ABSTRACT
Rhabdomyosarcoma (RMS) is a heterogeneous soft tissue neoplasm most frequently found in children and adolescents. As the prognosis for recurrent and metastatic RMS remains poor, immunotherapies are hoped to improve quality of life and survival. CD137 is a member of tumor necrosis factor receptor family and a T cell costimulatory molecule which induces potent cellular immune responses that are able to eliminate malignant cells. Therefore, it was puzzling to find expression of CD137 on an RMS tissue microarray by multiplex staining. CD137 is not only expressed by infiltrating T cells but also by malignant RMS cells. Functional in vitro experiments demonstrate that CD137 on RMS cells is being transferred to adjacent antigen-presenting cells by trogocytosis, where it downregulates CD137 ligand, and thereby reduces T cell costimulation which results in reduced killing of RMS cells. The transfer of CD137 and the subsequent downregulation of CD137 ligand is a physiological negative feedback mechanism that is likely usurped by RMS, and may facilitate its escape from immune surveillance. In addition, CD137 signals into RMS cells and induces IL-6 and IL-8 secretion, which are linked to RMS metastasis and poor prognosis. However, the ectopic expression of CD137 on RMS cells is an Achilles’ heel that may be utilized for immunotherapy. Natural killer cells expressing an anti-CD137 chimeric antigen receptor specifically kill CD137-expressing RMS cells. Our study implicates ectopic CD137 expression as a pathogenesis mechanism in RMS, and it demonstrates that CD137 may be a novel target for immunotherapy of RMS.
Introduction
Rhabdomyosarcoma (RMS) is a heterogeneous soft tissue neoplasm, originating from primitive mesenchymal stem cells which are directed toward myogenesis but fail to complete the normal growth and differentiation program of myogenic precursor cellsCitation1. RMS has a high prevalence in children and adolescents, but is rarely found in adults. Based on histological features, RMS is categorized into four subtypes: alveolar RMS (ARMS), embryonal RMS (ERMS), pleomorphic RMS (PRMS), and sclerosing/spindle cell RMS (SC-RMS). ARMS has a particularly aggressive clinical course, and most ARMS cases are fusion positive, i.e., they are characterized by two canonical chromosomal translocations: t(2;13)(q35;q14) and t(1;13)(p36;q14) leading to the generation of PAX3-FOXO1 and PAX7-FOXO1 fusion oncoproteins, respectively,Citation2,Citation3 in addition to other rare gene fusions.
Conventional multimodal therapy (radiation, surgery, and chemotherapy) remains the standard treatment for RMS. However, overall prognosis remains poor, especially for fusion-positive, recurrent, and metastatic RMS.Citation4,Citation5 Since RMS is quite heterogeneous with a variable degree of mutational profile, protein expression, and myogenic differentiation, an RMS-specific-targeted therapy remains a challenge.
CD137 (TNFRSF9, 4–1BB) is a member of tumor necrosis factor receptor (TNFR) family.Citation6 Costimulation through CD137 enhances proliferation and survival of T cells, particularly of CD8+ T cells, with enhanced cytotoxic activity and IFNγ secretion.Citation7 CD137 agonists have been shown to potently enhance anti-cancer immune responses,Citation8–10 and are currently being tested in clinical trials for tumor immunotherapy.Citation11 Agonistic anti-CD137 antibodies also strongly enhance anti-virus immune responses.Citation12
CD137 ligand (CD137L) is expressed on antigen-presenting cells (APC), and APC use CD137L to costimulate T cells. CD137L is a cell surface protein and transduces signals into APC, making CD137 and CD137L a bidirectional signal transduction system.Citation13 The reverse signal through CD137L activates APC, and it induces differentiation of human monocytes to a specific type of dendritic cell (DC), the CD137L-DCCitation14,Citation15 that induces strong cellular, Th1/Tc1 immune responses, and more potent anti-cancer immune responses than DC generated by GM-CSF and IL-4.Citation16 Human CD137L associates with CD32a, TNFRI, and potentially other molecules to form a signaling complex.Citation17,Citation18 Natural inhibitors of the CD137/CD137L system are soluble forms of CD137 which are generated by differential splicing.Citation19,Citation20
Since CD137 is a powerful T cell costimulatory molecule, it was surprising to find expression of CD137 on Hodgkin and Reed-Sternberg (HRS) cells, the malignant cells in Hodgkin Lymphoma (HL).Citation21,Citation22 The significance became clear with the discovery of a negative feedback mechanism that is hijacked by certain viruses and by HRS cells to quench anti-pathogen or anti-cancer immune responses.Citation23 CD137 on HRS cells can be transferred to CD137L-expressing adjacent APCs, and form a complex with CD137L, which is subsequently internalized, thereby diminishing costimulation of a T cell response against HL.Citation21 This trogocytic transfer of CD137 and the subsequent depletion of CD137L from APC helps HL to evade immune surveillance.Citation17,Citation24,Citation25
Here, we report that the same negative feed-back mechanism is also hijacked by RMS. Ectopic expression of CD137 on RMS cells allows RMS cells to downregulate CD137/CD137L signaling, leading to reduced immune cell activation, cytokine secretion, and tumor cell killing. In addition, CD137 signaling into RMS cells leads to increased IL-6 and IL-8 secretion, which are linked to poor prognosis in RMS. However, the ectopic CD137 expression that malignant RMS cells usurp to evade immune surveillance makes them susceptible as targets for immunotherapy by a CD137-specific chimeric antigen receptor (CAR).
Materials and methods
Patient samples
Tissue microarrays (TMA) containing tissue cores of various types of RMS were purchased from US Biomax, Inc (Rockville, USA) (Catalog number: SO2082b). Types of RMS include spindle cell RMS (n = 10), embryonal RMS (n = 19), pleomorphic RMS (n = 24) and alveolar RMS (n = 20). For some patients, more than one core was provided. Human tonsil formalin-fixed and paraffin-embedded (FFPE) sections, which served as positive controls for staining of immune markers, were obtained from the Department of Pathology of the National University Hospital, Singapore with approval from the Institutional Review Board, Singapore (IRB number: B16-309). Human RMS FFPE sections, which served as positive controls for staining of RMS surface marker, were obtained from Singhealth Tissue Repository, Singapore. Supplementary Table 1 shows the baseline characteristics of patients among different types of RMS.
Multiplex immunofluorescence staining
TMAs were stained using multiplex immunofluorescent system (Opal staining) (Perkin Elmer, Waltham, USA) according to manufacturer’s protocol. Primary antibodies used in the Opal staining were mouse anti-human CD137 (clone: BBK2, Thermo Fisher Scientific, Waltham, USA), human anti-human CD137L (clone: P1F3, Genscript), rabbit anti-human CD3 (Dako, Santa Clara, USA), mouse anti-human CD8a (clone: AMC908; Thermo Fisher Scientific), rabbit anti-human ionized calcium-binding adapter molecule-1 (Iba-1) (FUJIFILM Wako Pure Chemical Corporation, Osaka, Japan) and mouse anti-human MyoD antibodies (clone: 5.8A; Thermo Fisher Scientific). Isotype controls include human (clone: IgG3207, Morphosys), mouse (clone: MOPC-21, Sigma Aldrich), and rabbit IgG (clone: DA1E, Cell Signaling Technology). Secondary antibodies consisted of horseradish peroxidase (HRP) labeled antibodies against rabbit and mouse (GBI Labs, Bothell, USA) and HRP-labeled goat anti-human IgG (H + L) (Thermo Fisher Scientific). Images were taken and analyzed using the Vectra microscope system (Perkin Elmer) and inForm® Cell Analysis™ (Perkin Elmer) at the Genome Institute of Singapore, A*STAR. For each core, number of cells were counted both manually and by inForm® software. Mean number of cells for each surface marker were calculated for each patient. Supplementary Table 2 shows the number of cells per core for each surface marker in each patient.
Generation of CD137-expressing RMS cell lines
The human RMS cell lines Rd18, Rh41, Rd and JR1 were kindly provided by Professor Reshma Taneja (The National University of Singapore, Singapore) and Dr Rossella Rota (Childrens’ Hospital Bambino Gesu, Roma, Italy). The cDNA for full length CD137 was cloned into pLenti6 vector (Addgene, Massachusetts, USA), and lentiviral particles were produced using HEK293T cells with the Lenti-vpak packaging kit (Origene, Maryland, USA) for transduction of Rd18 cells. Transduced Rd18 cells were then selected with medium containing 12.5 μg/ml of blasticidin (Santa Cruz Biotechnology Inc, Santa Cruz, USA) for 14 days. Rh41 was transfected with TurboFect (#R0532, Thermo Scientific) and selected with Geneticin Selective Antibiotic (G418 Sulfate) at 120 μg/ml (Thermo Scientific). Parental (Rd18 and Rh41) and CD137-expressing (Rd18-CD137 and Rh41-CD137) RMS cells were cultured in DMEM (Biowest, Utah, USA) supplemented with 10% fetal bovine serum (FBS).
Induction of CD137 expression on RMS cell lines
Parental RMS cell lines (Rd18, Rh41, Rd and JR1) were seeded in tissue culture plates at a density of 5×105 cells/well. 75 ng/ml of TNF was added to RMS cells for 24 h. Untreated cells serve as controls. Cells were then harvested for flow cytometry.
Activation of RMS cells
CD137-expressing and parental RMS cells were cultured at a density of 2×105 cells/well in tissue culture plates coated with either 5 μg/ml of recombinant human CD137L (R&D Systems, Minneapolis, MN) in phosphate-buffered saline (PBS) or 5 μg/ml of bovine serum albumin (BSA) in PBS for 24 h. Supernatants were collected and stored at −80°C.
Trogocytosis experiment
CD137-expressing and parental RMS cell lines were seeded in tissue culture plates at a density of 5×105 cells/well. DG-75 cells (DSMZ; Braunschweig, Germany), which are B cells expressing CD137L, were first labeled with CellTrace™ Violet Cell Proliferation Kit (Thermo Fisher Scientific), and then added to the respective RMS cell lines at a cell ratio of 1:2. DG-75 cells were harvested after 20 min, 2 h and 24 h of co-culture for flow cytometry.
To determine if cell-to-cell contact is required for downregulation of CD137L, CD137L-expressing DG-75 cells were cocultured with control or Rd18-CD137 cells at a ratio of 1:1. Alternatively, conditioned supernatants of control or Rd18-CD137 cells, untreated or treated with either 5 μg/ml of recombinant CD137L protein or BSA, were added. CD137L levels on DG-75 cells were determined by flow cytometry after 24 h. To further demonstrate the transfer of CD137 between RMS cells and DG-75 cells, another set of trogocytosis experiment was performed. Rd18 and Rd18-CD137 cells were labeled with CellTrace Violet, and DG-75 cells were labeled with Vybrant DIL (Thermo Fisher Scientific). Then, these two cell types were cocultured at a 1:1 ratio for 20 h, and then stained for CD137L by flow cytometry.
Peripheral blood mononuclear cell co-culture
All blood samples were obtained from healthy donors at the Health Sciences Authority, Singapore with approval from the Institutional Review Board, Singapore (IRB number: 13–079E) in accordance with the guidelines of the Health Sciences Authority of Singapore. Peripheral blood mononuclear cells (PBMC) were prepared by Ficoll-Pague (GE Healthcare, Chicago, USA) density gradient centrifugation. Isolated PBMC were stimulated with 0.5 ng/ml of anti-human CD3 antibody (clone OKT3; Biolegend, San Diego, USA) and added to tissue culture wells seeded with either CD137-expressing or parental RMS cell lines (2x105 cells/well) at a cell ratio of 5:1 for up to 3 days. PBMC were then harvested for flow cytometry and supernatants were stored at −80°C.
Flow cytometry
RMS and DG-75 cells were stained with PE-conjugated anti-human CD137 (BD Pharmingen, San Jose, USA) and APC-conjugated anti-human CD137L antibodies (eBioscience, CA, USA). PBMC were stained with PE-conjugated anti-human CD137 (BD Pharmingen), APC-conjugated anti-CD3 antibody (eBioscience) and Efluor 450-conjugated anti-human CD69 (Biolegend) antibodies. RMS cells harvested after co-culture were stained with Efluor 450-conjugated anti-human CD45 antibody (eBioscience), FITC Annexin V Apoptosis Detection Kit (BD Pharmingen) and 7-AAD Viability Staining Solution (Biolegend). The isotype control was mouse-IgG1k (eBioscience, BD Pharmingen and Biolegend). CountBright™ Absolute Counting Beads were used to determine the absolute number of cells (Thermo Fisher Scientific). All results were obtained using Fortessa Analyzer (BD Biosciences, New Jersey, USA) and analyzed using FlowJo software (FlowJo, LLC, Ashland, USA).
Generation of anti-CD137 CAR-NK cells
KHYG-1 cells were kindly provided by Professor Jeak Ling Ding (The National University of Singapore). Anti-CD137 antibodies were isolated by phage display technology.Citation26 The anti-CD137-CAR gene was constructed by combining the anti-CD137 single-chain fragment, the CD8 hinge and transmembrane domain, CD137 and CD3z intracellular domain. The anti-CD137-CAR gene was cloned into pLenti6-V5 D/TOPO vector and generated 3rd generation lentiviruses together with pRSV-Rev, pMDLg/pRRE, and pMD2.G plasmids (Addgene). Anti-CD137-CAR KHYG-1 cells were generated by lentiviral spin-inoculation and sorting by flow cytometry.
Cell lysis assay
5x103 RMS cells were seeded on 96-well plate overnight before being co-cultured with KHYG-1 cells at an E:T = 10:1 ratio for 24 h. The supernatants were collected for the measurement of LDH activities by CyQUANT™ LDH Cytotoxicity Assay (Thermo Fisher Scientific). The percentage of cell lysis was calculated according to manufacturer’s protocol.
Fusion gene detection of RMS cell lines
Total RNA was extracted from cell pellets using the Promega ReliaPrep™ FFPE Total RNA Miniprep System (Promega, Madison, WI, USA) following the recommended protocol. The quantity of RNA extracted was measured using the spectrophotometer. 200 ng of RNA was used for Nanostring Elements fusion protocol on the NanoString nCounter Analysis system (NanoString Technologies) according to the manufacturer’s instructions. The target code set tags are specific to detecting 174 fusion variants across 24 sarcoma types including the following gene fusions which are known to occur in alveolar rhabdomyosarcoma PAX3-FOXO1, PAX7-FOXO1, PAX3-FOXO4, PAX3-NCOA1, PAX3-NCOA2, PAX3-MAML3, and PAX3-INO80D.Citation27
Enzyme-linked immunosorbent assay (ELISA)
Levels of TNF, IL-2, IL-6, and IL-8 in supernatants were measured using the respective Human ELISA kits (Invitrogen, San Diego, USA). Levels of IL-10 and granulocyte-monocyte colony stimulating factor (GM-CSF) were measured using respective Human ELISA MAX kits (Biolegend). Levels of IFNγ were measured using Human IFN-Gamma ELISA Kit (R&D). All experiments were performed according to manufacturer’s protocol and measurements were performed in duplicates. Readings were analyzed using Sunrise™ microplate reader (Tecan Group Ltd., Männedorf, Switzerland).
Multiplex assay
Cytokine levels in RMS culture supernatants were determined by a 13-plex cytokine multiplex assay comprising IL-1β, IL-6, IL-8, IL-10, IL-12p70, IL-13, IL-15, IL-17A, IFNβ, IFNγ, LT-α, MCP-1, and TNF ProcartaPlex™ Singlexes (ThermoFisher Scientific) which was run on a MAGPIX® System (Luminex Corporation, Austin, USA).
Statistical analysis
Statistical significance was determined by Student t-test and Mann Whitney test using GraphPad Prism software (San Diego, CA, USA). A p value of <0.05 was considered as significant.
Results
CD137 is expressed in RMS tissue
A total of 134 cores from 73 patients were analyzed on the TMA. One case (patient 34) was excluded from analysis due to insufficient core material. Sixty-six cores (49.2%) stained positive for CD137. CD137 expression was found on CD3+ T cells in 56 out of 134 cores (37.6%) across all RMS subtypes. While the percentages of cores with CD137+, CD3+ cells were around 45% for ARMS, PRMS, and SC-RMS, only 31% of ERMS had CD137+ T cells (). CD137-expressing RMS cells (CD137+, CD3−) were present in all four types of RMS with 27% of ARMS cores and around 45% for ERMS, PRMS and SC-RMS cores having CD137+ RMS cells (). There was no significant correlation of CD137 expression with patient age (not shown).
Figure 1. CD137 expression in RMS tissue cores. (a) Percentages of tissue cores with CD137 expression among different types of RMS. Number of (b) CD3+ T cells, (c) CD3+CD137+ T cells and (d) CD3−CD137+ cells in different types of RMS. Each symbol represents one patient. Lines represent medians and bars represent interquartile ranges. * p < .05 using Mann Whitney test. ARMS: alveolar rhabdomyosarcoma, ERMS: embryonal rhabdomyosarcoma, PRMS: pleomorphic rhabdomyosarcoma, SC-RMS: Spindle cell-rhabdomyosarcoma
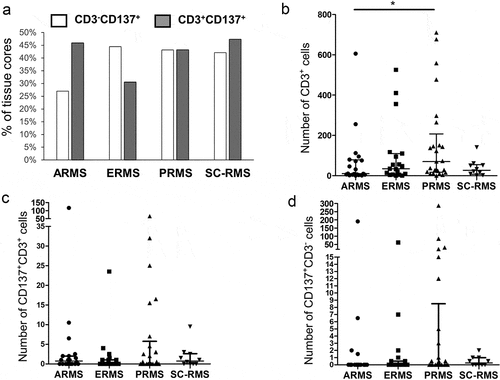
By enumerating total T cells, CD137+ T cells and CD137+ RMS cells using multiplex immunofluorescent staining, we found the highest number of T cells in PRMS although statistical significance was only reached in comparison to ARMS (p = .03) (). There was no significant difference in the number of CD137+ cells (T or RMS cells) among the different subtypes of RMS. However, in some patients exceptionally high numbers of CD137+CD3− cells were identified, especially in PRMS ().
CD137 is expressed on cytotoxic T cells in RMS
Since CD137 expression is dependent on T cell receptor engagement, CD137 labels antigen-specific activated T cells,Citation28 and their presence could be evidence of an ongoing anti-RMS T cell response. However, Treg have been shown to express high levels of CD137, and CD137+ Treg are more immunosuppressive than CD137− Treg.Citation29,Citation30
In order to gain insight into the role of CD137+ T cells in RMS, we identified their subtype. Many CD137+ T cells could be detected in several ERMS and PRMS cores, that coexpressed CD8a, demonstrating the presence of cytotoxic T cells. Representative stainings are shown in , and positive and isotype controls are shown in Suppl. Figure 1. This finding is in line with an earlier study that reported the near absence of Treg in RMS.Citation31
Figure 2. CD137 expression on T cells (white arrowheads) and RMS cells (red arrowheads). (a-j) Images from cores of a patient (56/M) with ERMS. A is the image from core G5. B-E are magnified images of the area highlighted in A. F is the image from core G6. White arrowheads indicate colocalization of CD3 and CD8. G-J are magnified images of the area highlighted in F. (k-r) Images from cores of a patient (50/F) with PRMS. K is the image from core F3. L-N are magnified images of the area highlighted in K. O is the image from core F4. P-R are magnified images of the area highlighted in O. Red arrowheads indicate colocalization of CD137 and MyoD. Scale bar = 400 μm
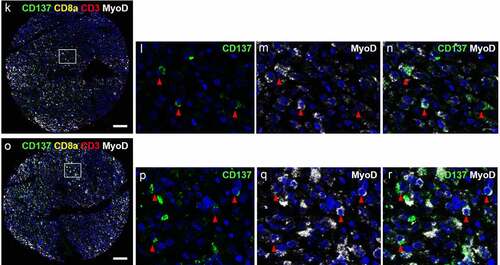
Within the clusters of cytotoxic T cells, characteristic punctuate CD137 staining was seen in some of the CD3+CD8a+ T cells, indicating the formation of a signaling complex. Since CD137 signaling into T cells drives a Th1/Tc1 response, the presence of CD3+CD8a+ T cells would be consistent with an ongoing anti-RMS T cell response.Citation32
We also stained the RMS tissue microarray for CD137L which revealed that CD137L-expressing cells were present in some RMS cores (Suppl. figure 2a, B), indicating that CD137 signaling into CD137-expressing RMS cells and/or cytotoxic T cells can be induced. However, CD137 and CD137L expression levels did not correlate (Suppl. figure 2c).
Ectopic expression of CD137 on RMS cells
CD137 is not only expressed by T cells but also by other cells including NK cells and vascular endothelial cells.Citation33,Citation34 Therefore, we stained for MyoD, a marker of muscle cells, to unequivocally identify RMS cells as being CD137+. MyoD was detected in the nucleus and the cytoplasm, which is in line with a previous study.Citation35 Co-localization of MyoD and CD137 was detected in PRMS, and in general less than 10% of the MyoD+ cells expressed CD137 (). Since CD137 is not found on healthy muscle cells, our finding suggests ectopic expression of CD137 in RMS, or at least some subtypes RMS.
Previous studies have shown that TNF can induce CD137 expression on nonimmune cells such as vascular endothelial cells.Citation36 When RMS cells were exposed to TNF, CD137 expression was induced on four different RMS cell lines, namely Rd18, Rh41, Rd and JR1, with 9–20% of the RMS cells being positive for CD137 (). Aside from TNF, we also tested IL-6, IFNγ, PMA, hydrogen peroxide (H2O2), and hypoxia but no induction of CD137 expression was observed (not shown). As TNF is frequently present in the tumor stroma,Citation37 it could be responsible for the ectopic CD137 expression on malignant muscle cells.
Figure 3. Induction of CD137 expression on RMS cells by TNF and CD137 signaling into RMS cells induces IL-6 and IL-8 secretion. (a) Rd18, Rh41, Rd and JR1 cells were cultured with 75 ng/ml of TNF for 24 h. CD137 expression was measured by flow cytometry. Numbers in the histogram represent percentages of cells with CD137 staining. Two independent experiments for each cell line were performed with consistent results. (b-c) CD137-expressing and parental RMS cell lines were activated with either recombinant CD137L protein or BSA (negative control) for 24 h. IL-6 and IL-8 levels were determined by ELISA. Results are pooled from three independent experiments and presented as means ± errors of means. ** p < .01 using two-sided unpaired t-test
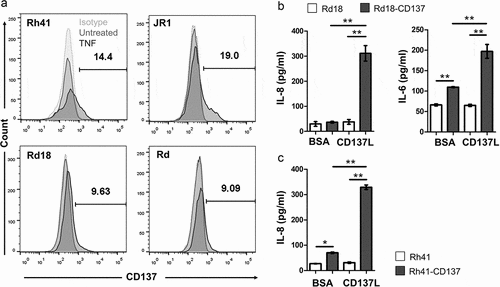
CD137 signaling into RMS cells induces cytokine secretion
In order to understand the effect of ectopic CD137 expression in RMS, we generated two stable CD137-expressing RMS cell lines (Rd18-CD137 and Rh41-CD137) to be used for functional experiments. Parental Rd18 and Rh41 cells do not express CD137 ( and Suppl. Figure 3). Expression of CD137 by itself increased the baseline secretion of IL-6 in Rd18 cells, and of IL-8 in Rh41 cells. Activation with rCD137L protein increased IL-6 and IL-8 secretion 2–6 fold in Rd18-CD137 cells (), and IL-8 more than 4-fold in Rh41-CD137 cells (). No IL-6 secretion was observed in Rh41 cells, neither in CD137-expressing cells nor in parental cells. These findings suggest a functional role for the ectopically expressed CD137 in enhancing cytokine secretion by RMS cells. As Rd18 and Rh41 are of embryonal and alveolar origin, respectively, it is uncertain whether the subtype of RMS influences the function of CD137 in RMS.
In order to investigate whether other cytokines are induced by CD137 signaling, we performed a multiplex assay. Induction of IL-6 and IL-8 as shown in previous ELISA was confirmed. In addition, induction of MCP1 and GM-CSF was found for Rd18-CD137 and Rh41-CD137 cells, respectively (Suppl. Figure 4). IL-1β, IL-10, IL-12p70, IL-13, IL-15, IL-17A, IFNβ, IFNγ and TNFβ were not detected.
The RMS classification system is partly based on the fusion gene status. The PAX3-FOXO1 (or PAX7-FOXO1) fusion gene is generated as a result of reciprocal translocation of chromosome 2 (or chromosome 1) and chromosome 13, which leads to a fusion between the DNA binding domain of PAX3 (or PAX7) and the transactivation domain of FOXO1.Citation38 Cytogenetic profiling of Rd18 and Rh41 cell lines revealed a different fusion protein status. Rd18 was found to be negative for ARMS gene fusions, while Rh41 was identified to have PAX3 (exon 7)-FOXO1 (exon 2), (normalized probe count of 89.71, where counts >5 indicate a positive result for the corresponding gene fusion). The fact that both Rd18 and Rh41 cells show similar cytokine secretion profiles, suggests that the fusion status may not have an influence on the function of CD137 in RMS. Indeed, immunohistochemical staining of fusion-positive and -negative RMS samples revealed that 3 out of 7 and 2 out of 6, respectively, expressed CD137.
CD137 expression on RMS cells downregulates CD137L on APC through trogocytosis
Molecules that are ectopically expressed by tumors generally provide a growth and/or selection advantage which drives their expression. Since trogocytic transfer of CD137 is a negative feed-back mechanism regulating CD137L levels,Citation23,Citation39 we tested if CD137-expressing RMS cells are able to downregulate CD137L on adjacent APC. As APC we used DG-75, a Burkitt lymphoma cell line, which constitutively expresses CD137L but not CD137.
CD137L expression decreased much more substantially on those DG-75 cells that were co-cultured with Rd18-CD137 compared to those co-cultured with parental Rd18 cells, and this decrease was observed already after 20 min (not shown). The decrease in CD137L became more pronounced at 24 h of co-culture (from 47% to 3.5%) () and this decrease in CD137L levels was statistically significant (). A parallel increase in CD137 level on was observed at the same time (from 0.4% to 1.2%), suggesting a transfer of CD137 from Rd-CD137 cells to DG-75 cells (). The same trend was observed with Rh41-CD137 cells (). Cell to cell contact was required between RMS cells and DG-75 cells as conditioned supernatant of control or CD137-expressing Rd18 cells was not sufficient for downregulation of CD137L (Suppl. figure 5). Even conditioned supernatant of rhCD137L-treated RMS cells could not downregulate CD137L (Suppl. figure 6). During the cell to cell contact, trogocytosis occurred as demonstrated by the enhanced exchange of membrane fragments between the Rd18 and DG-75 cells when RMS cells expressed CD137 (Suppl. figure 7a), and the concurrent transfer of CD137L to the RMS cells (Suppl. figure 7b).
Figure 4. Downregulation of CD137L on DG-75 by CD137-expressing RMS cells. CD137-expressing and parental RMS cells were seeded on tissue culture plates. CellTrace™ Violet-labeled CD137L-expressing DG-75 cells were added to RMS cells at a ratio of 1:2. Cells were collected 24 h later for flow cytometry. DG-75 cells without RMS cell co-culture serve as baseline. Flow cytometry results of (a) Rd18-CD137 and Rd-18 are shown. Numbers in the scatter plots represent percentages of respective cell populations. Experiments were repeated twice for each cell line with consistent results. (b) Comparison of the percentages of CD137L-expressing DG-75 cells during coculture with Rd18-CD137 and Rd18, respectively. Each symbol represents one independent experiment. Lines represent means ± standard errors of means. * p < .05 using two-sided unpaired t-test. Experiments were repeated four times for each cell line with consistent results. (c) Coculture as in (A) but with Rh-41 instead of Rd18 and cells
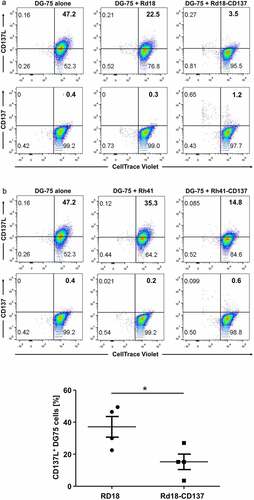
Downregulation of CD137 expression on T cells with reduced RMS killing and secretion of cytokines
APCs such as dendritic cells, monocytes and B cells may interact with RMS cells via trogocytosis leading to downregulation of their CD137L expression. Thus, the downregulation of CD137L on APC by RMS cells is predicted to lead to reduced CD137 signaling and reduced activation of RMS-infiltrating leukocytes. We modeled this situation by co-culturing PBMC of healthy donors (n = 4) with RMS cells. In the presence of Rd18-CD137 cells, expression of CD137 (serving here as a marker for Th1 and Tc1 cell activation) on activated T cells was significantly reduced compared to control Rd18 cells after 24 h of co-culture (p = .005) while expression of CD69, an earlier and more general activation marker, was not changed ().Citation32
Figure 5. Downregulation of CD137 expression on T cells with reduced RMS killing and cytokine secretion. PBMCs isolated from 4 healthy donors were activated with 0.5 ng/ml of anti-CD3 antibody, and cultured with either Rd18-CD137 or parental Rd18 cells for 3 days. Cells were harvested on days 1, 2 and 3 and analyzed by flow cytometry. PBMCs without the RMS cell co-culture provided the baseline. T cells were gated by positive CD3 staining, and RMS cells were gated by negative CD45 staining. Viable RMS cells were defined as CD45−, Annexin V−, 7-AAD− and the number of viable cells was calculated using counting beads. (a) CD137 and CD69 expression on T cells of one donor is shown. Numbers in the histogram represent percentages of cells with CD137 or CD69 staining. (b) Normalized ratios (Rd18-CD137/Rd18) of percentages for CD137 and CD69 expression. (c) Number of viable RMS cells at day 2 and day 3. (d) Normalized ratios (Rd18-CD137/Rd18) of cytokine levels at day 1, 2 and 3 of co-culture. Each symbol represents one donor. A ratio of 1 (dotted line) indicates no difference between Rd18-CD137 and Rd-18 control. Lines and bars represent means ± standard errors of means. * p < .05 and ** p < .01 using two-sided unpaired t-test
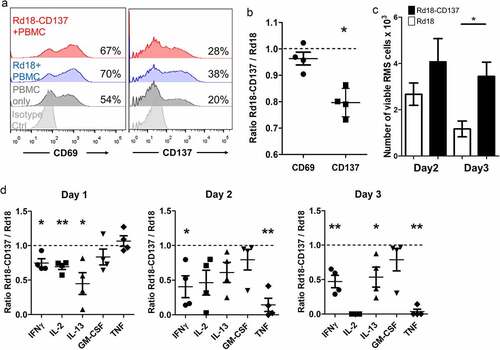
When the RMS cells expressed CD137 they were protected from being killed by the activated PBMC as shown by the higher number of remaining viable RMS cells at day 2 and day 3 of co-culture (). More Rd18-CD137 cells than Rd18 control cells survived, and the difference was statistically significant after 3 days of co-culture (3.5 ± 0.6 x103 vs 1.2 ± 0.3 x103, p = .02).
We also investigated the effect of CD137 expression by RMS cells on cytokine release in the RMS cell – PBMC co-culture. Since cytokine levels vary among different donors, we normalized the concentrations of cytokines of the Rd18-CD137 condition to those of the Rd18 control for each donor. The secretion of T cell cytokines including IFNγ, IL-2 and IL-13 was significantly lower in the co-culture of PBMC with Rd18-CD137 cell than with the parental Rd18 control cells at day 1. IFNγ decreased from day 1 to 2 and remained low until day 3 (). IL-2 concentrations gradually declined over the 3 days, and IL-2 was not detectable anymore by day 3. The release of IL-13 was also reduced, while GM-CSF was not affected. The levels of TNF were not changed on day 1 but had dramatically decreased by day 2 and day 3 ( and Suppl Fig. 8).
These data demonstrate that the downregulation of CD137L levels by CD137-expressing RMS cells leads to a reduced CD137-mediated costimulation of T cells, which may translate to a reduced anti-RMS T cell response, and an enhanced survival of RMS cells.
Anti-CD137 CAR- KHYG-1 cells specifically kill CD137-expressing RMS cells
The ectopic expression of CD137 could provide RMS with growth and selection advantages. However, it may make RMS also susceptible to immunotherapy by targeting CD137. For proof of concept, we generated an anti-CD137 CAR that targets CD137 cell surface expression, and armed it on the NK cell line KHYG-1. Higher percentages of RMS cell lysis were seen when CD137-expressing Rd18 or Rh41 cells were treated with anti-CD137 CAR-KHYG-1 cells, compared to Rd18 controls and KHYG-1 controls (). This higher cytolytic activity was accompanied by significantly higher levels of TNF and IFNγ secretion, indicating a specific activation of the anti-CD137 CAR-KHYG-1 cells by CD137-expressing RMS cells (). This finding opens the possibility of developing a novel immunotherapeutic treatment approach for RMS.
Figure 6. Anti-CD137 CAR-KHYG-1 cells specifically kill CD137-expressing RMS cells. 5000 RMS cells were co-cultured with KHYG-1 cells at a ratio of 1:10 for 24 h. The supernatants were collected for the measurement of LDH activities and cytokine levels. (a) Percentages of RMS cell lysis (b) IFNγ level (c) TNF level. Top row: Rd18 cells, bottom row: Rh41 cells. Results are pooled from three independent experiments and presented as means ± standard errors of means. ** p < .01 using two-sided unpaired t-test
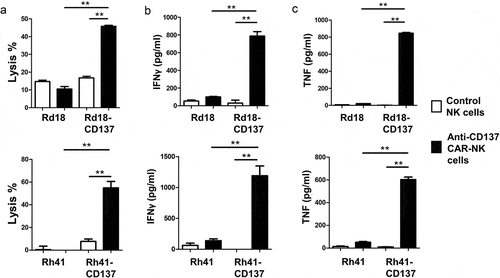
Discussion
In this study, we demonstrate evidence that RMS, similar as HL, can hijack an immunoregulatory feed-back mechanism that helps it to evade immune surveillance. By ectopically expressing CD137, and transferring it to APC via trogocytosis, RMS cells downregulate CD137L on APCs in vitro which reduces costimulation of T cells and polarization toward a Th1-mediated cytotoxic T cell response, secretion of the T cell cytokines IL-2 and IFNγ, and most importantly, the killing of RMS cells by the PBMC ().
Figure 7. Schematic diagram illustrating the proposed role of CD137 in RMS. (a) Via CD137L – CD137, APC costimulate activated T cells which kill RMS cells. (b) TAM induce ectopic CD137 expression on RMS cells via TNF. CD137 on RMS cells downregulates CD137L on APC via trogocytosis, reducing T cell costimulation and thus anti-tumor response. CD137 signaling into RMS cell induces secretion of IL-6 and IL-8 which drive RMS growth and metastasis. (c) NK cells transduced with an anti-CD137 CAR kills RMS cells that ectopically express CD137
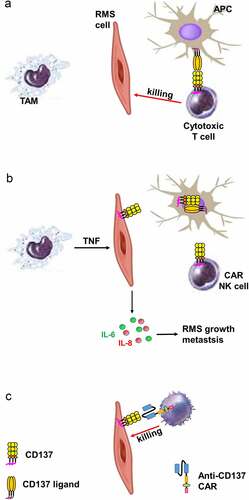
CD137L levels on APC were reduced within 20 min of contact with CD137-expressing RMS cells, which is consistent with trogocytosis being a fast process.Citation40 Since CD137L levels return to near normal within 3 hours after CD137-expressing cells are removed, the continuous presence of CD137 on RMS cells is required for long-term suppression of an anti-RMS immune response.Citation39
Under physiological conditions, CD137 is expressed by leukocytes and vascular endothelial cells. However, CD137 expression can be induced ectopically on nonimmune cells and malignant cells. Epstein Barr virus-encoded latent membrane protein-1 (LMP-1) induces CD137 expression on HRS cells in HL via the PI3K-AKT-mTOR pathway.Citation41,Citation42 Similarly, the human T-lymphotropic virus-1 protein tax induces CD137 on NK/T cell lymphomas.Citation43 Since RMS cells originate from mesenchymal myogenic progenitors with no known association to oncogenic viruses, we hypothesized that molecules in the tumor microenvironment may induce the ectopic CD137 expression. A prime candidate is TNF since CD137 is expressed by blood vessel walls in inflammatory tissues and in tumors, and is induced on vascular endothelial cells by TNF.Citation34,Citation36
Ectopic CD137 expression was induced by TNF in all four RMS cell lines, representing the ERMS and ARMS subtypes. Neither parental nor CD137-expressing RMS cells secreted TNF implying stromal cells in RMS microenvironment to be the source of TNF. This is in agreement with studies demonstrating that, in mice on high-fat diet, CD137 expression was induced on inflamed skeletal muscle cells by obesity-induced factors, including TNF, and this observation was accompanied by an increased infiltration of macrophages.Citation44 Macrophages are major producers of TNF during inflammation and are present as tumor-associated macrophages (TAM) at high numbers in the tumor stroma of RMS.Citation31 It can therefore by hypothesized that the ectopic CD137 expression on RMS cells is – at least partly – due to TNF, released by tumor-associated macrophages.
Besides augmenting inflammation, TNF can support tumorigenesis by enhancing the epithelial–mesenchymal transition, promoting metastasis and negatively regulating anti-tumor immune responses.Citation45 TNF secreted by TAM upregulates PD-L1 expression, which leads to immune suppression.Citation46 In our study, we identify another potential mechanism of how TAM suppress tumor cell killing. TNF secreted by TAM may induce CD137 expression on RMS cells, which then downregulates CD137L expression on APCs and CD137-mediated costimulation of T cells.
TNF is likely not the only factor responsible for CD137 expression in RMS as only about 10% of the malignant cells in RMS express CD137. Cases with CD137-expressing RMS cells were more frequent in ERMS, PRMS and SC-RMS than in ARMS. The exact reason for this is not known. However, 80% of ARMS cases have PAX3-FOXO1 or PAX7-FOXO1 fusion genes while these chromosomal aberrations are absent in ERMS, PRMS and SC-RMS.Citation38
Another interesting correlation is that the frequency of Ras gene mutations correlates with the absence of Pax gene fusions, i.e., Ras mutations are completely absent in fusion-positive ARMS.Citation47 Since oncogenic K-ras has been shown to promote CD137 expression in pancreatic cancer, mutated Ras may contribute to the higher prevalence of CD137+ cases in ERMS, PRMS and SC-RMS.Citation48 Although hypoxia has been shown to induce CD137 in malignant cells, we could not confirm that for RMS cell lines.Citation49
Ectopic CD137 expression on RMS also allows signaling into the malignant cells, leading to secretion of IL-6 and IL-8. IL-8 secretion was induced in both Rd18-CD137 and Rh41-CD137 cells, which are of embryonal and alveolar origin, respectively. IL-8 has been shown to act as a growth factor for malignant cells, and to sustain their expansion. Further, IL-8 supports tumor angiogenesis through induction of endothelial cell proliferation and migration, as well as through recruitment of inflammatory cells that release pro-angiogenic factors.Citation50,Citation51
RMS are highly vascularized tumors, and IL-8 has been demonstrated to be the pivotal angiogenic factor secreted by RMS cells via the PI3K/Akt and MAPK pathways in hypoxic conditions to promote vascularization of the growing RMS.Citation52 In addition, CD137 signaling induced IL-6 secretion in Rd18-CD137 cells. Interaction of IL-6 with its receptor IL-6Rα and receptor subunit GP130 activates the PI3K/Akt, Ras/MEK/ERK and JAK/STAT3 pathways.Citation53,Citation54 Among all these downstream effectors, STAT3 and Akt, in particular, mediate proliferation, apoptotic resistance and metastasis of rhabdomyosarcoma, suggesting the role of IL-6 in promoting the oncogenic transformation of RMS.Citation55–57 Since IL-6 and IL-8 contribute to RMS pathogenesis, and are upregulated by CD137 signaling in RMS cells, an approach that neutralizes CD137 on RMS but on effector T cells, should be of benefit for RMS patients.
Information concerning the immune cell profile in RMS is limited. One study identified increased leukocyte infiltration in adolescent and adult types of RMS compared to pediatric RMS, suggesting age-related immune profile differences and possibly a prognostic significance.Citation58 In this study, we analyzed the prevalence of cytotoxic T cells among the different subtypes of RMS. We could not find a significant correlation between age and the number of T cells, but PRMS has significantly more T cells than ARMS. CD137+ T cells were found in all subtypes with no major difference.
For evaluating the effect of RMS-expressed CD137 on immune functions, we cocultured RMS cells with PBMC, since PBMC, like the tumor microenvironment, contains several different leukocyte subpopulations. The presence of CD137 on RMS cells reduced RMS cell killing. Since the MHC status of the PBMC and RMS cells is unknown, this killing may not have been based on cognate interaction. However, whether allogeneic or syngeneic, T cells are being costimulated by CD137L, and reducing CD137L levels by adding CD137-expressing RMS cells reduces T cell costimulation.
As the coculture of RMS cells with PBMC is a complex system, it raises the question about the mechanism underlying the reduced T cell activity. The fact that immobilized recombinant CD137 protein also leads to a reduced activation status of PBMCCitation59,Citation60 indicates that reduced T cell activity was indeed due to RMS-expressed CD137.
Current treatments for RMS include chemotherapy, radiation therapy and surgery. Although these conventional therapies achieve a favorable outcome in around 60% of pediatric and adolescent RMS patients, the overall prognosis for patients with metastatic and recurrent RMS remains poor.Citation4,Citation5 For those patients, an immunotherapy-based treatment either as a second-line therapy or a combination therapy may be advantageous.Citation61
CD137 is expressed on MyoD+ tumor cells, and therefore CD137 may serve as a target in a CAR-based treatment. Anti-CD137 CAR-NK cells are activated by and specifically kill CD137+ RMS cells. Although only about 10% of malignant cells in RMS express CD137, a larger anti-tumor effect is possible via a bystander effect, e.g., the induction of immunogenic cell death.Citation62 Further, the killing of the CD137-expressing RMS cells would deprive all malignant cells of the downregulation of CD137L and reduced T cell costimulation, and of the tumor-promoting effects of CD137-induced IL-6 and IL-8. CD137 is expressed strongly by Treg.Citation29,Citation30 Their elimination together with the CD137+ RMS cells would be an extra gain.
However, other cells that express CD137, such as activated T cells, NK cells and vascular endothelial cells, may also be depleted by anti-CD137 CAR-NK cells. The transient depletion of activated effector T cells and NK cells may be acceptable for the benefit of a significant reduction in tumor mass and/or progression. The killing of CD137-expressing vascular endothelial cells may even be beneficial since CD137 is expressed by blood vessels at sites of inflammation, particularly in tumors,Citation34 and could help in starving the tumor by reducing its vascularization. An adverse effect however would be expected if the patient would suffer from non-tumor-related inflammation and a destruction of CD137+ blood vessels in non-tumor tissue. One of the potential solutions is to further engineer CAR-NK cell activity by logic gates so that they specifically target malignant RMS cells.
Our study identifies ectopic CD137 expression on RMS, which may facilitate immune evasion by downregulating the anti-tumor response of activated T cells. Anti-CD137 CAR-NK cells specifically kill CD137-expressing RMS cells. These findings open up the opportunity of developing a novel immunotherapy for RMS.
Disclosure of Potential Conflicts of Interest
No potential conflicts of interest were disclosed.
Authors’ contributions
Kang Yi Lee and Hiu Yi Wong did the immunofluorescence staining and the functional in vitro experiments. Qun Zeng generated and tested the anti-CD137 CAR. Man Si Cheng and Jia Le Lin generated the RMS-CD137 cell lines. Kuick Chik Hong, Tou En Kenneth Chang, Amos Hong Peng Loh did the cytogenetic analysis and provided patient material. Hiu Yi Wong and Herbert Schwarz wrote the manuscript. Herbert Schwarz supervised the study.
Supplemental Material
Download ()Acknowledgments
The authors would like to thank Professor Reshma Taneja and Professor Jeak Ling Ding of the National University of Singapore, and Dr Rossella Rota, Childrens’ Hospital Bambino Gesu, Roma, Italy for providing us with RMS cell lines and KHYG-1 cells. We would also like to thank the Life Sciences Institute flow cytometry core facility under the leadership of Dr Paul Hutchinson for excellent assistance. We would also like to thank Mr Shivaji Rikka of Genome Institute of Singapore, A*STAR for their technical support in Vectra microscope system. This study was funded by the National Medical Research Council, Singapore (NMRC/OFIRG/0022/2016).
Supplementary material
Supplemental data for this article can be accessed on the publisher’s website.
Additional information
Funding
References
- Soleimani VD, Rudnicki MA. New insights into the origin and the genetic basis of rhabdomyosarcomas. Cancer Cell. 2011;19(2):157–13. doi:10.1016/j.ccr.2011.01.044.
- Lizard-Nacol S, Mugneret F, Volk C, Turc-Carel C, Favrot M, Translocation PT. 2;13)(q37;q14) in alveolar rhabdomyosarcoma: a new case. Cancer Genet Cytogenet. 1987;25(2):373–374. doi:10.1016/0165-4608(87)90202-0.
- Xia SJ, Pressey JG, Barr FG. Molecular pathogenesis of rhabdomyosarcoma. Cancer Biol Ther. 2002;1(2):97–104. doi:10.4161/cbt.51.
- Dasgupta R, Fuchs J, Rodeberg D. Rhabdomyosarcoma. Semin Pediatr Surg. 2016;25(5):276–283. doi:10.1053/j.sempedsurg.2016.09.011.
- Hibbitts E, Chi YY, Hawkins DS, Barr FG, Bradley JA, Dasgupta R, Meyer WH, Rodeberg DA, Rudzinski ER, Spunt SL, et al. Refinement of risk stratification for childhood rhabdomyosarcoma using FOXO1 fusion status in addition to established clinical outcome predictors: a report from the Children’s Oncology Group. Cancer Med. 2019;8(14):6437–6448. doi:10.1002/cam4.2504.
- Schwarz H, Tuckwell J, Lotz M. A receptor induced by lymphocyte activation (ILA): a new member of the human nerve-growth-factor/tumor-necrosis-factor receptor family. Gene. 1993;134(2):295–298. doi:10.1016/0378-1119(93)90110-O.
- Takahashi C, Mittler RS, Vella AT. Cutting edge: 4-1BB is a bona fide CD8 T cell survival signal. J Immunol. 1999;162:5037–5040.
- Wang S, Chen L. Immunobiology of cancer therapies targeting CD137 and B7-H1/PD-1 cosignal pathways. Curr Top Microbiol Immunol. 2011;344:245–267. doi:10.1007/82_2010_81.
- Vinay DS, Kwon BS. 4-1BB (CD137), an inducible costimulatory receptor, as a specific target for cancer therapy. BMB Rep. 1999;162(3):5037–5040. doi:10.5483/BMBRep.2014.47.3.283.
- Guillerey C, Nakamura K, Pichler AC, Barkauskas D, Krumeich S, Stannard K, Miles K, Harjunpaa H, Yu Y, Casey M, et al. Chemotherapy followed by anti-CD137 mAb immunotherapy improves disease control in a mouse myeloma model. JCI Insight. 2019;5:5. doi:10.1172/jci.insight.125932.
- Sanmamed MF, Etxeberria I, Otano I, Melero I. Twists and turns to translating 4-1BB cancer immunotherapy. Sci Transl Med. 2019;11(496):eaax4738.
- Wang KC, Chu KL, Batista NV, Watts TH. Conserved and Differential Features of TNF Superfamily Ligand Expression on APC Subsets over the Course of a Chronic Viral Infection in Mice. Immunohorizons. 2018;2(11):407–417. doi:10.4049/immunohorizons.1800047.
- Shao Z, Schwarz H. CD137 ligand, a member of the tumor necrosis factor family, regulates immune responses via reverse signal transduction. J Leukoc Biol. 2011;89(1):21–29. doi:10.1189/jlb.0510315.
- Kwajah MMS, Schwarz H. CD137 ligand signaling induces human monocyte to dendritic cell differentiation. Eur J Immunol. 2010;40(7):1938–1949. doi:10.1002/eji.200940105.
- Harfuddin Z, Kwajah S, Chong Nyi Sim A, Macary PA, Schwarz H. CD137L-stimulated dendritic cells are more potent than conventional dendritic cells at eliciting cytotoxic T-cell responses. Oncoimmunology. 2013;2(11):e26859. doi:10.4161/onci.26859.
- Dharmadhikari B, Nickles E, Harfuddin Z, Ishak NDB, Zeng Q, Bertoletti A, Schwarz H. CD137L dendritic cells induce potent response against cancer-associated viruses and polarize human CD8+ T cells to Tc1 phenotype. CII. 2018;67(6):893–905. doi:10.1007/s00262-018-2144-x.
- Zeng Q, Soe YM, Lim Y, Sobota RM, Schwarz H. CD137 ligand interacts with CD32a to trigger reverse CD137 ligand signaling. Cell Mol Immunol. 2020 Nov;17(11):1188-1189.
- Moh MC, Lorenzini PA, Gullo C, Schwarz H. Tumor necrosis factor receptor 1 associates with CD137 ligand and mediates its reverse signaling. FASEB Journal: Official Pub Federat Am Soc Experimen Biol. 2013;27(8):2957–2966. doi:10.1096/fj.12-225250.
- Setareh M, Schwarz H, Lotz M. A mRNA variant encoding a soluble form of 4-1BB, a member of the murine NGF/TNF receptor family. Gene. 1995;164(2):311–315. doi:10.1016/0378-1119(95)00349-B.
- Michel J, Langstein J, Hofstadter F, Schwarz H. A soluble form of CD137 (ILA/4-1BB), a member of the TNF receptor family, is released by activated lymphocytes and is detectable in sera of patients with rheumatoid arthritis. Eur J Immunol. 1998;28(1):290–295. doi:10.1002/(SICI)1521-4141(199801)28:01<290::AID-IMMU290>3.0.CO;2-S.
- Ho WT, Pang WL, Chong SM, Castella A, Al-Salam S, Tan TE, Moh MC, Koh LK, Gan SU, Cheng CK, et al. Expression of CD137 on Hodgkin and Reed-Sternberg cells inhibits T-cell activation by eliminating CD137 ligand expression. Cancer Res. 2013;73(2):652–661. doi:10.1158/0008-5472.CAN-12-3849.
- Anderson MW, Zhao S, Freud AG, Czerwinski DK, Kohrt H, Alizadeh AA, Houot R, Azambuja D, Biasoli I, Morais JC, et al. CD137 is expressed in follicular dendritic cell tumors and in classical Hodgkin and T-cell lymphomas: diagnostic and therapeutic implications. Am J Pathol. 2012;181(3):795–803. doi:10.1016/j.ajpath.2012.05.015.
- Wu M, Wong HY, Lin JL, Moliner A, Schwarz H. Induction of CD137 expression by viral genes reduces T cell costimulation. J Cell Physiol. 2019;234(11):21076–21088. doi:10.1002/jcp.28710.
- Shao Z, Harfuddin Z, Pang WL, Nickles E, Koh LK, Trogocytic SH. CD137 transfer causes an internalization of CD137 ligand on murine APCs leading to reduced T cell costimulation. J Leukoc Biol. 2015;97(5):909–919.
- Zeng Q, Schwarz H. The role of trogocytosis in immune surveillance of Hodgkin lymphoma. Oncoimmunology. 2020;9(1):1781334. doi:10.1080/2162402X.2020.1781334.
- Rajendran S, Li Y, Ngoh E, Wong HY, Cheng MS, Wang CI, Schwarz H. Development of a bispecific antibody targeting CD30 and CD137 on Hodgkin and reed-Sternberg cells. Front Oncol. 2019;9:945. doi:10.3389/fonc.2019.00945.
- Chang KTE, Goytain A, Tucker T, Karsan A, Lee CH, Nielsen TO, Ng TL. Development and evaluation of a pan-sarcoma fusion gene detection assay using the nanostring nCounter platform. JMD. 2018;20(1):63–77. doi:10.1016/j.jmoldx.2017.09.007.
- Wolfl M, Kuball J, Ho WY, Nguyen H, Manley TJ, Bleakley M, Greenberg PD. Activation-induced expression of CD137 permits detection, isolation, and expansion of the full repertoire of CD8+ T cells responding to antigen without requiring knowledge of epitope specificities. Blood. 2007;110(1):201–210. doi:10.1182/blood-2006-11-056168.
- Bacher P, Heinrich F, Stervbo U, Nienen M, Vahldieck M, Iwert C, Vogt K, Kollet J, Babel N, Sawitzki B, et al. Regulatory T cell specificity directs tolerance versus allergy against aeroantigens in humans. Cell. 2016;167(4):1067–78 e16. doi:10.1016/j.cell.2016.09.050.
- Kachapati K, Adams DE, Wu Y, Steward CA, Rainbow DB, Wicker LS, Mittler RS, Ridgway WM. The B10 Idd9.3 locus mediates accumulation of functionally superior CD137+ regulatory T cells in the nonobese diabetic type 1 diabetes Model. J Immunol. 2012;189(10):5001–5015. doi:10.4049/jimmunol.1101013.
- Kather JN, Horner C, Weis CA, Aung T, Vokuhl C, Weiss C, Scheer M, Marx A, Simon-Keller K. CD163+ immune cell infiltrates and presence of CD54+ microvessels are prognostic markers for patients with embryonal rhabdomyosarcoma. Sci Rep. 2019;9(1):9211. doi:10.1038/s41598-019-45551-y.
- Dharmadhikari B, Wu M, Abdullah NS, Rajendran S, Ishak ND, Nickles E, Harfuddin Z, Schwarz H. CD137 and CD137L signals are main drivers of type 1, cell-mediated immune responses. Oncoimmunology. 2016;5(4):e1113367. doi:10.1080/2162402X.2015.1113367.
- Melero I, Johnston JV, Shufford WW, Mittler RS, Chen L. NK1.1 cells express 4-1BB (CDw137) costimulatory molecule and are required for tumor immunity elicited by anti-4-1BB monoclonal antibodies. Cell Immunol. 1998;190(2):167–172. doi:10.1006/cimm.1998.1396.
- Broll K, Richter G, Pauly S, Hofstaedter F, Schwarz H. CD137 expression in tumor vessel walls. High correlation with malignant tumors. Am J Clin Pathol. 2001;115(4):543–549. doi:10.1309/E343-KMYX-W3Y2-10KY.
- Wang NP, Marx J, McNutt MA, Rutledge JC, Gown AM. Expression of myogenic regulatory proteins (myogenin and MyoD1) in small blue round cell tumors of childhood. Am J Pathol. 1995;147:1799–1810.
- Drenkard D, Becke FM, Langstein J, Spruss T, Kunz-Schughart LA, Tan TE, Lim YC, Schwarz H. CD137 is expressed on blood vessel walls at sites of inflammation and enhances monocyte migratory activity. FASEB Journal: Official Pub Federat Am Soc Experimen Biol. 2007;21(2):456–463. doi:10.1096/fj.05-4739com.
- Ham B, Fernandez MC, D’Costa Z, Brodt P. The diverse roles of the TNF axis in cancer progression and metastasis. Trends Cancer Res. 2016;11:1–27.
- Sun X, Guo W, Shen JK, Mankin HJ, Hornicek FJ, Duan Z. Rhabdomyosarcoma: advances in molecular and cellular biology. Sarcoma. 2015;2015:232010. doi:10.1155/2015/232010.
- Shao Z, Harfuddin Z, Pang WL, Nickles E, Koh LK, Schwarz H. Trogocytic CD137 transfer causes an internalization of CD137 ligand on murine APCs leading to reduced T cell costimulation. J Leukoc Biol. 2015;97(5):909–919. doi:10.1189/jlb.3A0213-079RRR.
- Joly E, Hudrisier D. What is trogocytosis and what is its purpose? Nat Immunol. 2003;4(9):815. doi:10.1038/ni0903-815.
- Aravinth SP, Rajendran S, Li Y, Wu M, Wong AH, Schwarz H. Epstein–Barr virus-encoded LMP1 induces ectopic CD137 expression on Hodgkin and Reed–Sternberg cells via the PI3K-AKT-mTOR pathway. Leuk Lymphoma. 2019;60(11):2697–2704. doi:10.1080/10428194.2019.1607330.
- Yoshimori M, Imadome K, Komatsu H, Wang L, Saitoh Y, Yamaoka S, Fukuda T, Kurata M, Koyama T, Shimizu N, et al. CD137 expression is induced by Epstein-Barr virus infection through LMP1 in T or NK cells and mediates survival promoting signals. PloS One. 2014;9(11):e112564. doi:10.1371/journal.pone.0112564.
- Pichler K, Kattan T, Gentzsch J, Kress AK, Taylor GP, Bangham CR, Grassmann R. Strong induction of 4-1BB, a growth and survival promoting costimulatory receptor, in HTLV-1-infected cultured and patients’ T cells by the viral Tax oncoprotein. Blood. 2008;111(9):4741–4751. doi:10.1182/blood-2007-10-115220.
- Le NH, Kim C-S, Tu TH, Choi H-S, Kim B-S, Kawada T, Goto T, Park T, Park JH, Yu R. Blockade of 4-1BB and 4-1BBL interaction reduces obesity-induced skeletal muscle inflammation. Mediators Inflamm. 2013;2013:865159. doi:10.1155/2013/865159.
- Chen Y, Tan W, Wang C. Tumor-associated macrophage-derived cytokines enhance cancer stem-like characteristics through epithelial–mesenchymal transition. Onco Targets Ther. 2018;11:3817–3826. doi:10.2147/OTT.S168317.
- Hartley G, Regan D, Guth A, Dow S. Regulation of PD-L1 expression on murine tumor-associated monocytes and macrophages by locally produced TNF-α. Cancer Immunol Immunother. 2020;9(1):1781334–35. doi:10.1007/s00262-017-1955-5.
- Shern JF, Chen L, Chmielecki J, Wei JS, Patidar R, Rosenberg M, Ambrogio L, Auclair D, Wang J, Song YK, et al. Comprehensive genomic analysis of rhabdomyosarcoma reveals a landscape of alterations affecting a common genetic axis in fusion-positive and fusion-negative tumors. Cancer Discov. 2014;4(2):216–231. doi:10.1158/2159-8290.CD-13-0639.
- Glorieux C, Huang P. Regulation of CD137 expression through K-Ras signaling in pancreatic cancer cells. Cancer Communications. 2016;167(1):41. doi:10.1186/s40880-019-0386-4.
- Labiano S, Palazon A, Bolanos E, Azpilikueta A, Sanchez-Paulete AR, Morales-Kastresana A, Quetglas JI, Perez-Gracia JL, Gurpide A, Rodriguez-Ruiz M, et al. Hypoxia-induced soluble CD137 in malignant cells blocks CD137L-costimulation as an immune escape mechanism. Oncoimmunology. 2016;5(1):e1062967. doi:10.1080/2162402X.2015.1062967.
- Li A, Dubey S, Varney ML, Dave BJ, Singh RK. IL-8 directly enhanced endothelial cell survival, proliferation, and matrix metalloproteinases production and regulated angiogenesis. J Immunol. 2003;170(6):3369–3376. doi:10.4049/jimmunol.170.6.3369.
- Long X, Ye Y, Zhang L, Liu P, Yu W, Wei F, Ren X, Yu J. IL-8, a novel messenger to cross-link inflammation and tumor EMT via autocrine and paracrine pathways (Review). Int J Oncol. 1995;147(1):5–12. doi:10.3892/ijo.2015.3234.
- Wysoczynski M, Shin DM, Kucia M, Ratajczak MZ. Selective upregulation of interleukin-8 by human rhabdomyosarcomas in response to hypoxia: therapeutic implications. Int J Cancer. 2010;126(2):371–381. doi:10.1002/ijc.24732.
- Kishimoto T. Signal transduction through homo- or heterodimers of gp130. Stem Cells. 2016;11:37–44.
- Narazaki M, Witthuhn BA, Yoshida K, Silvennoinen O, Yasukawa K, Ihle JN, Kishimoto T, Taga T. Activation of JAK2 kinase mediated by the interleukin 6 signal transducer gp130. Proc Natl Acad Sci U S A. 2016;11(6):1–27. doi:10.1073/pnas.91.6.2285.
- Chen C-L, Loy A, Cen L, Chan C, Hsieh F-C, Cheng G, Wu B, Qualman SJ, Kunisada K, Yamauchi-Takihara K, et al. Signal transducer and activator of transcription 3 is involved in cell growth and survival of human rhabdomyosarcoma and osteosarcoma cells. BMC Cancer. 2007;7(1):111. doi:10.1186/1471-2407-7-111.
- Renshaw J, Taylor KR, Bishop R, Valenti M, De Haven Brandon A, Gowan S, Eccles SA, Ruddle RR, Johnson LD, Raynaud FI, et al. Dual Blockade of the PI3K/AKT/mTOR (AZD8055) and RAS/MEK/ERK (AZD6244) pathways synergistically inhibits rhabdomyosarcoma cell growth in vitro and in vivo. Clinical Cancer Res Offici J Am Associ Can Res. 2013;19(21):5940–5951. doi:10.1158/1078-0432.CCR-13-0850.
- Xiao H, Bid HK, Chen X, Wu X, Wei J, Bian Y, Zhao C, Li H, Li C, Lin J. Repositioning Bazedoxifene as a novel IL-6/GP130 signaling antagonist for human rhabdomyosarcoma therapy. PloS One. 2017;12(7):e0180297. doi:10.1371/journal.pone.0180297.
- Gasparini P, Fortunato O, De Cecco L, Casanova M, Iannó MF, Carenzo A, Centonze G, Milione M, Collini P, Boeri M, et al. Age-related alterations in immune contexture are associated with aggressiveness in rhabdomyosarcoma. Cancers (Basel). 2019;11(9):E1380. doi:10.3390/cancers11091380.
- Schwarz H, Blanco FJ, von Kempis J, Valbracht J, Lotz M. ILA, a member of the human nerve growth factor/tumor necrosis factor receptor family, regulates T-lymphocyte proliferation and survival. Blood. 1996;87(7):2839–2845. doi:10.1182/blood.V87.7.2839.bloodjournal8772839.
- Michel J, Pauly S, Langstein J, Krammer PH, Schwarz H. CD137-induced apoptosis is independent of CD95. Immunology. 1999;98(1):42–46. doi:10.1046/j.1365-2567.1999.00851.x.
- Chen C, Dorado Garcia H, Scheer M, Henssen AG. Current and future treatment strategies for rhabdomyosarcoma. Front Oncol. 2019;8:1458. doi:10.3389/fonc.2019.01458.
- Kroemer G, Galluzzi L, Kepp O, Zitvogel L. Immunogenic cell death in cancer therapy. Annu Rev Immunol. 2013;31(1):51–72. doi:10.1146/annurev-immunol-032712-100008.