ABSTRACT
Exosomes are extracellular vesicles released from numerous types of cells that are involved in multiple tumors development. Exosomes contribute to the modulation of tumor microenvironment (TME) through intercellular communication. As essential immune stromal cells in the TME, tumor-associated macrophages (TAMs) participate in tumor development by mediating angiogenesis, metastasis, chemoresistance, and immune escape. Due to communication with multiple cells in the TME, they exhibit plasticity and heterogeneity during the progress of polarization from monocytes to macrophages. Previous studies suggest that targeting TAMs is a promising therapeutic strategy; however, the detailed mechanism by which TAMs regulate tumor development still remains unclear. In this review, we provide an overview of the roles of exosomes as messengers in the communication between tumor cells and polarization of TAMs; we also describe the effects of their interaction on tumor development. Finally, we comprehensively discussed the potential application of exosomes as the promising tumor immunotherapy strategy.
1. Introduction
The malignant phenotypes of tumors are determined by both the cancer cells themselves and the surrounding tumor microenvironment (TME).Citation1,Citation2 In addition to tumor cells, the TME includes multiple stromal cells, including fibroblasts, lymphocytes, inflammatory cells, endothelial cells, and mesenchymal stem cells;Citation3 these cells are always evolving together with the tumor cells. Cellular communication in the TME significantly influences tumor occurrence and development, as well as invasion, metastasis, and other malignant biological behaviors.Citation4 However, the molecular mechanisms involved in communication between cancer and immune cells have not been fully elucidated. Previous studies have focused on the heterotypic interactions between tumor cells and immune cells through direct contact and secretion of signaling molecules, such as soluble cytokines and chemokines.Citation5 The discovery of exosomes, regulatory agents in the intercellular communication of cancer, provides a new perspective for the in-depth study of tumor immunity. Increased infiltration of tumor-associated macrophages (TAMs) is observed in most human cancer tissues compared with control para-cancer or normal tissue,Citation6–9 suggesting an essential role of TAMs in tumor development. Multiple studies have shown that TAMs interact with multiple cell types that reprogram and induce macrophage polarizationCitation10,Citation11. TAMs lose their killing ability and subsequently acquire an inhibitory phenotype, which promotes tumor development. In this process, exosomes derived from tumor cells play important roles in macrophage polarization. Simultaneously, TAMs release exosomes that contribute to cancer progression. This review focuses on the effects of exosomes on the communication between cancer cells and TAMs, and mainly focuses on the emerging role of engineered exosome-mediated immune therapy. Further, potential applications for exosomes in targeted therapy are addressed with the aim of providing novel options.
2. TAMs
As a vital plastic and heterogeneous population of cells in the TME, TAMs account for 30%-50% of infiltrating immune cells.Citation12 Circulating monocytes extravasate from nearby blood vessels and enter tumor tissue, contributing to their polarization into different phenotypes.Citation13,Citation14
Generally, macrophages differentiate into two main phenotypes: classically activated (M1) and alternatively activated (M2).Citation15,Citation16 In detailed, M2 macrophages are further subdivided into M2a, M2b, M2c, and M2d phenotypes depending on the polarizing stimuli and gene expression profiles.Citation17,Citation18 Classical and alternative types of TAMs occupy two extremes of a phenotypic continuum in which macrophages respond to secreted factors that evoke distinct functional responses. Previous reviewsCitation19,Citation20 have addressed the influential factors of M1 and M2 polarization, as well as the associated phenotypic changes; thus, these topics are not reviewed in detail here. Due to the heterogeneity of macrophages, the dichotomous M1/M2 distinction is insufficient to explain macrophage polarization in the TME.Citation21,Citation22 Recently, with the development of single-cell technology and the emergence of multi-omics, researchers could have a further glimpse into TAM phenotypic diversity and the dynamics of TAM polarization ecosystems.Citation22,Citation23 Increasing evidence has confirmed that TAMs are composed not only of a homogeneous population, but also a mixed population of macrophages in malignant solid tumor tissues, including in hepatocellular carcinoma (HCC),Citation24 breast cancer,Citation25 human renal cell carcinoma,Citation22 and gastric cancer (GC).Citation26 In these cases, apart from M1 and M2 properties, other macrophage clusters have been explored, especially those in the subset of PD-L1 positive TAMs and CD163+ TAMs, which can significantly promote tumor progression. Thus, the coexistence of M1 and M2 signatures indicates that TAMs exhibit more complexity than that simply described by the classical M1 and M2 models.
The mechanisms related to macrophage polarization and communication between TAMs and tumor cells in the TME is rather intricate. Previous studies have demonstrated the dependent role of the TME in TAMs phenotype acquisition, which depends on sophisticated intercellular communication via direct cell-to-cell contact or classical paracrine signaling loops involving cytokines or growth factors.Citation27–29 Emerging reports suggests that exosomes are important as one of essential regulators of macrophage polarization in the TME.Citation30–33
3. Exosomes
Exosomes are extracellular vesicles (EVs) with diameters ranging between 30 and 150 nm, have an endosomal origin, and are present in the blood, urine, and other cerebrospinal fluid.Citation34 Depending on the cell of origin, exosomes contain multiple cellular components as cargos, including DNA (mitochondrial and genomic), lipids (membranes), proteins, transposable elements, and RNAs (coding and non-coding).Citation35 These cargos are elaborately sorted and packaged into endosomes. When endosomes mature, they transform into multivesicular bodies (MVBs) and transported to the plasma membrane, and released as exosomes. Notably, several studies have identified that sorting mechanisms of MVBs contribute to the selective enrichment of exosomes with specific proteins and RNA molecules. These mechanisms suggest that molecular sorting into exosomes is a regulated process rather than a pathological resemblance.Citation36,Citation37 Posttranslational modifications have been involved in protein sorting into exosomes. For example, ubiquitination is responsible for sorting the major histocompatibility complex II (MHC-II)Citation38 and the oncogenic protein epidermal growth factor receptor (EGFR).Citation39 In addition, RNA-induced silencing complex (RISC) proteins, including AGO2, GW182;Citation40 and RNA binding proteins (RBPs), which contain the GAGG sequence motif,Citation41 have been confirmed to be necessary for the sorting of particular RNAs into exosomes. Exosomes are internalized by recipient cells through receptor-mediated endocytosis, pinocytosis, phagocytosis, or fusion with the cell membrane, resulting in direct release of their cargo into the cytoplasm. Transmission of molecular signals by exosomes has been shown to change the function and phenotypes of recipient cells. The targeted accumulation of specific cellular components in exosomes suggests that they play essential roles in regulating intercellular communication.Citation42,Citation43
Exosomes participate in tumor pathogenesis and progression by modulating the TME. Similarly, exosome-mediated intercellular communication also exists between tumor cells and TAMs, which can regulate the biological characteristics of tumor cells and influence the polarization of the TAMs, thus promoting or impeding tumor progression.Citation44,Citation45 The functions of exosomes have primarily been characterized by their origin and content.Citation46,Citation47 Furthermore, other studies have shown that exosome-induced cellular communication is specialized in long-distance interaction,Citation48,Citation49 facilitating the transfer of proteinsCitation50 and functional mRNA and microRNAs (miRNAs) for subsequent protein expression in target cells.Citation51 This communication mechanism is a highly efficient, robust, and economical way of exchanging information among cells. Therefore, an in-depth understanding of the molecular mechanisms by which exosomes facilitate communication between TAMs and tumor cells has the potential to contribute to the development of new anti-tumor therapies that reverse tumor suppressor immune response.
4. Tumor-derived exosomes modulate macrophage polarization
Tumor-derived exosomes also play crucial roles in immune modulation. Several studies have shown that they can remodel the tumor immune microenvironment (TIME), favoring a tumor-promoting status that supports tumorigenesis, invasion, angiogenesis, premetastatic niche formation, and metastatic dissemination.Citation52–54 Based on various molecular constituents, exosomes can switch macrophages either to the M1 or M2 phenotype.Citation55
4.1 miRNAs
miRNAs are small, non-coding RNAs that are usually 20–25 nucleotides in length. They bind to particular mRNA sequences and inhibit target-gene translation.Citation56 One of the most distinctive hallmarks of cancer-derived exosomes is a cargo consisting of multiple miRNAs, with mature miRNAs accounting for 41.7% of all RNAs.Citation57 Multiple miRNAs have been suggested to be critical bioactive components of exosomes. Exosomal microRNAs are able to regulate TAM function by affecting macrophage phenotype, cytokine secretion, T cell-related immune exhaustion and hypoxic microenvironment.
Increasing evidence has revealed that exosomal miRNAs exert important physiological functions in TAMs infiltration and polarization by regulating target molecules. Zhao et al. demonstrated elevated expression of miR-934 in areas with abundant CD163+ TAM infiltration in colorectal cancer (CRC) liver metastasis tissue; moreover, exosomal miR-934 induced M2 macrophage polarization via downregulation of phosphatase and tensin homolog deleted on chromosome ten (PTEN) expression and activation of the PI3K/AKT signaling pathway.Citation58 Many essential transcription factors, such as PPARs, STAT3, and STAT6 are involved in macrophage polarization in the TME. For example, Ying et al.Citation59 showed that epithelial ovarian cancer (EOC)-derived exosomal miR-222-3p induced a TAM-like macrophage phenotype and polarization into the M2 phenotype via the SOCS3/STAT3 pathway.
Recent studies have shown that abnormal chemokines secreted by tumor cells recruit macrophages to infiltrate tumors and affect macrophage phenotype.Citation60,Citation61 For example, activation of the chemokine receptor CXCR4/CXCL12 signaling has been implicated in TAM recruitment by melanomas.Citation62 Moreover, upon CXCL12/CXCR4 axis activation, CRC cells transferred several miRNAs, including miR-25-3p, miR-130b-3p, and miR-425-5p, to TAMs via exosomes. These exosomal miRNAs elicited the M2-polarized macrophage phenotype by suppressing the common target gene PTEN and activating the PI3K/AKT pathway.Citation63
Exosomes affect macrophage polarization and influence the interactions between them with other immune cells, especially T cells that participate in TIME regulation. For example, endoplasmic reticulum (ER) stress induces HCC cells to release exosomal miR-23a-3p, which upregulates PD-L1 expression in macrophages by activating the STAT3 signaling pathway, attenuating the anti-HCC immune response.Citation64 Exosomal miR-21 and miR-29a from cancer cells trigger human TLR8 – and murine TLR7-mediated nuclear factor-κB (NF-κB) activation in macrophages to produce IL-6 and TNF-α, which promote melanoma lung metastasis and lung cancer progression.Citation65
Besides immunosuppression, another crucial characteristic of TME is hypoxia. In the TME, hypoxic tumor cells extensively communicate with immune cells to establish conditions favorable for cancer cell immune escape. Several studies have demonstrated that hypoxia promotes exosome secretion, leading to an aggressive phenotype of cancer cells and angiogenesis.Citation66–68 Numerous studies have demonstrated that exosomes from hypoxia-induced tumors contain various non-coding RNAs, especially miRNAs involved in tumor progression. These exosomes also promote M2 macrophage polarization and microRNA-mediated macrophage metabolic shifts. Chen et al.Citation69 demonstrated that miR-21-3p, miR-125b-5p, and miR-181-5p were enriched with hypoxic exosomes derived from EOC. These miRNAs were endocytosed by the original macrophages and promoted TAM polarization by activating the SOCS4/5/STAT3/HIF-1α pathway.
Hypoxia creates an indispensable environment for the progression of epithelial-mesenchymal transition (EMT) by activating numerous transcriptional factors such as Snail and Twist.Citation70 In Snail-overexpressing head and neck squamous cell carcinoma (HNSCC) cells, Snail can enhance miR-21 transcription by binding directly, followed by sorting into exosomes. After being untaken by macrophages, exosomal miR-21 downregualted PDCD4 and IL-12A expression and mediated M2 polarization.Citation71 In addition to miRNAs, immunomodulatory proteins and chemokines, including CSF-1, CCL12, FTH, FTL, and TGF-β, have been found in exosomes derived from hypoxic tumor cells. These proteins, together with miRNAs, influence macrophage recruitment and promoted M2 polarization.Citation72
Summarizing our current understanding for above studies, exosomal miRNAs derived from tumor cells have critical influence on TAM polarization in the TME. These miRNAs can be used as tumor biomarkers to monitor tumor progression or can be utilized as important drug design targets or drug carriers, which will be discussed in a later section.
4.2 Long non-coding RNAs (lncRNAs)
LncRNAs provide essential transcriptomic information for transcripts longer than 200 nucleotides but have no/little protein-coding capacity.Citation73 LncRNAs exhibit low sequence identity (~50-60%) and low abundance; their median expression level is ~10-fold lower than that of mRNAs.Citation74 Circulating lncRNAs are unstable in the presence of high amounts of RNase in peripheral blood, while they are relatively stable when packaged into multiple types of EVs which prevent RNase digestion for lncRNAs.Citation75
Kogure et al. reported that lncRNA TUC339 is highly expressed in HCC-derived exosomes. It can transfer from one to another among HCC cells to promote tumor growth and metastasis.Citation76 Furthermore, the exosomal lncRNA TUC339 can transfer into neighborhood macrophages and induce macrophage polarization to the M2 phenotype, which dampens the anti-tumor immune response. Microarrays revealed that Toll-like receptor (TLR) signaling and FcR-mediated phagocytosis pathways were downregulated in macrophages by exosomal TUC339, with TUC339 knockdown increasing their phagocytic activity. TUC339 is also involved in cytokine and chemokine receptor signaling pathways, although the underlying mechanisms need further elucidation.Citation44 Similarly, exosomal lncRNA BCRT1 derived from breast cancer cells was internalized by macrophages to promote M2 polarization. It conferred increased migration and chemotaxis abilities to cancer cells, accelerating breast cancer progression.Citation77
Many lncRNAs, which are mainly located in the cytoplasm, serve as competing endogenous RNA (ceRNA) sponges to modulate the expression and biological functions of miRNAs; importantly, they are involved in tumor progression.Citation78–80 Cytoplasmic lncRNAs can be sorted into exosomes and absorbed by TAMs, but whether they regulate TAM polarization via sponge adsorption is yet to be further studied. Moreover, other non-coding RNAs, such as circular RNAs (circRNAs), have been found in cancer cell-derived exosomes.Citation81–83 These exosomal circRNAs can affect cellular communication in the TME. For example, HCC-derived exosomal circUHRF1 induces NK cell exhaustion by targeting miR-449 c-5p/TIM-3, causing resistance to anti-PD-L1 therapy.Citation84 Whether exosomal circRNAs affect macrophage polarization remains to be investigated.
4.3 Proteins
In addition to RNAs, plasma-membrane proteins are selectively sorted into the extracellular space through ectodomain shedding and microvesicle release. In contrast to vesicles released by plasma membrane budding,Citation85 exosomes are generated in multivesicular bodies through endocytic pathways, then are removed when these bodies fuse with the plasma membrane.Citation86 Peinado et al. have identified an oncoprotein transported via exosomes from melanoma cells, the receptor tyrosine kinase MET, which initiates long-distance inflammation to chemotactically attract circulating cancer cells.Citation87 Oncoproteins packaged into exosomes can maintain their activity after exosome uptake by recipient cells.Citation88,Citation89 Chen et al.Citation90 developed a SILAC-based mass spectrometry (MS) strategy to characterize the proteome functionally transported from CRC cells to bone marrow-derived macrophages (BMMs) via exosomes. Results showed that among the 1092 differentially expressed proteins, cytoskeleton-centric proteins were the most abundant functional class. Cytoskeletal rearrangement is a primary phenotype of monocyte/macrophage activation and maturation.Citation91,Citation92 Exosomal vimentin enhanced F-actin cytoskeleton rearrangement in macrophages, as indicated by the shape index and polarization. Similarly, Gabrusiewicz et al. reported that exosomal STAT3 derived from glioblastoma stem cells caused reorganization of the actin cytoskeleton and induced monocytes to shift toward the immune-suppressive M2 phenotype.Citation93 Exosomes derived from metastatic osteosarcoma cells could induce M2 polarization, while decreasing phagocytosis, efferocytosis, and macrophage-mediated cytotoxicity. These effects are modulated by the overexpression of immunosuppressive TGF-β2.Citation94
Emerging evidence indicates that tumor-derived exosomal proteins alter the phenotypes of monocyte-derived macrophages. Liang et al.Citation95 found that ribonuclease P RNA component H1 (RPPH1), binds to β-III tubulin (TUBB3) to prevent its ubiquitination and, then induces EMT to promote metastasis. Furthermore, RPPH1 was transferred by exosomes to macrophages to mediate macrophage M2 polarization. After treatment with exosomes derived from CRC cells, monocyte-derived macrophages (MDMs) exhibited a CD206 high/HLA-DRlow phenotype, with a stretched and elongated M2 cellular morphology. Similarly, Ham et al.Citation96 revealed that the IL-6 receptor gp130 was present in breast cancer cell-derived exosomes, where it stimulated IL6/STAT3 signaling in bone marrow-derived macrophages (BMDMs). After activation, phosphorylated STAT3 translocated to the nucleus and induced the transcription of target genes, including macrophage polarization-associated genes, IL-6, IL-10, CXCR4, and CCL2. This activation subsequently promoted BMDM survival and induced the switching of BMDMs toward a cancer-promoting phenotype. Comparing with studies of RNA in exosomes, the function and mechanism of exosomal proteins in macrophage and TME need more further research to elucidate.
4.4 Lipids
Some fusogenic membrane lipids have been identified in exosomes and play essential roles in fusing exosomes with the plasma membrane. Arachidonic acid (AA) is the primary polyunsaturated fatty acid precursor for the production of pro-inflammatory lipid mediators. It destabilizes membranes by converting closely associated lipid bilayers into a hexagonal structure to promote annexin2-mediated membrane fusion.Citation97–99 Exosomes derived from pancreatic cancer (PC) cells AsPC-1 contain higher AA levels than the recipient cells, facilitating their fusion with THP-1-derived macrophages. AA elevated the secretion of PGE2, increased the levels of the M2 phenotype (CD14hiCD163hiCD206hi), and enhanced the secretion of protumoral bioactive molecules, including VEGF, MCP-1, IL-6, IL-1β, MMP-9, and TNF-α, which contribute to tumor progression.Citation100
A recent study demonstrated that metabolic reprogramming of macrophages plays a predominant role in regulating their phenotype and plasticity.Citation101 LPS (+IFN)-activated inflammatory macrophages convert arginine into nitric oxide (NO) through inducible NO synthase (iNOS) activity.Citation17,Citation102 Macrophages also increased the accumulation of succinate, thus stabilizing HIF-1α and induction of IL-1β.Citation103 Conversely, arginine is metabolized by arginase-1 in IL-4-induced alternatively activated macrophages (M2).Citation104,Citation105 However, it is unclear whether tumor cell-derived exosomes are involved in metabolic reprogramming of TAMs. Thus, this question needs more attention to settle.
The above studies focused on exosomal induction of TAM polarization toward the acquisition of the M2-phenotype. However, researchers should not ignore the possibility of exosome-mediated induction of macrophage polarization into the M1-phenotype. Xiao et al. confirmed this hypothesis and found that exosomal THBS1 derived from OSCC significantly upregulated the expression of M1-related genes, including TNFα, IL-1β, and IL-6 in macrophages. Furthermore, the p38, Akt, and SAPK/JNK signaling pathways activated by exosomes were found to be involved in M1 phenotype polarization.Citation106 Similarly, miR-125b-5p, which increases in primary melanoma, is delivered to macrophages by exosomes and can reinforce the activation of M1 macrophages through the induction of CCL1, CCL2, and IL-1β.Citation107 These findings further demonstrate the complexity of exosomal function in regulating macrophage polarization; further studies are needed to elucidate the detailed mechanisms. In summary, these studies describe the critical effectors of macrophage polarization in TME induced by tumor-derived exosomes, suggesting that most signal molecules are loaded toward immune tolerance, which benefits tumor progressionCitation77,Citation108 (). A summary of the molecules involved in macrophage polarization is provided in .
Table 1. Tumor cells – derived exosomes involve in macrophages polarization
Figure 1. Tumor-derived exosomes modulate macrophage polarization
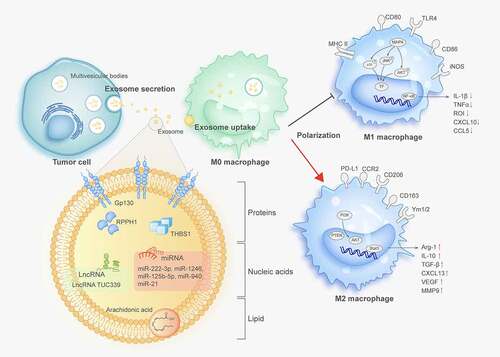
Notably, existing studies have focused on the effects of exosomes on macrophages polarization into M1 or M2-type states. However, as mentioned above, this dichotomous classification is far from sufficient to account for the complexity of TAMs, because polarization is a dynamic and reversible process in the TME; thus, further research is needed to understand how exosomes determine macrophage polarization to various TAM phenotypes. It is necessary to establish model systems to study different subtypes of macrophages and to search for more accurate molecular markers to characterize TAMs. It is also very crucial to point out that, in recent studies, endocytosis has been considered as the primary mechanism for exosome to deliver its contents to TAMs. Furthermore, the interaction between exosome surface molecules and the surface molecules of target cells that do not take up exosomes is still capable of signals of target cells.Citation109,Citation110 Whether this process plays a role in the polarization of TAMs, and whether it is different from the effects of endocytosis, is worth further investigation.
5. Macrophage-derived exosomes are involved in tumor progression
Most TAMs lack the ability to phagocytize tumor cells while facilitate tumor cell escape from killing to enable their metastases to distant tissues. Reciprocal exosome exchanges between TAMs and cancer cells also modulate cancer progression.
5.1 Regulating cancer proliferation, migrationand invasion
Increasing evidence has revealed the essential roles of TAM-derived exosomes in fostering the pace of cancer initiation and progression. Song et al. reported that macrophages transfer miRNAs via exosomes to breast cancer cells to promote migration and invasion. miR-223 is a macrophage-dominant miRNA that is enriched in exosomes derived from IL-4-activated macrophages. After incubation with breast cancer cells, exosomal miR-223 was internalized more efficiently and activated the miR-223/Mef2c/β-catenin pathway to promote the invasiveness of breast cancer cells.Citation111 Similarly, miR-21-5p and miR-155-5p derived from macrophages are critical for colon cancer cell migration and invasion.Citation112 In contrast, other exosomal miRNAs from TAMs inhibit tumor progression. For example, exosomal miR-7 was found to be transferred from TAMs to EOC cells, in which it suppressed metastasis by reducing the activity of the EGFR/AKT/ERK1/2 pathway.Citation113 Exosomal miR-142-3p and miR-223 from macrophages functionally inhibited the proliferation of HCC cells by decreasing the expression levels of stathmin-1 and insulin-like growth factor-1 receptor (ILGF1R).Citation114
LncRNAs in macrophage-derived exosomes also participate in tumor development. LncRNA SBF2-AS1 is enriched in the exosomes from M2 macrophages. It was taken up by pancreatic cancer cells PANC-1 and promoted proliferation, invasion, and migration. Mechanistically, exosomal lncRNA SBF2-AS1 increased miR-122-5p expression and restrained X-linked inhibitor of apoptosis protein (XIAP) from PC progression, suggesting that the lncRNA SBF2-AS1/miR-122-5p/XIAP axis is involved in communication between macrophages and cancer cells.Citation115
Arginase-1, an enzyme critical for converting arginine to ornithine and urea, is mainly expressed on the macrophage surface. Juliana et al. observed that arginase-1 levels were increased in TAM-derived exosomes, which is critical for promoting glioblastoma cell proliferation.Citation116 Tumor cells leave the primary site, enter the blood circulation, and attach to vascular endothelial cells, creating a critical link in the metastatic process.
The migratory potential of invasive cells is dependent on matrix metalloproteinases (MMPs) anchored on the cell surface via the integrin family. Wu et al.Citation117 found that CD11b/CD18, an integrin derived from M2 macrophage exosomes, expedited HCC cell potential and metastasis by activating MMP-9, but did not affect cancer cell proliferation. Apolipoprotein E (ApoE), a major protein component of very-low-density and high-density lipoproteins, induces macrophages to convert the pro-inflammatory M1 phenotype to the anti-inflammatory M2 phenotype due to its antiatherogenic activity.Citation118 Zheng et al. revealed that ApoE, a particular protein in M2 macrophage-derived exosomes, mediates the intercellular transfer of the ApoE-activating PI3K/AKT pathway in recipient GC cells and enhances metastatic potential.Citation119 These results highlight the importance of macrophage exosome-derived proteins in the crosstalk between TAMs and tumor cells during tumor progression.
5.2 Promoting angiogenesis
Tumor growth and metastasis largely depend on angiogenesis, the process by which new blood vessels grow from existing vessels surrounded by a growing tumor mass. Some studies have shown that the number of TAMs infiltrating tissue is closely related to the vascular density in tumors.Citation120,Citation121 Large aggregates of TAMs were found in anoxic areas, especially in necrotic tissues.Citation122 TAMs are regarded as significant producers of proangiogenic factors in malignant tumors because they release IL-1β, VEGF, TNF-α, and other cytokines.Citation123,Citation124 Studies have recently shown that exosomes play an important role in cancer invasiveness by harboring various cargos to accelerate angiogenesis. El-Arabey et al. found that the transcription factor GATA3 was released in abundance from TAM-derived exosomes, in which it played a critical role in the interactions between the TAMs and mutant TP53-expressing high-grade serous ovarian carcinoma (HGSOC) to promote angiogenesis and EMT through epigenetic regulation.Citation125 Similarly, M2 macrophage-derived exosomal miR-501-3p enhanced the tube-formation ability of pancreatic ductal adenocarcinoma (PDAC) cells by increasing their expression of angiogenesis-related proteins VEGF-A and VEGFR-2, suggesting that exosomal miR-501-3p is essential for the angiogenesis in PDAC.Citation126
5.3 Generating drug resistance
Drug therapy is the primary therapeutic strategy for controlling tumor progression. However, drug resistance is a significant obstacle in devising and implementing such therapies. Recent studies have indicated that both tumor and stromal cells can secrete exosomes containing drug resistance-related factors and transfer them to the TME, where they interact with each other, thus increasing drug resistance.Citation84,Citation127–129
Cisplatin-based chemotherapy is mostly used to treat advanced GC; however, drug resistance still occurs frequently. Zheng et al.Citation130 showed that exosomes derived from M2 macrophages are involved in mediating cisplatin resistance. Using miRNA profiles, researchers identified significantly higher levels of miR-21 in exosomes and cell lysates of polarized M2 macrophage. Exosomal miR-21 can be directly transferred from macrophages to GC cells, suppressing apoptosis, and enhancing the activation of the PI3K/AKT signaling pathway by downregulating PTEN. Similarly, the activated PTEN/PI3K/AKT pathway has also been associated with drug resistance in EOC cells induced by TAM-derived exosomes containing miR-223.Citation45
Gemcitabine is a cytidine analog that inhibits cell division by arresting DNA replication. PDAC is known for its development of gemcitabine resistance within weeks of therapy initiation. Macrophages transmit molecular signals to cancer cells by shuttling exosomes that are selectively internalized by PDAC cells. The transfer of exosomal miR-365 among macrophages and PDAC cells inhibits the effect of gemcitabine by upregulating pyrimidine metabolism and increasing triphosphate-nucleotide (NTP) levels in cancer cells.Citation131 These studies suggest that macrophage-derived exosomes mediate drug resistance primarily through the horizontal transfer of molecules that confer drug resistance to otherwise sensitive cells.
5.4 Promoting immune escape of tumor cells
The responses of T lymphocytes are critical for anti-tumor immunity. Several studies have demonstrated that TAM-derived exosomes can affect the immune response of different T cell subtypes in the TME, and therefore promote tumor cell escape from immune recognition and eradication. CD8+ T cells are cytotoxic T cells, which are also effector cells that play vital roles in anti-tumor effects. TAMs suppress the CD8+ T cell response to cancer by secreting immunosuppressive factors, including CCL5, CCL17, and CCL22.Citation132 In addition to these chemokines, Yang et al.Citation133 found that M2-BMDM-derived exosomes (BMDM-Exos) shuttle miR-21 to promote migration, proliferation, and invasion, and inhibit the apoptosis of glioma cells by reducing the expression of paternally expressed gene 3 (PEG3). More importantly, this work provided evidence that BMDM-Exos can reduce the percentage of CD8+ T cells and inhibit the extent of cell cytotoxicity by accelerating the immune escape of gliomas and enhancing their progression. Tregs and T helper 17 (Th17) cells are two subsets of CD4+ T cells that play opposing immune regulatory roles. Treg/Th17 cell imbalance has been observed in several tumors and is associated with worse patient outcomes. Zhou et al.Citation134 showed that TAM-derived exosomes transferred miR-29a-3p and miR-21-5p to synergistically induce the Treg/Th17 cell imbalance by targeting STAT3 in CD4+ T cells, driving EOC progression and metastasis.
5.5 Reprogramming tumor metabolism
Metabolic reprogramming is a hallmark of malignant tumors.Citation135 Initially, Warburg found that the rate of glycolysis in cancer cells was much higher than oxidative phosphorylation (OXPHOS), even under sufficient oxygen known as the Warburg effect.Citation136 Since then, a series of studies have demonstrated that, except for glucose, abnormal metabolites involved in glucose, amino acid, and lipid metabolism pathways also induce tumor-related metabolic reprogramming, giving rise to the field of immunometabolism research.Citation137–139 These molecular metabolites are transferred into the TME via exosomal pathways and influence the metabolism of recipient cells to favor cancer progression. For example, Wang et al. demonstrated that the metabolic enzymes ALDOA and ALDH3A1, containing in exosomes from irradiated lung cancer cells, enhanced the migration and invasiveness of non-irradiated lung cancer cells by accelerating glycolysis.Citation140
A recent study revealed that HIF-1α-stabilizing long noncoding RNA (HISLA), an EV-packaged lncRNA, enhanced aerobic glycolysis and apoptotic resistance of breast cancer cells via EV transmission from TAMs to tumor cells. Mechanistically, HISLA interacted with PHD2 to inhibit the hydroxylation and degradation of HIF-1α. Moreover, lactate released from tumor cells upregulated HISLA in macrophages, constituting a feed-forward loop between TAMs and tumor cells.Citation141 These results suggest that exosome transfer is a useful method for cellular communication among multiple cell types in the TME. In addition to glycolysis, tumor metabolic reprogramming also includes lipid, amino acid, and tricarboxylic acid cycle (TCA) metabolism. Exosomes might also take part in these metabolic processes.Citation142 For example, cancer-associated fibroblasts (CAFs) secrete exosomal miR-522 to inhibit ferroptosis in GC cells by targeting arachidonate lipoxygenase 15 (ALOX15) and blocking lipid-ROS accumulation to support tumor progression and drug resistance.Citation129 However, whether TAM-derived exosomes are involved in these processes is unknown and requires in-depth study.
As discussed in this review, the latest evidence confirms that TAM-derived exosomes govern the cancer-promoting immune response in the TME. Therefore, we speculate that targeting the regulation of exosome-related mechanisms may be an attractive strategy to overcome chemical resistance by the immune transfer of miRNA to primary tumors via macrophages (). The molecules identified in TAM-derived exosomes are summarized in .
Table 2. Exosomes derived from TAMs modulate tumor progression
Figure 2. TAM-derived exosomes modulate tumor progression
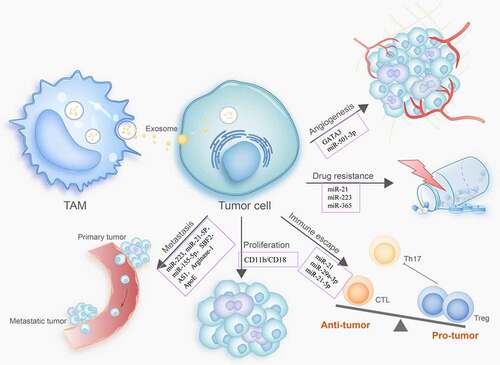
6.Exosomal function in targeted TAM-related immunotherapy
As the enrichment of TAMs is closely associated with poor prognosis for patients,Citation143,Citation144 TAMs are promising candidate targets for immuno-therapeutics. As described above, one of the critical characteristics of macrophages is plasticity, which enables them to acquire different phenotypes depending on the TME. The M1 phenotype imbues macrophages with the ability to eliminate and destroy tumor cells. Therefore, reprogramming TAMs to an anti-tumor phenotype is a very promising therapeutic strategy. A new avenue for developing interventions aims to potentiate the switch of M2 macrophages into M1 macrophages and block their immunosuppressive effects.Citation145,Citation146 Traditional methods usually use nanoparticles and liposomes to deliver targeted therapy.Citation147 However, the benefits of such methods are limited due to poor bioavailability, histocompatibility, and tumor selectivity.Citation120,Citation148,Citation149
Fortunately, exosomes can be considered a competent vehicle for the delivery of therapeutics, as they have several unique advantages. For example, exosomes have a unique lipid bilayer structure, which protects their contents from being degraded by ribonuclease and other enzymes, thus providing exosomal contents the biological stability. Moreover, comparing with traditional liposome carriers, exosomes have lower toxicity, as well as better tissue tolerance and biocompatibility. Thus, it is possible to design and modify engineered exosomes for immunological therapeutic strategies.Citation150 Taken together, the role of exosomes in macrophage polarization has prompted the design of therapeutic exosomes to trigger reprogramming from M2 to M1.
6.1 Modification of exosomes derived from tumor cells to promote TAM polarization
As explained above, miRNAs in exosomes regulate TAM polarization into the M2 phenotype; thus, alteration in their levels may mediate macrophage repolarization toward the M1 phenotype. Trivedi et al.Citation151 established a platform for manipulating exosomal content using dual-targeted hyaluronic acid-based nanoparticles. After transfecting wt-p53 and miR-125b into SK-LU-1 human lung adenocarcinoma cells, the exo-miRNA profile of the SK-LU-1 cells was reprogrammed. Moreover, TAMs were polarized to the M1 phenotype, suggesting that exosome modification may be a viable therapeutic strategy for affecting TME. Similarly, Su et al.Citation152 used hyaluronic acid-poly (ethylene imine)/hyaluronic acid-poly (ethylene glycol) (HA-PEI/HA-PEG) self-assembling nanoparticles to transfect miR-155 and miR-125b2 into PANC-1 cells. They found that the PANC-1-derived exosomes had high levels of miR-155 and miR-125b2, which triggered the repolarization of J774A.1 macrophages from the M2 to the M1 type by increasing IL-1β/Arg1 and iNOS/Arg1 ratios. These experiments demonstrate the feasibility of modulating tumor cell-derived exosome cargo to achieve anti-tumor effects through M1 macrophage reprogramming.
Construction of engineered exosomes has become a strategy in the design of anti-tumor drugs. Nie et al. constructed pH-responsive M1 exosome nano-bio-conjugates. Exosomes were conjugated with aCD47 and aSIRPα through acid-cleavable benzoic-imine bonds. These exosomes efficiently accumulated in tumor tissues and released the antibodies. Consequently, M1 exosomes reeducated pro-tumoral M2 macrophages driving their switch to M1 macrophages, and enhancing their phagocytic capacity.Citation153 This engineering strategy provides an exosomal platform that can be loaded with modified ligands that recognize and/or eradicate tumor cells.
Recently, researchers presented the results of a preclinical trial on engineered exosomes targeting macrophages,Citation154 in which they confirmed that the engExTM Platform program (exoASO), incorporating an antisense oligonucleotide (ASO), could selectively reprogram M2 macrophages into the M1 phenotype and promote targeted anti-tumor activity. Mechanistically, exoASO inhibited STAT6 and C/EBPβ expression and increased the level of TNF-α in macrophages. This study opens up new possibilities for engineered exosomes to regulate TAM polarization in tumor therapy.
In addition to the direct modification of exosomes, some anti-tumor drugs are involved in the regulation of macrophage polarization. Epigallocatechin gallate (EGCG), a polyphenol present in green tea, has been shown to inhibit tumorigenesis by inhibiting tumor cell proliferation, invasion, metastasis, and angiogenesis.Citation155 Studies have shown that in murine breast-cancer cells, EGCG upregulates miR-16, which was transferred to TAMs via exosomes, in which it inhibited TAM infiltration and M2 polarization.Citation156 This type of modification might provide a new strategy for exploring anti-tumor effects by manipulating TAMs and the TME. Recently, preclinical evidence showed that the STAT3 inhibitor pacritinib mediated a reduction in oncomiR-21-5p-enriched exosomes secreted by glioblastomas, targeting the tumor suppressor PDCD4. Treatment with a combination of pacritinib and temozolomide appeared to reduce tumorigenesis and retard M2 polarization.Citation157
6.2 Modified macrophage exosomes for the delivery of chemotherapeutic drugs
Classically activated M1 macrophages suppress tumor growth by releasing pro-inflammatory factors. M1 macrophage-derived exosomes propagate pro-inflammatory signals and establish a local immunostimulatory microenvironment. Wang et al. indicated that M1-exosomes (M1-Exos) act as carriers to deliver paclitaxel into tumors, where they enhance anti-tumor effects by increasing the activation of caspase-3 induced apoptosis and the production of pro-inflammatory cytokines.Citation158 Similarly, cisplatin-loaded M1 macrophage-secreted exosomes (DDP-M1-Exos) significantly inhibited cell proliferation and induced apoptosis by upregulating Bax and Caspase-3 in lung cancer. Furthermore, an in vivo study showed that M1-Exos encapsulated DDP and enhanced its anti-lung cancer activity.Citation159
6.3 Exosomes derived from M1 cells can be combined with other immunotherapies to treat cancer
It is well known that most tumor-associated antigens (TAAs) have weak immunogenicity, allowing them to quickly escape the immune surveillance. Thus, it is necessary to provide appropriate biological adjuvants to enhance antigen presentation and improve the efficacy of tumor vaccines. Vaccine adjuvants enhance the body’s adaptive immune responses to antigens and/or change the type of immune response when used simultaneously with or before antigen administration.Citation160 Many molecules and complex formulations have been used as vaccine adjuvants, including aluminum hydroxide,Citation161 lipopolysaccharide,Citation162 and mycobacteria.Citation163
Exosomal membrane lipids may possess adjuvant activity. Cheng et al. found that exosomes from M1 macrophages, but not M2 macrophages, display tropism toward lymph nodes, which are enriched with naïve macrophages and dendritic cells (DCs). Exosomes improved the activity of lipid calcium phosphate (LCP) nanoparticle-encapsulated Trp2 vaccine and enhanced the antigen-specific cytotoxic T cell response.Citation164 These data suggest that exosomes derived from M1 macrophages have potential as vaccine adjuvants.
The application of immune-checkpoint inhibitors is an important milestone in anti-tumor immunotherapy. The application of anti-PD-1 and anti-PD-L1 has been shown to improve the recognition and killing ability of T cells that recognize and eradicate tumor cells.Citation165,Citation166 However, their curative effect is limited in some solid tumors, where many TAMs infiltrate the TME to induce T cell exhaustion, decreasing immunotherapeutic efficacy.Citation167 Therefore, a synergistic immunotherapy scheme based on a combination of targeting TAMs and T cells may enhance anti-tumor therapies. Yeon et al.Citation168 demonstrated that exosome-mimetic nanovesicles derived from M1 macrophages (M1NVs) polarized M2 macrophages to acquire the M1 phenotype by changing miRNA and mRNA expression profiles. The repolarization of M1 macrophages resulted in the secretion of pro-inflammatory cytokines, which potentiated the anti-tumor efficacy of PD-L1. Injection of M1NVs and PD-L1 into tumor-bearing mice induced the repolarization of M2 TAMs into M1 macrophages, leading to a significant inhibitory effect on tumor growth.
These data suggest that exosomes derived from M1 macrophages can be used as immune regulators in the TME to potentiate checkpoint inhibitor therapies for cancer ().
Figure 3. Overview of exosomes in the communication between tumor cells and TAMs in the tumor microenvironment (TME)
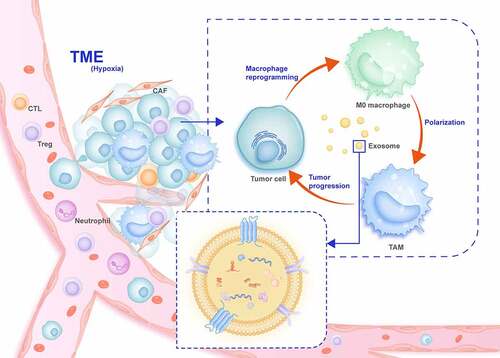
7. Perspective
Multiple studies have inexorably demonstrated that exosomes reflect their cellular origin and disease stage through the bioactive cargos they transport, making them useful as potential biomarkers for diagnosing and predicting clinical prognosis. By adjusting the body’s immune defense, tumor immunotherapy can transform immune cells or use multiple types of immuno-active substances to balance the immune system and tumors. As crucial members of the TME, macrophages are attractive targets for immunotherapy drug development because of their characteristics. Using molecularly drugs targeted to treat tumors has led to breakthroughs through in-depth research into cancer molecular biology.
Additional in-depth studies of the unique biological activities of exosomes are still necessary. The heterogeneity of macrophages has been described for a variety of solid tumors; however, it is not clear whether, in addition to their effects on M1 and M2 macrophages, exosomes are involved in the phenotypic polarization of other macrophages or whether particular macrophage subsets produce unique exosomes that regulate the TME. Exosomes as biomarkers can be used to explore new TAM cell subtypes, as well as phenotypes and key regulatory molecules related to tumors. Moreover, they can reverse TAM-mediated immune escape, regulate the TME, and enhance tumor cell recognition and killing. This may be an essential treatment strategy in addition to immune checkpoint inhibitor treatment. However, it is worth noting that even though engineered exosomes perform well in vitro experiments, other factors must be considered for the complexity of TME in cancer patients to ensure that sufficient exosomes can reliably access target cells in vivo. Recent research ongoing in vivo, in vitro, and preclinical studies, as well as clinical trials on TAMs, are expected to yield encouraging results.
Declaration of competing interest
All authors have declared that no conflict of interest exists.
Acknowledgments
This work was supported by the National Natural Science Foundation of China Grant No. 81772550 (LM. Zhao) and Outstanding Youth Funding of Hebei Province Grant No. H2019206697 (LM. Zhao).
Additional information
Funding
References
- Bhowmick NA, Moses HL. Tumor-stroma interactions. Curr Opin Genet Dev. 2005;15(1):97–16. doi:10.1016/j.gde.2004.12.003.
- Quail DF, Joyce JA. Microenvironmental regulation of tumor progression and metastasis. Nat Med. 2013;19(11):1423–1437. doi:10.1038/nm.3394.
- Naito Y, Yoshioka Y, Yamamoto Y, Ochiya T. How cancer cells dictate their microenvironment: present roles of extracellular vesicles. Cell Mol Life Sci. 2017;74(4):697–713. doi:10.1007/s00018-016-2346-3.
- Lee K, Hwang H, Nam KT. Immune response and the tumor microenvironment: how they communicate to regulate gastric cancer. Gut Liver. 2014;8(2):131–139. doi:10.5009/gnl.2014.8.2.131.
- Sarode P, Schaefer MB, Grimminger F, Seeger W, Savai R. Macrophage and tumor cell cross-talk is fundamental for lung tumor progression: we need to talk. Front Oncol. 2020;10:324. doi:10.3389/fonc.2020.00324.
- Huang YK, Wang M, Sun Y, Di Costanzo N, Mitchell C, Achuthan A, Hamilton JA, Busuttil RA, Boussioutas A. Macrophage spatial heterogeneity in gastric cancer defined by multiplex immunohistochemistry. Nat Commun. 2019;10(1):3928. doi:10.1038/s41467-019-11788-4.
- Franklin RA, Liao W, Sarkar A, Kim MV, Bivona MR, Liu K, Pamer EG, Li MO. The cellular and molecular origin of tumor-associated macrophages. Science. 2014;344(6186):921–925. doi:10.1126/science.1252510.
- Sumitomo R, Hirai T, Fujita M, Murakami H, Otake Y, Huang CL. PD-L1 expression on tumor-infiltrating immune cells is highly associated with M2 TAM and aggressive malignant potential in patients with resected non-small cell lung cancer. Lung Cancer. 2019;136:136–144. doi:10.1016/j.lungcan.2019.08.023.
- Lian G, Chen S, Ouyang M, Li F, Chen L, Yang J. Colon cancer cell secretes EGF to promote M2 polarization of TAM through EGFR/PI3K/AKT/mTOR pathway. Technol Cancer Res Treat. 2019;18:1533033819849068. doi:10.1177/1533033819849068.
- Qian BZ, Pollard JW. Macrophage diversity enhances tumor progression and metastasis. Cell. 2010;141(1):39–51. doi:10.1016/j.cell.2010.03.014.
- Lin X, Wang S, Sun M, Zhang C, Wei C, Yang C, Dou R, Liu Q, Xiong B. miR-195-5p/NOTCH2-mediated EMT modulates IL-4 secretion in colorectal cancer to affect M2-like TAM polarization. J Hematol Oncol. 2019;12(1):20. doi:10.1186/s13045-019-0708-7.
- Derks S, de Klerk LK, Xu X, Fleitas T, Liu KX, Liu Y, Dietlein F, Margolis C, Chiaravalli AM, Da Silva AC, et al. Characterizing diversity in the tumor-immune microenvironment of distinct subclasses of gastroesophageal adenocarcinomas. Ann Oncol. 2020;31(8):1011–1020. doi:10.1016/j.annonc.2020.04.011.
- Fujimura T, Kambayashi Y, Fujisawa Y, Hidaka T, Aiba S. Tumor-associated macrophages: therapeutic targets for skin cancer. Front Oncol. 2018;8:3. doi:10.3389/fonc.2018.00003.
- Martinez FO, Sica A, Mantovani A, Locati M. Macrophage activation and polarization. Front Biosci. 2008;13(13):453–461. doi:10.2741/2692.
- Yang M, McKay D, Pollard JW, Lewis CE. Diverse functions of macrophages in different tumor microenvironments. Cancer Res. 2018;78(19):5492–5503. doi:10.1158/0008-5472.CAN-18-1367.
- Namgaladze D, Zukunft S, Schnutgen F, Kurrle N, Fleming I, Fuhrmann D, Brune B. Polarization of human macrophages by interleukin-4 does not require ATP-citrate lyase. Front Immunol. 2018;9:2858. doi:10.3389/fimmu.2018.02858.
- Murray PJ, Allen JE, Biswas SK, Fisher EA, Gilroy DW, Goerdt S, Gordon S, Hamilton JA, Ivashkiv LB, Lawrence T, et al. Macrophage activation and polarization: nomenclature and experimental guidelines. Immunity. 2014;41(1):14–20. doi:10.1016/j.immuni.2014.06.008.
- Ferrante CJ, Leibovich SJ. Regulation of macrophage polarization and wound healing. Adv Wound Care (New Rochelle). 2012;1(1):10–16. doi:10.1089/wound.2011.0307.
- Gambardella V, Castillo J, Tarazona N, Gimeno-Valiente F, Martinez-Ciarpaglini C, Cabeza-Segura M, Rosello S, Roda D, Huerta M, Cervantes A, et al. The role of tumor-associated macrophages in gastric cancer development and their potential as a therapeutic target. Cancer Treat Rev. 2020;86:102015. doi:10.1016/j.ctrv.2020.102015.
- Mehla K, Singh PK. Metabolic regulation of macrophage polarization in cancer. Trends in Cancer. 2019;5(12):822–834. doi:10.1016/j.trecan.2019.10.007.
- Sica A, Mantovani A. Macrophage plasticity and polarization: in vivo veritas. J Clin Invest. 2012;122(3):787–795. doi:10.1172/JCI59643.
- Nirschl TR, El Asmar M, Ludwig WW, Ganguly S, Gorin MA, Johnson MH, Pierorazio PM, Drake CG, Allaf ME, Zarif JC. Transcriptional profiling of tumor associated macrophages in human renal cell carcinoma reveals significant heterogeneity and opportunity for immunomodulation. Am J Clin Exp Urol. 2020;8:48–58.
- Azizi E, Carr AJ, Plitas G, Cornish AE, Konopacki C, Prabhakaran S, Nainys J, Wu K, Kiseliovas V, Setty M, et al. Single-cell map of diverse immune phenotypes in the breast tumor microenvironment. Cell. 2018;174(5):1293–1308 e1236. doi:10.1016/j.cell.2018.05.060.
- Zhang Q, He Y, Luo N, Patel SJ, Han Y, Gao R, Modak M, Carotta S, Haslinger C, Kind D, et al. Landscape and dynamics of single immune cells in hepatocellular carcinoma. Cell. 2019;179(4):829–845 e820. doi:10.1016/j.cell.2019.10.003.
- Wagner J, Rapsomaniki MA, Chevrier S, Anzeneder T, Langwieder C, Dykgers A, Rees M, Ramaswamy A, Muenst S, Soysal SD, et al. A single-cell atlas of the tumor and immune ecosystem of human breast cancer. Cell. 2019;177(5):1330–1345 e1318. doi:10.1016/j.cell.2019.03.005.
- Sathe A, Grimes SM, Lau BT, Chen J, Suarez C, Huang RJ, Poultsides G, Ji HP. Single-cell genomic characterization reveals the cellular reprogramming of the gastric tumor microenvironment. Clin Cancer Res. 2020;26(11):2640–2653. doi:10.1158/1078-0432.CCR-19-3231.
- Li D, Ji H, Niu X, Yin L, Wang Y, Gu Y, Wang J, Zhou X, Zhang H, Zhang Q. Tumor-associated macrophages secrete CC-chemokine ligand 2 and induce tamoxifen resistance by activating PI3K/Akt/mTOR in breast cancer. Cancer Sci. 2020;111(1):47–58. doi:10.1111/cas.14230.
- Lan Q, Lai W, Zeng Y, Liu L, Li S, Jin S, Zhang Y, Luo X, Xu H, Lin X, et al. CCL26 participates in the prl-3-induced promotion of colorectal cancer invasion by stimulating tumor-associated macrophage infiltration. Mol Cancer Ther. 2018;17(1):276–289. doi:10.1158/1535-7163.MCT-17-0507.
- Madeddu C, Gramignano G, Kotsonis P, Coghe F, Atzeni V, Scartozzi M, Maccio A. Microenvironmental M1 tumor-associated macrophage polarization influences cancer-related anemia in advanced ovarian cancer: key role of interleukin-6. Haematologica. 2018;103(9):e388–e391. doi:10.3324/haematol.2018.191551.
- Chang YT, Peng HY, Hu CM, Huang SC, Tien SC, Jeng YM. Pancreatic cancer-derived small extracellular vesical Ezrin regulates macrophage polarization and promotes metastasis. Am J Cancer Res. 2020;10:12–37.
- Ren W, Hou J, Yang C, Wang H, Wu S, Wu Y, Zhao X, Lu C. Extracellular vesicles secreted by hypoxia pre-challenged mesenchymal stem cells promote non-small cell lung cancer cell growth and mobility as well as macrophage M2 polarization via miR-21-5p delivery. J Exp Clin Cancer Res. 2019;38(1):62. doi:10.1186/s13046-019-1027-0.
- Andrade LNDS, Otake AH, Cardim SGB, da Silva FI, Ikoma Sakamoto MM, Furuya TK, Uno M, Pasini FS, Chammas R. Extracellular vesicles shedding promotes melanoma growth in response to chemotherapy. Sci Rep. 2019;9(1):14482. doi:10.1038/s41598-019-50848-z.
- Liu J, Wu F, Zhou H. Macrophage-derived exosomes in cancers: biogenesis, functions and therapeutic applications. Immunol Lett. 2020;227:102–108. doi:10.1016/j.imlet.2020.08.003.
- Kalluri R, LeBleu VS. The biology function and biomedical applications of exosomes. Science. 2020;367(6478):eaau6977. doi:10.1126/science.aau6977.
- Ruivo CF, Adem B, Silva M, Melo SA. The biology of cancer exosomes: insights and new perspectives. Cancer Res. 2017;77(23):6480–6488. doi:10.1158/0008-5472.CAN-17-0994.
- Gangoda L, Liem M, Ang CS, Keerthikumar S, Adda CG, Parker BS, Mathivanan S. Proteomic profiling of exosomes secreted by breast cancer cells with varying metastatic potential. Proteomics. 2017;17(23–24). doi:10.1002/pmic.201600370.
- Cha DJ, Franklin JL, Dou Y, Liu Q, Higginbotham JN, Demory Beckler M, Weaver AM, Vickers K, Prasad N, Levy S, et al. KRAS-dependent sorting of miRNA to exosomes. Elife. 2015;4:e07197. doi:10.7554/eLife.07197.
- Gauvreau ME, Cote MH, Bourgeois-Daigneault MC, Rivard LD, Xiu F, Brunet A, Shaw A, Steimle V, Thibodeau J. Sorting of MHC class II molecules into exosomes through a ubiquitin-independent pathway. Traffic. 2009;10(10):1518–1527. doi:10.1111/j.1600-0854.2009.00948.x.
- Trajkovic K, Hsu C, Chiantia S, Rajendran L, Wenzel D, Wieland F, Schwille P, Brugger B, Simons M. Ceramide triggers budding of exosome vesicles into multivesicular endosomes. Science. 2008;319(5867):1244–1247. doi:10.1126/science.1153124.
- Gibbings DJ, Ciaudo C, Erhardt M, Voinnet O. Multivesicular bodies associate with components of miRNA effector complexes and modulate miRNA activity. Nat Cell Biol. 2009;11(9):1143–1149. doi:10.1038/ncb1929.
- Villarroya-Beltri C, Gutierrez-Vazquez C, Sanchez-Cabo F, Perez-Hernandez D, Vazquez J, Martin-Cofreces N, Martinez-Herrera DJ, Pascual-Montano A, Mittelbrunn M, Sanchez-Madrid F. Sumoylated hnRNPA2B1 controls the sorting of miRNAs into exosomes through binding to specific motifs. Nat Commun. 2013;4(1):2980. doi:10.1038/ncomms3980.
- Sun Z, Shi K, Yang S, Liu J, Zhou Q, Wang G, Song J, Li Z, Zhang Z, Yuan W. Effect of exosomal miRNA on cancer biology and clinical applications. Mol Cancer. 2018;17(1):147. doi:10.1186/s12943-018-0897-7.
- Wan Z, Gao X, Dong Y, Zhao Y, Chen X, Yang G, Liu L. Exosome-mediated cell-cell communication in tumor progression. Am J Cancer Res. 2018;8:1661–1673.
- Li X, Lei Y, Wu M, Li N. Regulation of macrophage activation and polarization by hcc-derived exosomal lncRNA TUC339. Int J Mol Sci. 2018;19:9-19. doi:10.3390/ijms19102958.
- Zhu X, Shen H, Yin X, Yang M, Wei H, Chen Q, Feng F, Liu Y, Xu W, Li Y. Macrophages derived exosomes deliver miR-223 to epithelial ovarian cancer cells to elicit a chemoresistant phenotype. J Exp Clin Cancer Res. 2019;38(1):81. doi:10.1186/s13046-019-1095-1.
- Lobb RJ, Lima LG, Moller A. Exosomes: key mediators of metastasis and pre-metastatic niche formation. Semin Cell Dev Biol. 2017;67:3–10. doi:10.1016/j.semcdb.2017.01.004.
- Cheng J, Meng J, Zhu L, Peng Y. Exosomal noncoding RNAs in glioma: biological functions and potential clinical applications. Mol Cancer. 2020;19(1):66. doi:10.1186/s12943-020-01189-3.
- Thery C. Exosomes: secreted vesicles and intercellular communications. F1000 Biol Rep. 2011;3:15. doi:10.3410/B3-15.
- van der Pol E, Boing AN, Harrison P, Sturk A, Nieuwland R, Mattson MP. Classification, functions, and clinical relevance of extracellular vesicles. Pharmacol Rev. 2012;64(3):676–705. doi:10.1124/pr.112.005983.
- Johnstone RM. The jeanne manery-fisher memorial lecture 1991. maturation of reticulocytes: formation of exosomes as a mechanism for shedding membrane proteins. Biochem Cell Biol. 1992;70(3–4):179–190. doi:10.1139/o92-028.
- Zomer A, Vendrig T, Hopmans ES, van Eijndhoven M, Middeldorp JM, Pegtel DM. Exosomes: fit to deliver small RNA. Commun Integr Biol. 2010;3(5):447–450. doi:10.4161/cib.3.5.12339.
- Hessvik NP, Llorente A. Current knowledge on exosome biogenesis and release. Cell Mol Life Sci. 2018;75(2):193–208. doi:10.1007/s00018-017-2595-9.
- Yang H, Zhang H, Ge S, Ning T, Bai M, Li J, Li S, Sun W, Deng T, Zhang L, et al. Exosome-derived mir-130a activates angiogenesis in gastric cancer by targeting c-myb in vascular endothelial cells. Mol Ther. 2018;26(10):2466–2475. doi:10.1016/j.ymthe.2018.07.023.
- Jeppesen DK, Nawrocki A, Jensen SG, Thorsen K, Whitehead B, Howard KA, Dyrskjot L, Orntoft TF, Larsen MR, Ostenfeld MS. Quantitative proteomics of fractionated membrane and lumen exosome proteins from isogenic metastatic and nonmetastatic bladder cancer cells reveal differential expression of EMT factors. Proteomics. 2014;14(6):699–712. doi:10.1002/pmic.201300452.
- Baig MS, Roy A, Rajpoot S, Liu D, Savai R, Banerjee S, Kawada M, Faisal SM, Saluja R, Saqib U, et al. Tumor-derived exosomes in the regulation of macrophage polarization. Inflamm Res. 2020;69(5):435–451. doi:10.1007/s00011-020-01318-0.
- Mori MA, Ludwig RG, Garcia-Martin R, Brandao BB, Kahn CR. Extracellular miRNAs: from biomarkers to mediators of physiology and disease. Cell Metab. 2019;30:656–673. doi:10.1010/j.cmet.2019.07.011.
- Huang X, Yuan T, Liang M, Du M, Xia S, Dittmar R, Wang D, See W, Costello BA, Quevedo F, et al. Exosomal miR-1290 and miR-375 as prognostic markers in castration-resistant prostate cancer. Eur Urol. 2015;67(1):33–41. doi:10.1016/j.eururo.2014.07.035.
- Zhao S, Mi Y, Guan B, Zheng B, Wei P, Gu Y, Zhang Z, Cai S, Xu Y, Li X, et al. Tumor-derived exosomal miR-934 induces macrophage M2 polarization to promote liver metastasis of colorectal cancer. J Hematol Oncol. 2020;13(1):156. doi:10.1186/s13045-020-00991-2.
- Ying X, Wu Q, Wu X, Zhu Q, Wang X, Jiang L, Chen X, Wang X. Epithelial ovarian cancer-secreted exosomal miR-222-3p induces polarization of tumor-associated macrophages. Oncotarget. 2016;7(28):43076–43087. doi:10.18632/oncotarget.9246.
- Frankenberger C, Rabe D, Bainer R, Sankarasharma D, Chada K, Krausz T, Gilad Y, Becker L, Rosner MR. Metastasis suppressors regulate the tumor microenvironment by blocking recruitment of prometastatic tumor-associated macrophages. Cancer Res. 2015;75(19):4063–4073. doi:10.1158/0008-5472.CAN-14-3394.
- Yusen W, Xia W, Shengjun Y, Shaohui Z, Hongzhen Z. The expression and significance of tumor associated macrophages and CXCR4 in non-small cell lung cancer. J Buon. 2018;23:398–402.
- Mota JM, Leite CA, Souza LE, Melo PH, Nascimento DC, de-Deus-Wagatsuma VM, Temporal J, Figueiredo F, Noushmehr H, Alves-Filho JC, et al. Post-sepsis state induces tumor-associated macrophage accumulation through CXCR4/CXCL12 and favors tumor progression in mice. Cancer Immunol Res. 2016;4(4):312–322. doi:10.1158/2326-6066.CIR-15-0170.
- Wang D, Wang X, Si M, Yang J, Sun S, Wu H, Cui S, Qu X, Yu X. Exosome-encapsulated miRNAs contribute to CXCL12/CXCR4-induced liver metastasis of colorectal cancer by enhancing M2 polarization of macrophages. Cancer Lett. 2020;474:36–52. doi:10.1016/j.canlet.2020.01.005.
- Liu J, Fan L, Yu H, Zhang J, He Y, Feng D, Wang F, Li X, Liu Q, Li Y, et al. Endoplasmic reticulum stress causes liver cancer cells to release exosomal mir-23a-3p and up-regulate programmed death ligand 1 expression in macrophages. Hepatology. 2019;70(1):241–258. doi:10.1002/hep.30607.
- Fabbri M, Paone A, Calore F, Galli R, Gaudio E, Santhanam R, Lovat F, Fadda P, Mao C, Nuovo GJ, et al., MicroRNAs bind to Toll-like receptors to induce prometastatic inflammatory response, Proc Natl Acad Sci USA.,2012;109(31):E2110–2146. doi:10.1073/pnas.1209414109.
- Kumar A, Deep G. Hypoxia in tumor microenvironment regulates exosome biogenesis: molecular mechanisms and translational opportunities. Cancer Lett. 2020;479:23–30. doi:10.1016/j.canlet.2020.03.017.
- King HW, Michael MZ, Gleadle JM. Hypoxic enhancement of exosome release by breast cancer cells. BMC Cancer. 2012;12(1):421. doi:10.1186/1471-2407-12-421.
- Kucharzewska P, Christianson HC, Welch JE, Svensson KJ, Fredlund E, Ringner M, Morgelin M, Bourseau-Guilmain E, Bengzon J, Belting M, Exosomes reflect the hypoxic status of glioma cells and mediate hypoxia-dependent activation of vascular cells during tumor development, Proc Natl Acad Sci USA, 2013;110(18):7312–7317. doi:10.1073/pnas.1220998110.
- Chen X, Zhou J, Li X, Wang X, Lin Y, Wang X. Exosomes derived from hypoxic epithelial ovarian cancer cells deliver microRNAs to macrophages and elicit a tumor-promoted phenotype. Cancer Lett. 2018;435:80–91. doi:10.1016/j.canlet.2018.08.001.
- Zhang J, Jin HY, Wu Y, Zheng ZC, Guo S, Wang Y, Yang D, Meng XY, Xu X, Zhao Y. Hypoxia-induced lncRNA PCGEM1 promotes invasion and metastasis of gastric cancer through regulating SNAI1. Clin Transl Oncol. 2019;21(9):1142–1151. doi:10.1007/s12094-019-02035-9.
- Hsieh CH, Tai SK, Yang MH. Snail-overexpressing cancer cells promote m2-like polarization of tumor-associated macrophages by delivering mir-21-abundant exosomes. Neoplasia. 2018;20(8):775–788. doi:10.1016/j.neo.2018.06.004.
- Park JE, Dutta B, Tse SW, Gupta N, Tan CF, Low JK, Yeoh KW, Kon OL, Tam JP, Sze SK. Hypoxia-induced tumor exosomes promote M2-like macrophage polarization of infiltrating myeloid cells and microRNA-mediated metabolic shift. Oncogene. 2019;38(26):5158–5173. doi:10.1038/s41388-019-0782-x.
- Iyer MK, Niknafs YS, Malik R, Singhal U, Sahu A, Hosono Y, Barrette TR, Prensner JR, Evans JR, Zhao S, et al. The landscape of long noncoding RNAs in the human transcriptome. Nat Genet. 2015;47(3):199–208. doi:10.1038/ng.3192.
- Xiao J, Lv Y, Jin F, Liu Y, Ma Y, Xiong Y, Liu L, Zhang S, Sun Y, Tipoe GL, et al. LncRNA HANR promotes tumorigenesis and increase of chemoresistance in hepatocellular carcinoma. Cell Physiol Biochem. 2017;43(5):1926–1938. doi:10.1159/000484116.
- Han D, Fang Y, Guo Y, Hong W, Tu J, Wei W. The emerging role of long non-coding RNAs in tumor-associated macrophages. J Cancer. 2019;10(26):6738–6746. doi:10.7150/jca.35770.
- Kogure T, Yan IK, Lin WL, Patel T. Extracellular vesicle-mediated transfer of a novel long noncoding rna tuc339: a mechanism of intercellular signaling in human hepatocellular cancer. Genes Cancer. 2013;4(7–8):261–272. doi:10.1177/1947601913499020.
- Liang Y, Song X, Li Y, Chen B, Zhao W, Wang L, Zhang H, Liu Y, Han D, Zhang N, et al. LncRNA BCRT1 promotes breast cancer progression by targeting miR-1303/PTBP3 axis. Mol Cancer. 2020;19(1):85. doi:10.1186/s12943-020-01206-5.
- Zheng R, Du M, Wang X, Xu W, Liang J, Wang W, Lv Q, Qin C, Chu H, Wang M, et al. Exosome-transmitted long non-coding RNA PTENP1 suppresses bladder cancer progression. Mol Cancer. 2018;17(1):143. doi:10.1186/s12943-018-0880-3.
- Luan Y, Li X, Luan Y, Zhao R, Li Y, Liu L, Hao Y, Oleg Vladimir B, Jia L. Circulating lncRNA UCA1 promotes malignancy of colorectal cancer via the miR-143/MYO6 axis. Mol Ther Nucleic Acids. 2020;19:790–803. doi:10.1016/j.omtn.2019.12.009.
- Li Z, Qin X, Bian W, Li Y, Shan B, Yao Z, Li S. Exosomal lncRNA ZFAS1 regulates esophageal squamous cell carcinoma cell proliferation, invasion, migration and apoptosis via microRNA-124/STAT3 axis. J Exp Clin Cancer Res. 2019;38(1):477. doi:10.1186/s13046-019-1473-8.
- Xie M, Yu T, Jing X, Ma L, Fan Y, Yang F, Ma P, Jiang H, Wu X, Shu Y, et al. Exosomal circSHKBP1 promotes gastric cancer progression via regulating the miR-582-3p/HUR/VEGF axis and suppressing HSP90 degradation. Mol Cancer. 2020;19(1):112. doi:10.1186/s12943-020-01208-3.
- Ding C, Yi X, Wu X, Bu X, Wang D, Wu Z, Zhang G, Gu J, Kang D. Exosome-mediated transfer of circRNA CircNFIX enhances temozolomide resistance in glioma. Cancer Lett. 2020;479:1–12. doi:10.1016/j.canlet.2020.03.002.
- Han K, Wang FW, Cao CH, Ling H, Chen JW, Chen RX, Feng ZH, Luo J, Jin XH, Duan JL, et al. CircLONP2 enhances colorectal carcinoma invasion and metastasis through modulating the maturation and exosomal dissemination of microRNA-17. Mol Cancer. 2020;19(1):60. doi:10.1186/s12943-020-01184-8.
- Zhang PF, Gao C, Huang XY, Lu JC, Guo XJ, Shi GM, Cai JB, Ke AW. Cancer cell-derived exosomal circUHRF1 induces natural killer cell exhaustion and may cause resistance to anti-PD1 therapy in hepatocellular carcinoma. Mol Cancer. 2020;19(1):110. doi:10.1186/s12943-020-01222-5.
- van Niel G, D’Angelo G, Raposo G. Shedding light on the cell biology of extracellular vesicles. Nat Rev Mol Cell Biol. 2018;19(4):213–228. doi:10.1038/nrm.2017.125.
- Veerman RE, Gucluler Akpinar G, Eldh M, Gabrielsson S. Immune cell-derived extracellular vesicles – functions and therapeutic applications. Trends Mol Med. 2019;25(5):382–394. doi:10.1016/j.molmed.2019.02.003.
- Peinado H, Aleckovic M, Lavotshkin S, Matei I, Costa-Silva B, Moreno-Bueno G, Hergueta-Redondo M, Williams C, Garcia-Santos G, Ghajar C, et al. Melanoma exosomes educate bone marrow progenitor cells toward a pro-metastatic phenotype through MET. Nat Med. 2012;18(6):883–891. doi:10.1038/nm.2753.
- Song X, Ding Y, Liu G, Yang X, Zhao R, Zhang Y, Zhao X, Anderson GJ, Nie G. Cancer cell-derived exosomes induce mitogen-activated protein kinase-dependent monocyte survival by transport of functional receptor tyrosine kinases. J Biol Chem. 2016;291(16):8453–8464. doi:10.1074/jbc.M116.716316.
- Jella KK, Yu L, Yue Q, Friedman D, Duke BJ, Alli AA, Reddy H. Exosomal GAPDH from proximal tubule cells regulate enac activity. PLoS One. 2016;11(11):e0165763. doi:10.1371/journal.pone.0165763.
- Chen Z, Yang L, Cui Y, Zhou Y, Yin X, Guo J, Zhang G, Wang T, He QY. Cytoskeleton-centric protein transportation by exosomes transforms tumor-favorable macrophages. Oncotarget. 2016;7(41):67387–67402. doi:10.18632/oncotarget.11794.
- Wang T, Gong N, Liu J, Kadiu I, Kraft-Terry SD, Mosley RL, Volsky DJ, Ciborowski P, Gendelman HE, Zhang L. Proteomic modeling for HIV-1 infected microglia-astrocyte crosstalk. PLoS One. 2008;3(6):e2507. doi:10.1371/journal.pone.0002507.
- Meconi S, Capo C, Remacle-Bonnet M, Pommier G, Raoult D, Mege JL. Activation of protein tyrosine kinases by Coxiella burnetii: role in actin cytoskeleton reorganization and bacterial phagocytosis. Infect Immun. 2001;69:2520–2526. doi:10.1128/IAI.69.4.2520-2526.2001.
- Gabrusiewicz K, Li X, Wei J, Hashimoto Y, Marisetty AL, Ott M, Wang F, Hawke D, Yu J, Healy LM, et al. Glioblastoma stem cell-derived exosomes induce M2 macrophages and PD-L1 expression on human monocytes. Oncoimmunology. 2018;7(4):e1412909. doi:10.1080/2162402X.2017.1412909.
- Wolf-Dennen K, Gordon N, Kleinerman ES. Exosomal communication by metastatic osteosarcoma cells modulates alveolar macrophages to an M2 tumor-promoting phenotype and inhibits tumoricidal functions. Oncoimmunology. 2020;9(1):1747677. doi:10.1080/2162402X.2020.1747677.
- Liang ZX, Liu HS, Wang FW, Xiong L, Zhou C, Hu T, He XW, Wu XJ, Xie D, Wu XR, et al. LncRNA RPPH1 promotes colorectal cancer metastasis by interacting with TUBB3 and by promoting exosomes-mediated macrophage M2 polarization. Cell Death Dis. 2019;10(11):829. doi:10.1038/s41419-019-2077-0.
- Ham S, Lima LG, Chai EPZ, Muller A, Lobb RJ, Krumeich S, Wen SW, Wiegmans AP, Moller A. Breast cancer-derived exosomes alter macrophage polarization via gp130/STAT3 signaling. Front Immunol. 2018;9:871. doi:10.3389/fimmu.2018.00871.
- Chattopadhyay S, Sun P, Wang P, Abonyo B, Cross NL, Liu L. Fusion of lamellar body with plasma membrane is driven by the dual action of annexin II tetramer and arachidonic acid. J Biol Chem. 2003;278(41):39675–39683. doi:10.1074/jbc.M212594200.
- Valkonen S, Holopainen M, Colas RA, Impola U, Dalli J, Kakela R, Siljander PR, Laitinen S. Lipid mediators in platelet concentrate and extracellular vesicles: molecular mechanisms from membrane glycerophospholipids to bioactive molecules. Biochim Biophys Acta Mol Cell Biol Lipids. 2019;1864(8):1168–1182. doi:10.1016/j.bbalip.2019.03.011.
- McIntosh TJ, Kulkarni KG, Simon SA. Membrane fusion promoters and inhibitors have contrasting effects on lipid bilayer structure and undulations. Biophys J. 1999;76(4):2090–2098. doi:10.1016/S0006-3495(99)77365-0.
- Linton SS, Abraham T, Liao J, Clawson GA, Butler PJ, Fox T, Kester M, Matters GL, Ahmad A. Tumor-promoting effects of pancreatic cancer cell exosomes on THP-1-derived macrophages. PLoS One. 2018;13(11):e0206759. doi:10.1371/journal.pone.0206759.
- Van den Bossche J, O’Neill LA, Menon D. Macrophage immunometabolism: where are we (going)? Trends Immunol. 2017;38(6):395–406. doi:10.1016/j.it.2017.03.001.
- Van den Bossche J, Baardman J, Otto NA, van der Velden S, Neele AE, van den Berg SM, Luque-Martin R, Chen HJ, Boshuizen MC, Ahmed M, et al. Mitochondrial dysfunction prevents repolarization of inflammatory macrophages. Cell Rep. 2016;17(3):684–696. doi:10.1016/j.celrep.2016.09.008.
- Tannahill GM, Curtis AM, Adamik J, Palsson-McDermott EM, McGettrick AF, Goel G, Frezza C, Bernard NJ, Kelly B, Foley NH, et al. Succinate is an inflammatory signal that induces IL-1beta through HIF-1alpha. Nature. 2013;496(7444):238–242. doi:10.1038/nature11986.
- Van den Bossche J, Lamers WH, Koehler ES, Geuns JM, Alhonen L, Uimari A, Pirnes-Karhu S, Van Overmeire E, Morias Y, Brys L, et al. Pivotal advance: arginase-1-independent polyamine production stimulates the expression of IL-4-induced alternatively activated macrophage markers while inhibiting LPS-induced expression of inflammatory genes. J Leukoc Biol. 2012;91(5):685–699. doi:10.1189/jlb.0911453.
- Sui Y, Zeng W. MS4A4A regulates arginase 1 induction during macrophage polarization and lung inflammation in mice. Eur J Immunol. 2020;50(10):1602–1605. doi:10.1002/eji.202048585.
- Xiao M, Zhang J, Chen W, Chen W. M1-like tumor-associated macrophages activated by exosome-transferred THBS1 promote malignant migration in oral squamous cell carcinoma. J Exp Clin Cancer Res. 2018;37(1):143. doi:10.1186/s13046-018-0815-2.
- Gerloff D, Lutzkendorf J, Moritz RKC, Wersig T, Mader K, Muller LP, Sunderkotter C. Melanoma-derived exosomal mir-125b-5p educates tumor associated macrophages (tams) by targeting lysosomal acid lipase a (lIPA). Cancers (Basel). 2020;12(2):464. doi:10.3390/cancers12020464.
- Qian M, Wang S, Guo X, Wang J, Zhang Z, Qiu W, Gao X, Chen Z, Xu J, Zhao R, et al. Hypoxic glioma-derived exosomes deliver microRNA-1246 to induce M2 macrophage polarization by targeting TERF2IP via the STAT3 and NF-kappaB pathways. Oncogene. 2020;39(2):428–442. doi:10.1038/s41388-019-0996-y.
- Cossetti C, Iraci N, Mercer TR, Leonardi T, Alpi E, Drago D, Alfaro-Cervello C, Saini HK, Davis MP, Schaeffer J, et al. Extracellular vesicles from neural stem cells transfer IFN-gamma via ifngr1 to activate stat1 signaling in target cells. Mol Cell. 2014;56(2):193–204. doi:10.1016/j.molcel.2014.08.020.
- Gabrielli M, Battista N, Riganti L, Prada I, Antonucci F, Cantone L, Matteoli M, Maccarrone M, Verderio C. Active endocannabinoids are secreted on extracellular membrane vesicles. EMBO Rep. 2015;16(2):213–220. doi:10.15252/embr.201439668.
- Yang M, Chen J, Su F, Yu B, Su F, Lin L, Liu Y, Huang JD, Song E. Microvesicles secreted by macrophages shuttle invasion-potentiating microRNAs into breast cancer cells. Mol Cancer. 2011;10(1):117. doi:10.1186/1476-4598-10-117.
- Lan J, Sun L, Xu F, Liu L, Hu F, Song D, Hou Z, Wu W, Luo X, Wang J, et al. M2 macrophage-derived exosomes promote cell migration and invasion in colon cancer. Cancer Res. 2019;79(1):146–158. doi:10.1158/0008-5472.CAN-18-0014.
- Hu Y, Li D, Wu A, Qiu X, Di W, Huang L, Qiu L. TWEAK-stimulated macrophages inhibit metastasis of epithelial ovarian cancer via exosomal shuttling of microRNA. Cancer Lett. 2017;393:60–67. doi:10.1016/j.canlet.2017.02.009.
- Aucher A, Rudnicka D, Davis DM. MicroRNAs transfer from human macrophages to hepato-carcinoma cells and inhibit proliferation. J Immunol. 2013;191(12):6250–6260. doi:10.4049/jimmunol.1301728.
- Yin Z, Zhou Y, Ma T, Chen S, Shi N, Zou Y, Hou B, Zhang C. Down-regulated lncRNA SBF2-AS1 in M2 macrophage-derived exosomes elevates miR-122-5p to restrict XIAP, thereby limiting pancreatic cancer development. J Cell Mol Med. 2020;24(9):5028–5038. doi:10.1111/jcmm.15125.
- Azambuja JH, Ludwig N, Yerneni SS, Braganhol E, Whiteside TL. Arginase-1+ exosomes from reprogrammed macrophages promote glioblastoma progression. Int J Mol Sci. 2020;21(11):3990. doi:10.3390/ijms21113990.
- Wu J, Gao W, Tang Q, Yu Y, You W, Wu Z, Fan Y, Zhang L, Wu C, Han G, et al. M2 macrophage-derived exosomes facilitate hepatocarcinoma metastasis by transferring alphaM beta2 integrin to tumor cells. Hepatology. 2020. doi:10.1002/hep.31432.
- Baitsch D, Bock HH, Engel T, Telgmann R, Muller-Tidow C, Varga G, Bot M, Herz J, Robenek H, von Eckardstein A, et al. Apolipoprotein E induces antiinflammatory phenotype in macrophages. Arterioscler Thromb Vasc Biol. 2011;31(5):1160–1168. doi:10.1161/ATVBAHA.111.222745.
- Zheng P, Luo Q, Wang W, Li J, Wang T, Wang P, Chen L, Zhang P, Chen H, Liu Y, et al. Tumor-associated macrophages-derived exosomes promote the migration of gastric cancer cells by transfer of functional apolipoprotein E. Cell Death Dis. 2018;9(4):434. doi:10.1038/s41419-018-0465-5.
- Shobaki N, Sato Y, Suzuki Y, Okabe N, Harashima H. Manipulating the function of tumor-associated macrophages by siRNA-loaded lipid nanoparticles for cancer immunotherapy. J Control Release. 2020;325:235–248. doi:10.1016/j.jconrel.2020.07.001.
- Cowman S, Fuja DG, Liu XD, Tidwell RSS, Kandula N, Sirohi D, Agarwal AM, Emerson LL, Tripp SR, Mohlman JS, et al. Macrophage HIF-1alpha is an independent prognostic indicator in kidney cancer. Clin Cancer Res. 2020;26(18). doi:10.1158/1078-0432.CCR-19-3890.
- Godet I, Shin YJ, Ju JA, Ye IC, Wang G, Gilkes DM. Fate-mapping post-hypoxic tumor cells reveals a ROS-resistant phenotype that promotes metastasis. Nat Commun. 2019;10(1):4862. doi:10.1038/s41467-019-12412-1.
- Tacconi C, Ungaro F, Correale C, Arena V, Massimino L, Detmar M, Spinelli A, Carvello M, Mazzone M, Oliveira AI, et al. Activation of the VEGFC/VEGFR3 pathway induces tumor immune escape in colorectal cancer. Cancer Res. 2019;79(16):4196–4210. doi:10.1158/0008-5472.CAN-18-3657.
- Hughes R, Qian BZ, Rowan C, Muthana M, Keklikoglou I, Olson OC, Tazzyman S, Danson S, Addison C, Clemons M, et al. Perivascular M2 macrophages stimulate tumor relapse after chemotherapy. Cancer Res. 2015;75(17):3479–3491. doi:10.1158/0008-5472.CAN-14-3587.
- El-Arabey AA, Denizli M, Kanlikilicer P, Bayraktar R, Ivan C, Rashed M, Kabil N, Ozpolat B, Calin GA, Salama SA, et al. GATA3 as a master regulator for interactions of tumor-associated macrophages with high-grade serous ovarian carcinoma. Cell Signal. 2020;68:109539. doi:10.1016/j.cellsig.2020.109539.
- Yin Z, Ma T, Huang B, Lin L, Zhou Y, Yan J, Zou Y, Chen S. Macrophage-derived exosomal microRNA-501-3p promotes progression of pancreatic ductal adenocarcinoma through the TGFBR3-mediated TGF-beta signaling pathway. J Exp Clin Cancer Res. 2019;38(1):310. doi:10.1186/s13046-019-1313-x.
- Feng Y, Zhong M, Tang Y, Liu X, Liu Y, Wang L, Zhou H. The role and underlying mechanism of exosomal ca1 in chemotherapy resistance in diffuse large b cell lymphoma. Mol Ther Nucleic Acids. 2020;21:452–463. doi:10.1016/j.omtn.2020.06.016.
- Pan X, Hong X, Lai J, Cheng L, Cheng Y, Yao M, Wang R, Hu N. Exosomal microrna-221-3p confers adriamycin resistance in breast cancer cells by targeting PIK3R1. Front Oncol. 2020;10:441. doi:10.3389/fonc.2020.00441.
- Zhang H, Deng T, Liu R, Ning T, Yang H, Liu D, Zhang Q, Lin D, Ge S, Bai M, et al. CAF secreted miR-522 suppresses ferroptosis and promotes acquired chemo-resistance in gastric cancer. Mol Cancer. 2020;19(1):43. doi:10.1186/s12943-020-01168-8.
- Zheng P, Chen L, Yuan X, Luo Q, Liu Y, Xie G, Ma Y, Shen L. Exosomal transfer of tumor-associated macrophage-derived miR-21 confers cisplatin resistance in gastric cancer cells. J Exp Clin Cancer Res. 2017;36(1):53. doi:10.1186/s13046-017-0528-y.
- Binenbaum Y, Fridman E, Yaari Z, Milman N, Schroeder A, Ben David G, Shlomi T, Gil Z. Transfer of miRNA in macrophage-derived exosomes induces drug resistance in pancreatic adenocarcinoma. Cancer Res. 2018;78(18):5287–5299. doi:10.1158/0008-5472.CAN-18-0124.
- Liu C, Yao Z, Wang J, Zhang W, Yang Y, Zhang Y, Qu X, Zhu Y, Zou J, Peng S, et al. Macrophage-derived CCL5 facilitates immune escape of colorectal cancer cells via the p65/STAT3-CSN5-PD-L1 pathway. Cell Death Differ. 2020;27(6):1765-1781. doi:10.1038/s41418-019-0460-0.
- Yang F, Wang T, Du P, Fan H, Dong X, Guo H. M2 bone marrow-derived macrophage-derived exosomes shuffle microRNA-21 to accelerate immune escape of glioma by modulating PEG3. Cancer Cell Int. 2020;20(1):93. doi:10.1186/s12935-020-1163-9.
- Zhou J, Li X, Wu X, Zhang T, Zhu Q, Wang X, Wang H, Wang K, Lin Y, Wang X. Exosomes released from tumor-associated macrophages transfer mirnas that induce a treg/th17 cell imbalance in epithelial ovarian cancer. Cancer Immunol Res. 2018;6(12):1578–1592. doi:10.1158/2326-6066.CIR-17-0479.
- Hanahan D, Weinberg RA. Hallmarks of cancer: the next generation. Cell. 2011;144:646–674.
- Warburg O. On the origin of cancer cells. Science. 1956;123(3191):309–314. doi:10.1126/science.123.3191.309.
- Muri J, Kopf M. Redox regulation of immunometabolism. Nat Rev Immunol. 2020. doi:10.1038/s41577-020-00478-8 (Online ahead of print).
- Ringel AE, Drijvers JM, Baker GJ, Catozzi A, Garcia-Canaveras JC, Gassaway BM, Miller BC, Juneja VR, Nguyen TH, Joshi S, et al. Obesity shapes metabolism in the tumor microenvironment to suppress anti-tumor immunity. Cell. 2020;183(7). doi:10.1016/j.cell.2020.11.009.
- Dey P, Li J, Zhang J, Chaurasiya S, Strom A, Wang H, Liao WT, Cavallaro F, Denz P, Bernard V, et al. Oncogenic KRAS-driven metabolic reprogramming in pancreatic cancer cells utilizes cytokines from the tumor microenvironment. Cancer Discov. 2020;10(4):608–625. doi:10.1158/2159-8290.CD-19-0297.
- Wang C, Xu J, Yuan D, Bai Y, Pan Y, Zhang J, Shao C. Exosomes carrying ALDOA and ALDH3A1 from irradiated lung cancer cells enhance migration and invasion of recipients by accelerating glycolysis. Mol Cell Biochem. 2020;469(1–2):77–87. doi:10.1007/s11010-020-03729-3.
- Chen F, Chen J, Yang L, Liu J, Zhang X, Zhang Y, Tu Q, Yin D, Lin D, Wong PP, et al. Extracellular vesicle-packaged HIF-1alpha-stabilizing lncRNA from tumour-associated macrophages regulates aerobic glycolysis of breast cancer cells. Nat Cell Biol. 2019;21(4):498–510. doi:10.1038/s41556-019-0299-0.
- Yang E, Wang X, Gong Z, Yu M, Wu H, Zhang D. Exosome-mediated metabolic reprogramming: the emerging role in tumor microenvironment remodeling and its influence on cancer progression. Signal Transduct Target Ther. 2020;5(1):242. doi:10.1038/s41392-020-00359-5.
- Ramos RN, Rodriguez C, Hubert M, Ardin M, Treilleux I, Ries CH, Lavergne E, Chabaud S, Colombe A, Tredan O, et al. CD163+tumor-associated macrophage accumulation in breast cancer patients reflects both local differentiation signals and systemic skewing of monocytes. Clin Transl Immunology. 2020;9(2):e1108. doi:10.1002/cti2.1108.
- Zhang H, Li R, Cao Y, Gu Y, Lin C, Liu X, Lv K, He X, Fang H, Jin K, et al. Poor clinical outcomes and immunoevasive contexture in intratumoral il-10-producing macrophages enriched gastric cancer patients. Ann Surg. 2020 . doi:10.1097/SLA.0000000000004037.(Online ahead of print)
- Travers M, Brown SM, Dunworth M, Holbert CE, Wiehagen KR, Bachman KE, Foley JR, Stone ML, Baylin SB, Casero RA Jr., et al. DFMO and 5-azacytidine increase m1 macrophages in the tumor microenvironment of murine ovarian cancer. Cancer Res. 2019;79(13):3445–3454. doi:10.1158/0008-5472.CAN-18-4018.
- Banerjee S, Halder K, Ghosh S, Bose A, Majumdar S. The combination of a novel immunomodulator with a regulatory T cell suppressing antibody (DTA-1) regress advanced stage B16F10 solid tumor by repolarizing tumor associated macrophages in situ. Oncoimmunology. 2015;4(3):e995559. doi:10.1080/2162402X.2014.995559.
- Song M, Liu T, Shi C, Zhang X, Chen X. Bioconjugated manganese dioxide nanoparticles enhance chemotherapy response by priming tumor-associated macrophages toward m1-like phenotype and attenuating tumor hypoxia. ACS Nano. 2016;10(1):633–647. doi:10.1021/acsnano.5b06779.
- Xu B, Cui Y, Wang W, Li S, Lyu C, Wang S, Bao W, Wang H, Qin M, Liu Z, et al. Immunomodulation-enhanced nanozyme-based tumor catalytic therapy. Adv Mater. 2020;32(33):e2003563. doi:10.1002/adma.202003563.
- Zanganeh S, Hutter G, Spitler R, Lenkov O, Mahmoudi M, Shaw A, Pajarinen JS, Nejadnik H, Goodman S, Moseley M, et al. Iron oxide nanoparticles inhibit tumour growth by inducing pro-inflammatory macrophage polarization in tumour tissues. Nat Nanotechnol. 2016;11(11):986–994. doi:10.1038/nnano.2016.168.
- Tian Y, Li S, Song J, Ji T, Zhu M, Anderson GJ, Wei J, Nie G. A doxorubicin delivery platform using engineered natural membrane vesicle exosomes for targeted tumor therapy. Biomaterials. 2014;35(7):2383–2390. doi:10.1016/j.biomaterials.2013.11.083.
- Trivedi M, Talekar M, Shah P, Ouyang Q, Amiji M. Modification of tumor cell exosome content by transfection with wt-p53 and microRNA-125b expressing plasmid DNA and its effect on macrophage polarization. Oncogenesis. 2016;5(8):e250. doi:10.1038/oncsis.2016.52.
- Su MJ, Aldawsari H, Amiji M. Pancreatic cancer cell exosome-mediated macrophage reprogramming and the role of micrornas 155 and 125b2 transfection using nanoparticle delivery systems. Sci Rep. 2016;6(1):30110. doi:10.1038/srep30110.
- Nie W, Wu G, Zhang J, Huang LL, Ding J, Jiang A, Zhang Y, Liu Y, Li J, Pu K, et al. Responsive exosome nano-bioconjugates for synergistic cancer therapy. Angew Chem Int Ed Engl. 2020;59(5):2018–2022. doi:10.1002/anie.201912524.
- Doble A, Fox A, Glynn LE, Kingston D. The non-passage of mycobacteria from freund’s complete adjuvant granuloma depots to arthritic joints. Br J Exp Pathol. 1975;56:537–543.
- Tauber AL, Schweiker SS, Levonis SM. From tea to treatment; epigallocatechin gallate and its potential involvement in minimizing the metabolic changes in cancer. Nutr Res. 2020;74:23–36. doi:10.1016/j.nutres.2019.12.004.
- Jang JY, Lee JK, Jeon YK, Kim CW. Exosome derived from epigallocatechin gallate treated breast cancer cells suppresses tumor growth by inhibiting tumor-associated macrophage infiltration and M2 polarization. BMC Cancer. 2013;13(1):421. doi:10.1186/1471-2407-13-421.
- Chuang HY, Su YK, Liu HW, Chen CH, Chiu SC, Cho DY, Lin SZ, Chen YS, Lin CM. Preclinical evidence of stat3 inhibitor pacritinib overcoming temozolomide resistance via downregulating mir-21-enriched exosomes from m2 glioblastoma-associated macrophages. J Clin Med. 2019;8(7). doi:10.3390/jcm8070959.
- Wang P, Wang H, Huang Q, Peng C, Yao L, Chen H, Qiu Z, Wu Y, Wang L, Chen W. Exosomes from M1-polarized macrophages enhance paclitaxel antitumor activity by activating macrophages-mediated inflammation. Theranostics. 2019;9(6):1714–1727. doi:10.7150/thno.30716.
- Li J, Li N, Wang J. M1 macrophage-derived exosome-encapsulated cisplatin can enhance its anti-lung cancer effect. Minerva Med. 2020. doi:10.23736/S0026-4806.20.06564-7.
- Schijns VE, Lavelle EC. Trends in vaccine adjuvants. Expert Rev Vaccines. 2011;10(4):539–550. doi:10.1586/erv.11.21.
- Hou Y, Wang Y, Tang Y, Zhou Z, Tan L, Gong T, Zhang L, Sun X. Co-delivery of antigen and dual adjuvants by aluminum hydroxide nanoparticles for enhanced immune responses. J Control Release. 2020;326:120–130. doi:10.1016/j.jconrel.2020.06.021.
- Melssen MM, Petroni GR, Chianese-Bullock KA, Wages NA, Grosh WW, Varhegyi N, Smolkin ME, Smith KT, Galeassi NV, Deacon DH, et al. A multipeptide vaccine plus toll-like receptor agonists LPS or polyICLC in combination with incomplete Freund’s adjuvant in melanoma patients. J Immunother Cancer. 2019;7(1):163. doi:10.1186/s40425-019-0625-x.
- Takamura S, Matsuo K, Takebe Y, Yasutomi Y. Ag85B of mycobacteria elicits effective CTL responses through activation of robust th1 immunity as a novel adjuvant in DNA vaccine. J Immunol. 2005;175(4):2541–2547. doi:10.4049/jimmunol.175.4.2541.
- Cheng L, Wang Y, Huang L. Exosomes from M1-polarized macrophages potentiate the cancer vaccine by creating a pro-inflammatory microenvironment in the lymph node. Molecular Therapy: The Journal of the American Society of Gene Therapy. 2017;25(7):1665–1675. doi:10.1016/j.ymthe.2017.02.007.
- Braun DA, Hou Y, Bakouny Z, Ficial M, Sant’ Angelo M, Forman J, Ross-Macdonald P, Berger AC, Jegede OA, Elagina L, et al. Interplay of somatic alterations and immune infiltration modulates response to PD-1 blockade in advanced clear cell renal cell carcinoma. Nat Med. 2020;26(6):909–918. doi:10.1038/s41591-020-0839-y.
- Capdevila J, Wirth LJ, Ernst T, Ponce Aix S, Lin CC, Ramlau R, Butler MO, Delord JP, Gelderblom H, Ascierto PA, et al. PD-1 blockade in anaplastic thyroid carcinoma. J Clin Oncol. 2020;38(23):2620-2627. doi:10.1200/JCO.19.02727.
- Miller AM, DeAngelis LM. Reevaluation of the frequent use of pd-1 checkpoint inhibitors for treatment of glioblastoma. JAMA. 2020;323(24):2482. doi:10.1001/jama.2020.5934.
- Choo YW, Kang M, Kim HY, Han J, Kang S, Lee JR, Jeong GJ, Kwon SP, Song SY, Go S, et al. M1 macrophage-derived nanovesicles potentiate the anticancer efficacy of immune checkpoint inhibitors. ACS Nano. 2018;12(9):8977–8993. doi:10.1021/acsnano.8b02446.