ABSTRACT
CD80 is recognized as one of the most potent costimulatory molecules by which immune cells limit cancer progression; however, the current understanding of the regulation of its expression on human tumor cells is limited. The TP53 tumor suppressor plays a critical role in cancer and its significant role in the control of immune responses is emerging. Here, we evaluated the role of TP53 as a regulator of CD80 expression in human cancer cells. A set of well-known TP53–reactivating compounds were used on TP53-wild-type, TP53-deficient, TP53-mutated and TP53-knockdown cancer cell lines to determine if TP53 can regulate CD80. CD80 expression was analyzed in samples from patients with TP53-active vs TP53-inactive Colon Adenocarcinomas (COAD) from TCGA panCancer Atlas. We report that the pharmacological activation of TP53 can stimulate the expression of CD80 in human tumor cells of epithelial origin. We also provide evidence that CD80 expression exhibits a strong correlation with TP53 activation in a subgroup of colon tumors with better overall survival. These results confirm the link between TP53 and immune surveillance in human cancer and provide the possibility that conventional TP53-activation approaches for tumoricidal effects may be repurposed for immunotherapy strategies.
KEYWORDS:
INTRODUCTION
Inactivating mutations of the tumor suppressor gene TP53 or the abrogation of its pathway through the inactivation of other signaling or effector components are the most common features of cancer cellsCitation1. Several studies documented TP53 critical role in the regulation of cell cycle arrest, senescence, apoptosis, DNA damage, and other stress signals. Recent studies extended our understanding in the biological activities of TP53 as it emerged also as a significant player in the control of immune responses, specifically in several aspects of the cytotoxic T-lymphocyte (CTL)-tumor cell interaction.Citation2 TP53 status was shown to influence both innate and cell-mediated immunity through the regulation of the MHC-I antigen processing pathway, the transcriptional control of immunomodulatory ligands, and by shaping the immune landscape in the microenvironment.Citation3,Citation4 In general, perturbations in TP53 contributed to the ability of tumor cells to escape from immune surveillance, thus promoting an immunosuppressive environment. However, the underlying mechanisms are still poorly understood.
Tumor cells employ several immune-suppressive strategies to overcome antitumor immunity. One such method is the modulation on the tumor cell surface of the expression of T cell cosignaling molecules, which are required for effective T cell activation and consequently a successful elimination of cancer cells.Citation5 CD80 is recognized as one of the most potent costimulatory molecules by which immune cells limit malignant growth.Citation6 It is upregulated upon cell stress and it is critical for efficacious immune surveillance during carcinogenesis.Citation7–9 Low surface expression of CD80 was reported as an immune escape mechanism of colon carcinoma;Citation10 on the other hand, its expression resulted enhanced in high-frequency microsatellite instability (MSI) colorectal cancers, a CRC subtype which is highly immunogenic and associated with a better prognosis.Citation11 Both in vivo and in vitro studies showed that the upregulation of CD80 on tumor cell surface successfully activates anti-tumor immune responses, while its expression is frequently lost during tumor progression probably due to selective pressure by the immune system.Citation7,Citation12–14 Thus, to develop effective approaches for cancer immunotherapy, strategies for enhancing CD80 expression in tumors are urgently required.Citation15 However, the current understanding of the regulation of CD80 expression is limited.
Recently, data from murine oral cancer cells suggested that functional TP53 is responsible for modulation of immunogenic components such as CD80Citation16. Moreover, a patent was granted to CD80 as a biomarker of TP53 activity in murine models and cell lines.Citation17 Since an increasing number of reports has identified differences in the p53 pathway between mouse and human,Citation18 in this study we investigated the potential role of TP53 in the regulation of CD80 in human cancer cells. Using a set of well-known TP53 – reactivating compounds as tools to address the biology of TP53, we confirm the potential mechanism of anti-tumor immune response evoked by TP53 via induction of CD80 in human cancer epithelial cells.
MATERIALS AND METHODS
Cell culture and treatments
Human cancer cell lines were obtained from ATCC (Manassas, VA, USA) and cultured in DMEM medium supplemented with 10% FBS and 1% penicillin/streptomycin (all from Gibco-Thermo Fisher Scientific, Waltham, USA) at 37°C in a humidified incubator containing 5% CO2. Cells were treated with 10 μM Nutlin-3a (Cayman Chemical, Ann Arbor, MO, USA), 1 μM RITA (Cayman Chemical), or 10 μM 5-Fluorouracil (FU) (Sigma-Aldrich, St. Louis, MO, USA) for 24 h. HCT116 and A549 cell lines were transfected with Silencer Pre-Designed Validated TP53 siRNA (ID:106141 and s605, Thermo Fisher Scientific) or Silencer Negative Control #1 siRNA (Thermo Fisher Scientific). The transfection was performed on 60% confluent cells using the Lipofectamine RNAiMAX (Thermo Fisher Scientific) according to the manufacturer instructions and with 10 nM siRNAs. SAOS2 and H1299 cell lines were transfected with pcDNA3 p53 WT or pcDNA3 (Invitrogen-Thermo Fisher Scientific) as control; pcDNA3 p53 WT was a gift from David Meek (Addgene plasmid # 69003). The transfection was performed on 60% confluent cells using Lipofectamine2000 transfection agent (Invitrogen-Thermo Fisher Scientific) according to the manufacturer instructions. Transfected cells were incubated in 5% CO2 at 37°C for 24 h; fresh medium was then added together with TP53 activators or vehicle as control, and the cells were incubated for another 6 h before harvesting for gene expression analysis or 24 h for flow cytometry and western blotting.
Gene expression
Total RNA was isolated using the SV Total RNA Isolation System kit following the manufacturer’s instructions (Promega, Madison, USA). Complementary DNA (cDNA) synthesis was performed using the iScript™ cDNA Synthesis Kit (Bio-Rad, Hercules, CA, USA) according to the manufacturer’s directions. Specific TP53 and CD80 mRNA transcripts were quantified with TaqMan Gene Expression Assay (ID: Hs011034249_m1 and Hs01045163_m1, respectively) using TaqMan Universal Master Mix II with UNG in an ABI PRISM 7000 Sequence Detection System (all by Thermo Fisher Scientific). Other transcripts were quantified with SYBR Green PCR Master Mix (Thermo Fisher Scientific). The expression of the target molecule was normalized to the expression of the ACTB housekeeping gene. The specific forward and reverse primers used for CDKN1A were 5ʹGTCTTGTACCCTTGTGCC3ʹ and 5ʹGGTAGAAATCTGTCATGCTGG3ʹ; for ACTB were 5ʹCTGGACTTCGAGCAAGAGATG3ʹ and 5ʹAGTTGAAGGTAGTTTCGTGGATG3ʹ.
Flow Cytometry
Cell lines treated with pharmacological activators of TP53 or siRNA were trypsinized and washed with 1X PBS before staining. For staining, 105 cells were suspended in PBS/2% FBS with anti-human CD80-PE (clone 2D10.4, eBioscience-Thermo Fisher Scientific) monoclonal antibody for 30 min on ice. Flow cytometric analysis was performed using a FACSCalibur based on CellQuest software (BD-Becton Dickinson, Franklin Lakes, USA).
Western blotting
Western blotting was done as previously described.Citation7 Briefly, 20 μg of total proteins were loaded on 10% SDS-PAGE gel, then transferred on a PVDF membrane (Bio-Rad®). Blots were blocked in 5% skimmed milk TBST (120 mM Tris-HCl [pH 7.4], 150 mM NaCl, and 0.05% Tween 20) overnight at 4°C. After washing, blots were incubated with anti-CD80 (Cell Signaling Technology, Massachusetts, USA; dilution 1:1000) or anti β-actin (Sigma Aldrich, Missouri, USA; dilution 1:5000) diluted in 5% Bovine Serum Albumin (BSA, Sigma-Aldrich) TBST for 1 hour at room temperature. Then membranes were washed and incubated with HRP-conjugated secondary antibody (Sigma-Aldrich) diluted in 5% BSA TBST for 1 h at room temperature. Protein bands were visualized using Clarity™ Western ECL substrate substrates (Bio-rad) and images were captured using the Alliance Q9 system (Uvitec, Cambridge, UK).
The Cancer Genome Atlas (TCGA) dataset analysis
Explorative series consisted of gene expression, mutation, and copy number alteration data from colon adenocarcinoma samples of the TCGA PanCancer Atlas dataset,Citation19 which were analyzed through the cBioPortal (www.cbioportal.org).Citation20
Statistics
Data are shown as mean +/− SEM. Statistical analysis was performed using GraphPad Prism Software 6.0 (GraphPad Software Inc., La Jolla, USA). Comparisons were performed using unpaired, two-tailed Student’s t-test. Differences were considered significant at p < 0,05.
RESULTS
Activation of TP53 induces CD80 expression in human cancer epithelial cells
To address the effect of TP53 activation on CD80 expression regulation, we examined both transcriptional and cell surface expression levels of CD80 by quantitative real-time PCR (qPCR) and flow cytometry analysis in wild-type TP53 (HCT116, A549) or TP53-null (SAOS2 and H1299, transfected with pcDNA3 plasmid vector alone as control or to overexpress TP53) cancer cell lines following treatment with the small molecule Nutlin-3a, which stabilizes TP53. In TP53 expressing-cells, but not in TP53-null cells, the classical TP53 target gene cyclin-dependent kinase inhibitor CDKN1A was induced after TP53 activation by Nutlin-3a, thus demonstrating TP53 responsiveness (). Interestingly, we observed increased CD80 expression upon TP53 activation by Nutlin-3a at both mRNA and protein levels in HCT116 and A549 cell lines (-c). However, Nutlin-3a failed to induce CD80 in TP53-null SAOS2 and H1299 cells, unless they were transfected with a wild-type TP53 expression plasmid, confirming the TP53-dependence. Next, we investigated the effect of other TP53 activating molecules on CD80 cell surface expression. Both RITA and 5-fluorouracil (5FU) treatments induced CD80 expression significantly in TP53-positive but not in TP53-null cells (). The TP53-dependent induction of CD80 expression by these TP53 activators suggests that the induction of CD80 we observed is a general TP53-mediated phenomenon, not limited to the effects of a specific compound only. Moreover, other types of cancer cell lines of epithelial origin and TP53 status were tested for the effect of TP53 activation on CD80 induction. As shown in , Nutlin-3a induced CD80 expression in MCF-7 and U2OS (both TP53 +/+) but not in HT29 (TP53 mut). Finally, to confirm that the induction of CD80 by Nutlin-3a was TP53-dependent, we examined the effects of TP53 silencing with siRNA. As expected, TP53 knock-down reduced Nutlin-3a-induced CDKN1A mRNA levels in both HCT116 and A549 cells (). Because, as shown in -h, TP53 silencing dramatically lowered Nutlin-3a-induced CD80 mRNA and protein levels, we conclude that TP53 is required for CD80 induction in human cancer cells.
Figure 1. TP53 activation induces CD80 expression in human tumor epithelial cells. (a) 10 µM Nutlin-3a treatment induced CD80 expression in TP53-positive tumor cells. Levels of CD80 and CDKN1A transcripts upon Nutlin-3a treatment for 6 h were assessed by quantitative Real-Time polymerase chain reaction (PCR) in HCT116 and A549 cells (TP53-positive) and Saos2 and H1299 cells (TP53-null, transfected with pcDNA3 plasmid vector alone as control or to overexpress TP53). (b) Induction of cell surface expression of CD80 by Nutlin-3a treatment for 24 h relative to background staining determined by isotype-matched control was measured by flow cytometry. (c) Induction of CD80 protein expression by Nutlin-3a treatment for 24 h determined by western blotting. (d) CD80 expression was induced by 600 µM 5FU or 1 µM RITA treatment for 24 h in HCT116 and A549 TP53-positive cells but not in TP53-null cells. (e) Induction of cell surface expression of CD80 by Nutlin-3a was measured by flow cytometry in MCF7 and U2OS cells (TP53-positive) and HT29 cells (TP53-mutant). (f) Efficient TP53 depletion by TP53 siRNA inhibited CDKN1A induction by Nutlin-3a in HCT-116 and A549 cells as assessed by qPCR. Efficient TP53 depletion by TP53 siRNA inhibited CD80 induction by Nutlin-3a in HCT-116 and A549 cells as assessed by qPCR and flow cytometry (g) and western blotting(h). Data shown are representative results of at least 3 independent experiments. Data are presented as mean ± S.E.M. Unpaired, two-tailed Student’s t-test was used for comparison. *p < 0,05; **p < 0,01; ***p < 0,001 vs control (ctrl) or scramble siRNA
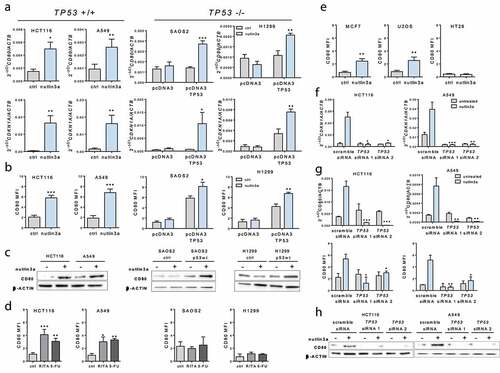
CD80 expression exhibits a strong correlation with TP53 activation in MSI colon tumors
In a previous study, we showed that CD80 expression is enhanced in high-frequency microsatellite instability (MSI) colorectal cancers.Citation11 To test whether TP53 activation plays any role in regulating CD80 expression in MSI tumors, we analyzed the TCGA PanCancer dataset for colon adenocarcinoma by partitioning tumors based on TP53 and MSI status. Tumor subtype data (n = 44 MSI vs n = 247 MSS) were available for 291 samples out of 378. Because TP53 protein function can be inactivated not only by mutation but also by the overexpression of key regulatory proteins (such as MDM2, MDM4, or PPM1D),Citation21 the groups designated as “TP53-inactive” included samples with one or more missense or nonsense coding sequence mutations in the TP53 gene, or with TP53 deletion, or MDM2, MDM4 and PPM1D amplification (n = 10 in MSI and n = 177 in MSS). The other groups, designated as “TP53-active”, consisted of samples that did not reveal any coding sequence alteration in the TP53 gene (n = 34 in MSI and n = 70 in MSS); however, it is still possible that these tumors contained TP53 mutations in essential non-coding regions (e.g. promoters, enhancers or introns) or other genomic or epigenetic alterations that can lead to functional TP53 inactivation. As expected, CD80 expression resulted significantly increased in MSI tumors compared to MSS (), whereas there was a trend of reduced expression in ‘TP53-inactive’ tumors when compared to ‘TP53-active’ tumors (), suggesting a role of TP53 in controlling CD80 expression. Interestingly, the frequency of “TP53 active” samples resulted significantly higher in MSI tumors compared to MSS tumors () and CD80 expression was significantly enhanced in MSI TP53 active samples compared to MSI TP53 inactive samples, whereas MSS tumors did not show any differential CD80 expression between the “TP53 active “ and “TP53 inactive” groups, suggesting that CD80 increased expression may rely on TP53 function in MSI colon tumors (). Notably, in MSI tumors without distant metastasis (n = 40), CD80 expression was still significantly augmented in MSI TP53 active samples compared to MSI TP53 inactive samples (), and patients with an inactive TP53 had a worse overall survival than those with active TP53 ().
Figure 2. Correlation between TP53 status and CD80 expression in MSI colon cancer. TCGA colon adenocarcinoma dataset (total n = 291) was partitioned according to the MSI and TP53 status (Cancer Genome Atlas Network, 2018). CD80 expression was analyzed in MSI (n = 44) vs MSS (n = 247) tumors (a) and in “TP53 active” (n = 104) vs “TP53 inactive” (n = 187) group (b). P values were calculated from Student’s t-test. Data are represented as boxplots showing median and min to max values. (c) The frequency of patients with active TP53 was compared in MSI and MSS patients. Fisher exact test was performed. (d) Expression of CD80 was compared in “MSI TP53 active” (n = 34), “MSI TP53 inactive” (n = 10), “MSS TP53 active” (n = 70) and “MSS TP53 inactive” (n = 177) groups. (e) The expression of CD80 was compared in “MSI TP53 active” (n = 31), “MSI TP53 inactive” (n = 9) without distant metastasis (no M) groups. P values were calculated from Student’s t-test. Data are represented as boxplots showing median and min to max values. (f) In the MSI without metastasis group, the Kaplan‐Meier estimate was used to perform the survival analysis from the date of the initial diagnosis, and the log‐rank test was used to compare “TP53 active” and “TP53 inactive” subgroup survival
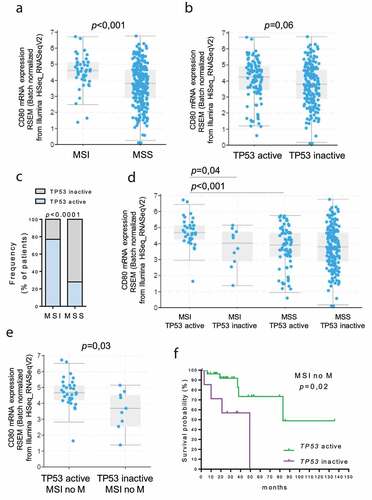
DISCUSSION
The emerging role of TP53 as a regulator of immune surveillance continues to unfold. TP53 enhances anti-tumor immunity by regulation of chemokine production and major histocompatibility complex (MHC) class I expression for recruitment of cytotoxic T cells, enhancement of NKG2D receptors for NK cell activation, Toll-like receptor (TLR) expression for pattern recognition, and inhibition of immune checkpoint molecule PD-L1.Citation3 Our data suggest that TP53 may guard against cancer development also by enhancing CD80 expression on human tumor cells, thus confirming previously described molecular mechanisms controlling CD80 transcription in mouse models.Citation16,Citation17 Moreover, we provide evidence for potential in vivo TP53 triggered induction of CD80 in a subgroup of human MSI CRC, which might partially explain the reason why they are associated with a better prognosis.Citation11
One of the crucial factors that dictates successful anti-tumor immunity and immunotherapy outcome is the productive recruitment of immune effectors to the tumor microenvironment (TME), especially activated tumor-specific CD8 T cells. Compelling data suggest a correlation between TP53 status and immune cell infiltrates in human cancers. Within a subset of breast cancer samples, a correlation between loss of p53 function and the absence of a T-cell gene signature was observed.Citation22 Immunogenomics analysis revealed that the TP53-mutated subtype had a significantly lower degree of immune infiltration than the TP53-wildtype subtype in various cancer types.Citation23 Moreover, in mouse cancer models, loss of TP53 in tumor cells promoted immune tolerance through the recruitment of both myeloid cells and Treg cells.Citation24 Augmented expression of CD80 results in the induction of anti-tumor NK cell cytotoxicity,Citation14 prevention of PD-L1-mediated immune suppression by tumor cells, and restoration of T cell activation,Citation15 as well as sensitization to novel small molecule inhibitor cancer therapies.Citation15 Thus, restoration of CD80 following local pharmacological reactivation of TP53 may serve as a powerful approach for reversing immunosuppression of the TME and promoting systemic, lasting antitumor immunity.Citation6,Citation25,Citation26 Several ongoing clinical trials are using p53-reactivating compounds in combination with different chemotherapeutic drugs, so clues for the applicability and limitations from these clinical studies are eagerly awaited.Citation27
Numerous studies examined whether TP53 mutation affects survival and, in some cases, TP53 mutation has been associated with poorer prognosis.Citation28 However, using TP53 mutation as a prognostic marker may not be useful in all contexts,Citation20 since TP53 protein function can be inactivated not only by mutation but also by the overexpression of key TP53 regulatory proteins with enhanced amplification loci in wild-type TP53 tumors. Our analysis provides evidence that, in the non-metastatic MSI CRC phenotype, a TP53-active pathway signature is associated with better overall survival. This observation has potential clinical application because the concomitant status of the TP53 pathway and CD80 expression could be useful markers of response to therapy. Nevertheless, further study will be needed to confirm this supposition before they can be used as biomarkers in the clinic.
In conclusion, our present findings confirm the link between TP53 and immune surveillance also in human cancer epithelial cells, suggesting that the effect of TP53 on stimulation of anticancer immune response may directly occur via regulation of CD80 expression. This ties tumor immune evasion to other tumor-suppressive pathways previously described for TP53, and suggest that conventional TP53-activation approaches for tumoricidal effects could be repurposed for immunotherapy strategies.
FINANCIAL SUPPORT
This work was partially supported by the Current Research Funding from Italian Ministry of Health to Veneto Institute of Oncology IOV-IRCCS.
DISCLOSURE OF INTEREST
The authors declare no conflicts of interest.
Acknowledgments
The authors are extremely grateful to Professor Giuseppe Opocher, Scientific Director of the Veneto Institute of Oncology, for his constant support.
References
- Brown CJ, Lain S, Verma CS, Fersht AR, Lane DP. Awakening guardian angels: drugging the p53 pathway. Nat Rev Cancer. 2009;9(12):862–6. doi:10.1038/nrc2763.
- Braun MW, Iwakuma T. Regulation of cytotoxic T-cell responses by p53 in cancer. Transl Cancer Res. 2016;5(6):692–697. doi:10.21037/tcr.2016.11.76.
- Blagih J, Buck MD, Vousden KH. p53, cancer and the immune response. J Cell Sci. 2020;133(5):jcs237453. doi:10.1242/jcs.237453.
- Levine AJ. P53 and The Immune Response: 40 Years of Exploration—A Plan for the Future. Int J Mol Sci. 2020;21(2):541. doi:10.3390/ijms21020541.
- Driessens G, Kline J, Gajewski TF. Costimulatory and coinhibitory receptors in anti-tumor immunity. Immunol Rev. 2009;229(1):126–144. doi:10.1111/j.1600-065X.2009.00771.x.
- Chen R, Ganesan A, Okoye I, Arutyunova E, Elahi S, Lemieux MJ, Barakat K. Targeting B7-1 in immunotherapy. Med Res Rev. 2020;40(2):654–682. doi:10.1002/med.21632.
- Marchiori C, Scarpa M, Kotsafti A, Morgan S, Fassan M, Guzzardo V, Porzionato A, Angriman I, Ruffolo C, Sut S, et al. Epithelial CD80 promotes immune surveillance of colonic preneoplastic lesions and its expression is increased by oxidative stress through STAT3 in colon cancer cells. Journal of Experimental & Clinical Cancer Research. 2019;38(1):190. doi:10.1186/s13046-019-1205-0.
- Scarpa M, Fassan M, Kotsafti A, Realdon S, Dall’Olmo L, Morbin T, Cavallin F, Saadeh L, Cagol M, Alfieri R, et al. CD80 expression promotes immune surveillance in Barrett’s metaplasia. OncoImmunology. 2019;8(10):e1636618. doi:10.1080/2162402X.2019.1636618.
- Scarpa M, Brun P, Scarpa M, Morgan S, Porzionato A, Kotsafti A, Bortolami M, Buda A, D’Incà R, Macchi V, et al. CD80-CD28 signaling controls the progression of inflammatory colorectal carcinogenesis. Oncotarget. 2015;6(24):20058–20069. doi:10.18632/oncotarget.2780.
- Tirapu I, Huarte E, Guiducci C, Arina A, Zaratiegui M, Murillo O, Gonzalez A, Berasain C, Berraondo P, Fortes P, et al. Low Surface Expression of B7-1 (CD80) Is an Immunoescape Mechanism of Colon Carcinoma. Cancer Res. 2006;66(4):2442–2450. doi:10.1158/0008-5472.CAN-05-1681.
- Scarpa M, Ruffolo C, Canal F, Scarpa M, Basato S, Erroi F, Fiorot A, Dall’Agnese L, Pozza A, Porzionato A, et al. Mismatch repair gene defects in sporadic colorectal cancer enhance immune surveillance. Oncotarget. 2015;6(41):43472–43482. doi:10.18632/oncotarget.6179.
- Hu J, Batth IS, Xia X, Li S. Regulation of NKG2D+CD8+ T-cell-mediated antitumor immune surveillance: identification of a novel CD28 activation-mediated, STAT3 phosphorylation-dependent mechanism. OncoImmunology. 2016;5(12):e1252012. doi:10.1080/2162402X.2016.1252012.
- Haile ST, Bosch JJ, Agu NI, Zeender AM, Somasundaram P, Srivastava MK, Britting S, Wolf JB, Ksander BR, Tumor Cell O-RS. Programmed Death Ligand 1-Mediated T Cell Suppression Is Overcome by Coexpression of CD80. J Immunol. 2011;186(12):6822–6829. doi:10.4049/jimmunol.1003682.
- Luque I, Reyburn H, Strominger JL. Expression of the CD80 and CD86 molecules enhances cytotoxicity by human natural killer cells. Hum Immunol. 2000;61(8):721–728. doi:10.1016/S0198-8859(00)00136-1.
- Canel M, Taggart D, Sims AH, Lonergan DW, Waizenegger IC, Serrels A. T-cell co-stimulation in combination with targeting FAK drives enhanced anti-tumor immunity . eLife. 2020;9:e48092. Kawakami Y, Settleman J, editors. doi:10.7554/eLife.48092.
- Moore EC, Sun L, Clavijo PE, Friedman J, Harford JB, Saleh AD, Van Waes C, Chang EH, Allen CT. Nanocomplex-based TP53 gene therapy promotes anti-tumor immunity through TP53-and STING-dependent mechanisms. Oncoimmunology. 2018;7(7). doi:10.1080/2162402X.2017.1404216
- CD80 as a Biomarker of p53 Activity and Target for Cancer Therapy | MIT Technology Licensing Office. [accessed February 17, 2021. http://tlo.mit.edu/technologies/cd80-biomarker-p53-activity-and-target-cancer-therapy.
- Fischer M. Conservation and divergence of the p53 gene regulatory network between mice and humans. Oncogene. 2019;38(21):4095–4109. doi:10.1038/s41388-019-0706-9.
- Liu J, Lichtenberg T, Hoadley KA, Poisson LM, Lazar AJ, Cherniack AD, Kovatich AJ, Benz CC, Levine DA, Lee AV, et al. An Integrated TCGA Pan-Cancer Clinical Data Resource to Drive High-Quality Survival Outcome Analytics. Cell. 2018;173(2):400–416.e11. doi:10.1016/j.cell.2018.02.052.
- Cerami E, Gao J, Dogrusoz U, Be G, So S, Ba A, Jacobsen A, Cj B, Ml H, Larsson E, et al. The cBio Cancer Genomics Portal: an Open Platform for Exploring Multidimensional Cancer Genomics Data. Cancer Discov. 2012;2(5):401–404. doi:10.1158/2159-8290.CD-12-0095.
- Donehower LA, Soussi T, Korkut A, Liu Y, Schultz A, Cardenas M, Li X, Babur O, Hsu T-K, Lichtarge O, et al. Integrated Analysis of TP53 Gene and Pathway Alterations in The Cancer Genome Atlas. Cell Rep. 2019;28(5):1370–1384.e5. doi:10.1016/j.celrep.2019.07.001.
- Quigley D, Silwal-Pandit L, Dannenfelser R, Langerød A, Vollan HKM, Vaske C, Siegel JU, Troyanskaya O, Chin S-F, Caldas C, et al. Lymphocyte Invasion in IC10/Basal-Like Breast Tumors Is Associated with Wild-Type TP53. Mol Cancer Res. 2015;13(3):493–501. doi:10.1158/1541-7786.MCR-14-0387.
- Jiang Z, Liu Z, Li M, Chen C, Wang X. Immunogenomics Analysis Reveals that TP53 Mutations Inhibit Tumor Immunity in Gastric Cancer. Transl Oncol. 2018;11(5):1171–1187. doi:10.1016/j.tranon.2018.07.012.
- Blagih J, Zani F, Chakravarty P, Hennequart M, Pilley S, Hobor S, Hock AK, Walton JB, Morton JP, Gronroos E, et al. Cancer-Specific Loss of p53 Leads to a Modulation of Myeloid and T Cell Responses. Cell Rep. 2020;30(2):481–496.e6. doi:10.1016/j.celrep.2019.12.028.
- Guo G, Yu M, Xiao W, Celis E, Cui Y. Local activation of p53 in the tumor microenvironment overcomes immune suppression and enhances antitumor immunity. Cancer Res. 2017;77(9):2292–2305. doi:10.1158/0008-5472.CAN-16-2832.
- DeLeo AB, Appella E. The p53 Saga: early Steps in the Development of Tumor Immunotherapy. The Journal of Immunology. 2020;204(9):2321–2328. doi:10.4049/jimmunol.1901343.
- Sanz G, Singh M, Peuget S, Selivanova G. Inhibition of p53 inhibitors: progress, challenges and perspectives. J Mol Cell Biol. 2019;11(7):586–599. doi:10.1093/jmcb/mjz075.
- Robles AI, Jen J, Harris CC. Clinical Outcomes of TP53 Mutations in Cancers. Cold Spring Harb Perspect Med. 2016;6(9):a026294. doi:10.1101/cshperspect.a026294.