ABSTRACT
Formyl peptide receptor 1 (FPR1) is a pattern-recognition receptor that detects bacterial as well as endogenous danger-associated molecular patterns to trigger innate immune responses by myeloid cells. A single nucleotide polymorphism, rs867228 (allelic frequency 19–20%), in the gene coding for FPR1 accelerates the manifestation of multiple carcinomas, likely due to reduced anticancer immunosurveillance secondary to a defect in antigen presentation by dendritic cells. Another polymorphism in FPR1, rs5030880 (allelic frequency 12–13%), has been involved in the resistance to plague, correlating with the fact that FPR1 is the receptor for Yersinia pestis. Driven by the reported preclinical effects of FPR1 on lung inflammation and fibrosis, we investigated whether rs867228 or rs5030880 would affect the severity of coronavirus disease-19 (COVID-19). Data obtained on patients from two different hospitals in Paris refute the hypothesis that rs867228 or rs5030880 would affect the severity of COVID-19.
Introduction
Coronavirus disease 19-(COVID-19) poses a major challenge because of its dangerousness for susceptible individuals (i.e. persons with preexisting medical conditions, in particular, obesity and the elderly) as well as its political (mis-)management that disrupted the economic, cultural and social life of entire nations.Citation1,Citation2 In nonsusceptible individuals lacking discernible risk factors,Citation3,Citation4 infection by SARS-CoV-2, the agent responsible for COVID-19 is usually asymptomatic or paucisymptomatic, although exceptions have been reported.Citation5–9 For this reason, strategies for identifying individuals at risk might help to guide strategies for the selective confinement or vaccination of those persons who are at risk of severe or lethal SARS-CoV-2.Citation5
Formyl peptide receptor 1 (FPR1) is a pattern-recognition receptor (PRR)Citation10 that is mostly expressed by myeloid cells including granulocytes, macrophages, and dendritic cells.Citation11 Like other PRRs, FPR1 recognizes pathogen-associated molecular patterns (PAMPs), which are microbial structures, and danger-associated molecular patterns (DAMPs), which are host molecules displayed on, or released by, stressed and dying cells.Citation12–15 As its name indicates, FPR1 recognizes formylated peptides, mostly peptides from bacteria that have undergone a prokaryote-specific post-translation protein modification called formylation.Citation12 However, formylated peptides are also generated by mitochondria (which, in evolutionary terms, are relics of prokaryotes incorporated into the eukaryotic proto-organism).Citation16,Citation17 Moreover, FPR1 interacts with other endogenous ligands including annexin A1 (ANXA1), a ubiquitous protein contained in the cytosol of all nucleated cells that leak into the extracellular space when cells die.Citation18–23 Thus, FPR1 plays a major role in the response to pathogens as well as in the regulation of immune and inflammatory responses.Citation24
The roles of FPR1 in the response to infectious pathogens can be either positive or negative.Citation11,Citation22 Thus, FPR1-deficient mice are more susceptible to lethal infection by Escherichia coli and Listeria monocytogenesCitation25,Citation26 but resistant against Yersinia pestis, the agent causing plague.Citation27 Similarly, FPR1 has an ambiguous role in the context of noninfectious diseases.Citation11 FPR1-deficient mice are resistant against pathogenic inflammation in the context of ischemia-reperfusion damage of the heart,Citation28 celiac disease,Citation29 endotoxin-induced lung injury,Citation30,Citation31 cigarette smoke-induced emphysema,Citation32,Citation33 as well as bleomycin-induced pulmonary fibrosis.Citation34 Conversely, FPR1-negative mice are unable to mount immune responses against cancers following chemotherapy with anthracyclines or oxaliplatin.Citation14,Citation22,Citation35
The ambiguous role of FPR1 extends to humans. In the context of plague, the single nucleotide polymorphism (SNP) rs5030880 (R190W affecting the extracellular loop 2 of FPR1)Citation36 may constitute a resistance allele.Citation27 In the context of cancer, the loss-of-function SNP rs867228 (E346A affecting the intracellular C-terminus of FPR1)Citation36 is associated with reduced survival in breast and colorectal cancer patients treated by adjuvant anthracycline-based and oxaliplatin-based chemotherapy, respectively.Citation35 Moreover, rs867228 is associated with the precocious manifestation of the breast, colorectal, esophageal, and head & neck cancer.Citation37
Intrigued by these findings, in particular, the impact of FPR1 on lung inflammation, we wondered whether the cancer-associated FPR1 SNP rs867228 and the plague resistance-associated SNP rs5030880 might affect patient susceptibility to severe COVID-19.
Materials and methods
Patients
This non-interventional multicenter CPP2020-04-048b study enrolled 140 patients () who were diagnosed with COVID-19 based on positive RT-PCR of pharyngeal swabs. One hundred and forty patients were successfully genotyped for rs5030880 and 139 patients for rs867228 SNPs. Patients were stratified according to disease severity. Mild disease was defined by no or limited clinical symptoms, not requiring computed tomography (CT) scanning or hospitalization. Moderate disease was defined as being symptomatic, with dyspnea and radiological findings of pneumonia upon thoracic CT scan, requiring hospitalization with a maximum of 9 L/min of oxygen. Severe disease was defined as respiratory distress requiring admission at the intensive care unit (ICU). Critical disease was defined as respiratory failure, septic shock, and/or multiple organ dysfunction.
Table 1. Allelic frequencies of FPR1 SNPs and characteristics of patient population from Hôpital Cochin and Hôpital Européen George Pompidou
DNA extraction
DNA was isolated form peripheral blood after elimination of red blood cells using Maxwell® 16 LEV Blood DNA Kit (Hôpital Cochin) or DNeasy Blood & Tissue Kits (Hôpital Européen George Pompidou) from Promega Corporation (Wisconsin, USA) and QiagenTM (Germany), respectively.
Genotyping
After extraction, genomic DNA was quantified using a NanoDrop instrument 1000 Spectrophotometer (Thermo Fisher ScientificTM, Rockford, IL, USA). Then, DNA was amplified using the assay C_3266374_1 and C_25628595_20 (Thermo Fisher ScientificTM, Rockford, IL, USA) for rs867228 and rs5030880 SNPs, respectively. Genotype-specific fluorescently labeled-probes allowed allelic discrimination upon a comparison of signals from fluorescent probes (VIC and FAM).
Statistical analysis
Statistical analysis of data from Hôpital Cochin and Hôpital Européen George Pompidou was performed individually as well as cumulatively. Additionally, heterozygous and mutated patients were grouped for the statistical analysis. Proportions of patients falling in each category were compared using the fisher’s exact test or chi-squared test to investigate survival or severity, respectively, using the GraphPad Prism software (San Diego, CA, USA). * p value < .05; ns, nonsignificant.
Results and discussion
One hundred forty patients diagnosed with COVID-19 were enrolled in this study following the usual procedures (written informed consent, permission by the ethical committee, anonymization of all records) at two major Paris hospitals, Hôpital Cochin, and Hôpital Européen George Pompidou (HEGP). Patients were stratified according to COVID-19 severity () and were genotyped for the cancer-relevant SNP rs867228 and the plague-relevant SNP rs5030880. Results were represented for patients from Hôpital Cochin (), HEGP () or both hospitals (). In the overall population, rs867228 is found in homozygosity (TT) and heterezygosity (GT) at a frequency of 3.8% and 15.7% and rs5030880 at a frequency of 1.4% (AA) and 10.5% (TA), respectively. Both SNPs were found at a frequency that is similar to that reported to the general population. No significant trend in favor of a disease severity-associated under- or overrepresentation of either of the two SNPs was detected, suggesting that none of the two SNPs has a major impact on disease severity. Thus, within the limits of this study (which may be underpowered to detect small effects), the results invalidate the hypothesis that major disease-relevant SNPs in FPR1 would have a strong impact on COVID-19 pathogenesis.
Figure 1. Severity and survival of patients diagnosed with COVID19 bearing at least one copy of the variant allele of rs867228 and rs5030880 SNPs. Proportion of patients diagnosed with critical, severe, moderate and mild COVID19 disease from Hôpital Cochin (a) and Hôpital Européen George Pompidou (HEGP) (c). Patients’ survival is shown as proportion of alive and dead subjects from Hôpital Cochin (b) and HEGP (d). Severity (e) and survival (f) of cumulative data from the two above mentioned hospitals. Statistical significance was calculated using the chi-squared test
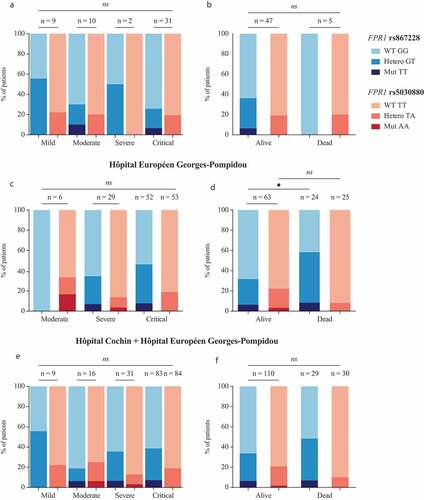
Genome-wide association studies have shown that progression of COVID-19 to respiratory failure is associated with locus 3p21.31, spanning the genes solute carrier family 6 member 20 (SLC6A20), leucine zipper transcription factor-like 1 (LZTFL1), C-C motif chemokine receptor 9 (CCR9), FYVE and coiled-coil domain autophagy adaptor 1 (FYCO1), C-X-C motif chemokine receptor 6 (CXCR6) and X-C motif chemokine receptor 1 (XCR1), as well as with locus 9q34.2 coinciding with the alpha 1-3-N-acetylgalactosaminyltransferase and alpha 1-3-galactosyltransferase (ABO) blood group locus, but no association with 19q.13.3, where FPR1 is located.Citation38Another study demonstrated that SNPs affecting angiotensin I converting enzyme 2 (ACE2) and two key host factors of SARS-CoV-2 were likely associated with the genetic susceptibility of COVID-19.Citation39,Citation40 Thus, the literature published during the execution of this study confirms the conjecture that SNPs in FPR1 have no major disease-modulatory role in COVID-19.Citation41
In conclusion, neither rs867228 nor rs5030880 affects the severity of the clinical course of COVID-19. It appears plausible to speculate that polymorphisms affecting the function of PRRs such as FPR1 or Toll-like receptors are prevalent in the population to create heterogeneity in the response to major life-threatening infectious pathogens, avoiding, for example, excessive inflammatory responses in a fraction of the population. In this sense, such PRR polymorphisms might have a similar function as the heterogeneity in major histocompatibility complex (MHC) genes.Citation42,Citation43 While MHC polymorphisms create interindividual variation in cognate antigen recognition because of their impact on the immunopeptidome, PRR polymorphism would create variation in the recognition of innate adjuvant signals.Citation44 The combination of both mechanisms then may increase the chance that a sizable fraction of the population is protected against one or the other infectious agent. However, at this point, it remains to be determined which communicable diseases are modulated in their severity by rs867228 or rs5030880.
Acknowledgments
We are indebted to Drs. Aurélien Philippe, Nadia Rivet (all at Université de Paris, Innovative Therapies in Haemostasis, INSERM; and Hematology department and Biosurgical research lab (Carpentier Foundation) Assistance Publique Hôpitaux de Paris, Paris, France) and Dr. Jérome Duchemin (Biological Hematology Department, Assistance Publique-Hôpitaux de Paris. Centre-Université de Paris, Hôpital Cochin, Paris, France) for providing patient samples and information on clinical samples. We also thank Amandine Houvert, research technician (Université de Paris, Institut Cochin, CNRS UMR8104, INSERM U1016, F-75006 Paris, France); Prof. Frédéric Pène (Service de Médecine Intensive et Réanimation, Assistance Publique-Hôpitaux de Paris.Centre-Université de Paris, Hôpital Cochin, Paris, France), Dr. Tali-Ann Szwebel (Service de Médecine Interne, Assistance Publique-Hôpitaux de Paris. Centre-Université de Paris, Hôpital Cochin, Paris, France), and Dr. Delphine Cantin (Service des Urgences, Assistance Publique-Hôpitaux de Paris. Centre-Université de Paris, Hôtel-Dieu, Paris, France). GK is supported by the Ligue contre le Cancer (équipe labellisée); Agence National de la Recherche (ANR) – Projets blancs; AMMICa US23/CNRS UMS3655; Association pour la recherche sur le cancer (ARC); Association “Ruban Rose”; Cancéropôle Ile-de-France; Chancelerie des universités de Paris (Legs Poix), Fondation pour la Recherche Médicale (FRM); a donation by Elior; European Research Area Network on Cardiovascular Diseases (ERA-CVD, MINOTAUR); Gustave Roussy Odyssea, the European Union Horizon 2020 Project Oncobiome; Fondation Carrefour; High-end Foreign Expert Program in China (GDW20171100085), Institut National du Cancer (INCa); Inserm (HTE); Institut Universitaire de France; LeDucq Foundation; the LabEx Immuno-Oncology (ANR-18-IDEX-0001); the RHU Torino Lumière; the Seerave Foundation; the SIRIC Stratified Oncology Cell DNA Repair and Tumor Immune Elimination (SOCRATE); and the SIRIC Cancer Research and Personalized Medicine (CARPEM). This study contributes to the IdEx Université de Paris ANR-18-IDEX-0001. AP was in receipt of a European Union Horizon 2020 Marie Skłodowska-Curie Innovative Training Network (ITN-ETN) Grant, Award No.: 675712.
References
- Raoult D, Zumla A, Locatelli F, Ippolito G, Kroemer G. Coronavirus infections: epidemiological, clinical and immunological features and hypotheses. Cell Stress. 2020;4(4):66–6. doi:10.15698/cst2020.04.216.
- Zhu N, Zhang D, Wang W, Li X, Yang B, Song J, Zhao X, Huang B, Shi W, Lu R, et al. A novel coronavirus from patients with pneumonia in China, 2019. N Engl J Med. 2020;382(8):727–733. doi:10.1056/NEJMoa2001017.
- Jordan RE, Adab P, Cheng KK. Covid-19: risk factors for severe disease and death. BMJ. 2020;368:m1198. doi:10.1136/bmj.m1198.
- Zheng Z, Peng F, Xu B, Zhao J, Liu H, Peng J, Li Q, Jiang C, Zhou Y, Liu S, et al. Risk factors of critical & mortal COVID-19 cases: A systematic literature review and meta-analysis. J Infect. 2020;81(2):e16–e25. doi:10.1016/j.jinf.2020.04.021.
- Berlin DA, Gulick RM, Martinez FJ. Severe Covid-19. N Engl J Med. 2020. doi:10.1056/NEJMcp2009575.
- Coronaviridae Study Group of the International Committee on Taxonomy of V. The species Severe acute respiratory syndrome-related coronavirus: classifying 2019-nCoV and naming it SARS-CoV-2. Nat Microbiol. 2020;5(4):536–544. doi:10.1038/s41564-020-0695-z.
- Melenotte C, Silvin A, Goubet AG, Lahmar I, Dubuisson A, Zumla A, Raoult D, Merad M, Gachot B, Hénon C, et al. Immune responses during COVID-19 infection. Oncoimmunology. 2020;9(1):1807836. doi:10.1080/2162402X.2020.1807836.
- Petersen E, Koopmans M, Go U, Hamer DH, Petrosillo N, Castelli F, Storgaard M, Al Khalili S, Simonsen L, et al. Comparing SARS-CoV-2 with SARS-CoV and influenza pandemics. Lancet Infect Dis. 2020;20(9):e238–e44. doi:10.1016/S1473-3099(20)30484-9.
- Yuki K, Fujiogi M, Koutsogiannaki S. COVID-19 pathophysiology: A review. Clin Immunol. 2020;215:108427. doi:10.1016/j.clim.2020.108427.
- Kawai T, Akira S. The role of pattern-recognition receptors in innate immunity: update on Toll-like receptors. Nat Immunol. 2010;11(5):373–384. doi:10.1038/ni.1863.
- Vacchelli E, Le Naour J, Kroemer G. The ambiguous role of FPR1 in immunity and inflammation. Oncoimmunology. 2020;9(1):1760061. doi:10.1080/2162402X.2020.1760061.
- Gong T, Liu L, Jiang W, Zhou R. DAMP-sensing receptors in sterile inflammation and inflammatory diseases. Nat Rev Immunol. 2020;20:95–112.
- Rodriguez-Nuevo A, Zorzano A. The sensing of mitochondrial DAMPs by non-immune cells. Cell Stress. 2019;3(6):195–207. doi:10.15698/cst2019.06.190.
- Vacchelli E, Enot DP, Pietrocola F, Zitvogel L, Kroemer G. Impact of pattern recognition receptors on the prognosis of breast cancer patients undergoing adjuvant chemotherapy. Cancer Res. 2016;76(11):3122–3126. doi:10.1158/0008-5472.CAN-16-0294.
- Venereau E, Ceriotti C, Bianchi ME. DAMPs from cell death to new life. Front Immunol. 2015;6:422. doi:10.3389/fimmu.2015.00422.
- Banoth B, Cassel SL. Mitochondria in innate immune signaling. Transl Res. 2018;202:52–68. doi:10.1016/j.trsl.2018.07.014.
- Kroemer G. Mitochondrial implication in apoptosis. Towards an endosymbiont hypothesis of apoptosis evolution. Cell Death Differ. 1997;4(6):443–456. doi:10.1038/sj.cdd.4400266.
- Ernst S, Lange C, Wilbers A, Goebeler V, Gerke V, Rescher U. An annexin 1 N-terminal peptide activates leukocytes by triggering different members of the formyl peptide receptor family. J Immunol. 2004;172(12):7669–7676. doi:10.4049/jimmunol.172.12.7669.
- Foo SL, Yap G, Cui J, Lim LHK. Annexin-A1 – A blessing or a curse in cancer? Trends Mol Med. 2019;25(4):315–327. doi:10.1016/j.molmed.2019.02.004.
- Perretti M. The annexin 1 receptor(s): is the plot unravelling? Trends Pharmacol Sci. 2003;24(11):574–579. doi:10.1016/j.tips.2003.09.010.
- Scannell M, Flanagan MB, deStefani A, Wynne KJ, Cagney G, Godson C, Maderna P. Annexin-1 and peptide derivatives are released by apoptotic cells and stimulate phagocytosis of apoptotic neutrophils by macrophages. J Immunol. 2007;178(7):4595–4605. doi:10.4049/jimmunol.178.7.4595.
- Vacchelli E, Ma Y, Baracco EE, Sistigu A, Enot DP, Pietrocola F, Yang H, Adjemian S, Chaba K, Semeraro M, et al. Chemotherapy-induced antitumor immunity requires formyl peptide receptor 1. Science. 2015;350(6263):972–978. doi:10.1126/science.aad0779.
- Walther A, Riehemann K, Gerke V. A novel ligand of the formyl peptide receptor: annexin I regulates neutrophil extravasation by interacting with the FPR. Mol Cell. 2000;5(5):831–840. doi:10.1016/S1097-2765(00)80323-8.
- Dorward DA, Lucas CD, Chapman GB, Haslett C, Dhaliwal K, Rossi AG. The role of formylated peptides and formyl peptide receptor 1 in governing neutrophil function during acute inflammation. Am J Pathol. 2015;185(5):1172–1184. doi:10.1016/j.ajpath.2015.01.020.
- Liu M, Chen K, Yoshimura T, Liu Y, Gong W, Wang A, Gao J-L, Murphy PM, Wang JM. Formylpeptide receptors are critical for rapid neutrophil mobilization in host defense against Listeria monocytogenes. Sci Rep. 2012;2(1):786. doi:10.1038/srep00786.
- Zhang M, Gao J-L, Chen K, Yoshimura T, Liang W, Gong W, Li X, Huang J, McDermott DH, Murphy PM, et al. A critical role of formyl peptide receptors in host defense against Escherichia coli. J Immunol. 2020;204(9):2464–2473. doi:10.4049/jimmunol.1900430.
- Osei-Owusu P, Charlton TM, Kim HK, Missiakas D, Schneewind O. FPR1 is the plague receptor on host immune cells. Nature. 2019;574(7776):57–62. doi:10.1038/s41586-019-1570-z.
- Zhou QL, Teng F, Zhang YS, Sun Q, Cao YX, Meng GW. FPR1 gene silencing suppresses cardiomyocyte apoptosis and ventricular remodeling in rats with ischemia/reperfusion injury through the inhibition of MAPK signaling pathway. Exp Cell Res. 2018;370(2):506–518. doi:10.1016/j.yexcr.2018.07.016.
- Lammers KM, Chieppa M, Liu L, Liu S, Omatsu T, Janka-Junttila M, Casolaro V, Reinecker H-C, Parent CA, Fasano A, et al. Gliadin induces neutrophil migration via engagement of the formyl peptide receptor, FPR1. PLoS One. 2015;10(9):e0138338. doi:10.1371/journal.pone.0138338.
- Grommes J, Drechsler M, Soehnlein O. CCR5 and FPR1 mediate neutrophil recruitment in endotoxin-induced lung injury. J Innate Immun. 2014;6(1):111–116. doi:10.1159/000353229.
- Zhang X, Wang T, Yuan ZC, Dai LQ, Zeng N, Wang H, Liu L, Wen FQ. Mitochondrial peptides cause proinflammatory responses in the alveolar epithelium via FPR-1, MAPKs, and AKT: a potential mechanism involved in acute lung injury. Am J Physiol Lung Cell Mol Physiol. 2018;315(5):L775–L86. doi:10.1152/ajplung.00466.2017.
- Cardini S, Dalli J, Fineschi S, Perretti M, Lungarella G, Lucattelli M. Genetic Ablation of the Fpr1 gene confers protection from smoking-induced lung emphysema in mice. Am J Respir Cell Mol Biol. 2012;47(3):332–339. doi:10.1165/rcmb.2012-0036OC.
- Stockley RA, Grant RA, Llewellyn-Jones CG, Hill SL, Burnett D. Neutrophil formyl-peptide receptors. Relationship to peptide-induced responses and emphysema. Am J Respir Crit Care Med. 1994;149(2):464–468. doi:10.1164/ajrccm.149.2.8306047.
- Leslie J, Millar BJ, Del Carpio Pons A, Burgoyne RA, Frost JD, Barksby BS, Luli S, Scott J, Simpson AJ, Gauldie J, et al. FPR-1 is an important regulator of neutrophil recruitment and a tissue-specific driver of pulmonary fibrosis. JCI Insight. 2020;5(4). doi:10.1172/jci.insight.125937.
- Baracco EE, Pietrocola F, Buque A, Bloy N, Senovilla L, Zitvogel L, Vacchelli E, Kroemer G. Inhibition of formyl peptide receptor 1 reduces the efficacy of anticancer chemotherapy against carcinogen-induced breast cancer. Oncoimmunology. 2016;5(6):e1139275. doi:10.1080/2162402X.2016.1139275.
- Sahagun-Ruiz A, Colla JS, Juhn J, Gao JL, Murphy PM, McDermott DH. Contrasting evolution of the human leukocyte N-formylpeptide receptor subtypes FPR and FPRL1R. Genes Immun. 2001;2(6):335–342. doi:10.1038/sj.gene.6363787.
- Le Naour J, Liu P, Zhao L, Adjemian S, Sztupinszki Z, Taieb J, Mulot C, Silvin A, Dutertre CA, Ginhoux F, et al. A TLR3 ligand reestablishes chemotherapeutic responses in the context of FPR1 deficiency. Cancer Discov. pp.CD-20-0465. 2020. doi:10.1158/2159-8290.CD-20-0465
- Ellinghaus D, Degenhardt F, Bujanda L, Buti M, Albillos A, Invernizzi P, Fernández J, Prati D, Baselli G, Asselta R, et al. Genomewide Association study of severe Covid-19 with respiratory failure. N Engl J Med. 2020;383:1522-1534. doi:10.1056/NEJMoa2020283.
- Hou Y, Zhao J, Martin W, Kallianpur A, Chung MK, Jehi L,Sharifi N, Erzurum S, Eng C, Cheng F. New insights into genetic susceptibility of COVID-19: an ACE2 and TMPRSS2 polymorphism analysis. BMC Med. 2020;18:216. doi:10.1186/s12916-020-01673-z.
- Kuba K, Imai Y, Ohto-Nakanishi T, Penninger JM. Trilogy of ACE2: a peptidase in the renin-angiotensin system, a SARS receptor, and a partner for amino acid transporters. Pharmacol Ther. 2010;128(1):119–128. doi:10.1016/j.pharmthera.2010.06.003.
- Ovsyannikova IG, Haralambieva IH, Crooke SN, Poland GA, Kennedy RB. The role of host genetics in the immune response to SARS-CoV-2 and COVID-19 susceptibility and severity. Immunol Rev. 2020;296(1):205–219. doi:10.1111/imr.12897.
- Matzaraki V, Kumar V, Wijmenga C, Zhernakova A. The MHC locus and genetic susceptibility to autoimmune and infectious diseases. Genome Biol. 2017;18(1):76. doi:10.1186/s13059-017-1207-1.
- Sambaturu N, Mukherjee S, Lopez-Garcia M, Molina-Paris C, Menon GI, Chandra N. Role of genetic heterogeneity in determining the epidemiological severity of H1N1 influenza. PLoS Comput Biol. 2018;14(3):e1006069. doi:10.1371/journal.pcbi.1006069.
- Skevaki C, Pararas M, Kostelidou K, Tsakris A, Routsias JG. Single nucleotide polymorphisms of Toll-like receptors and susceptibility to infectious diseases. Clin Exp Immunol. 2015;180(2):165–177. doi:10.1111/cei.12578.