ABSTRACT
Increased exposure of calreticulin (CALR) on malignant cells is associated with therapy-relevant adaptive immune responses and superior therapeutic outcome in solid tumors and haemato-oncological diseases, because surface-exposed CALR acts as an ‘eat-me’ signal facilitating the phagocytosis of stressed and dying cancer cells by immature dendritic cells, thus favoring antitumor immune responses. On the contrary, mutations of the CALR gene that cause the omission of the C-terminal KDEL endoplasmic reticulum retention motif from CALR protein, resulting in its secretion from cells, act as oncogenic drivers in myeloproliferative neoplasms via the autocrine activation of the thrombopoietin receptor. We recently showed that soluble CALR inhibited the phagocytosis of cancer cells by dendritic cells, thus dampening anticancer immune responses. Furthermore, systemic elevations of soluble CALR that is secreted from tumors or that is artificially supplied by injection of the recombinant protein decreased the efficacy of immunotherapy. Thus, depending on its location, CALR can have immunostimulatory or immunosuppressive functions.
Calreticulin (CALR) is an endoplasmic reticulum (ER)-resident chaperone that mediates protein (re)folding and facilitates cellular Ca2+ storage and homeostasis. The full-body knockout of CALR is embryonically lethal, emphasizing its crucial function for the regulation of cellular homeostasis.Citation1 The CALR protein consists of three structurally and functionally distinct domains, the N-terminus that contains the ER-signal sequence, followed by the proline-rich P-domain harboring the chaperone function and the C-terminus with the KDEL tetrapeptide motif for ER retention.Citation2 The compartmental targeting (N-terminus) and the KDEL-mediated retention (C-terminus) together dictate the confinement of CALR to the ER lumen in cellular homeostasis. However, in response to specific stress signals impacting the ER, CALR can translocate to distinct cellular compartments, thereby modulating a broad variety of physiological processes including cell adhesion and migration as well as the phagocytosis of apoptotic cells, thus impacting on diverse processes such as wound healing and immune responses.Citation3
In specific circumstances, for instance in response to chemotherapy with anthracyclines or oxaliplatin (which are chemotherapeutics inducing “immunogenic cell death”, ICD), cancer cells experience a partial ER stress response, that involves the phosphorylation of eukaryotic translation initiation factor 2α (EIF2A, better known as eIF2α), mostly by eukaryotic EIF2A kinase 3 (EIF2AK3, better known as PERK) and in some instances by EIF2AK4 (better known as GCN2), but does not comprise the activation of other facets of the unfolded stress response involving ATF4, ATF6 or XBP1. It is in this particular context of a focused ER stress response that CALR can translocate to the surface of the cytoplasmic membrane. Once exposed at the outer leaflet of the cellular membrane, CALR serves as a danger associated molecular pattern (DAMP) for the recognition of stressed and dying cells by the innate immune system, in particular immature dendritic cells (DCs), which are the most important antigen-presenting cells.Citation4-Citation8 Indeed, eIF2α phosphorylation appears to be required for ICD, as this has been shown for both anticancer chemotherapy and radiotherapy.Citation7,Citation9-Citation12 In this settings, surface-exposed CALR acts as de novo uptake signal via the ligation of pattern recognition receptors (PRRs) such as low density lipoprotein receptor-related protein 1 (LRP1, better known as CD91) on DCs, thus facilitating the engulfment of tumor-associated antigens expressed by malignant cells and their MHC class I-restricted cross-presentation to CD8+ T lymphocytes.Citation13,Citation14 () As such, CALR exposure constitutes a central adjuvant component of ICD, that ultimately facilitates the induction of cytotoxic T cell (CTL)-mediated anticancer immune responses.Citation9 Elevated CALR exposure on the outer leaflet of cancer cells has been linked with anti-cancer immunity and superior therapeutic outcome in patients with non-small cell lung carcinoma (NSCLC), colorectal carcinoma (CRC), acute myeloid leukemia (AML), ovarian cancer and high-grade serous carcinomas (HGSCs).Citation15-Citation19 () In this setting, elevated levels of CALR mRNA and protein expression at the site of the tumor correlate with beneficial disease outcome. A recent work from Truxova and collaborators discussed the ability of CALR exposed by malignant blasts from acute myeloid leukemia patients to trigger an IL-15-mediated trans-presentation and activation of natural killer cells further corroborating its importance for anti-cancer immunity.Citation21 On the contrary, the expression of the surface protein CD47 on cancer cells can transmit an inhibitory “don’t eat me” signal upon the ligation with its receptor signal regulatory protein α (SIRPα), primarily expressed on phagocytic cells such as DCs. Thus the overabundance of CD47 may potentially play an important role in tumor cell evasion.Citation22-Citation27
Table 1. CALR as a biomarker in human cancer.
Table 2. Secreted CALR as a biomarker in myelofibrosis and solid tumors.
Figure 1. Surface-exposed calreticulin serves as an uptake signal for dendritic cells. Certain anticancer regimens induce T cell-dependent adaptive anticancer immunity via the initiation of immunogenic cell death (ICD). One of the apical hallmarks of ICD is a partial endoplasmic reticulum (ER) stress response that leads to the phosphorylation of eIF2α in the absence of other manifestations of the unfolded protein response. The resultant exposure of calreticulin (CALR) on the surface of dying cells facilitates their recognition by dendritic cells (DC) and thus enables tumor-associated antigen transfer culminating in adaptive anticancer immunity.
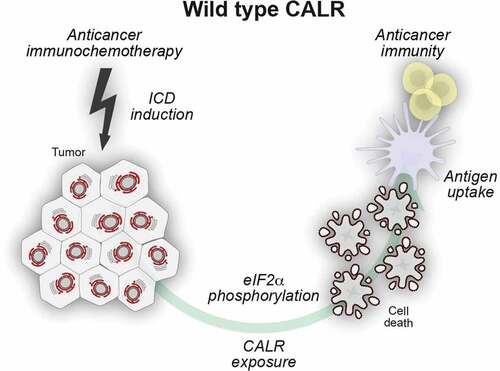
Soluble CALR can be passively released or actively secreted from cells, as this occurs in the context of massive cell death upon anticancer chemotherapy. Moreover, CALR can be secreted by macrophages stimulated by toll-like receptor (TLR) agonists and then contributes to the recognition and phagocytosis of adjacent tumor cells.Citation32 In addition, extracellular soluble CALR stimulates wound healing, as well as neoangiogenesis.Citation31
Exon 9 mutations of CALR have been identified in up to 30% of patients affected by myeloproliferative neoplasms (MPNs) such as essential thrombocythemia (ET) and myelofibrosis (MF).Citation33,Citation34 The most recurrent mutations typically manifest as either a 52 base pair deletion of residues 1092 to 1142 (CALRdel52) or a 5 base pair insertion between residues 1154 and 1155 (CALRins5). Both mutations lead to an alternative open reading frame, resulting in similar changes in the C-terminal amino acid sequence of the protein that becomes positively charged and loses the KDEL ER retention signal.Citation35 Consequently, mutant CALR protein fails to be detected by KDEL retention receptors and thus enters the conventional protein secretion pathway and is released via Golgi-mediated exocytosis.Citation30,Citation36,Citation37 Secreted CALR mutants bind (via their lectin binding sites) to the extracellular domain of the thrombopoietin receptor (MPL) in a cell autonomously or paracrine fashion thus leading to a downstream activation of the Janus kinase 2 (JAK2) and signal transducer and activator of transcription (STAT) proteins STAT1, STAT3 and STAT5.Citation38-Citation41 Introduction of analogous CALR mutations into mice recapitulates the ET-like disease and its progression to myelofibrosis.Citation41-Citation43 Thus, CALR mutants act as oncogenic driver of MPN.Citation39,Citation41,Citation44,Citation45 Moreover, patients with MPN-associated CALR mutations exhibit an increase in myeloid derived suppressor cells (MDSC) and immunosuppressive B cells, suggesting that mutated CALR may subvert immune responses.Citation44,Citation46
Recently, we showed that exon 9-mutated CALR is not only secreted from cells via Golgi-mediated protein secretion but also acquires a novel immunosuppressive function, once it accumulates in the extracellular space. Indeed, soluble CALR acts as a decoy, preventing the phagocytosis of CALR-exposing cells by DC in vitro, as well as in vivo, in mice (). The release of soluble CALR from tumors mediated robust immunosuppressive effects and abolished therapeutic responses to immunogenic chemotherapy (with anthracyclines or oxaliplatin) as well as immune checkpoint blockade targeting the PD-1/PD-L1 interaction.Citation29
Figure 2. Secreted calreticulin negatively impacts on phagocytosis. Calreticulin (CALR) mutants that affect the C-terminal endoplasmic reticulum (ER)-retention signal KDEL can enter the canonical protein secretion pathway. Soluble CALR protein is secreted via Golgi-dependent exocytosis and ligates surface receptors on antigen presenting cells. In this setting, soluble CALR acts as a decoy that triggers receptor saturation and inhibits the dendritic cell (DC)-mediated phagocytosis of stressed and dying cancer cells, thus blunting adaptive immunity to tumor-associated antigens.
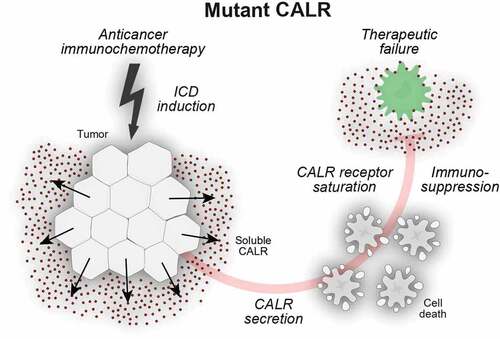
In the context of MPNs, soluble CALR provides both an autocrine signal for the activation of an oncogenic driver fostering disease progression and subverts phagocytosis of malignant cells thus jeopardizing anticancer immune responses. This might explain the precocious initiation of MPN associated with CALR mutants, occurring some 10 years earlier than in MPN associated with the JAK2V617 F mutation.Citation44 This correlates with the fact that CALR mutations are present in all hematopoietic cells (both myeloid and lymphoid) and give a high clonal dominance to the hematopoietic stem compartment compared to JAK2V617 F in ET.Citation43,Citation47 () Preclinical experiments suggest that mutations leading to the release of CALR in MPNs would interfere with therapeutic measure designed to (re)establish an anticancer immune response, a conjecture that must be validated at the clinical level. Of note, we found that a small percentage (<1%) of human carcinomas also exhibited CALR mutations in exon 9 and that such mutations, which are different from the MPN-associated ones, also led to the secretion of CALR protein, as well as to the subversion of therapeutic responses to immunogenic chemotherapy or PD-1 blockade in preclinical experiments. It will be important to investigate the clinical impact of such CALR mutations for patient prognosis.
Disclosure of potential conflicts of interest
OK and GK are scientific co-founders of Samsara Therapeutics.
Acknowledgments
GK is supported by the Ligue contre le Cancer (équipe labellisée); Agence National de la Recherche (ANR) – Projets blancs; ANR under the frame of E-Rare-2, the ERA-Net for Research on Rare Diseases; AMMICa US23/CNRS UMS3655; Association pour la recherche sur le cancer (ARC); Association “Le Cancer du Sein, Parlons-en!”; Cancéropôle Ile-de-France; Chancelerie des universités de Paris (Legs Poix), Fondation pour la Recherche Médicale (FRM); a donation by Elior; European Research Area Network on Cardiovascular Diseases (ERA-CVD, MINOTAUR); Gustave Roussy Odyssea, the European Union Horizon 2020 Project Oncobiome; Fondation Carrefour; High-end Foreign Expert Program in China (GDW20171100085), Institut National du Cancer (INCa); Inserm (HTE); Institut Universitaire de France; LeDucq Foundation; the LabEx Immuno-Oncology (ANR-18-IDEX-0001); the RHU Torino Lumière; the Seerave Foundation; the SIRIC Stratified Oncology Cell DNA Repair and Tumor Immune Elimination (SOCRATE); and the SIRIC Cancer Research and Personalized Medicine (CARPEM).
References
- Mesaeli N, Nakamura K, Zvaritch E, Dickie P, Dziak E, Krause K-H, Opas M, MacLennan DH, Michalak M. Calreticulin is essential for cardiac development. J Cell Biol. 1999;144(5):857–5. doi:10.1083/jcb.144.5.857.
- Gelebart P, Opas M, Michalak M. Calreticulin, a Ca2+-binding chaperone of the endoplasmic reticulum. Int J Biochem Cell Biol. 2005;37(2):260–266. doi:10.1016/j.biocel.2004.02.030.
- Gold LI, Eggleton P, Sweetwyne MT, Van Duyn LB, Greives MR, Naylor S-M, Michalak M, Murphy‐Ullrich JE. Calreticulin: non-endoplasmic reticulum functions in physiology and disease. FASEB J. 2010;24:665–683. doi:10.1096/fj.09-145482.
- Bezu L, Sauvat A, Humeau J, Gomes-da-Silva LC, Iribarren K, Forveille S, Garcia P, Zhao L, Liu P, Zitvogel L. eIF2α phosphorylation is pathognomonic for immunogenic cell death. Cell Death Differ. 2018;25(8):1375–1393. doi:10.1038/s41418-017-0044-9.
- Garg AD, Krysko DV, Verfaillie T, Kaczmarek A, Ferreira GB, Marysael T, Rubio N, Firczuk M, Mathieu C, Roebroek AJM, et al. A novel pathway combining calreticulin exposure and ATP secretion in immunogenic cancer cell death. Embo J. 2012;31(5):1062–1079. doi:10.1038/emboj.2011.497.
- Obeid M, Panaretakis T, Joza N, Tufi R, Tesniere A, van Endert P, Zitvogel L, Kroemer G. Calreticulin exposure is required for the immunogenicity of γ-irradiation and UVC light-induced apoptosis. Cell Death Differ. 2007;14(10):1848–1850. doi:10.1038/sj.cdd.4402201.
- Panaretakis T, Kepp O, Brockmeier U, Tesniere A, Bjorklund A-C, Chapman DC, Durchschlag M, Joza N, Pierron G, van Endert P. Mechanisms of pre-apoptotic calreticulin exposure in immunogenic cell death. Embo J. 2009;28(5):578–590. doi:10.1038/emboj.2009.1.
- van Vliet AR, Giordano F, Gerlo S, Segura I, Van Eygen S, Molenberghs G, Rocha S, Houcine A, Derua R, Verfaillie T, et al. The ER stress sensor PERK coordinates ER-plasma membrane contact site formation through interaction with Filamin-A and F-Actin remodeling. Mol Cell. 2017;65(5):885–899 e886. doi:10.1016/j.molcel.2017.01.020.
- Galluzzi L, Buque A, Kepp O, Zitvogel L, Kroemer G. Immunogenic cell death in cancer and infectious disease. Nat Rev Immunol. 2017;17(2):97–111. doi:10.1038/nri.2016.107.
- Garg AD, Galluzzi L, Apetoh L, Baert T, Birge RB, Bravo-San Pedro JM, Breckpot K, Brough D, Chaurio R, Cirone M, et al. Molecular and translational classifications of DAMPs in immunogenic cell death. Front Immunol. 2015;6:588. doi:10.3389/fimmu.2015.00588.
- Galluzzi L, Vitale I, Warren S, Adjemian S, Agostinis P, Martinez AB, Chan TA, Coukos G, Demaria S, Deutsch E, et al. Consensus guidelines for the definition, detection and interpretation of immunogenic cell death. J Immuno Cancer. 2020;8(1):e000337. doi:10.1136/jitc-2019-000337.
- Obeid M, Tesniere A, Ghiringhelli F, Fimia GM, Apetoh L, Perfettini J-L, Castedo M, Mignot G, Panaretakis T, Casares N, et al. Calreticulin exposure dictates the immunogenicity of cancer cell death. Nat Med. 2007;13(1):54–61. doi:10.1038/nm1523.
- Brown GC, Neher JJ. Eaten alive! Cell death by primary phagocytosis: ‘phagoptosis’. Trends Biochem Sci. 2012;37(8):325–332. doi:10.1016/j.tibs.2012.05.002.
- Gardai SJ, Xiao Y-Q, Dickinson M, Nick JA, Voelker DR, Greene KE, Henson PM. By binding SIRPalpha or calreticulin/CD91, lung collectins act as dual function surveillance molecules to suppress or enhance inflammation. Cell. 2003;115:13–23. doi:10.1016/S0092-8674(03)00758-X.
- Kasikova L, Hensler M, Truxova I, Skapa P, Laco J, Belicova L, Praznovec I, Vosahlikova S, Halaska MJ, Brtnicky T. Calreticulin exposure correlates with robust adaptive antitumor immunity and favorable prognosis in ovarian carcinoma patients. J Immuno Cancer. 2019;7(1):312. doi:10.1186/s40425-019-0781-z.
- Peng RQ, Chen YB, Ding Y, Zhang R, Zhang X, Yu XJ, Zhou ZW, Zeng YX, Zhang XS. Expression of calreticulin is associated with infiltration of T-cells in stage IIIB colon cancer. World J Gastroenterol. 2010;16:2428–2434. doi:10.3748/wjg.v16.i19.2428.
- Stoll G, Iribarren K, Michels J, Leary A, Zitvogel L, Cremer I, Kroemer G. Calreticulin expression: interaction with the immune infiltrate and impact on survival in patients with ovarian and non-small cell lung cancer. Oncoimmunology. 2016;5(7):e1177692. doi:10.1080/2162402X.2016.1177692.
- Fucikova J, Becht E, Iribarren K, Goc J, Remark R, Damotte D, Alifano M, Devi P, Biton J, Germain C. Calreticulin expression in human non–small cell lung cancers correlates with increased accumulation of antitumor immune cells and favorable prognosis. Cancer Res. 2016;76(7):1746–1756. doi:10.1158/0008-5472.CAN-15-1142.
- Fucikova J, Truxova I, Hensler M, Becht E, Kasikova L, Moserova I, Vosahlikova S, Klouckova J, Church SE, Cremer I, et al. Calreticulin exposure by malignant blasts correlates with robust anticancer immunity and improved clinical outcome in AML patients. Blood. 2016;128(26):3113–3124. doi:10.1182/blood-2016-08-731737.
- Wemeau M, Kepp O, Tesnière A, Panaretakis T, Flament C, De Botton S, Zitvogel L, Kroemer G, Chaput N. Calreticulin exposure on malignant blasts predicts a cellular anticancer immune response in patients with acute myeloid leukemia. Cell Death Dis. 2010;1(12):e104. doi:10.1038/cddis.2010.82.
- Truxova I, Kasikova L, Salek C, Hensler M, Lysak D, Holicek P, Bilkova P, Holubova M, Chen X, Mikyskova R, et al. Calreticulin exposure on malignant blasts correlates with improved natural killer cell-mediated cytotoxicity in acute myeloid leukemia patients. Haematologica. 2019. doi:10.3324/haematol.2019.223933.
- Chao MP, Jaiswal S, Weissman-Tsukamoto R, Alizadeh AA, Gentles AJ, Volkmer J, Weiskopf K, Willingham SB, Raveh T, Park CY, et al. Calreticulin is the dominant pro-phagocytic signal on multiple human cancers and is counterbalanced by CD47. Sci Transl Med. 2010;2(63):63ra94. doi:10.1126/scitranslmed.3001375.
- Chen J, Zheng D-X, Yu X-J, Sun H-W, Xu Y-T, Zhang Y-J, Xu J. Macrophages induce CD47 upregulation via IL-6 and correlate with poor survival in hepatocellular carcinoma patients. Oncoimmunology. 2019;8(11):e1652540. doi:10.1080/2162402X.2019.1652540.
- Galluzzi L, Yamazaki T, Kroemer G. Linking cellular stress responses to systemic homeostasis. Nature Reviews Molecular Cell Biology. 2018;19(11):731–745. doi:10.1038/s41580-018-0068-0.
- Iribarren K, Buque A, Mondragon L, Xie W, Lévesque S, Pol J, Zitvogel L, Kepp O, Kroemer G. Anticancer effects of anti-CD47 immunotherapy in vivo. Oncoimmunology. 2019;8(3):1550619. doi:10.1080/2162402X.2018.1550619.
- Majeti R, Chao MP, Alizadeh AA, Pang WW, Jaiswal S, Gibbs KD, van Rooijen N, Weissman IL. CD47 is an adverse prognostic factor and therapeutic antibody target on human acute myeloid leukemia stem cells. Cell. 2009;138(2):286–299. doi:10.1016/j.cell.2009.05.045.
- Martinek J, Wu TC, Cadena D, Banchereau J, Palucka K. Interplay between dendritic cells and cancer cells. Int Rev Cell Mol Biol. 2019;348:179–215. doi:10.1016/bs.ircmb.2019.07.008.
- Pecquet C, Chachoua I, Roy A, Balligand T, Vertenoeil G, Leroy E, Albu R-I, Defour J-P, Nivarthi H, Hug E. Calreticulin mutants as oncogenic rogue chaperones for TpoR and traffic-defective pathogenic TpoR mutants. Blood. 2019;133(25):2669–2681. doi:10.1182/blood-2018-09-874578.
- Liu P, Zhao L, Loos F, Marty C, Xie W, Martins I, Lachkar S, Qu B, Waeckel-Énée E, Plo I, et al. Immunosuppression by mutated calreticulin released from malignant cells. Mol Cell. 2019. doi:10.1016/j.molcel.2019.11.004.
- Garbati MR, Welgan CA, Landefeld SH, Newell LF, Agarwal A, Dunlap JB, Chourasia TK, Lee H, Elferich J, Traer E, et al. Mutant calreticulin-expressing cells induce monocyte hyperreactivity through a paracrine mechanism. Am J Hematol. 2016;91(2):211–219. doi:10.1002/ajh.24245.
- Pike SE, Yao L, Jones KD, Cherney B, Appella E, Sakaguchi K, Nakhasi H, Teruya-Feldstein J, Wirth P, Gupta G, et al. Vasostatin, a calreticulin fragment, inhibits angiogenesis and suppresses tumor growth. J Exp Med. 1998;188(12):2349–2356. doi:10.1084/jem.188.12.2349.
- Feng M, Chen JY, Weissman-Tsukamoto R, Volkmer J-P, Ho PY, McKenna KM, Cheshier S, Zhang M, Guo N, Gip P. Macrophages eat cancer cells using their own calreticulin as a guide: roles of TLR and Btk. Proc Natl Acad Sci U S A. 2015;112(7):2145–2150. doi:10.1073/pnas.1424907112.
- Klampfl T, Gisslinger H, Harutyunyan AS, Nivarthi H, Rumi E, Milosevic JD, Them NCC, Berg T, Gisslinger B, Pietra D, et al. Somatic mutations of calreticulin in myeloproliferative neoplasms. N Engl J Med. 2013;369(25):2379–2390. doi:10.1056/NEJMoa1311347.
- Nangalia J, Massie CE, Baxter EJ, Nice FL, Gundem G, Wedge DC, Avezov E, Li J, Kollmann K, Kent DG, et al. Somatic CALR Mutations in Myeloproliferative Neoplasms with Nonmutated JAK2. N Engl J Med. 2013;369(25):2391–2405. doi:10.1056/NEJMoa1312542.
- Grinfeld J, Nangalia J, Baxter EJ, Wedge DC, Angelopoulos N, Cantrill R, Godfrey AL, Papaemmanuil E, Gundem G, MacLean C, et al. Classification and personalized prognosis in myeloproliferative neoplasms. N Engl J Med. 2018;379(15):1416–1430. doi:10.1056/NEJMoa1716614.
- Arshad N, Cresswell P. Tumor-associated calreticulin variants functionally compromise the peptide loading complex and impair its recruitment of MHC-I. J Biol Chem. 2018;293(25):9555–9569. doi:10.1074/jbc.RA118.002836.
- Han L, Schubert C, Köhler J, Schemionek M, Isfort S, Brümmendorf TH, Koschmieder S, Chatain N. Calreticulin-mutant proteins induce megakaryocytic signaling to transform hematopoietic cells and undergo accelerated degradation and Golgi-mediated secretion. J Hematol Oncol. 2016;9(1):45. doi:10.1186/s13045-016-0275-0.
- Araki M, Yang Y, Masubuchi N, Hironaka Y, Takei H, Morishita S, Mizukami Y, Kan S, Shirane S, Edahiro Y. Activation of the thrombopoietin receptor by mutant calreticulin in CALR-mutant myeloproliferative neoplasms. Blood. 2016;127(10):1307–1316. doi:10.1182/blood-2015-09-671172.
- Chachoua I, Pecquet C, El-Khoury M, Nivarthi H, Albu R-I, Marty C, Gryshkova V, Defour J-P, Vertenoeil G, Ngo A. Thrombopoietin receptor activation by myeloproliferative neoplasm associated calreticulin mutants. Blood. 2016;127(10):1325–1335. doi:10.1182/blood-2015-11-681932.
- Elf S, Abdelfattah NS, Chen E, Perales-Paton J, Rosen EA, Ko A, Peisker F, Florescu N, Giannini S, Wolach O. Mutant calreticulin requires both its mutant C-terminus and the thrombopoietin receptor for oncogenic transformation. Cancer Discov. 2016;6(4):368–381. doi:10.1158/2159-8290.CD-15-1434.
- Marty C, Pecquet C, Nivarthi H, El-Khoury M, Chachoua I, Tulliez M, Villeval J-L, Raslova H, Kralovics R, Constantinescu SN. Calreticulin mutants in mice induce an MPL-dependent thrombocytosis with frequent progression to myelofibrosis. Blood. 2016;127(10):1317–1324. doi:10.1182/blood-2015-11-679571.
- Balligand T, Achouri Y, Pecquet C, Gaudray G, Colau D, Hug E, Rahmani Y, Stroobant V, Plo I, Vainchenker W, et al. Knock-in of murine Calr del52 induces essential thrombocythemia with slow-rising dominance in mice and reveals key role of Calr exon 9 in cardiac development. Leukemia. 2019. doi:10.1038/s41375-019-0538-1.
- Kjaer L, Holmström MO, Cordua S, Andersen MH, Svane IM, Thomassen M, Kruse TA, Pallisgaard N, Skov V, Hasselbalch HC. Sorted peripheral blood cells identify CALR mutations in B- and T-lymphocytes. Leuk Lymphoma. 2018;59(4):973–977. doi:10.1080/10428194.2017.1359743.
- Vainchenker W, Kralovics R. Genetic basis and molecular pathophysiology of classical myeloproliferative neoplasms. Blood. 2017;129(6):667–679. doi:10.1182/blood-2016-10-695940.
- Elf S, Abdelfattah NS, Baral AJ, Beeson D, Rivera JF, Ko A, Florescu N, Birrane G, Chen E, Mullally A. Defining the requirements for the pathogenic interaction between mutant calreticulin and MPL in MPN. Blood. 2018;131(7):782–786. doi:10.1182/blood-2017-08-800896.
- Hong C, Zhang T, Gao XM. Recombinant murine calreticulin fragment 39-272 expands CD1d(hi)CD5+ IL-10-secreting B cells that modulate experimental autoimmune encephalomyelitis in C57BL/6 mice. Mol Immunol. 2013;55:237–246. doi:10.1016/j.molimm.2013.02.003.
- Nam AS, Kim K-T, Chaligne R, Izzo F, Ang C, Taylor J, Myers RM, Abu-Zeinah G, Brand R, Omans ND. Somatic mutations and cell identity linked by Genotyping of Transcriptomes. Nature. 2019;571(7765):355–360. doi:10.1038/s41586-019-1367-0.