ABSTRACT
Hodgkin lymphoma (HL) is a unique type of hematopoietic cancer that has few tumor cells but a massive infiltration of immune cells. Findings on how the cancerous Hodgkin and Reed-Sternberg (HRS) cells survive and evade immune surveillance have facilitated the development of novel immunotherapies for HL. Trogocytosis is a fast process of intercellular transfer of membrane patches, which can significantly affect immune responses. In this review, we summarize the current knowledge of how trogocytosis contributes to the suppression of immune responses in HL. We focus on the ectopic expression of CD137 on HRS cells, the cause of its expression, and its implication on developing novel therapies for HL. Further, we review data demonstrating that similar mechanisms apply to CD30, PD-L1 and CTLA-4.
Hodgkin lymphoma
Hodgkin lymphoma (HL) affects around 80,000 people worldwide each year, accounting for around 0.4% of all cancers.Citation1 HL is a very common cancer in young adolescents (15–19 years old), amounting to 12% of all cancers in this age group in the United States.Citation2 The standard diagnosis and classification of HL are based on the morphological and immunohistochemical identification of the Hodgkin and Reed-Sternberg (HRS) cells in excisional biopsies. HRS cells are large cells that can be mononuclear or multinucleate. Classical HL (cHL) makes up over 90% of all HL cases and is the focus of this review, while the other 10% represent the nodular lymphocyte predominant type of HL. Another unique feature of HL is that HRS cells only constitute around 1% of the tumor mass whereas the majority of the tumor is made up of infiltrating immune cells.Citation3,Citation4 Therefore, it is an interesting question how HRS cells evade immune surveillance in such an immune cell-rich environment. Based on current understanding, HRS cells express inhibitory ligands (e.g. PD-L1), reduce expression of immunogenic proteins (e.g. major histocompatibility complex class I (MHCI)), secrete immunosuppressive cytokines, chemokines, metabolites and extracellular vesicles to attract normal cells, and transform them into suppressive cells (e.g. regulatory T cells (Tregs) and Tumor associated macrophages (TAMs)).Citation5,Citation6 Their complex interactions within the HL microenvironment have been well summarized and discussed.Citation5–Citation8 This review will focus on the contribution of trogocytosis,Citation9,Citation10 also called transendocytosis,Citation11 to the establishment of the immunosuppressive microenvironment in HL.
HL used to be a more deadly disease in previous times. Thanks to irradiation therapy and combination chemotherapy, the 5-year survival rate is currently above 90%. Nowadays, minimizing the toxicity while preserving the efficacy of the therapy is a pivotal issue of HL treatment.Citation12 Developing novel therapeutic approaches for refractory/relapsed HL (r/r HL) has become a focus of HL treatment since 10–20% of patients suffer from this condition.Citation13 Immunotherapies have changed the strategy of r/r HL treatment significantly. Brentuximab vedotin (an anti-CD30-drug conjugate) and anti-PD-1 antibodies achieve good objective response rates (>50%) in r/r HL.Citation12,Citation14 Other immunotherapies, such as chimeric antigen receptor T (CAR-T) cells,Citation15–Citation18 are attracting intense research interest.Citation19
Trogocytosis
Trogocytosis is a fast intercellular transfer of membrane fragments along with the proteins inserted into or associated with them, which can happen within minutes. Although intercellular transfer of membranes had been observed earlier in prokaryotes, the term trogocytosis was only applied to describe these transfers in mammalian cells in 2002.Citation20 The proteins in the small membrane fragments pinched off from the donor cell are either displayed on the acceptor cell surface or are internalized.Citation9,Citation10 Therefore, trogocytosis helps explaining why certain cell type-specific proteins are present on other cells that do not express them.Citation21 For example, myelin basic protein-specific T cells, after being activated by allogenic antigen presenting cells (APCs), can present myelin basic protein and conalbumin antigens to other T cells in a second co-culture, indicating the transfer of membrane patches containing specific and irrelevant peptide-MHC class II (pMHCII) from the APC to the T cells.Citation22 Although the transfer of proteins is not specific, trogocytosis is mediated and facilitated by specific ligand-receptor interactions. The anti-MHC antibody (OX6) could block the transfer of pMHCII in aforementioned allogenic reactions.Citation22 Therefore, trogocytosis mediated by specific antigen-TCR interactions can aid in the discovery of novel epitopes for clinically employed TCR.Citation23
Consequences of trogocytic transfer of membrane patches are diverse depending on the functions of proteins embedded in these membrane patches. Although trogocytosis has also been reported between many nonimmune cells and even between tumor cells,Citation21,Citation24 trogocytosis has been most commonly observed among immune cells during close cell-cell contacts. This intercellular transfer of membrane patches and proteins contained within them, influences ongoing immune responses. During activation by APCs, ovalbumin-specific CD4+ helper T (Th) cells acquired pMHCI and costimulatory ligands along with pMHCII, and the ability to mimick APCs in stimulating ovalbumin-specific CD8+ T cell responses.Citation25,Citation26 Strikingly, mice receiving this APC-like Th cells as a vaccine, were completely protected from developing lung metastasis caused by tumor cells with this specific antigen.Citation25 However, trogocytosis can also suppress immune responses. During therapeutic antibody treatments for tumors, tumor cells can escape from antibody-dependent cellular phagocytosis and cytotoxicity by Fc receptor-mediated trogocytosis of antigen-antibody complexes.Citation27 HLA-G, an immunosuppressive molecule, has been reported to be transferred among tumor cells and immune cells, leading to a suppressive tumor environment and a dampened anti-tumor immunity.Citation28 Therefore, the effect of trogocytosis on immune responses needs to be analyzed on a case-by-case basis.Citation21 Below, we will summarize recent results on the effect of trogocytosis on HL.
Ectopic CD137 expression on HRS cells induced by Epstein-Barr virus suppresses immune responses via trogocytosis
CD137 (TNFRSF9, 4–1BB, ILA) is expressed on several types of immune cells, endothelial cells and some tumor cells.Citation29–Citation31 But the function of CD137 is best studied in activated T cells. The expression of CD137 on T cells is activation-dependent.Citation32 When crosslinked by CD137 ligand (CD137 L), CD137 transduces a co-stimulatory signal to T cells, polarizing T cells to Th1 and type 1 cytotoxic T cell responses,Citation33–Citation35 which are pivotal for anti-tumor immunotherapy. Because of their strong costimulatory effect, agonistic antibodies for CD137, i.e. urelumab and utomilumab, are being developed for cancer immunotherapy, and are currently being tested in clinical trials.Citation36 Moreover, the CD137 intracellular domain is incorporated as a costimulatory signaling domain in CARs.Citation18 CAR-T cells with the CD137 signaling domain displayed longer persistence than CD28-containing CAR-T cells in several cancers.Citation37 Furthermore, CD137 L expression on tumors, such as Ewing’s sarcoma and B cell lymphoma, increases tumor rejection.Citation38,Citation39 Therefore, the expression of CD137 for T cell activation is of great importance for tumor eradication.
To dampen anti-tumor immune responses, some tumors such as chronic lymphocytic leukemia, elevate the plasma level of soluble CD137 (sCD137) .Citation40,Citation41 HL patients do not have higher plasma levels of sCD137 at diagnosis, though sCD137 slightly decreases after HL remission.Citation42 Instead, HL uses another strategy to suppress CD137 L-CD137 interaction between APCs and T cells, i.e. trogocytosis. CD137 is expressed by HRS cells in 86% of HL cases as demonstrated by immunohistochemistry.Citation30,Citation43 Later studies found CD137 expression on HRS cells in less but still more than half of the HL tissues (57.4%, 60% and 65%).Citation44–Citation46 This ectopically expressed CD137 not only transduces an activation signal into HRS cells, but also dampens immune activation. When activated murine B and T cells interacted, a trogocytic transfer of CD137 from T to B cells and a downregulation of CD137L on B cells were observed.Citation47 Similarly, the ectopically expressed CD137 on HRS cell can bind CD137L and cause an internalization of CD137L on the HRS cells themselves as well as on surrounding APCs, resulting in decreased PBMC proliferation and IFNγ secretionCitation43 ().
Figure 1. Trogocytosis in HL contributes to immune suppression. (a). The EBV protein LMP-1 induces the ectopic expression of CD137 on HRS cells, which can bind CD137 L and downregulate CD137 L on adjacent APCs by trogocytosis, leading to decreased costimulation of T cells. CD137 can also signal into HRS cells and induce the secretion of IL-13 that deviates the tumoricidal Th1 response away to a Th2 response. (b). CD30 on HL upon binding to CD30 L triggers a pro-growth and pro-survival signal to HRS cells, which can be enhanced by the trogocytosis of the CD30-CD30 L complex into HRS cells. (c). PD-L1 and PD-L2 on HRS cells can transfer to neighboring TAMs through trogocytosis (unknown mechanism), enhancing the T cell inhibition via PD-L1/PD-L2 – PD-1 interactions.
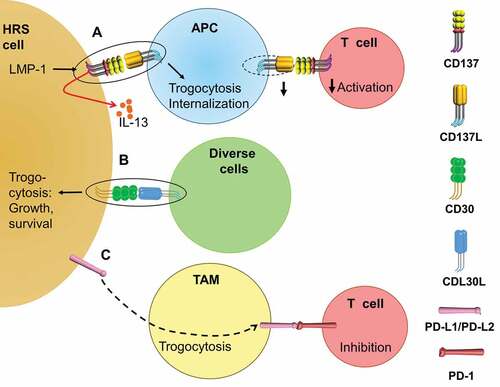
It should be noted that all the available functional studies of CD137 on HRS cells are based on HRS cell lines not primary HRS cells. However, that is unavoidable since primary HRS cells are not available. Their ex vivo enrichment is close to impossible due to their scarcity, and because HL is generally not treated by surgery but by chemo and radiation therapy. Simultaneous staining of CD137 and CD137L in primary HL tissues to study the correlation between CD137L expression levels and the proximity to CD137+ HRS cells would help translating cell line results to the in vivo situation of HL.
Moreover, ectopic CD137 decreases immune activation by a cell contact-independent manner. When treated by CD137L recombinant protein, HRS cells proliferate and secrete IL-13. IL-13 is a Th2 cytokine that can inhibit Th1 responses. Indeed, the conditioned medium from a co-culture of CD137+ HRS cells and monocytic THP-1 cells suppresses IFNγ secretion by PBMCs in an IL-13-dependent mannerCitation45 (). Supporting the findings from HRS cell lines, the immunohistochemical staining in primary HL tissue detected IL-13+ CD137+ HRS cells in 58% of HL cases.Citation45
HRS cells are in most cases derived from B cells, which do not readily express CD137. Nevertheless, CD137 expression on B cells can be induced by B cell receptor and CD40 signaling.Citation48,Citation49 Latent membrane protein 1 (LMP1) is an Epstein-Barr virus (EBV) protein that is thought to mimic CD40 signaling, and can induce NF-κB and c-Jun N-terminal kinase activation.Citation50–Citation52 Interestingly, Yoshimori et al. found that EBV induces CD137 expression on T and NK cells in lymphoproliferative disorders.Citation53 EBV is associated with HL pathogenesis. EBV proteins can be detected in most HL cases, especially in developing countries.Citation12 Finally, our group found 96% of the CD137+ HRS cells being positive for LMP1, and 72% LMP1+ HRS cells being positive for CD137,Citation46 and demonstrated that LMP1 can induce ectopic CD137 expression on HRS cells via the PI3K-Akt-mTOR pathway.Citation46,Citation54 Recently, three groups reported that CD137 deficiencies in humans lead to impaired lymphocytic responses and EBV-induced lymphoproliferation or lymphomagenesis.Citation55–Citation57 It seems that CD137 costimulation of T cells is crucial for the control of EBV, and that EBV can hijack the ectopic CD137 expression to prevent CD137 costimulation of T cells, and thereby evade immune rejection.Citation58
HL is rich in infiltrating immune cells, with Tregs being among them. It has been reported that Tregs express CD137, and that CD137 signaling activates Tregs.Citation59–Citation62 Moreover, Tregs in non-small-cell lung cancer express CD137, and high CD137 expression correlates with poor survival.Citation63 In HL, CD137 is expressed not only on HRS cells but also on surrounding immune cells.Citation43,Citation46 It remains to be determined what these cells are, and whether Tregs in HL express CD137 and dampen T cell activation by trogocytosis. Furthermore, EBV positivity is associated with a higher degree of Treg infiltration in HL.Citation64 It would be interesting to know if EBV can also induce CD137 expression on infiltrated Tregs in HL. In conclusion, ectopic CD137 expression on HRS cells, induced by EBV, reduces CD137L levels on APCs by trogocytosis, and signals into HRS cells leading to the secretion of IL-13, that weakens a cellular anti-HL immune responses by deviating immunity toward a Th2 response ().
Table 1. Main findings on the induction of CD137 in HRS cells, and the role of CD137 in HL pathogenesis.
Trogocytosis of CD30, PD-L1 and CTLA-4 aids HL in escaping immune surveillance
CD30 (TNFRSF8) is strongly expressed on HRS cells in cHL. The soluble form of CD30 is also highly elevated in cHL plasma, suggesting a diagnostic potential for cHL.Citation42 When crosslinked by CD30 ligand (CD30L), CD30 can recruit TNFR-associated factors (TRAF) and TRAF-binding proteins, leading to NF-κB and mitogen-activated protein kinase activation.Citation65 Although controversial results on the responsiveness of HRS cell lines to CD30 stimulation exist,Citation65 several groups reported the contribution of CD30 to the constitutive NF-κB activation and advantages in growth and survival for HRS cells.Citation66–Citation68 Besides the conventional ligand-receptor interaction between CD30L and CD30, the CD30 L-CD30 complex can also be internalized by HRS cells via trogocytosis (). The internalized CD30 L-CD30 seems to be important for CD30 signaling since Latrunculin A, an actin polymerization inhibitor, significantly suppressed the calcium influx.Citation69
Programmed cell death protein 1 (PD-1) is a well-established checkpoint molecule expressed on T cells. Its ligation by its ligands, PD-L1 and PD-L2, triggers strong inhibitory signals into T cells, inducing T cell apoptosis or anergy. PD-L1 and PD-L2 are overexpressed on HRS cells by the amplification of the 9p24.1 locus, by activator protein 1 signaling and due to EBV infection, contributing to the immunosuppressive microenvironment in HL.Citation70–Citation72 Moreover, TAMs surrounding HRS cells also express high levels of PD-L1 and PD-L2.Citation73 The overexpression of PD-L1 and PD-L2, and the high abundance of infiltrating TAMs have been shown to correlate with a poor prognosis of HL.Citation72,Citation74 Not surprisingly, anti-PD1 therapies have achieved good response rates in r/r cHL patients. PD-L1/PD-L2 expression may endow HRS cells not only to deliver inhibitory signals into T cells. Gary et al. demonstrated that PD-L1 transferred to CD8+ T cells via trogocytosis is able to further crosslink PD-1, and to induce apoptosis in surrounding T cells.Citation75 In HL, PD-L1+ HRS cells and TAMs are in close contact to T cells, which are enriched in PD-1 expression.Citation73 It is possible that those PD-1+ T cells that are in closer contact with PD-L1+ HRS cells or TAMs gain surface expression of PD-L1 by trogocytosis, and inhibit newly infiltrating PD-1+ T cells or other PD1+ T cells that are further away from PD-L1+ HRS cells or TAMs. In support of this, more PD-L1+ than PD-L1− TAMs are found in close proximity to PD-L1+ HRS cells.Citation73 Interestingly, Kawashima et al. discovered that monocytes and TAMs in close contact with HRS cells gain PD-L1 and PD-L2 expression via trogocytosis.Citation76 Whether this trogocytic transfer of PD-L1 to surrounding TAMs or myeloid-derived suppressor cells also occurs in other types of tumors remains to be investigated ().
Cytotoxic T lymphocyte-associated antigen 4 (CTLA-4 or CD152) is another well-known checkpoint molecule expressed on T cells, especially on Tregs. Compared to PD-1, CTLA-4 expression is more restricted to activated T cells and Tregs, and inhibits early T cell activation. CTLA-4 has a higher affinity to CD80 and CD86 than CD28 does, limiting the co-stimulatory signals T cells receive through CD28. In addition, CTLA-4 has also been shown to transendocytose CD80 and CD86, leading to CD80 and CD86 depletion on APCs.Citation77 Following up on the discovery of CTLA-4 transendocytosis, Gu et al. reported that induced Tregs deplete CD80 and CD86 on APCs via trogocytosis in a CTLA-4 or CD28-independent manner.Citation78 It would be most interesting to learn the underlying mechanism of action. It also remains to be determined if natural Tregs, especially Tregs in HL, can deplete CD80 and CD86 by trogocytosis. Clinical trials treating HL with antagonistic anti-CTLA-4 antibodies are very limited,Citation79,Citation80 partially because of the more severe adverse effects compared to anti-PD-1 or anti-PD-L1 therapies. More clinical trial data will surely aid our understanding of how significant trogocytosis mediated by CTLA-4 is for maintaining the immunosuppressive microenvironment in HL.
In summary, evidence is emerging that CD30, PD-L1 and CTLA-4 are being transferred by trogocytosis, and may bestow HRS cells with growth advantages and facilitate immune evasion of HL.
Perspective
The cancerous HRS cells manipulate the surrounding immune cells and nonimmune cells to create an immunosuppressive microenvironment. Reversing this immunosuppressive microenvironment supports the eradication of HL, as exemplified by the success of anti-PD1 antibodies for the treatment of HL.Citation81 Trogocytosis, a common phenomenon during immune cell interactions, is hijacked by HRS cells to downregulate co-stimulatory molecules on APCs, leading to dampened anti-tumor T cell responses. Trogocytic transfer of ectopically expressed CD137 on HRS cells allows the downregulation of the immunostimulatory CD137L, and thereby helps to evade immune surveillance. The fact that CD137-deficient patients develop EBV-associated pathologies further confirms the importance of CD137-mediated T cell costimulation for immune surveillance.Citation55–Citation57 Therefore, it is important that agonistic anti-CD137 antibodies used for cancer immunotherapy directly costimulate T cells via CD137, bypassing the need of interaction of T cells with CD137L on APCs. It is worth investigating whether CD137 agonistic antibodies show efficacy in curing HL, and whether they synergize with anti-PD1 or anti-PD-L1 antibodies in treating r/r HL.
Alternatively, a CD30 and CD137-bispecific antibody could be a novel therapeutic approach for HL since CD30 and CD137 are both highly expressed on HRS cells but not present on healthy cells at the same time.Citation83 Such a bispecific blocking antibody could not only inhibit CD30 and CD137 signaling into HRS cells, and cause antibody-dependent cellular cytotoxicity/phagocytosis (ADCC/ADCP) but also preserve CD137 L on APCs for T cell costimulation. When CD30 is targeted by brentuximab vedotin, a FDA-approved antibody-drug conjugate for cHL,Citation84 the transfer of CD30 to surrounding tissues by trogocytosis or extracellular vesicles may lead to an expanded CD30 sink and increased tissue damages.Citation85 Dual targeting of CD30 and CD137 could minimize such risks. Nevertheless, HRS cells might still evade CD30-targeting therapies by FcR-mediated extraction of antigen-antibody complexes from HRS cells via trogocytosis, leading to a reduced antigen density on HRS cells, and therefore a decreased ADCC/ADCP, as has been found for anti-CD20 therapies.Citation27,Citation86,Citation87 Orthogonal binding partners-empowered drug-free macromolecular therapeutics could be one strategy to eliminate FcR-mediated trogocytosis and at the same time induce target cell death.Citation88
Besides CD137, CD40 (TNFRSF5) may also contribute to the suppressive microenvironment in HL via trogocytosis since (1) CD40-CD40 L interaction potently activates anti-tumor immune responses, (2) CD40 is highly expressed on HRS cells, and (3) trogocytosis has been reported for the CD40-CD40 L interaction during B – T cell contact.Citation89 Moreover, an antagonistic anti-CD40 antibody has shown modest responses in HL patients.Citation90 Since PD-1 and PD-L1 are a receptor/ligand pair, we expect that antagonistic anti-PD-1 antibodies will generate a similar outcome as anti-PD-L1 antibodies in terms of inhibiting trogocytosis. Nevertheless, whether the trogocytic transfer of suppressive proteins or trogocytic depletion of co-stimulatory molecules in HL can be targeted for immunotherapies, and whether they play a role in other tumors warrant more research.
Disclosure of potential conflicts of interest
The authors declare no potential conflicts of interest.
Additional information
Funding
References
- Bray F, Ferlay J, Soerjomataram I, Siegel RL, Torre LA, Jemal A. Global cancer statistics 2018: GLOBOCAN estimates of incidence and mortality worldwide for 36 cancers in 185 countries. CA. 2018;68:394–7.
- Siegel RL, Miller KD, Jemal A. Cancer statistics, 2019. CA Cancer J Clin. 2019;69:7–34.
- Kuppers R, Hansmann ML. The Hodgkin and Reed/Sternberg cell. Int J Biochem Cell Biol. 2005;37:511–517.
- Aldinucci D, Gloghini A, Pinto A, De Filippi R, Carbone A. The classical Hodgkin’s lymphoma microenvironment and its role in promoting tumour growth and immune escape. The J Pathol. 2010;221:248–263.
- Aldinucci D, Borghese C, Casagrande N. Formation of the immunosuppressive microenvironment of classic hodgkin lymphoma and therapeutic approaches to counter it. int j mol sci. 2019;20:2416.
- Calabretta E, d’Amore F, Carlo-Stella C. Immune and inflammatory cells of the tumor microenvironment represent novel therapeutic targets in classical hodgkin lymphoma. Int J Mol Sci. 2019;20:5503.
- Aldinucci D, Celegato M, Casagrande N. Microenvironmental interactions in classical Hodgkin lymphoma and their role in promoting tumor growth, immune escape and drug resistance. Cancer Lett. 2016;380:243–252.
- Cirillo M, Reinke S, Klapper W, Borchmann S. The translational science of hodgkin lymphoma. Br J Haematol. 2019;184:30–44.
- Dance A. Core Concept: cells nibble one another via the under-appreciated process of trogocytosis. Proc Nat Acad Sci. 2019;116:17608–17610.
- Bettadapur A, Miller HW, Ralston KS. B iting off what can be chewed: trogocytosis in health, infection and disease. Infect Immunity. 2020.
- Soskic B, Qureshi OS, Hou T, Sansom DM. A transendocytosis perspective on the CD28/CTLA-4 pathway. Adv Immunol. 2014;124:95–136.
- Shanbhag S, Ambinder RF. Hodgkin lymphoma: A review and update on recent progress. CA. 2018;68:116–132.
- von Tresckow B, Moskowitz CH. Treatment of relapsed and refractory Hodgkin Lymphoma. Semin Hematol. 2016;53:180–185.
- Renner C, Stenner F. Cancer immunotherapy and the immune response in hodgkin lymphoma. Front Oncol. 2018;8:193.
- Wang D, Zeng C, Xu B, Xu JH, Wang J, Jiang LJ, Wang QX, Li CR, Wang N, Huang L, et al. Anti-CD30 chimeric antigen receptor T cell therapy for relapsed/refractory CD30+ lymphoma patients. Blood Cancer J. 2020;10:8.
- Wang CM, Wu ZQ, Wang Y, Guo YL, Dai HR, Wang XH, Li X, Zhang YJ, Zhang WY, Chen MX, et al. Autologous T cells expressing CD30 chimeric antigen receptors for relapsed or refractory hodgkin lymphoma: an open-label phase I trial. Clin Cancer Res. 2017;23:1156–1166.
- Ramos CA, Ballard B, Zhang H, Dakhova O, Gee AP, Mei Z, Bilgi M, Wu MF, Liu H, Grilley B, et al. Clinical and immunological responses after CD30-specific chimeric antigen receptor-redirected lymphocytes. J Clin Invest. 2017;127:3462–3471.
- Campana D, Schwarz H, Imai C. 4-1BB chimeric antigen receptors. Cancer J. 2014;20:134–140.
- Mottok A, Steidl C. Biology of classical Hodgkin lymphoma: implications for prognosis and novel therapies. Blood. 2018;131:1654–1665.
- Hudrisier D, Joly E. Plasma membrane nibbling: all lymphocytes do it, but why. ELSO Gaz. 2002;9:1–5.
- Ahmed KA, Munegowda MA, Xie Y, Xiang J. Intercellular trogocytosis plays an important role in modulation of immune responses. Cell Mol Immunol. 2008;5:261–269.
- Arnold PY, Davidian DK, Mannie MD. Antigen presentation by T cells: T cell receptor ligation promotes antigen acquisition from professional antigen-presenting cells. Eur J Immunol. 1997;27:3198–3205.
- Li G, Bethune MT, Wong S, Joglekar AV, Leonard MT, Wang JK, Kim JT, Cheng D, Peng S, Zaretsky JM, et al. T cell antigen discovery via trogocytosis. Nat Meth. 2019;16:183–190.
- LeMaoult J, Caumartin J, Daouya M, Switala M, Rebmann V, Arnulf B, Carosella ED. Trogocytic intercellular membrane exchanges among hematological tumors. J Hematol Oncol. 2015;8:24.
- Xiang J, Huang H, Liu Y. A new dynamic model of CD8+ T effector cell responses via CD4+ T helper-antigen-presenting cells. J Immunol. 2005;174:7497–7505.
- He T, Zong S, Wu X, Wei Y, Xiang J. CD4+ T cell acquisition of the bystander pMHC I colocalizing in the same immunological synapse comprising pMHC II and costimulatory CD40, CD54, CD80, OX40L, and 41BBL. Biochemi Biophys Res Com. 2007;362:822–828.
- Taylor RP, Lindorfer MA. Fcγ-receptor–mediated trogocytosis impacts mAb-based therapies: historical precedence and recent developments. Blood. 2015;125:762–766.
- Lin A, Yan W-H. Intercellular transfer of HLA-G: its potential in cancer immunology. Clin Transl Immunol. 2019;8:e1077.
- Drenkard D, Becke FM, Langstein J, Spruss T, Kunz-Schughart LA, Tan TE, Lim YC, Schwarz H. CD137 is expressed on blood vessel walls at sites of inflammation and enhances monocyte migratory activity. Faseb J. 2007;21:456–463.
- Anderson MW, Zhao S, Freud AG, Czerwinski DK, Kohrt H, Alizadeh AA, Houot R, Azambuja D, Biasoli I, Morais JC, et al. CD137 is expressed in follicular dendritic cell tumors and in classical Hodgkin and T-cell lymphomas: diagnostic and therapeutic implications. Am J Pathol. 2012;181:795–803.
- Broll K, Richter G, Pauly S, Hofstaedter F, Schwarz H. CD137 expression in tumor vessel walls. High correlation with malignant tumors. Am J Clin Pathol. 2001;115:543–549.
- Schwarz H, Valbracht J, Tuckwell J, von Kempis J, Lotz M. ILA, the human 4-1BB homologue, is inducible in lymphoid and other cell lineages. Blood. 1995;85:1043–1052.
- Melero I, Shuford WW, Newby SA, Aruffo A, Ledbetter JA, Hellstrom KE, Mittler RS, Chen L. Monoclonal antibodies against the 4-1BB T-cell activation molecule eradicate established tumors. Nat Med. 1997;3:682–685.
- Dharmadhikari B, Wu M, Abdullah NS, Rajendran S, Ishak ND, Nickles E, Harfuddin Z, Schwarz H. CD137 and CD137L signals are main drivers of type 1, cell-mediated immune responses. Oncoimmunol. 2016;5:e1113367.
- Thum E, Shao Z, Schwarz H. CD137, implications in immunity and potential for therapy. Front Biosci. 2009;14:4173–4188.
- Chu D-T, Bac ND, Nguyen K-H, Tien NLB, Thanh VV, Nga VT, Ngoc VTN, Anh Dao DT, Hoan LN, Hung NP, et al. An update on anti-CD137 antibodies in immunotherapies for cancer. Int J Mol Sci. 2019;20:1822.
- Weinkove R, George P, Dasyam N, McLellan AD. Selecting costimulatory domains for chimeric antigen receptors: functional and clinical considerations. Clin Transl Immunol. 2019;8:e1049–e.
- Zhang H, Merchant MS, Chua KS, Khanna C, Helman LJ, Telford B, Ward Y, Summers J, Toretsky J, Thomas EK, et al. Tumor expression of 4-1BB ligand sustains tumor lytic T cells. Cancer Biol Ther. 2003;2:579–586.
- Guinn BA, DeBenedette MA, Watts TH, Berinstein NL. 4-1BBL cooperates with B7-1 and B7-2 in converting a B cell lymphoma cell line into a long-lasting antitumor vaccine. J Immunol. 1999;162:5003–5010.
- Labiano S, Palazon A, Bolanos E, Azpilikueta A, Sanchez-Paulete AR, Morales-Kastresana A, Quetglas JI, Perez-Gracia JL, Gurpide A, Rodriguez-Ruiz M, et al. Hypoxia-induced soluble CD137 in malignant cells blocks CD137L-costimulation as an immune escape mechanism. Oncoimmunol. 2016;5:e1062967.
- Furtner M, Straub RH, Kruger S, Schwarz H. Levels of soluble CD137 are enhanced in sera of leukemia and lymphoma patients and are strongly associated with chronic lymphocytic leukemia. Leukemia. 2005;19:883–885.
- Zeng Q, Gupta A, Xin L, Poon M, Schwarz H. plasma factors for the differentiation of hodgkin’s lymphoma and diffused large B cell lymphoma and for monitoring remission. J Hematol. 2019;8:47–54.
- Ho WT, Pang WL, Chong SM, Castella A, Al-Salam S, Tan TE, Moh MC, Koh LK, Gan SU, Cheng CK, et al. Expression of CD137 on hodgkin and reed-sternberg cells inhibits T-cell activation by eliminating CD137 ligand expression. Cancer Res. 2013;73:652–661.
- Shi YF, Gao ZF, Liu CL, Huang X, Song YQ, Zhang C, Lin DM, Zhou LX, Zhao M, Lai YM, et al. Expression of CD137 in tumor cells of Hodgkin lymphoma from Northern China and its application in pathological differential diagnosis. Zhonghua Xue Ye Xue Za Zhi. 2016;37:484–490.
- Rajendran S, Ho WT, Schwarz H. CD137 signaling in Hodgkin and Reed-Sternberg cell lines induces IL-13 secretion, immune deviation and enhanced growth. Oncoimmunol. 2016;5:e1160188.
- Aravinth SP, Rajendran S, Li Y, Wu M, Yi Wong AH, Schwarz H. Epstein-Barr virus-encoded LMP1 induces ectopic CD137 expression on Hodgkin and Reed-Sternberg cells via the PI3K-AKT-mTOR pathway. Leuk Lymphoma. 2019;60:2697–2704.
- Shao Z, Harfuddin Z, Pang WL, Nickles E, Koh LK, Schwarz H. Trogocytic CD137 transfer causes an internalization of CD137 ligand on murine APCs leading to reduced T cell costimulation. J Jeukoc Biol. 2015;97:909–919.
- Zhang X, Voskens CJ, Sallin M, Maniar A, Montes CL, Zhang Y, Lin W, Li G, Burch E, Tan M, et al. CD137 promotes proliferation and survival of human B cells. J Immunol. 2010;184:787–795.
- Nakaima Y, Watanabe K, Koyama T, Miura O, Fukuda T. CD137 Is Induced by the CD40 signal on chronic lymphocytic leukemia b cells and transduces the survival signal via NF-κB activation. PLoS One. 2013;8:e64425.
- Izumi KM, McFarland EC, Ting AT, Riley EA, Seed B, Kieff ED. The epstein-barr virus oncoprotein latent membrane protein 1 engages the tumor necrosis factor receptor-associated proteins TRADD and receptor-interacting protein (RIP) but does not induce apoptosis or require RIP for NF-κB activation. Mol Cell Biol. 1999;19:5759–5767.
- Kaykas A, Worringer K, Sugden B. CD40 and LMP-1 both signal from lipid rafts but LMP-1 assembles a distinct, more efficient signaling complex. Embo J. 2001;20:2641–2654.
- Lam N, Sugden B. CD40 and its viral mimic, LMP1: similar means to different ends. Cell Signal. 2003;15:9–16.
- Yoshimori M, Imadome K, Komatsu H, Wang L, Saitoh Y, Yamaoka S, Fukuda T, Kurata M, Koyama T, Shimizu N, et al. CD137 expression is induced by Epstein-Barr virus infection through LMP1 in T or NK cells and mediates survival promoting signals. PLoS One. 2014;9:e112564.
- Wu M, Wong HY, Lin JL, Moliner A, Schwarz H. Induction of CD137 expression by viral genes reduces T cell costimulation. J Cell Physiol. 2019;234:21076–21088.
- Somekh I, Thian M, Medgyesi D, Gülez N, Magg T, Gallón Duque A, Stauber T, Lev A, Genel F, Unal E, et al. CD137 deficiency causes immune dysregulation with predisposition to lymphomagenesis. Blood. 2019;134:1510–1516.
- Alosaimi MF, Hoenig M, Jaber F, Platt CD, Jones J, Wallace J, Debatin K-M, Schulz A, Jacobsen E, Möller P, et al. Immunodeficiency and EBV-induced lymphoproliferation caused by 4-1BB deficiency. J Allergy Clin Immunol. 2019;144:574–83.e5.
- Rodriguez R, Fournier B, Cordeiro DJ, Winter S, Izawa K, Martin E, Boutboul D, Lenoir C, Fraitag S, Kracker S, et al. Concomitant PIK3CD and TNFRSF9 deficiencies cause chronic active Epstein-Barr virus infection of T cells. J Exp Med. 2019;216:2800–2818.
- Luu K, Nickles E, Schwarz H. Destroy, what destroys you. Oncoimmunology. 2020;9:1685301.
- Zheng G, Wang B, Chen A. The 4-1BB Costimulation Augments the Proliferation of CD4+ CD25+ Regulatory T Cells. J Immunol. 2004;173:2428–2434.
- Nowak A, Lock D, Bacher P, Hohnstein T, Vogt K, Gottfreund J, Giehr P, Polansky JK, Sawitzki B, Kaiser A, et al. CD137+CD154- Expression As a Regulatory T Cell (Treg)-Specific Activation Signature for Identification and Sorting of Stable Human Tregs from In Vitro Expansion Cultures. Front Immunol. 2018;9:199.
- Tsai YG, Yang KD, Wen YS, Hung CH, Chien JW, Lin CY. Allergen-specific immunotherapy enhances CD8(+) CD25(+) CD137(+) regulatory T cells and decreases nasal nitric oxide. Ped Allergy Immunol. 2019;30:531–539.
- Kao JK, Hsue YT, Lin CY. Role of new population of peripheral CD11c(+)CD8(+) T cells and CD4(+)CD25(+) regulatory T cells during acute and remission stages in rheumatoid arthritis patients. J Microbiol Immunol Infect. 2007;40:419–427.
- Guo X, Zhang Y, Zheng L, Zheng C, Song J, Zhang Q, Kang B, Liu Z, Jin L, Xing R, et al. Global characterization of T cells in non-small-cell lung cancer by single-cell sequencing. Nat Med. 2018;24:978–985.
- Pavlovic A, Glavina Durdov M, Capkun V, Jakelic Pitesa J, Bozic Sakic M. Classical hodgkin lymphoma with positive epstein-barr virus status is associated with more FOXP3 regulatory T cells. Med Sci Mon. 2016;22:2340–2346.
- van der Weyden CA, Pileri SA, Feldman AL, Whisstock J, Prince HM. Understanding CD30 biology and therapeutic targeting: a historical perspective providing insight into future directions. Blood Cancer J. 2017;7:e603–e.
- Horie R, Watanabe T, Morishita Y, Ito K, Ishida T, Kanegae Y, Saito I, Higashihara M, Mori S, Kadin ME, et al. Ligand-independent signaling by overexpressed CD30 drives NF-kappaB activation in Hodgkin-reed-sternberg cells. Oncogene. 2002;21:2493–2503.
- Guo F, Sun A, Wang W, He J, Hou J, Zhou P, Chen Z. TRAF1 is involved in the classical NF-κB activation and CD30-induced alternative activity in Hodgkin’s lymphoma cells. Mol Immunol. 2009;46:2441–2448.
- Gruss HJ, Boiani N, Williams DE, Armitage RJ, Smith CA, Goodwin RG. Pleiotropic effects of the CD30 ligand on CD30-expressing cells and lymphoma cell lines. Blood. 1994;83:2045–2056.
- Nakashima M, Watanabe M, Uchimaru K, Horie R. Trogocytosis of ligand–receptor complex and its intracellular transport in CD30 signalling. Biol Cell. 2018;110:109–124.
- Green MR, Rodig S, Juszczynski P, Ouyang J, Sinha P, O’Donnell E, Neuberg D, Shipp MA. Constitutive AP-1 activity and EBV infection induce PD-L1 in Hodgkin lymphomas and posttransplant lymphoproliferative disorders: implications for targeted therapy. Clin Cancer Res. 2012;18:1611–1618.
- Yamamoto R, Nishikori M, Kitawaki T, Sakai T, Hishizawa M, Tashima M, Kondo T, Ohmori K, Kurata M, Hayashi T, et al. PD-1-PD-1 ligand interaction contributes to immunosuppressive microenvironment of Hodgkin lymphoma. Blood. 2008;111:3220–3224.
- Roemer MG, Advani RH, Ligon AH, Natkunam Y, Redd RA, Homer H, Connelly CF, Sun HH, Daadi SE, Freeman GJ, et al. PD-L1 and PD-L2 genetic alterations define classical hodgkin lymphoma and predict outcome. J Clin Oncol. 2016;34:2690–2697.
- Carey CD, Gusenleitner D, Lipschitz M, Roemer MGM, Stack EC, Gjini E, Hu X, Redd R, Freeman GJ, Neuberg D, et al. Topological analysis reveals a PD-L1-associated microenvironmental niche for reed-sternberg cells in hodgkin lymphoma. Blood. 2017;130:2420–2430.
- Steidl C, Lee T, Shah SP, Farinha P, Han G, Nayar T, Delaney A, Jones SJ, Iqbal J, Weisenburger DD, et al. Tumor-associated macrophages and survival in classic hodgkin’s lymphoma. New Engl J Med. 2010;362:875–885.
- Gary R, Voelkl S, Palmisano R, Ullrich E, Bosch JJ, Mackensen A. Antigen-Specific Transfer of Functional Programmed Death Ligand 1 from Human APCs onto CD8+ T Cells via Trogocytosis. J Immunol. 2012;188:744–752.
- Kawashima M, Carreras J, Higuchi H, Kotaki R, Hoshina T, Okuyama K, Suzuki N, Kakizaki M, Miyatake Y, Ando K, et al. PD-L1/L2 protein levels rapidly increase on monocytes via trogocytosis from tumor cells in classical Hodgkin lymphoma. Leukemia. 2020. doi:10.1038/s41375-020-0737-9
- Qureshi OS, Zheng Y, Nakamura K, Attridge K, Manzotti C, Schmidt EM, Baker J, Jeffery LE, Kaur S, Briggs Z, et al. Trans-endocytosis of CD80 and CD86: a molecular basis for the cell-extrinsic function of CTLA-4. Science (New York, NY). 2011;332:600–603.
- Gu P, Fang Gao J, D’Souza CA, Kowalczyk A, Chou K-Y, Zhang L. Trogocytosis of CD80 and CD86 by induced regulatory T cells. Cell Mol Immunol. 2012;9:136–146.
- Bashey A, Medina B, Corringham S, Pasek M, Carrier E, Vrooman L, Lowy I, Solomon SR, Morris LE, Holland HK, et al. CTLA4 blockade with ipilimumab to treat relapse of malignancy after allogeneic hematopoietic cell transplantation. Blood. 2009;113:1581–1588.
- Davids MS, Kim HT, Bachireddy P, Costello C, Liguori R, Savell A, Lukez AP, Avigan D, Chen Y-B, McSweeney P, et al. Ipilimumab for Patients with Relapse after Allogeneic Transplantation. New Engl J Med. 2016;375:143–153.
- Zhou H, Fu X, Li Q, Niu T. Safety and efficacy of anti-PD-1 monoclonal antibodies in patients with relapsed or refractory lymphoma: a meta-analysis of prospective clinic trails. Front Pharmacol. 2019;10:387.
- Rodriguez R, Fournier B, Cordeiro DJ, Winter S, Izawa K, Martin E, Boutboul D, Lenoir C, Fraitag S, Kracker S, et al. Concomitant PIK3CD and TNFRSF9 deficiencies cause chronic active Epstein-Barr virus infection of T cells. J Exp Med. 2019;216:2800–2818.
- Rajendran S, Li Y, Ngoh E, Wong HY, Cheng MS, Wang CI, Schwarz H. Development of a Bispecific Antibody Targeting CD30 and CD137 on Hodgkin and Reed-Sternberg Cells. Front Oncol. 2019;9:945.
- Bhatt G, Maddocks K, Christian B. CD30 and CD30-targeted therapies in hodgkin lymphomA AND Other B cell Lymphomas. Cur Hematol Malign Rep. 2016;11:480–491.
- Hansen HP, Trad A, Dams M, Zigrino P, Moss M, Tator M, Schön G, Grenzi PC, Bachurski D, Aquino B, et al. CD30 on extracellular vesicles from malignant Hodgkin cells supports damaging of CD30 ligand-expressing bystander cells with Brentuximab-Vedotin, in vitro. Oncotarget. 2016;7:30523–30535.
- Lim SH, Vaughan AT, Ashton-Key M, Williams EL, Dixon SV, Chan HTC, Beers SA, French RR, Cox KL, Davies AJ, et al. Fc gamma receptor IIb on target B cells promotes rituximab internalization and reduces clinical efficacy. Blood. 2011;118:2530–2540.
- Beum PV, Kennedy AD, Williams ME, Lindorfer MA, Taylor RP. The Shaving Reaction: rituximab/CD20 complexes are removed from mantle cell lymphoma and chronic lymphocytic leukemia cells by THP-1 monocytes. J Immunol. 2006;176:2600–2609.
- Yang J, Li L, Kopeček J. Biorecognition: A key to drug-free macromolecular therapeutics. Biomaterials. 2019;190–191:11–23.
- Gardell JL, Parker DC. CD40L is transferred to antigen-presenting B cells during delivery of T-cell help. Eur J Immunol. 2017;47:41–50.
- Fanale M, Assouline S, Kuruvilla J, Solal-Céligny P, Heo DS, Verhoef G, Corradini P, Abramson JS, Offner F, Engert A, et al. Phase IA/II, multicentre, open-label study of the CD40 antagonistic monoclonal antibody lucatumumab in adult patients with advanced non-Hodgkin or Hodgkin lymphoma. Br J Haematol. 2014;164:258–265.