ABSTRACT
Resiquimod (R848) and motolimod (VTX-2337) are second-generation experimental derivatives of imiquimod, an imidazoquinoline with immunostimulatory properties originally approved by the US Food and Drug Administration for the topical treatment of actinic keratosis and genital warts more than 20 years ago. Both resiquimod and motolimod operate as agonists of Toll-like receptor 7 (TLR7) and/or TLR8, in thus far delivering adjuvant-like signals to antigen-presenting cells (APCs). In line with such an activity, these compounds are currently investigated as immunostimulatory agents for the treatment of various malignancies, especially in combination with peptide-based, dendritic cell-based, cancer cell lysate-based, or DNA-based vaccines. Here, we summarize preclinical and clinical evidence recently collected to support the development of resiquimod and motolimod and other TLR7/TLR8 agonists as anticancer agents.
Introduction
Immunotherapy with immune checkpoint blockers (ICBs) such as ipilimumab, which targets cytotoxic T lymphocyte-associated protein 4 (CTLA4), as well as nivolumab and pembrolizumab, both of which target programmed cell death 1 (PDCD1, best known as PD-1), has literally revolutionized the clinical management of a variety of tumors, including (but not limited to) melanoma, nonsmall cell lung carcinoma (NSCLC) and urothelial carcinoma, and it holds promise to benefit an even higher amount of patients, either alone or in the context of combinatorial regimens, over the next few years.Citation1-Citation8 Nonetheless, attention on alternative immunotherapeutic approaches has remained high, at least in part reflecting: (1) the relatively small fraction of patients responding to ICBs employed as single agents;Citation9-Citation11 (2) the considerable side effects associated with some ICBs, especially when combined with each other;Citation12-Citation15 and (3) the economic burden of ICB-based immunotherapy.Citation16,Citation17 Besides monoclonal antibodies that operate as agonists of co-stimulatory receptors on immune effector cells,Citation18-Citation20 such immunotherapeutic agents include small molecules that trigger Toll-like receptor (TLR) signaling,Citation21-Citation29 de facto providing antigen-presenting cells (APCs) such as dendritic cells (DCs)Citation30-Citation32 with adjuvant-like, maturation signals that support the initiation of anticancer immunity (rather than tolerance).Citation22,Citation33-Citation38
TLRs are evolutionary conserved pattern recognition receptors (PRRs) localized at the cell surface or in endosomal compartments, and act as key mediators of innate immunity.Citation26,Citation39-Citation43 As a family, TLRs play a crucial role in ensuring a first line of defense against pathogens, largely based on their ability to respond to conserved microbial structures commonly referred to as microbe-associated molecular patterns (MAMPs) by initiating the secretion of bioactive factors including numerous cytokines.Citation40,Citation44-Citation47 Alongside this pristine antimicrobial function, TLRs also respond to a number of endogenous cues commonly referred to damage-associated molecular patterns (DAMPs), which are critical for the detection of cell stress and death as immunogenic in sterile conditions.Citation48-Citation52 As of today, no less than 13 TLRs have been identified in mammals (10 in humans),Citation43,Citation53 each of which exhibits (at least some) degree of specificity for selected MAMPs or DAMPs.Citation43,Citation53,Citation54 Thus, while bacterial lipopolysaccharide generally operates as a mixed TLR2/TLR4 agonist,Citation55-Citation58 CpG-rich oligodeoxynucleotides initiate immune signaling upon binding to TLR9.Citation59-Citation61 However, while surface-exposed TLRs (i.e., TLR1, TLR2, TLR4, TLR5, TLR6, and TLR10) as a group recognize a panel of chemical entities, including (but not limited to) proteins, lipoproteins, and lipopolysaccharides, endosomal TLRs (i.e., TLR3, TLR7, TLR8, TLR9), generally respond to (both microbial and endogenous) nucleic acids.Citation21,Citation24,Citation62 In this setting, TLR signaling is largely governed by ligand (1) availability, (2) localization, and (3) structure.Citation43,Citation63 On activation, all TLRs but TLR3 signal via a molecular mechanism involving MYD88 innate immune signal transduction adaptor (MYD88), which culminates in the NF-κB-dependent or interferon regulatory factor 3 (IRF3)-dependent secretion of pro-inflammatory cytokines.Citation64-Citation66 Conversely, TLR3 obligatorily drives cytokine synthesis via toll like receptor adaptor molecule 1 (TICAM1, best known as TRIF).Citation67,Citation68
Over the past two decades, the potent immunostimulatory effects of TLRs have spurred efforts aimed at the development of TLR agonists as anticancer agents, especially (but not exclusively) for use as immunological adjuvants to DC-based,Citation69-Citation71 peptide-based,Citation72,Citation73 or DNA-based therapeutic vaccines.Citation73,Citation74 TLR7 and TLR8, which respond to single-stranded RNA rich in uridine and guanosine have been considered as promising targets in this sense, at least in part reflecting the notion that the TLR7 agonist imiquimod has been approved for use as topical standalone agents in subject with actinic keratosis and genital warts as early as in 1997.Citation75,Citation76 In this context, two imiquidazoline derivatives of imiquimod, namely resiquimod (a mixed TLR7/8 agonist also known as R848) and motolimod (a TLR8 agonist also known as VTX-2337) have been shown to mediate promising immunostimulatory activity in a variety of preclinical models,Citation77,Citation78 including models of anticancer immunotherapy.Citation79,Citation80 However, neither of these agents has yet been approved by regulatory agencies for use in cancer patients. Along similar lines, a few third-generation TLR7/TLR8 agonists has entered clinical development for the treatment of viral infection or cancer based on promising preclinical results, including PF-4878691, BDC-1001, LHC165, NKTR-262, TQ-A3334, RO7119929, DSP-0509, BNT411, and NJH395Citation81-Citation91 However, none of these molecules has obtained regulatory approval for use in humans so far.
Here, we summarize recent preclinical and clinical data on the ability of these TLR7 and/or TLR8 agonists to (re)instate anticancer immunosurveillance, as we delineate the current portfolio of clinical trials testing resiquimod and motolimod in patients with cancer.
Recent preclinical developments
Since the publication of the latest Trial Watch dealing with experimental TLR7/TLR8 agonists for cancer therapy (October 2018),Citation92 a number of preclinical studies on this topic have been published or presented at major international conferences on cancer (immune)therapy. We found the following selected reports of particular interest.
Masud Alam and collaborators (National Cancer Institute, Frederick, MD, USA) showed that the TH1-polarizing alarm high mobility group nucleosome binding domain 1 (HMGN1) and resiquimod synergize at driving the functional maturation of human monocyte-derived DCs (MoDCs) and mouse bone marrow-derived DCs (BMDCs),Citation93 which is accompanied by NF-κB activation,Citation92 upregulation of DC surface proteins including CD80, CD83, CD86, and HLA-DR, and production of pro-inflammatory cytokines such as interleukin (IL)-12, IL-1β, and tumor necrosis factor (TNF).Citation92 Moreover, HMGN1 and resiquimod were found to synergize at triggering the nuclear translocation of interferon regulatory factor 3 (IRF3) and IRF7,Citation92 and hence at driving the secretion of type I IFN from DCs.Citation92 Consistently, transcriptomic studies of human MoDCs revealed that the combination of HMGN1 and resiquimod synergistically upregulate the expression of genes involved in DC maturation and the polarization of immune responses toward a TH1 profile.Citation92
Ryan et al. (Washington State University, Pullman, WA, USA) developed an enzyme-directed immunostimulant (EDI) prodrug of resiquimod, which can be metabolized to the active drug by cancer cells and ultimately released into the tumor microenvironment to mediate immunostimulatory effects.Citation94 This agent exhibited immunostimulatory activity in a panel of transplantable mouse models of melanoma (B16 cells), prostate carcinoma (TC2 cells), and breast carcinoma (4T1 cells).Citation94 Lu and colleagues (University of Kansas, Lawrence, KS, USA) tested yet another prodrug-based nanocarrier delivery system for resiquimod.Citation92 Specifically, resiquimod was linked with α-tocopherol to generate a prodrug that was further modified with hyaluronic acid into a polymeric nano-suspension (HA-Toco).Citation92 In vitro studies demonstrated that this delivery system prolongs the kinetics of resiquimod release while maintaining its immunostimulatory activity.Citation92 When administered subcutaneously to rabbits, the nano-suspension formed a depot at the injection site, inducing localized immune responses without systemic expansion. This formulation also suppressed the growth of oral squamous cell carcinoma (OSCC) AT84 cells growing in immunocompetent syngeneic C3 H mice, correlating with the recruitment of CD8A+ and CD11b+ immune cells to the tumor bed.Citation92 Finally, HA-Toco induced a 67% response rate (three partial remissions and one complete remission) in dogs spontaneously developing mast cell tumors.Citation92 Lu and coauthors (Tianjin University, Tianjin, China) designed nanoparticles (NPs) based on polydopamine (PDA) loaded with resiquimod and a fluorescent agent that can be released by photothermal therapy, which they dubbed PDA-PEG-R848-CD NPs.Citation95 Upon exposure to photothermal therapy, this nanomedicine not only was able to eradicate mouse 4T1 mammary tumors established in immunocompetent BALB/c mice but also prevented metastatic dissemination to the lungs and liver and mediated abscopal effectsCitation92 on distant, nonirradiated lesions, suggesting a potential for combination with ICBs.Citation95
Liu and collaborators (Southern Medical University, Guangzhou, China) found that resiquimod reversed the inhibitory effect of oxaliplatin, an immunogenic chemotherapeutic agents commonly employed for the therapy of colorectal carcinoma,Citation92 on the differentiation of myeloid-derived suppressor cells (MDSCs) into M1-like macrophages,Citation92 and enhanced its cytotoxic effect on malignant cells to limit the growth of mouse colorectal carcinoma CT26 cells in vivo.Citation92 Along similar lines, Rodel et al. (Harvard Medical School, Boston, MA, USA) identified resiquimod as a potent driver of M1 macrophage polarization in a morphometry-based screen for drugs capable to reeducate macrophages, and showed that resiquimod-loaded β-cyclodextrin nanoparticles (CDNP-R848) efficiently deliver resiquimod to tumor-associated macrophages (TAMs) in C57BL/6 mice bearing MC38 colorectal tumors.Citation92 Moreover, CDNP-R848 altered the functional orientation of TAMs toward an M1 phenotype in vivo, culminating only with a decrease in tumor growth but also with the establishment of long-term immunological memory.Citation92 Taken together, these results suggest that chemoresistance to oxaliplatin might arise from the suppression of M1 differentiation in the tumor microenvironment, and that resiquimod constitutes a promising immunological adjuvant for oxaliplatin-based chemotherapy.
Michaelis and colleagues (Oregon Health & Science University, Portland, OR, USA) showed that resiquimod induces antitumor responses and attenuates cachexia in syngeneic orthotopic murine models of pancreatic ductal adenocarcinoma (PDAC).Citation92 This study revealed that resiquimod acts on both the host and the tumor to enable disease control. In particular, behavioral and molecular phenotyping on tumor-bearing mice delineated a resiquimod-mediated decrease in manifestations of cachexia,Citation92 resulting in a nearly doubled overall survival for tumor-bearing mice.Citation92 Moreover, tumors arising from two out of three PDAC cell lines responded to resiquimod with a decrease in growth and an increase in immune complexity, an increase in CD8+ T-cell infiltration and a decrease in the intratumoral frequency of CD4+CD25+FOXP3+ regulatory T (TREG) cells.Citation92 Finally, stromal (not neoplastic) TLR7 was a prerequisite for resiquimod responses, which is in line with TLR7 expression by stromal but not epithelial tissue from patient samples.Citation92 These data point to the possibility that resiquimod may be useful for the treatment of cachexia in patients with PDAC.
Mullins and collaborators (AstraZeneca Ltd, Cambridge, UK) harnessed quantitative methods including mass spectrometry imaging to demonstrate that the lipophilic TLR7/8 agonist MEDI9197 (also known as 3 M-052) is retained at the injection site in mouse bearing syngeneic B16-OVA melanoma cells, resulting in limited systemic exposure.Citation92 MEDI9197 injection led to promoted a TH1 polarized immune response accompanied by activation of natural killer (NK) and CD8+ T cells,Citation96 secretion of a broad range of cytokines including type I IFN, IL-12 and interferon gamma (IFNG) and inhibition of tumor growth.Citation92 These beneficial effects could be further boosted by the co-administration of immunostimulatory antibodiesCitation92 specific for TNF receptor superfamily member 4 (TNFRSF4, best known as OX40) and TNFRSF18 (best known as GITR).Citation92
Yosuke et al. (Sumitomo Dainippon Pharma Co., Ltd, Osaka, Japan) showed that DSP-0509 delivered i.v. efficiently controls the growth of mouse tumors with high mutational burden, robust CD8+ T cell infiltration and a globally inflamed microenvironment, such as colorectal carcinoma CT26 cells, but not tumors with low mutational rates, limited T-cell recruitment, and a globally suppressive microenvironment, such as mammary carcinoma 4T1 cells.Citation97 Conversely, the combination of DSP-0509 with ICBs targeting CTLA4 or PD-1 was effective in both models, consistent with an increased expression of genes linked to immune effector functions like IFNG, and a decreased in immunosuppressive cell populations including neutrophils and polymorphonuclear MDSCs.Citation97 This study suggest that DSP-0509 can be employed to convert immunologically “cold,” ICB-resistant tumors into immunologically “hot” lesions that respond to ICBs.
Finally, Ackerman et al. (Bolt Biotherapeutics, Redwood City, CA, USA) developed an immune-stimulating antibody conjugate (ISAC) composed of a TLR7/8 agonist conjugated to a tumor-targeting monoclonal antibody.Citation98 Cocultures of human leukocytes and cancer cell lines demonstrated that this ISAC can activate APCs, resulting in upregulation of costimulatory molecules, secretion of proinflammatory cytokines, as well as effector functions such as antibody-dependent cellular cytotoxicity (ADCC) and antibody-dependent cellular phagocytosis (ADCP).Citation98 Moreover, in syngeneic tumor models, ISAC administration mediated robust therapeutic effects coupled to the establishment of immunological memory.
Altogether, these studies suggest that considerable efforts are currently being dedicated to the development of delivery platform for TLR7/TLR8 agonists that enable robust local activity in the absence of systemic exposure.
Completed clinical trials
Since the publication of the latest Trial Watch dealing with TLR7/TLR8 agonists (October 2018),Citation92 the results of only two clinical studies testing these agents for the treatment of cancer patients have been published in the peer-reviewed literature.
Topical resiquimod has been tested as a standalone therapeutic intervention in 217 patients with actinic keratosis, a common precancerous lesion of the skin that is linked to excessive sun exposure,Citation78,Citation99,Citation100 with the purpose of determining optimal dose with respect to efficacy, safety and tolerability (NCT01583816).Citation92 Patients were randomize to daily 0.01% or 0.03% resiquimod cream in five different treatment schedules until skin erosion or up to 8 weeks. Complete clinical clearance was obtained in up 56% to 85% of patients, depending on study group, with maximal efficacy being observed among individual receiving the 0.03% formulation.Citation92 One hundred and twenty-eight patients experience treatment-related adverse effects (TRAEs), the majority of which was mild.Citation92
Motolimod has been tested as an adjuvant to cytotoxic chemotherapy in a Phase 2 clinical trial enrolling 195 adults with histologically confirmed recurrent or metastatic head and neck squamous cell carcinoma (HNSCC) (NCT01836029).Citation101,Citation102 In this setting, motolimod was well tolerated but failed to improve the therapeutic activity of the so-called “EXTREME” regimen,Citation102 consisting of a platinum derivative (generally carboplatin or cisplatin),Citation103-Citation107 fluorouracil and cetuximabCitation108-Citation110 However, patients with human papillomavirus (HPV)+ tumors as well as individuals experiencing injection site reactions upon motolimod administration experience improved disease outcome in response to the combinatorial regimen,Citation102 suggesting that this approach could at least benefit specific subsets of HNSCC patients.
The limited number of original reports on clinical studies investigating TLR7 and TLR8 agonists for cancer therapy points to a somehow decreased interest in this therapeutic paradigm, potentially linked to the emergence of other promising immunotherapeutic agents including ICBs,Citation2,Citation4 oncolytic viruses,Citation111,Citation112 and CAR T cells.Citation113,Citation114
Ongoing clinical trials
Since the submission of the latest Trial Watch dealing with this topic (October 2018),Citation22 only 10 clinical studies involving the administration of TLR7 (6 studies), TLR8 (2 studies), or mixed TLR7/TLR8 (2 studies) agonists to cancer patients have been initiated () (source https://www.clinicaltrials.gov).
Table 1. Clinical trials testing experimental TLR7 and TLR8 agonists in cancer patients*.
At least 6 different TLR7 agonists other than resiquimod have recently entered clinical development. Thus, the tolerability, safety, pharmacodynamics, pharmacokinetics, and preliminary activity profile of RO7119929 administered as standalone therapeutic intervention are being evaluated in patients with unresectable advanced or metastatic hepatocellular carcinoma (HCC), biliary tract cancer, or other solid tumors with predominant liver involvement and metastases (NCT04338685). Similarly, the erb-b2 receptor tyrosine kinase 2 (ERBB2, best known as HER2)-targeted TLR7 agonistic ISAC NJH395Citation115,Citation116 is being tested as monotherapy in patients with nonbreast HER2+ advanced solid neoplasms (NCT03696771). Along similar lines, the HER2-targeted TLR7/TLR8 mixed agonist ISAC BDC-1001 is being evaluated in a first-in-human trials enrolling individuals with HER2+ breast and gastric carcinoma (NCT04278144). This study is planned over four distinct phases aiming at: identifying the maximum tolerated dose (MTD) of BDC-1001 as monotherapy (phase 1) or combined with the PD-1 blocker pembrolizumab (phase 2), and testing preliminary efficacy of BD-1001 as standalone intervention (phase 3) or in combination with pembrolizumab (phase 4).
The Phase I/IIa dose-escalation clinical trial NCT04101357 is assessing the efficacy, pharmacodynamics, pharmacokinetics and safety of BNT411, a novel TLR7 agonist, either given as a monotherapy in individuals affected by advanced solid tumors or administered in combination with the PD-L1-targeted ICB atezolizumab,Citation3,Citation117-Citation119 carboplatin and etoposideCitation120,Citation121 in patients with chemotherapy-naïve extensive-stage small cell lung cancer (ES-SCLC). The synthetic TLR7 agonist DSP-0509 is being investigated for pharmacokinetic profile, tolerability, and safety upon intravenous administration as monotherapy or combined with pembrolizumab,Citation122-Citation124 in adults with advanced solid malignancies (NCT03416335). Similar approaches under clinical testing involve the hitherto experimental PD-1-targeting agent spartalizumab (also known as PDR001)Citation125-Citation128 and the TLR7 agonistic benzonapthyridine LHC165,Citation92 (NCT03301896), the PD-1-specific ICB nivolumab and the mixed TLR7/TLR8 agonist NKTR-262,Citation129 (NCT03435640), as well as nivolumab and motolimod (NCT04272333, NCT03906526). NCT03301896 consists of four dose escalation-phases followed by two expansion phases and is expected to include approximately 206 patients with advanced or metastatic solid tumors. In the context of NCT03435640, patients suffering from advanced solid neoplasms are planned to receive NKTR-262 in combination with NKTR-214 (a genetically engineered version of IL-2 specifically targeted to interleukin 2 receptor subunit beta, IL2RB)Citation130 ± nivolumab. NCT04272333 aims at exploring the therapeutic profile and immunogenicity of nivolumab plus motolimod delivered i.t. in microdosesCitation131-Citation134 to patients with HNSCC. Finally, the pharmacokinetics, tolerance, effectiveness, dose-limiting toxicity (DLT) and MTD of the highly selective TLR7 agonist TQ-A3334 are being assessed in the dose-escalation/expansion study NCT04273815. In this setting, patients with NSCLC will TQ-A3334 p.o. as monotherapy or in combination with the multitarget receptor tyrosine kinase inhibitor anlotinib.Citation135-Citation137
The status of the following clinical trials discussed in our previous Trial Watches dealing with TLR7 and TLR8 agonists,Citation22 has changed during the past 20 months: NCT00960752 which is now listed as “Active, not recruiting”; NCT00821652, NCT00948961, NCT01294293, NCT01334177, NCT01583816 and NCT01748747 which are listed as “Completed”; as well as NCT02124850, NCT02650635, NCT01094496, NCT01808950, and NCT01289210, which have been “Terminated”, due to sponsor request (NCT02650635), slow enrollment (NCT01094496 and NCT01289210) or safety issues (NCT01808950) (source https://www.clinicaltrials.gov/). To the best of our knowledge, the results of the aforementioned completed trials have not been released yet, with the exception of NCT01583816 and NCT01836029 (see above).
Concluding remarks
In conclusion, the development of resiquimod, motolimod and other TLR7/TLR8 agonists as immunostimulatory agents for use in cancer patients appears to stand at an impasse, at least in part reflecting the disappointing results obtained in recent clinical tests. It is tempting to speculate that the limited clinical success of therapeutic cancer vaccines adjuvanted with TLR7/TLR8 agonists may not (entirely) originate from suboptimal TLR stimulation, but rather reflect limitations that are intrinsic to DC-based, peptide-based, and DNA-based vaccines ().Citation138,Citation139 The identification of therapeutic strategies that circumvent resistance mechanisms such as antigen or beta-2-microglobulin (B2 M) loss,Citation140,Citation141 the robust immunosuppressive circuitries that characterize most solid tumors,Citation142-Citation144 as well as the loss of key proteins that underlie cancer cell sensitivity to immune effectors, such as caspase 8 (CASP8) and Janus kinase 2 (JAK2),Citation145,Citation146 may therefore pave the way to novel approaches that will ultimately benefit from TLR7/TLR8 agonism. Additional work in this sense is urgently awaited.
Figure 1. Current obstacles against the development of efficacious TLR7/TLR8 agonists for cancer therapy. Besides being hampered by specificity issues, the translation of currently available TLR7/TLR8 agonists to the clinic is limited by incomplete data on optimal delivery routes and administration schedules, as well as by the intrinsic issues associated with therapeutic cancer vaccines.
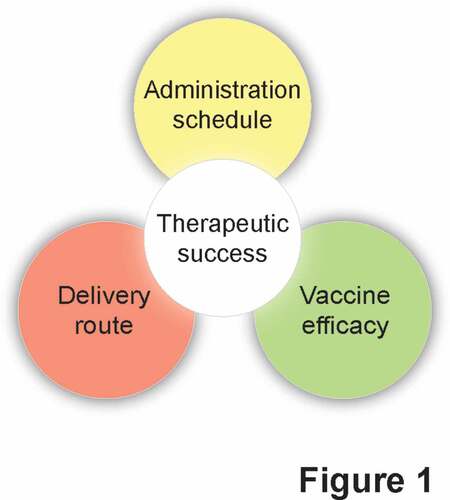
Abbreviations
ADCC | = | Antibody-dependent cell-mediated cytotoxicity |
ADCP | = | Antibody-dependent cellular phagocytosis |
AK | = | Actinic keratosis |
APC | = | Antigen presenting cell |
DAMP | = | Damage-associated molecular pattern |
DC | = | Dendritic cell |
DLT | = | Dose-limiting toxicity |
HNSCC | = | Head and neck squamous cell carcinoma |
HPV | = | Human papilloma virus |
HSV | = | Herpes simplex virus |
ICB | = | Immune checkpoint blocker |
IFN | = | Interferon |
IL | = | Interleukin |
ISAC | = | Immune stimulating antibody conjugate |
MAMP | = | Microbe-associated molecular pattern |
MTD | = | Maximum tolerated dose |
PLD | = | Pegylated liposomal doxorubicin |
PRR | = | Pattern recognition receptor |
TLR | = | Toll-like receptor |
Disclosure of Potential Conflicts of Interest
No potential conflicts of interest were disclosed.
Acknowledgments
LG is supported by a Breakthrough Level 2 grant from the US Department of Defense (DoD), Breast Cancer Research Program (BRCP) (#BC180476P1), by the 2019 Laura Ziskin Prize in Translational Research (#ZP-6177, PI: Formenti) from the Stand Up to Cancer (SU2C), by a Mantle Cell Lymphoma Research Initiative (MCL-RI, PI: Chen-Kiang) grant from the Leukemia and Lymphoma Society (LLS), by a startup grant from the Dept. of Radiation Oncology at Weill Cornell Medicine (New York, US), by a Rapid Response Grant from the Functional Genomics Initiative (New York, US), by industrial collaborations with Lytix (Oslo, Norway) and Phosplatin (New York, US), and by donations from Phosplatin (New York, US), the Luke Heller TECPR2 Foundation (Boston, US) and Sotio a.s. (Prague, Czech Republic). GK is supported by the Ligue contre le Cancer (équipe labellisée); Agence National de la Recherche (ANR) – Projets blancs; ANR under the frame of E-Rare-2, the ERA-Net for Research on Rare Diseases; AMMICa US23/CNRS UMS3655; Association pour la recherche sur le cancer (ARC); Association “Le Cancer du Sein, Parlons-en!”; Cancéropôle Ile-de-France; Chancelerie des universités de Paris (Legs Poix), Fondation pour la Recherche Médicale (FRM); a donation by Elior; European Research Area Network on Cardiovascular Diseases (ERA-CVD, MINOTAUR); Gustave Roussy Odyssea, the European Union Horizon 2020 Project Oncobiome; Fondation Carrefour; High-end Foreign Expert Program in China (GDW20171100085), Institut National du Cancer (INCa); Inserm (HTE); Institut Universitaire de France; LeDucq Foundation; the LabEx Immuno-Oncology (ANR-18- IDEX-0001); the RHU Torino Lumière; the Seerave Foundation; the SIRIC Stratified Oncology Cell DNA Repair and Tumor Immune Elimination (SOCRATE); and the SIRIC Cancer Research and Personalized Medicine (CARPEM).
References
- Galluzzi L, Chan TA, Kroemer G, Wolchok JD, Lopez-Soto A. The hallmarks of successful anticancer immunotherapy. Sci Transl Med. 2018;10:eaat7807. doi:10.1126/scitranslmed.aat7807.
- Sharma P, Allison JP. The future of immune checkpoint therapy. Science. 2015;348:56–10. doi:10.1126/science.aaa8172.
- Vitale I, Sistigu A, Manic G, Rudqvist N-P, Trajanoski Z, Galluzzi L. Mutational and antigenic landscape in tumor progression and cancer immunotherapy. Trends Cell Biol. 2019;29:396–416. doi:10.1016/j.tcb.2019.01.003.
- Sharma P, Allison JP. Immune checkpoint targeting in cancer therapy: toward combination strategies with curative potential. Cell. 2015;161:205–214. doi:10.1016/j.cell.2015.03.030.
- Xu W, Atkins MB, McDermott DF. Checkpoint inhibitor immunotherapy in kidney cancer. Nat Rev Urol. 2020;17:137–150. doi:10.1038/s41585-020-0282-3.
- Sharma P, Allison JP. Dissecting the mechanisms of immune checkpoint therapy. Nat Rev Immunol. 2020;20:75–76. doi:10.1038/s41577-020-0275-8.
- Petroni G, Formenti SC, Chen-Kiang S, Galluzzi L. Immunomodulation by anticancer cell cycle inhibitors. Nat Rev Immunol. 2020. doi:10.1038/s41577-020-0300-y.
- Rodriguez-Ruiz ME, Vitale I, Harrington KJ, Melero I, Galluzzi L. Immunological impact of cell death signaling driven by radiation on the tumor microenvironment. Nat Immunol. 2020;21:120–134. doi:10.1038/s41590-019-0561-4.
- Kalbasi A, Ribas A. Tumour-intrinsic resistance to immune checkpoint blockade. Nat Rev Immunol. 2020;20:25–39. doi:10.1038/s41577-019-0218-4.
- Chen DS, Mellman I. Elements of cancer immunity and the cancer-immune set point. Nature. 2017;541:321–330. doi:10.1038/nature21349.
- Palucka AK, Coussens LM. The basis of oncoimmunology. Cell. 2016;164:1233–1247. doi:10.1016/j.cell.2016.01.049.
- June CH, Warshauer JT, Bluestone JA. Is autoimmunity the Achilles’ heel of cancer immunotherapy? Nat Med. 2017;23:540–547. doi:10.1038/nm.4321.
- Esfahani K, Calabrese L. Moving towards personalized treatments of immune-related adverse events. Nat Rev Clin Oncol. 2020. doi:10.1038/s41571-020-0352-8.
- Ramos-Casals M, Brahmer JR, Callahan MK, Flores-Chávez A, Keegan N, Khamashta MA, Lambotte O, Mariette X, Prat A, Suárez-Almazor ME, et al. Immune-related adverse events of checkpoint inhibitors. Nat Rev Dis Primers. 2020;6:38. doi:10.1038/s41572-020-0160-6.
- Herrmann J. Adverse cardiac effects of cancer therapies: cardiotoxicity and arrhythmia. Nat Rev Cardiol. 2020;17:474–502. doi:10.1038/s41569-020-0348-1.
- Yu PP, Eton O, Garrison LP. Challenges in assessing the clinical utility and economic value of immune checkpoint inhibitor therapies of Cancer. J Immunother Cancer. 2019;7:235. doi:10.1186/s40425-019-0707-9.
- Chu JN, Choi J, Ostvar S, Torchia JA, Reynolds KL, Tramontano A, Gainor JF, Chung DC, Clark JW, Hur C, et al. Cost-effectiveness of immune checkpoint inhibitors for microsatellite instability-high/mismatch repair-deficient metastatic colorectal cancer. Cancer. 2019;125:278–289. doi:10.1002/cncr.31795.
- Melero I, Hervas-Stubbs S, Glennie M, Pardoll DM, Chen L. Immunostimulatory monoclonal antibodies for cancer therapy. Nat Rev Cancer. 2007;7:95–106. doi:10.1038/nrc2051.
- Levesque S, Le Naour J, Pietrocola F, Paillet J, Kremer M, Castoldi F, Baracco EE, Wang Y, Vacchelli E, Stoll G, et al. A synergistic triad of chemotherapy, immune checkpoint inhibitors, and caloric restriction mimetics eradicates tumors in mice. Oncoimmunology. 2019;8:e1657375. doi:10.1080/2162402X.2019.1657375.
- Ahern E, Harjunpää H, O’Donnell JS, Allen S, Dougall WC, Teng MWL, Smyth MJ. RANKL blockade improves efficacy of PD1-PD-L1 blockade or dual PD1-PD-L1 and CTLA4 blockade in mouse models of cancer. Oncoimmunology. 2018;7:e1431088. doi:10.1080/2162402X.2018.1431088.
- Medler T, Patel JM, Alice A, Baird JR, Hu HM, Gough MJ. Activating the nucleic acid-sensing machinery for anticancer immunity. Int Rev Cell Mol Biol. 2019;344:173–214. doi:10.1016/bs.ircmb.2018.08.006.
- Smith M, García-Martínez E, Pitter MR, Fucikova J, Spisek R, Zitvogel L, Kroemer G, Galluzzi L. Trial watch: toll-like receptor agonists in cancer immunotherapy. Oncoimmunology. 2018;7:e1526250. doi:10.1080/2162402X.2018.1526250.
- Pastor F, Berraondo P, Etxeberria I, Frederick J, Sahin U, Gilboa E, Melero I. An RNA toolbox for cancer immunotherapy. Nat Rev Drug Discov. 2018;17:751–767. doi:10.1038/nrd.2018.132.
- Vanpouille-Box C, Hoffmann JA, Galluzzi L. Pharmacological modulation of nucleic acid sensors - therapeutic potential and persisting obstacles. Nat Rev Drug Discov. 2019;18:845–867. doi:10.1038/s41573-019-0043-2.
- Khodarev NN. Intracellular RNA sensing in mammalian cells: role in stress response and cancer therapies. Int Rev Cell Mol Biol. 2019;344:31–89. doi:10.1016/bs.ircmb.2018.08.005.
- Heil M, Vega-Munoz I. Nucleic acid sensing in mammals and plants: facts and caveats. Int Rev Cell Mol Biol. 2019;345:225–285. doi:10.1016/bs.ircmb.2018.10.003.
- Guha M. Anticancer TLR agonists on the ropes. Nat Rev Drug Discov. 2012;11:503–505. doi:10.1038/nrd3775.
- Vascotto F, Petschenka J, Walzer KC, Vormehr M, Brkic M, Strobl S, Rösemann R, Diken M, Kreiter S, Türeci Ö, et al. Intravenous delivery of the toll-like receptor 7 agonist SC1 confers tumor control by inducing a CD8+ T cell response. Oncoimmunology. 2019;8:1601480. doi:10.1080/2162402X.2019.1601480.
- Hennessy EJ, Parker AE, O’Neill LA. Targeting toll-like receptors: emerging therapeutics? Nat Rev Drug Discov. 2010;9:293–307. doi:10.1038/nrd3203.
- Wculek SK, Cueto FJ, Mujal AM, Melero I, Krummel MF, Sancho D. Dendritic cells in cancer immunology and immunotherapy. Nat Rev Immunol. 2020;20:7–24. doi:10.1038/s41577-019-0210-z.
- Sprooten J, Agostinis P, Garg AD. Type I interferons and dendritic cells in cancer immunotherapy. Int Rev Cell Mol Biol. 2019;348:217–262. doi:10.1016/bs.ircmb.2019.06.001.
- Lee YS, Radford KJ. The role of dendritic cells in cancer. Int Rev Cell Mol Biol. 2019;348:123–178. doi:10.1016/bs.ircmb.2019.07.006.
- Blander JM. Regulation of the cell biology of antigen cross-presentation. Annu Rev Immunol. 2018;36:717–753. doi:10.1146/annurev-immunol-041015-055523.
- Cruz FM, Colbert JD, Merino E, Kriegsman BA, Rock KL. The biology and underlying mechanisms of cross-presentation of exogenous antigens on MHC-I molecules. Annu Rev Immunol. 2017;35:149–176. doi:10.1146/annurev-immunol-041015-055254.
- Unanue ER, Turk V, Neefjes J. Variations in MHC class II antigen processing and presentation in health and disease. Annu Rev Immunol. 2016;34:265–297. doi:10.1146/annurev-immunol-041015-055420.
- Kotsias F, Cebrian I, Alloatti A. Antigen processing and presentation. Int Rev Cell Mol Biol. 2019;348:69–121. doi:10.1016/bs.ircmb.2019.07.005.
- Vanmeerbeek I, Sprooten J, De Ruysscher D, Tejpar S, Vandenberghe P, Fucikova J, Spisek R, Zitvogel L, Kroemer G, Galluzzi L, et al. Trial watch: chemotherapy-induced immunogenic cell death in immuno-oncology. Oncoimmunology. 2020;9:1703449. doi:10.1080/2162402X.2019.1703449.
- Matzinger P. The danger model: a renewed sense of self. Science. 2002;296:301–305. doi:10.1126/science.1071059.
- Lupfer CR, Rippee-Brooks MD, Anand PK. Common differences: the ability of inflammasomes to distinguish between self and pathogen nucleic acids during infection. Int Rev Cell Mol Biol. 2019;344:139–172. doi:10.1016/bs.ircmb.2018.10.001.
- Tan X, Sun L, Chen J, Chen ZJ. Detection of microbial infections through innate immune sensing of nucleic acids. Annu Rev Microbiol. 2018;72:447–478. doi:10.1146/annurev-micro-102215-095605.
- Chow KT, Gale M Jr., Loo YM. RIG-I and other RNA sensors in antiviral immunity. Annu Rev Immunol. 2018;36:667–694. doi:10.1146/annurev-immunol-042617-053309.
- Burgueno JF, Abreu MT. Epithelial Toll-like receptors and their role in gut homeostasis and disease. Nat Rev Gastroenterol Hepatol. 2020;17:263–278. doi:10.1038/s41575-019-0261-4.
- Gong T, Liu L, Jiang W, Zhou R. DAMP-sensing receptors in sterile inflammation and inflammatory diseases. Nat Rev Immunol. 2020;20:95–112. doi:10.1038/s41577-019-0215-7.
- Choi HW, Klessig DF. DAMPs, MAMPs, and NAMPs in plant innate immunity. BMC Plant Biol. 2016;16:232. doi:10.1186/s12870-016-0921-2.
- Versluys M, Tarkowski LP, Van den Ende W. Fructans AS DAMPs or MAMPs: evolutionary prospects, cross-tolerance, and multistress resistance potential. Front Plant Sci. 2016;7:2061. doi:10.3389/fpls.2016.02061.
- Zitvogel L, Galluzzi L, Kepp O, Smyth MJ, Kroemer G. Type I interferons in anticancer immunity. Nat Rev Immunol. 2015;15:405–414. doi:10.1038/nri3845.
- McNab F, Mayer-Barber K, Sher A, Wack A, O’Garra A. Type I interferons in infectious disease. Nat Rev Immunol. 2015;15:87–103. doi:10.1038/nri3787.
- Galluzzi L, Vitale I, Warren S, Adjemian S, Agostinis P, Martinez AB, Chan TA, Coukos G, Demaria S, Deutsch E, et al. Consensus guidelines for the definition, detection and interpretation of immunogenic cell death. J Immunother Cancer. 2020;8. doi:10.1136/jitc-2019-000337.
- Pouwels SD, Heijink IH, Ten Hacken NH, Vandenabeele P, Krysko DV, Nawijn MC, van Oosterhout AJ. DAMPs activating innate and adaptive immune responses in COPD. Mucosal Immunol. 2014;7:215–226. doi:10.1038/mi.2013.77.
- Garg AD, Galluzzi L, Apetoh L, Baert T, Birge RB, Bravo-San Pedro JM, Breckpot K, Brough D, Chaurio R, Cirone M, et al. Molecular and translational classifications of DAMPs in immunogenic cell death. Front Immunol. 2015;6:588. doi:10.3389/fimmu.2015.00588.
- Krysko DV, Garg AD, Kaczmarek A, Krysko O, Agostinis P, Vandenabeele P. Immunogenic cell death and DAMPs in cancer therapy. Nat Rev Cancer. 2012;12:860–875. doi:10.1038/nrc3380.
- Galluzzi L, Buque A, Kepp O, Zitvogel L, Kroemer G. Immunogenic cell death in cancer and infectious disease. Nat Rev Immunol. 2017;17:97–111. doi:10.1038/nri.2016.107.
- Kawasaki T, Kawai T. Toll-like receptor signaling pathways. Front Immunol. 2014;5:461. doi:10.3389/fimmu.2014.00461.
- Iwasaki A, Medzhitov R. Regulation of adaptive immunity by the innate immune system. Science. 2010;327:291–295. doi:10.1126/science.1183021.
- Poltorak A, He X, Smirnova I, Liu MY, Van Huffel C, Du X, Birdwell D, Alejos E, Silva M, Galanos C, et al. Defective LPS signaling in C3H/HeJ and C57BL/10ScCr mice: mutations in Tlr4 gene. Science. 1998;282:2085–2088. doi:10.1126/science.282.5396.2085.
- Hirschfeld M, Ma Y, Weis JH, Vogel SN, Weis JJ. Cutting edge: repurification of lipopolysaccharide eliminates signaling through both human and murine toll-like receptor 2. J Immunol. 2000;165:618–622. doi:10.4049/jimmunol.165.2.618.
- Lippitz BE, Harris RA. A translational concept of immuno-radiobiology. Radiother Oncol. 2019;140:116–124. doi:10.1016/j.radonc.2019.06.001.
- Bryant CE, Spring DR, Gangloff M, Gay NJ. The molecular basis of the host response to lipopolysaccharide. Nat Rev Microbiol. 2010;8:8–14. doi:10.1038/nrmicro2266.
- Haas T, Metzger J, Schmitz F, Heit A, Müller T, Latz E, Wagner H. The DNA sugar backbone 2ʹ deoxyribose determines toll-like receptor 9 activation. Immunity. 2008;28:315–323. doi:10.1016/j.immuni.2008.01.013.
- Humbert M, Hugues S. Warming up the tumor microenvironment in order to enhance immunogenicity. Oncoimmunology. 2019;8:e1510710. doi:10.1080/2162402X.2018.1510710.
- Krieg AM. Therapeutic potential of Toll-like receptor 9 activation. Nat Rev Drug Discov. 2006;5:471–484. doi:10.1038/nrd2059.
- Roers A, Hiller B, Hornung V. Recognition of endogenous nucleic acids by the innate immune system. Immunity. 2016;44:739–754. doi:10.1016/j.immuni.2016.04.002.
- Schlee M, Hartmann G. Discriminating self from non-self in nucleic acid sensing. Nat Rev Immunol. 2016;16:566–580. doi:10.1038/nri.2016.78.
- Kawasaki T, Kawai T. Discrimination between self and non-self-nucleic acids by the innate immune system. Int Rev Cell Mol Biol. 2019;344:1–30. doi:10.1016/bs.ircmb.2018.08.004.
- Paludan SR, Reinert LS, Hornung V. DNA-stimulated cell death: implications for host defence, inflammatory diseases and cancer. Nat Rev Immunol. 2019;19:141–153. doi:10.1038/s41577-018-0117-0.
- O’Neill LA, Golenbock D, Bowie AG. The history of Toll-like receptors - redefining innate immunity. Nat Rev Immunol. 2013;13:453–460. doi:10.1038/nri3446.
- O’Neill LA, Bowie AG. The family of five: TIR-domain-containing adaptors in Toll-like receptor signalling. Nat Rev Immunol. 2007;7:353–364. doi:10.1038/nri2079.
- Farahnak S, Chronopoulos J, Martin JG. Nucleic acid sensing in allergic disorders. Int Rev Cell Mol Biol. 2019;345:1–33. doi:10.1016/bs.ircmb.2018.11.001.
- Vacchelli E, Vitale I, Eggermont A, Fridman WH, Fučíková J, Cremer I, Galon J, Tartour E, Zitvogel L, Kroemer G, et al. Trial watch: dendritic cell-based interventions for cancer therapy. Oncoimmunology. 2013;2:e25771. doi:10.4161/onci.25771.
- Kim YS, Park HJ, Park JH, Hong EJ, Jang G-Y, Jung ID, Han HD, Lee S-H, Vo M-C, Lee -J-J, et al. A novel function of API5 (apoptosis inhibitor 5), TLR4-dependent activation of antigen presenting cells. Oncoimmunology. 2018;7:e1472187. doi:10.1080/2162402X.2018.1472187.
- Berinstein NL. Enhancing cancer vaccines with immunomodulators. Vaccine. 2007;25(Suppl 2):B72–88. doi:10.1016/j.vaccine.2007.06.043.
- Bezu L, Kepp O, Cerrato G, Pol J, Fucikova J, Spisek R, Zitvogel L, Kroemer G, Galluzzi L. Trial watch: peptide-based vaccines in anticancer therapy. Oncoimmunology. 2018;7:e1511506. doi:10.1080/2162402X.2018.1511506.
- Di Lorenzo G, Buonerba C, Kantoff PW. Immunotherapy for the treatment of prostate cancer. Nat Rev Clin Oncol. 2011;8:551–561. doi:10.1038/nrclinonc.2011.72.
- Pierini S, Perales-Linares R, Uribe-Herranz M, Pol JG, Zitvogel L, Kroemer G, Facciabene A, Galluzzi L. Trial watch: DNA-based vaccines for oncological indications. Oncoimmunology. 2017;6:e1398878. doi:10.1080/2162402X.2017.1398878.
- Hoffman ES, Smith RE, Renaud RC Jr. From the analyst’s couch: TLR-targeted therapeutics. Nat Rev Drug Discov. 2005;4:879–880. doi:10.1038/nrd1880.
- Tyring S. Imiquimod applied topically: A novel immune response modifier. Skin Therapy Lett. 2001;6:1–4.
- Dietsch GN, Lu H, Yang Y, Morishima C, Chow LQ, Disis ML, Hershberg RM. Coordinated activation of toll-like receptor8 (TLR8) and NLRP3 by the TLR8 agonist, VTX-2337, ignites tumoricidal natural killer cell activity. PloS One. 2016;11:e0148764. doi:10.1371/journal.pone.0148764.
- Killock D. Haematological cancer: resiquimod-a topical CTCL therapy. Nat Rev Clin Oncol. 2015;12:563. doi:10.1038/nrclinonc.2015.142.
- Prins RM, Craft N, Bruhn KW, Khan-Farooqi H, Koya RC, Stripecke R, Miller JF, Liau LM. The TLR-7 agonist, imiquimod, enhances dendritic cell survival and promotes tumor antigen-specific T cell priming: relation to central nervous system antitumor immunity. J Immunol. 2006;176:157–164. doi:10.4049/jimmunol.176.1.157.
- Bialojan A, Sohl J, Rausch J, Aranda Lopez P, Denny M, Langguth P, Hartmann A-K, Yagita H, Probst HC, Schild H, et al. Transcutaneous immunization with CD40 ligation boosts cytotoxic T lymphocyte mediated antitumor immunity independent of CD4 helper cells in mice. Eur J Immunol. 2019;49:2083–2094. doi:10.1002/eji.201848039.
- Hanten JA, Vasilakos JP, Riter CL, Neys L, Lipson KE, Alkan SS, Birmachu W. Comparison of human B cell activation by TLR7 and TLR9 agonists. BMC Immunol. 2008;9:39. doi:10.1186/1471-2172-9-39.
- Weigel BJ, Cooley S, DeFor T, Weisdorf DJ, Panoskaltsis-Mortari A, Chen W, Blazar BR, Miller JS. Prolonged subcutaneous administration of 852A, a novel systemic toll-like receptor 7 agonist, to activate innate immune responses in patients with advanced hematologic malignancies. Am J Hematol. 2012;87:953–956. doi:10.1002/ajh.23280.
- Dudek AZ, Yunis C, Harrison LI, Kumar S, Hawkinson R, Cooley S, Vasilakos JP, Gorski KS, Miller JS. First in human phase I trial of 852A, a novel systemic toll-like receptor 7 agonist, to activate innate immune responses in patients with advanced cancer. Clin Cancer Res. 2007;13:7119–7125. doi:10.1158/1078-0432.CCR-07-1443.
- Fidock MD, Souberbielle BE, Laxton C, Rawal J, Delpuech-Adams O, Corey TP, Colman P, Kumar V, Cheng JB, Wright K, et al. The innate immune response, clinical outcomes, and ex vivo HCV antiviral efficacy of a TLR7 agonist (PF-4878691). Clin Pharmacol Ther. 2011;89:821–829. doi:10.1038/clpt.2011.60.
- Inglefield JR, Dumitru CD, Alkan SS, Gibson SJ, Lipson KE, Tomai MA, Larson CJ, Vasilakos JP. TLR7 agonist 852A inhibition of tumor cell proliferation is dependent on plasmacytoid dendritic cells and type I IFN. J Interferon Cytokine Res. 2008;28:253–263. doi:10.1089/jir.2007.0097.
- Astry C, Birmachu W, Harrison LI, Meng TC. Cutaneous pharmacodynamics of a toll-like receptor 7 agonist, 852A, in humans. J Clin Pharmacol. 2008;48:755–762. doi:10.1177/0091270008314466.
- Dummer R, Hauschild A, Becker JC, Grob -J-J, Schadendorf D, Tebbs V, Skalsky J, Kaehler KC, Moosbauer S, Clark R, et al. An exploratory study of systemic administration of the toll-like receptor-7 agonist 852A in patients with refractory metastatic melanoma. Clin Cancer Res. 2008;14:856–864. doi:10.1158/1078-0432.CCR-07-1938.
- Harrison LI, Astry C, Kumar S, Yunis C. Pharmacokinetics of 852A, an imidazoquinoline Toll-like receptor 7-specific agonist, following intravenous, subcutaneous, and oral administrations in humans. J Clin Pharmacol. 2007;47:962–969. doi:10.1177/0091270007303766.
- Bryden SR, Pingen M, Lefteri DA, Miltenburg J, Delang L, Jacobs S, Abdelnabi R, Neyts J, Pondeville E, Major J, et al. Pan-viral protection against arboviruses by activating skin macrophages at the inoculation site. Sci Transl Med. 2020;12:eaax2421. doi:10.1126/scitranslmed.aax2421.
- Cromarty R, Sigal A, Liebenberg LJP, McKinnon LR, Abdool Karim SS, Passmore JAS, Archary D. Diminished HIV infection of target CD4+ T cells in a toll-like receptor 4 stimulated in vitro model. Front Immunol. 2019;10:1705. doi:10.3389/fimmu.2019.01705.
- LaRue H, Ayari C, Bergeron A, Fradet Y. Toll-like receptors in urothelial cells–targets for cancer immunotherapy. Nat Rev Urol. 2013;10:537–545. doi:10.1038/nrurol.2013.153.
- Lu R, Groer C, Kleindl PA, Moulder KR, Huang A, Hunt JR, Cai S, Aires DJ, Berkland C, Forrest ML. Formulation and preclinical evaluation of a toll-likereceptor 7/8 agonist as an anti-tumoral immunomodulator. J Control Release. 2019;306:165-176. doi:10.1016/j.jconrel.2019.06.003 306.
- Balan S, Saxena M, Bhardwaj N. Dendritic cell subsets and locations. Int Rev Cell Mol Biol. 2019;348:1–68. doi:10.1016/bs.ircmb.2019.07.004.
- Ryan AT, Pulukuri AJ, Davaritouchaee M, Abbasi A, Hendricksen AT, Opp LK, Burt AJ, Nielsen AE, Mancini RJ. Comparing the immunogenicity of glycosidase-directed resiquimod prodrugs mediated by cancer cell metabolism. Acta Pharmacol Sin. 2020;41:995–1004. doi:10.1038/s41401-020-0432-4.
- Lu Q, Qi S, Li P, Yang L, Yang S, Wang Y, Cheng Y, Song Y, Wang S, Tan F, et al. Photothermally activatable PDA immune nanomedicine combined with PD-L1 checkpoint blockade for antimetastatic cancer photoimmunotherapy. J Mater Chem B. 2019;7:2499–2511. doi:10.1039/c9tb00089e.
- Rao S, Gharib K, Han A. Cancer immunosurveillance by T cells. Int Rev Cell Mol Biol. 2019;342:149–173. doi:10.1016/bs.ircmb.2018.08.001.
- Ota Y, Otsubo T, Goto M, Matsuki Y. Abstract 4078: enhanced anti-tumor activity through a combination of intravenous injectable TLR7 agonist, DSP-0509 and immune checkpoint inhibitors. Cancer Res. 2019;79:4078. doi:10.1158/1538-7445.am2019-4078.
- Ackerman SE, Gonzalez JC, Gregorio JD, Paik JC, Hartmann FJ, Kenkel JA, Lee A, Luo A, Pearson CI, Nguyen ML, et al. Abstract 1559: TLR7/8 immune-stimulating antibody conjugates elicit robust myeloid activation leading to enhanced effector function and anti-tumor immunity in pre-clinical models. Cancer Res. 2019;79:1559. doi:10.1158/1538-7445.am2019-1559.
- Wiedemann GM, Jacobi SJ, Chaloupka M, Krächan A, Hamm S, Strobl S, Baumgartner R, Rothenfusser S, Duewell P, Endres S, et al. A novel TLR7 agonist reverses NK cell anergy and cures RMA-S lymphoma-bearing mice. Oncoimmunology. 2016;5:e1189051. doi:10.1080/2162402X.2016.1189051.
- de Oliveira ECV, da Motta VRV, Pantoja PC, Ilha CSDO, Magalhães RF, Galadari H, Leonardi GR. Actinic keratosis - review for clinical practice. Int J Dermatol. 2019;58:400–407. doi:10.1111/ijd.14147.
- Chow LQM, Morishima C, Eaton KD, Baik CS, Goulart BH, Anderson LN, Manjarrez KL, Dietsch GN, Bryan JK, Hershberg RM, et al. Phase Ib trial of the toll-like receptor 8 agonist, motolimod (VTX-2337), combined with cetuximab in patients with recurrent or metastatic SCCHN. Clin Cancer Res. 2017;23:2442–2450. doi:10.1158/1078-0432.CCR-16-1934.
- Ferris RL, Saba NF, Gitlitz BJ, Haddad R, Sukari A, Neupane P, Morris JC, Misiukiewicz K, Bauman JE, Fenton M, et al. Effect of adding motolimod to standard combination chemotherapy and cetuximab treatment of patients with squamous cell carcinoma of the head and neck: the active8 randomized clinical trial. JAMA oncol. 2018;4:1583–1588. doi:10.1001/jamaoncol.2018.1888.
- Maekawa H, Inoue T, Ouchi H, Jao T-M, Inoue R, Nishi H, Fujii R, Ishidate F, Tanaka T, Tanaka Y, et al. Mitochondrial damage causes inflammation via cGAS-STING signaling in acute kidney injury. Cell Rep. 2019;29:1261–1273 e1266. doi:10.1016/j.celrep.2019.09.050.
- Grabosch S, Bulatovic M, Zeng F, Ma T, Zhang L, Ross M, Brozick J, Fang Y, Tseng G, Kim E, et al. Cisplatin-induced immune modulation in ovarian cancer mouse models with distinct inflammation profiles. Oncogene. 2019;38:2380–2393. doi:10.1038/s41388-018-0581-9.
- von der Maase H, Sengelov L, Roberts JT, Ricci S, Dogliotti L, Oliver T, Moore MJ, Zimmermann A, Arning M. Long-term survival results of a randomized trial comparing gemcitabine plus cisplatin, with methotrexate, vinblastine, doxorubicin, plus cisplatin in patients with bladder cancer. J clin oncol. 2005;23:4602–4608. doi:10.1200/JCO.2005.07.757.
- Yuh BE, Ruel N, Wilson TG, Vogelzang N, Pal SK. Pooled analysis of clinical outcomes with neoadjuvant cisplatin and gemcitabine chemotherapy for muscle invasive bladder cancer. J Urol. 2013;189:1682–1686. doi:10.1016/j.juro.2012.10.120.
- Obrist F, Michels J, Durand S, Chery A, Pol J, Levesque S, Joseph A, Astesana V, Pietrocola F, Wu GS, et al. Metabolic vulnerability of cisplatin-resistant cancers. Embo J. 2018;37. doi:10.15252/embj.201798597.
- Psyrri A, Burtness B. Targeted therapies: molecular selection for ‘smart’ study design in lung cancer. Nat Rev Clin Oncol. 2010;7:621–622. doi:10.1038/nrclinonc.2010.156.
- de La Motte Rouge T, Galluzzi L, Olaussen KA, Zermati Y, Tasdemir E, Robert T, Ripoche H, Lazar V, Dessen P, Harper F, et al. A novel epidermal growth factor receptor inhibitor promotes apoptosis in non-small cell lung cancer cells resistant to erlotinib. Cancer Res. 2007;67:6253–6262. doi:10.1158/0008-5472.CAN-07-0538.
- Kansy BA, Shayan G, Jie H-B, Gibson SP, Lei YL, Brandau S, Lang S, Schmitt NC, Ding F, Lin Y, et al. T cell receptor richness in peripheral blood increases after cetuximab therapy and correlates with therapeutic response. Oncoimmunology. 2018;7:e1494112. doi:10.1080/2162402X.2018.1494112.
- Savinskii GA, Khudaibergenov RU, Mansurov BM. Various structural and functional mechanisms of development of peptic ulcers after vagotomy. Klin Khir. 1989;8:13–15.
- Pol JG, Lévesque S, Workenhe ST, Gujar S, Le Boeuf F, Clements DR, Fahrner J-E, Fend L, Bell JC, Mossman KL, et al. Trial Watch: oncolytic viro-immunotherapy of hematologic and solid tumors. Oncoimmunology. 2018;7:e1503032. doi:10.1080/2162402X.2018.1503032.
- Brown CE, Mackall CL. CAR T cell therapy: inroads to response and resistance. Nat Rev Immunol. 2019;19:73–74. doi:10.1038/s41577-018-0119-y.
- Depil S, Duchateau P, Grupp SA, Mufti G, Poirot L. ‘Off-the-shelf’ allogeneic CAR T cells: development and challenges. Nat Rev Drug Discov. 2020;19:185–199. doi:10.1038/s41573-019-0051-2.
- De Santis R. Anti-ErbB2 immunotherapeutics: struggling to make better antibodies for cancer therapy. mAbs. 2020;12:1725346. doi:10.1080/19420862.2020.1725346.
- Oh DY, Bang YJ. HER2-targeted therapies - a role beyond breast cancer. Nat Rev Clin Oncol. 2020;17:33–48. doi:10.1038/s41571-019-0268-3.
- Mohan N, Hosain S, Zhao J, Shen Y, Luo X, Jiang J, Endo Y, Wu WJ. Atezolizumab potentiates Tcell-mediated cytotoxicity and coordinates with FAK to suppress cell invasion and motility in PD-L1+ triple negative breast cancer cells. Oncoimmunology. 2019;8:e1624128. doi:10.1080/2162402X.2019.1624128.
- Nie W, Qian J, Xu M-D, Gu K, Qian -F-F, Hu M-J, Lu J, Gan L, Zhang X-Y, Cao S-H, et al. A non-linear association between blood tumor mutation burden and prognosis in NSCLC patients receiving atezolizumab. Oncoimmunology. 2020;9:1731072. doi:10.1080/2162402X.2020.1731072.
- Patinote C, Karroum NB, Moarbess G, Cirnat N, Kassab I, Bonnet P-A, Deleuze-Masquéfa C. Agonist and antagonist ligands of toll-like receptors 7 and 8: ingenious tools for therapeutic purposes. Eur J Med Chem. 2020;193:112238. doi:10.1016/j.ejmech.2020.112238.
- Brown RA, Herzig RH, Wolff SN, Frei-Lahr D, Pineiro L, Bolwell BJ, Lowder JN, Harden EA, Hande KR, Herzig GP, et al. High-dose etoposide and cyclophosphamide without bone marrow transplantation for resistant hematologic malignancy. Blood. 1990;76:473–479. doi:10.1182/blood.V76.3.473.473.
- Sirachainan N, Pakakasama S, Anurathapan U, Hansasuta A, Dhanachai M, Khongkhatithum C, Jinawath A, Mahachoklertwattana P, Hongeng S. Outcome of newly diagnosed high risk medulloblastoma treated with carboplatin, vincristine, cyclophosphamide and etoposide. J clin neurosci. 2018;56:139–142. doi:10.1016/j.jocn.2018.06.028.
- Ansell SM. Pembrolizumab: living up to expectations. Blood. 2019;134:1114–1115. doi:10.1182/blood.2019002417.
- Kuryk L, Moller AW, Jaderberg M. Combination of immunogenic oncolytic adenovirus ONCOS-102 with anti-PD-1 pembrolizumab exhibits synergistic antitumor effect in humanized A2058 melanoma huNOG mouse model. Oncoimmunology. 2019;8:e1532763. doi:10.1080/2162402X.2018.1532763.
- Kroemer G, Galluzzi L. Combinatorial immunotherapy with checkpoint blockers solves the problem of metastatic melanoma-an exclamation sign with a question mark. Oncoimmunology. 2015;4:e1058037. doi:10.1080/2162402X.2015.1058037.
- Naing A, Gainor JF, Gelderblom H, Forde PM, Butler MO, Lin -C-C, Sharma S, Ochoa de Olza M, Varga A, Taylor M, et al. A first-in-human phase 1 dose escalation study of spartalizumab (PDR001), an anti-PD-1 antibody, in patients with advanced solid tumors. J Immunother Cancer. 2020;8(8):e000530. doi:10.1136/jitc-2020-000530.
- Ghanem S, Valecha GK, Hossri S, Ibrahim U, Atallah JP. Investigational PD-1 inhibitors for advanced non-small lung cancer: new players in a shifting paradigm. Expert Opin Investig Drugs. 2017;26(1317–1319):1317–1319. doi:10.1080/13543784.2017.1395019.
- Weber MM, Fottner C. Immune checkpoint inhibitors in the treatment of patients with neuroendocrine neoplasia. Oncol Res Treat. 2018;41:306–312. doi:10.1159/000488996.
- Kaplon H, Muralidharan M, Schneider Z, Reichert JM. Antibodies to watch in 2020. mAbs. 2020;12:1703531. doi:10.1080/19420862.2019.1703531.
- Diab A, Marcondes M, Tagliaferri MA, Hoch U, Zhang J, Rubas W, Kivimae S, Zalevsky J, Conley1 AP, Borazanci E, et al. REVEAL: A phase I/II, open-label, multicenter, dose escalation and dose expansion study of NKTR-262 [TLR 7/8 agonist] plus NKTR-214 [CD122-biased agonist] with or without nivolumab (nivo) in patients (pts) with locally advanced or metastatic solid tumor malignancies. Ann Oncol. 2018;29:viii146–viii147. doi:10.1093/annonc/mdy279.433.
- Sharma M, Khong H, Fa’ak F, Bentebibel S-E, Janssen LME, Chesson BC, Creasy CA, Forget M-A, Kahn LMS, Pazdrak B, et al. Bempegaldesleukin selectively depletes intratumoral Tregs and potentiates T cell-mediated cancer therapy. Nat Commun. 2020;11:661. doi:10.1038/s41467-020-14471-1.
- Klinghoffer RA, Bahrami SB, Hatton BA, Frazier JP, Moreno-Gonzalez A, Strand AD, Kerwin WS, Casalini JR, Thirstrup DJ, You S, et al. A technology platform to assess multiple cancer agents simultaneously within a patient’s tumor. Sci Transl Med. 2015;7:284ra258. doi:10.1126/scitranslmed.aaa7489.
- Frazier JP, Bertout JA, Kerwin WS, Moreno-Gonzalez A, Casalini JR, Grenley MO, Beirne E, Watts KL, Keener A, Thirstrup DJ, et al. Multidrug analyses in patients distinguish efficacious cancer agents based on both tumor cell killing and immunomodulation. Cancer Res. 2017;77:2869–2880. doi:10.1158/0008-5472.CAN-17-0084.
- Dey J, Kerwin WS, Grenley MO, Casalini JR, Tretyak I, Ditzler SH, Thirstrup DJ, Frazier JP, Pierce DW, Carleton M, et al. A platform for rapid, quantitative assessment of multiple drug combinations simultaneously in solid tumors in vivo. PloS One. 2016;11:e0158617. doi:10.1371/journal.pone.0158617.
- Moreno-Gonzalez A, Olson JM, Klinghoffer RA. Predicting responses to chemotherapy in the context that matters - the patient. Mol Cell Oncol. 2016;3:e1057315. doi:10.1080/23723556.2015.1057315.
- Syed YY. Anlotinib: first global approval. Drugs. 2018;78:1057–1062. doi:10.1007/s40265-018-0939-x.
- Han B, Li K, Wang Q, Zhang L, Shi J, Wang Z, Cheng Y, He J, Shi Y, Zhao Y, et al. Effect of anlotinib as a third-line or further treatment on overall survival of patients with advanced non-small cell lung cancer: the ALTER 0303 phase 3 randomized clinical trial. JAMA oncol. 2018;4:1569–1575. doi:10.1001/jamaoncol.2018.3039.
- Han B, Li K, Zhao Y, Li B, Cheng Y, Zhou J, Lu Y, Shi Y, Wang Z, Jiang L, et al. Anlotinib as a third-line therapy in patients with refractory advanced non-small-cell lung cancer: a multicentre, randomised phase II trial (ALTER0302). Br J Cancer. 2018;118:654–661. doi:10.1038/bjc.2017.478.
- Kobold S, Wiedemann G, Rothenfusser S, Endres S. Modes of action of TLR7 agonists in cancer therapy. Immunotherapy. 2014;6:1085–1095. doi:10.2217/imt.14.75.
- Sautes-Fridman C, Cherfils-Vicini J, Damotte D, Fisson S, Fridman WH, Cremer I, Dieu-Nosjean M-C. Tumor microenvironment is multifaceted. Cancer Metastasis Rev. 2011;30:13–25. doi:10.1007/s10555-011-9279-y.
- Leclerc M, Mezquita L, Guillebot De Nerville G, Tihy I, Malenica I, Chouaib S, Mami-Chouaib F. Recent advances in lung cancer immunotherapy: input of T-CELL epitopes associated with impaired peptide processing. Front Immunol. 2019;10:1505. doi:10.3389/fimmu.2019.01505.
- Gravett AM, Trautwein N, Stevanovic S, Dalgleish AG, Copier J. Gemcitabine alters the proteasome composition and immunopeptidome of tumour cells. Oncoimmunology. 2018;7:e1438107. doi:10.1080/2162402X.2018.1438107.
- Farhad M, Rolig AS, Redmond WL. The role of Galectin-3 in modulating tumor growth and immunosuppression within the tumor microenvironment. Oncoimmunology. 2018;7:e1434467. doi:10.1080/2162402X.2018.1434467.
- O’Donnell JS, Teng MWL, Smyth MJ. Cancer immunoediting and resistance to T cell-based immunotherapy. Nat Rev Clin Oncol. 2019;16:151–167. doi:10.1038/s41571-018-0142-8.
- Martinek J, Wu TC, Cadena D, Banchereau J, Palucka K. Interplay between dendritic cells and cancer cells. Int Rev Cell Mol Biol. 2019;348:179–215. doi:10.1016/bs.ircmb.2019.07.008.
- Quintas-Cardama A, Kantarjian H, Cortes J, Verstovsek S. Janus kinase inhibitors for the treatment of myeloproliferative neoplasias and beyond. Nat Rev Drug Discov. 2011;10:127–140. doi:10.1038/nrd3264.
- Budd RC, Yeh WC, Tschopp J. cFLIP regulation of lymphocyte activation and development. Nat Rev Immunol. 2006;6:196–204. doi:10.1038/nri1787.