ABSTRACT
The COVID-19 pandemic has afflicted most countries on the planet. As a result, immunity against SARS-CoV-2, induced via natural infections or imminent vaccinations, is expected to develop in a large fraction of the global population. Here, we propose to exploit SARS-CoV-2-specific CD8+ T cells for cancer immunotherapy strategies.
The pandemic of coronavirus disease 2019 (COVID-19), caused by the emergent virus named severe acute respiratory syndrome-coronavirus-2 (SARS-CoV-2), has put the whole world on pause. As of July 6, 2020, SARS-CoV-2 infections have been confirmed in approximately 11.4 M people, have caused more than 530,000 deaths, and have been reported by nearly all countries in the world. COVID-19 is a respiratory illness characterized by a range of symptoms such as cough, fever, and dyspnea.Citation1,Citation2 In severe COVID-19 cases, an acute respiratory distress syndrome (ARDS) is often manifested and requires mechanical ventilation. It is becoming clear that the outcome of SARS-CoV-2 infection and the underlying COVID-19 pathology is regulated by the interaction between the virus and the host immune system. In line with the key role of the immune system, patients who have immune-related co-morbidities are more prone to develop severe disease following SARS-CoV-2 infection, and the activation or suppression of the host immune response has become a reliable predictor of COVID-19 outcomes.Citation1–Citation3
SARS-CoV-2 induces antiviral CD8+ T cell responses
Antiviral immune defenses regulate the outcomes of viral infections wherein concerted actions of the innate and adaptive arms restrict the replication and spread of the virus.Citation4 Based on the available evidence, SARS-CoV-2 likely generates danger signals (e.g., microbe-associated molecular patterns [MAMPs] such as viral RNA and proteins, and damage-associated molecular patterns [DAMPs] from dying pneumocytes), thereby engaging various pattern recognition receptors (PRRs) including Toll-like receptors (TLRs), nucleotide-binding oligomerization domain [NOD]-like receptors (NLRs), and RIG-I-like receptors (RLRs).Citation3 Such virus-induced signaling through PRRs drives an inflammatory cascade encompassing type I and III interferons (IFNs) that inhibits virus replication and facilitates the presentation of viral epitopes by antigen-presenting cells (APCs) for priming of T and B cells. In particular, primed CD8+ T cells identify viral epitopes bound to major histocompatibility complex (MHC) class-I molecules of virus-infected cells, eliminate the virus replication niche in an antigen-specific manner, and maintain immunological memory following the resolution of the disease (herein termed as antiviral CD8+ T cells). Indeed, strong effector, as well as memory, CD8+ T cell immunity is a hallmark of many common human viral infections. Data from patients who have recovered from SARS-CoV-2 infection suggest that a prototypical antiviral CD8+ T cell response accompanies SARS-CoV-2 infection.Citation1,Citation2,Citation5–Citation7 Similar SARS-CoV-2-specific CD8+ T cell responses are expected to be induced following the administration of appropriate vaccines that are being developed by competing public and private laboratories. Thus, SARS-CoV-2-specific antiviral CD8+ T cell responses, raised upon natural infections or anticipated vaccine campaigns, are set to be prevalent in tens, perhaps hundreds, of millions of individuals.
Antitumor CD8+ T cells mediate the actions and clinical efficacy of mainstream immunotherapies
Tumor antigen-specific CD8+ T cells (herein called as antitumor CD8+ T cells) represent a major effector entity within modern cancer immunotherapies, as they identify and kill malignant cells expressing cognate cancer-specific epitopes without causing harm to nonmalignant cells. Once activated, antitumor CD8+ T cells can eliminate not only existing but also recurrent cancer cells, thus holding the key for long-term cancer-free health. Furthermore, in addition to targeting cancer cells within the local tumor microenvironment (TME), CD8+ T cells can detect and destroy cancer cells at distant metastatic niches. Not surprisingly, the actions of antitumor CD8+ T cells form the foundation of many promising cancer immunotherapies, including those employing immune checkpoint inhibitors (ICIs) with monoclonal antibodies against programmed death 1 (PD-1), programmed death-ligand 1 (PDL-1), or cytotoxic T-lymphocyte-associated protein 4 (CTLA-4).Citation8 Indeed, the antitumor CD8+ T cell response has become a highly reliable indicator of positive outcomes in cancer patients, and novel strategies activating antitumor CD8+ T cells are fiercely pursued.
Antiviral CD8+ T cells can be repurposed for antitumor actions
Although antiviral and antitumor CD8+ T cells bear distinct T cell receptors (TCRs) against virus- and tumor-derived MHC-I-presented ligands (i.e., epitopes), respectively, newly reported immunotherapy strategies now allow for ‘repurposing’ of antiviral CD8+ T cells for antitumor functions.Citation9,Citation10 For this purpose, synthetic immunogenic viral epitopes are administered in a way that they bind to MHC-I molecules on the surface of tumor cells. These viral epitope-presenting tumor cells simulate a reinfection event and ‘trick’ antiviral CD8+ T cells into an anticancer attack. Even adjuvant-free injection of synthetic viral epitopes suffices to stimulate an antiviral CD8+ T cell-mediated attack against cancer cells.Citation9 This repurposing of antiviral CD8+ T cells for anticancer therapy is potentiated by the actions of ICIs that are currently approved for clinical use.Citation9,Citation10 Of note, possible systemic side effects on healthy tissues within these repurposing approaches can be controlled by confining the expression of exogenously supplied viral peptide to the TME. These previously unknown nascent advances in the cancer immunotherapy fieldCitation9,Citation10 now pave a new direction for exploiting preexisting antiviral CD8+ T cells arising from common human viral infections for antitumor therapeutic implications. It is noteworthy that, as compared to antitumor CD8+ T cells, antiviral CD8+ T cells often display better breadth, polyclonality, frequency, and functionality as they are generated against highly immunogenic ‘non-self’ epitopes from viral antigens. Furthermore, the negative effects of host factors dampening the therapeutically relevant traits of antitumor CD8+ T cells, such as thymic or systemic tolerance and the immunosuppressive TME, remain negligible or absent in the context of antiviral CD8+ T cells. Finally, the specificity of antiviral CD8+ T cells against ‘non-self’ epitopes minimizes possible autoreactivity during the repurposing regimens. In summary, antiviral CD8+ T cells, bearing an inherently superior immunological competence of clinical importance than antitumor CD8+ T cells, represent a potent avenue to formulate highly efficacious cancer immunotherapies.
SARS-CoV-2-specific CD8+ T cells can be repurposed for cancer immunotherapy strategies
Antiviral CD8+ T cells specific for previously encountered viral infections (e.g., cytomegalovirus [CMV], Epstein-Barr virus [EBV], influenza virus) are abundantly found within human tumors, and have been successfully repurposed to induce antitumor immune responses.Citation9–Citation12 Considering the relatively recent emergence and transmission of SARS-CoV-2 in humans, the underlying antiviral adaptive immune response is expected to display appropriate epitope dominancy devoid of features of ‘antigenic sin’ observed for common infections like influenza. Evidence thus far shows that prototypical effector and memory CD8+ T cell responses are observed in patients who have recovered from COVID-19.Citation1,Citation3 Thus, administration of SARS-CoV-2 antigens or immunogenic epitopes directed to be presented by class-I human leukocyte antigens (HLAs) would represent a clinically feasible and readily applicable approach for repurposing SARS-CoV-2-specific CD8+ T cell responses for anticancer immunotherapy (). Herein, the delivery of SARS-CoV-2 epitopes within the TME could be achieved via direct intratumoral injectionCitation9,Citation10 or using antibody-peptide epitope conjugates (APECs) programed to specifically target tumor cells.Citation9,Citation10 It is noteworthy that worldwide major efforts are currently being dedicated to map SARS-CoV-2 epitopes in the context of diverse human HLA haplotypes and that this knowledge will facilitate the exploitation of SARS-CoV-2-specific CD8+ T cell immunity. Recent findings have demonstrated the cross-reactivity of SARS-CoV-2 specific epitopes in inducing T cell responses in SARS-CoV-1 recovered patients and SARS-CoV-1/2 unexposed individuals due to the high sequence homology between SARS-CoV-2 and other betacoronaviruses.Citation5–Citation7 The presence of such antiviral T cells in non-COVID-19 patients further increases the percentage of the general population who may benefit from repurposing SARS-CoV-2-specific immunity to target cancer. Furthermore, antiviral CD8+ T cells in patients with COVID-19 express immune checkpoints such as PD-1 and T cell immunoglobulin mucin 3 (TIM-3) and thus are amenable for ICI-based immune potentiation of cancer immunotherapy strategies. Thus, similar to the antiviral CD8+ T cells against CMV and EBV, SARS-CoV-2-specific CD8+ T cells possess antitumor potential that can be harnessed within cancer immunotherapy approaches.
Figure 1. Repurposing SARS-CoV-2-specific antiviral CD8+ T cells for cancer immunotherapy. Patients recovered from COVID-19 or immunized with the relevant vaccines are expected to develop CD8+ T cell immunity against SARS-CoV-2. Tumors developing later on in SARS-CoV-2-immunity-bearing individuals will likely host SARS-CoV-2-specific CD8+ T cells that could be repurposed to target cancer cells. In this therapeutic approach, delivery of SARS-CoV-2-derived immunogenic epitopes (via, for example, adjuvant-free intratumoral injection or antibody-peptide epitope conjugatesCitation9,Citation10) results in their presentation by MHC-I molecules on the surface of cancer cells. Consequently, antiviral CD8+ T cells recognize their cognate epitopes and get ‘tricked’ into killing these cancer cells, simulating a virus infection (direct antitumor benefits of antiviral CD8+ T cells). Additionally, the spreading of tumor antigens released during the initial direct killing by antiviral CD8+ T cells facilitates their cross-presentation by APCs and the induction of an antitumor immunity that will attack any residual or recurring cancer cells (indirect antitumor benefits of antiviral CD8+ T cells). Interestingly, the antitumor benefits of such antiviral CD8+ T cell repurposing approaches can be further potentiated with immune checkpoint inhibitors. CTL, cytotoxic CD8+ T lymphocyte; MHC-I, major histocompatibility complex class-I; TME, tumor microenvironment.
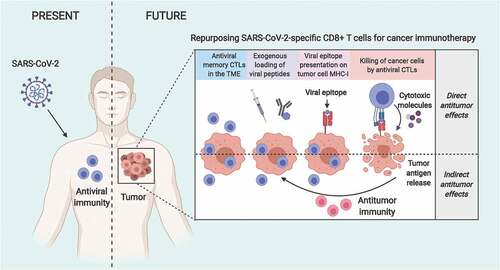
Viruses and antiviral immunity form the core of emerging cancer therapy approaches
Viruses, viral infections, virus-based therapeutics, and virus-induced host immune responses can be harnessed within cancer immunotherapy approaches. Full remissions from cancer following viral infections have been reported throughout the history of modern medicine, and virus-based platforms are poised to become mainstream cancer immunotherapies.Citation13 For example, remissions from leukemia, Hodgkin’s disease and lymphoma have been well documented after influenza, chicken pox, and measles infections. These historic observations led to the development of ‘cancer-killing’ viruses, commonly known as oncolytic viruses (OVs), which preferentially replicate in and kill malignant cells without causing similar effects on healthy cells. These OVs, which are under investigation in numerous clinical trials,Citation14 are now being widely acknowledged for their capacity to induce antitumor immunological benefits, including antitumor CD8+ T cell immune responses.Citation15 First, OV-induced antiviral immunity overrides tumor-associated immune evasion mechanisms and reinstates the effector functions of otherwise exhausted T cells. OV-expressed MAMPs and oncolysis-released DAMPs promote effective APC function and efficient priming of T cells. OV-mediated tumor cell lysis also favors antigen spreading.Citation16,Citation17 This phenomenon enlarges the spectrum of epitopes presented to the adaptive immune system and triggers a shift from a purely virus-oriented response toward a cancer cell-specific reactivity. In fine, OVs promote effective antitumor immunity and make tumors immunologically ‘hot’ through increasing T cell infiltration, thus sensitizing them to transversal immunotherapy with ICIs.Citation4 Similarly, other microbe-related compounds (e.g., bacillus Calmette-Guérin [BCG] instillations for bladder cancer treatment) can act as immunostimulatory agents, induce cancer immunogenic cell death (ICD), and promote antitumor immunity. Finally, the local/intratumoral injection of seasonal flu, rotavirus, or yellow fever vaccines have proven potent anticancer effects.Citation18 Thus, viruses (and bacteria) are contending to become a part of the legitimate armamentarium against cancers. Whether SARS-CoV-2 could be exploited within such anticancer strategies will be of great interest. These efforts could for example use SARS-CoV-2 for engineering novel OV platforms or making tumors hot. Similarly, as it has been done with vaccines against rotavirus, influenza, and yellow fever viruses,Citation18 anti-SARS-CoV-2 vaccines could be directly injected within the TME to promote tumoricidal immune activities. It is worth noting that the cancer immunotherapy strategies discussed in this section rely on engaging the actions of antitumor CD8+ T cells. Thus, in line with novel strategies allowing for repurposing of antiviral CD8+ T cells, cancer immunotherapies simultaneously harvesting both tumor- and SARS-CoV-2-specific CD8+ T cells promise to produce potentiated clinical benefits against cancers.
Conclusion
Based on the current state-of-the-art immunotherapy approaches, antiviral CD8+ T cell immunity induced by SARS-CoV-2 infections or vaccines might be harnessed for cancer immunotherapies against cancers of various types. Considering the tropism of SARS-CoV-2 for epithelial cells of nasal, bronchial, and bronchiolar mucosae,Citation19 stimulation of virus-specific tissue-resident memory T cells could be of particular interest for treating cancers developing in the respiratory tract of patients immunized with COVID-19-preventing vaccines or recovered from SARS-CoV-2 infections.
Abbreviations
APCs | = | Antigen-presenting cells |
APECs | = | Antibody-peptide epitope conjugates |
ARDS | = | Acute respiratory distress syndrome |
COVID-19 | = | Coronavirus disease 2019 |
DAMP | = | damage-associated molecular pattern |
HLA | = | Human leukocyte antigen |
ICD | = | Immunogenic cell death |
ICI | = | Immune checkpoint inhibitor |
MAMP | = | microbe-associated molecular pattern |
MHC | = | Major histocompatibility complex |
NLR | = | NOD-like receptor |
OV | = | Oncolytic virus |
PRR | = | pattern recognition receptor |
RLR | = | RIG-I-like receptor |
SARS-CoV-2 | = | Severe acute respiratory syndrome coronavirus 2 |
TCR | = | T cell receptor |
TLR | = | Toll-like receptor |
TME | = | Tumor microenvironment |
Disclosure of Potential Conflicts of Interest
JGP is named as an inventor on patents for cancer vaccination involving an oncolytic rhabdovirus. These patents have been licensed to Turnstone Biologics of which JGP is a shareholder. GK is a cofounder of Samsara Therapeutics, everImmune, and Therafast Bio.
Supplementary material
Supplemental data for this article can be accessed on the publisher’s website.
Additional information
Funding
References
- Chen G, Wu D, Guo W, Cao Y, Huang D, Wang H, Wang T, Zhang X, Chen H, Yu H, et al. Clinical and immunological features of severe and moderate coronavirus disease 2019. J Clin Invest. 2020;130:2620–4. doi:10.1172/JCI137244.
- Thevarajan I, Nguyen THO, Koutsakos M, Druce J, Caly L, van de Sandt CE, Jia X, Nicholson S, Catton M, Cowie B, et al. Breadth of concomitant immune responses prior to patient recovery: a case report of non-severe COVID-19. Nat Med. 26, 453–455 (2020).
- Vardhana SA, Wolchok JD. The many faces of the anti-COVID immune response. J Exp Med. 2020:217. doi:10.1084/jem.20200678.
- Gujar S, Pol JG, Kim Y, Lee PW, Kroemer G. Antitumor benefits of antiviral immunity: an underappreciated aspect of oncolytic virotherapies. Trends Immunol. 2018;39:209–221. doi:10.1016/j.it.2017.11.006.
- Grifoni A, Weiskopf D, Ramirez SI, Mateus J, Dan JM, Moderbacher CR, Rawlings SA, Sutherland A, Premkumar L, Jadi RS, et al. Targets of T cell responses to SARS-CoV-2 coronavirus in humans with COVID-19 disease and unexposed individuals. Cell. 2020;181:1489–1501.e15. doi:10.1016/j.cell.2020.05.015.
- Pia L. SARS-CoV-2-reactive T cells in patients and healthy donors. Nat Rev Immunol. 2020;20:2–353. doi:10.1038/s41577-020-0333-2.
- Weiskopf D, Schmitz KS, Raadsen MP, Grifoni A, Okba NMA, Endeman H, van den Akker JPC, Molenkamp R, Koopmans MPG, van Gorp ECM, et al. Phenotype and kinetics of SARS-CoV-2-specific T cells in COVID-19 patients with acute respiratory distress syndrome. Sci Immunol. 2020;5:eabd2071. doi:10.1126/sciimmunol.abd2071.
- Sharma P, Allison JP. Dissecting the mechanisms of immune checkpoint therapy. Nat Rev Immunol. 2020;20:75–76. doi:10.1038/s41577-020-0275-8.
- Rosato PC, Wijeyesinghe S, Stolley JM, Nelson CE, Davis RL, Manlove LS, Pennell CA, Blazar BR, Chen CC, Geller MA, et al. Virus-specific memory T cells populate tumors and can be repurposed for tumor immunotherapy. Nat. Commun. 2019;10:1–567. doi:10.1038/s41467-019-08534-1.
- Millar DG, Ramjiawan RR, Kawaguchi K, Gupta N, Chen J, Zhang S, Nojiri T, Ho WW, Aoki S, Jung K, et al. Antibody-mediated delivery of viral epitopes to tumors harnesses CMV-specific T cells for cancer therapy. Nat. Biotechnol. 2020;38:420–425. doi:10.1038/s41587-019-0404-8.
- Schuessler A, Smith C, Beagley L, Boyle GM, Rehan S, Matthews K, Jones L, Crough T, Dasari V, Klein K, et al. Autologous T-cell therapy for cytomegalovirus as a consolidative treatment for recurrent glioblastoma. Cancer Res. 2014;74(13):3466–3476. doi:10.1158/0008-5472.CAN-14-0296.
- Straathof KC, Bollard CM, Popat U, Huls MH, Lopez T, Morriss MC, Gresik MV, Gee AP, Russell HV, Brenner MK, et al. Treatment of nasopharyngeal carcinoma with Epstein-Barr virus–specific T lymphocytes. Blood. 2005;105(5):1898–1904. doi:10.1182/blood-2004-07-2975.
- Kelly E, Russell SJ. History of oncolytic viruses: genesis to genetic engineering. Mol Ther. 2007;15:651–659. doi:10.1038/sj.mt.6300108.
- Pol J, Lévesque S, Workenhe S, Gujar S, Le Boeuf F, Clements D, Bell JC, Mossman KL, Fucikova J, Spisek R, et al. Oncolytic viro-immunotherapy of hematologic and solid tumors. OncoImmunology. 2018;7. doi:10.1080/2162402X.2018.1503032.
- Gujar S, Bell J, Diallo JS. SnapShot: cancer immunotherapy with oncolytic viruses. Cell. 2019;176:1240–1240.e1. doi:10.1016/j.cell.2019.01.051.
- Borrie AE, Maleki Vareki S. T lymphocyte-based cancer immunotherapeutics. Int Rev Cell Mol Biol. 2018;341:201–276.
- Galluzzi L, Chan TA, Kroemer G, Wolchok JD, Lopez-Soto A. The hallmarks of successful anticancer immunotherapy. Sci. Transl. Med. 2018;10:eaat7807. doi:10.1126/scitranslmed.aat7807.
- Melero IP, Gato M, Shekarian T, Aznar A, Valsesia-Wittmann S, Caux C, Etxeberrria I, Teijeira A, Marabelle A. Repurposing infectious disease vaccines for intratumoral immunotherapy. J Immunother Cancer. 2020;8:e000443. doi:10.1136/jitc-000443.
- Rockx B, Kuiken T, Herfst S, Bestebroer T, Lamers MM, Oude Munnink BB, de Meulder D, van Amerongen G, van den Brand J, Okba NMA, et al. Comparative pathogenesis of COVID-19, MERS, and SARS in a nonhuman primate model. Science. 2020;368:1012–1015. doi:10.1126/science.abb7314.