer trials. Crit Rev Oncol Hematol. 2017;119:1–12. doi:10.1016/j.critrevonc.2017.09.002.
- Hellmann MD, Paz-Ares L, Bernabe Caro R, Zurawski B, Kim SW, Carcereny Costa E, Park K, Alexandru A, Lupinacci L, de la Mora Jimenez E, et al. Nivolumab plus ipilimumab in advanced non-small-cell lung cancer. N Engl J Med. 2019 Nov 21;381(21):2020–2031. doi:10.1056/NEJMoa1910231.
- Yoo SH, Keam B, Kim M, Kim SH, Kim YJ, Kim TM, Kim DW, Lee JS, Heo DS. Low-dose nivolumab can be effective in non-small cell lung cancer: alternative option for financial toxicity. ESMO Open. 2018 Jul 25;3(5):e000332. doi:10.1136/esmoopen-2018-000332.
- Schwarze JK, Vandersleyen V, Awada G, Jansen Y, Seremet T, Neyns B. A sequential dual cohort phase II clinical trial on adjuvant low-dose nivolumab with or without low-dose ipilimumab as adjuvant therapy following the resection of melanoma macrometastases (MM). JCO. 2019;37(15_suppl):9585. doi:10.1200/JCO.2019.37.15_suppl.9585.
- Sen S, Hess KR, Hong DS, Naing A, Huang L, Meric-Bernstam F, Subbiah V. Impact of immune checkpoint inhibitor dose on toxicity, response rate, and survival: a pooled analysis of dose escalation phase 1 trials. JCO. 2018;36(15_suppl):3077. doi:10.1200/JCO.2018.36.15_suppl.3077.