ABSTRACT
The vast majority (>90%) of glioblastoma (GBM) patients belong to the isocitrate dehydrogenase 1 wild type (IDH1WT) group which exhibits a poor prognosis with a median survival of less than 15 months. This study demonstrated numerous immunosuppressive genes as well as β-catenin gene, pivotal for Wnt/β-catenin signaling, were upregulated in 206 IDH1WT glioma patients using the Chinese Glioma Genome Atlas (CGGA) database. The increase in microglia with an immunosuppressive phenotype and the overexpression of β-catenin protein were further verified in IDH1WT GBM patients and IDH1WT GL261 glioma allografts. Subsequently, we found that IDH1WT GL261 cell-derived conditioned medium activated Wnt/β-catenin signaling in primary microglia and triggered their transition to an immunosuppressive phenotype. Blocking Wnt/β-catenin signaling not only attenuated microglial polarization to the immunosuppressive subtype but also reactivated immune responses in IDH1WT GBM allografts by simultaneously enhancing cytotoxic CD8+ T cell infiltration and downregulating regulatory T cells. Positron emission tomography imaging demonstrated enhanced proinflammatory activities in IDH1WT GBM allografts after the blockade of Wnt signaling. Finally, gavage administration of a Wnt signaling inhibitor significantly restrained tumor proliferation and improved the survival of model mice bearing IDH1WT GBM allografts. Depletion of CD8+ T cells remarkably abrogated the therapeutic efficacy induced by the Wnt signaling inhibitor. Overall, the present work indicates that the crosstalk between IDH1WT glioma cells and immunosuppressive microglia is important in maintaining the immunosuppressive glioma microenvironment. Blocking Wnt/β-catenin signaling is a promising complement for IDH1WT GBM treatment by improving the hostile immunosuppressive microenvironment.
1. Introduction
Glioblastoma (GBM) is the most common primary malignant glioma. As a molecular marker, isocitrate dehydrogenase 1 (IDH1) plays a key role in predicting the prognosis of GBM which is the glioma with the highest malignancy (WHO Grade IV).Citation1 The vast majority (>90%) of GBM patients belong to the IDH1 wild type (IDH1WT) group, and their median survival (< 15 months) is significantly lower than that of IDH1 mutated (IDH1MUT) patients (31 months).Citation2 According to the latest data of the Central Brain Tumor Registry of the United States,Citation1 ~71% of newly diagnosed diffuse gliomas are classified as IDH1WT GBM.Citation3 Therefore, it is urgent to develop new methods to improve the prognosis of IDH1WT GBM patients.
Although immunotherapeutic approaches have been proven to be effective in the treatment of multiple types of cancers, such as lymphoma and melanoma,Citation4 these strategies, including immune checkpoint blockade and therapeutic vaccination, failed to achieve satisfactory therapeutic efficacy in glioma. For example, treatment with the programmed death receptor 1 (PD-1) checkpoint blocker nivolumab (Opdivo) did not prolong overall survival in randomized phase III clinical trials of 369 recurrent glioblastoma patients (NCT02017717).Citation5 The compromised therapeutic responses may result from the immunosuppressive microenvironment of GBM.Citation6 Compared with IDH1MUT GBM, IDH1WT GBM exhibits more pronounced immunosuppressive characteristics,Citation7,Citation8 which may contribute to the different degrees of aggressiveness in IDH1WT GBM and IDH1MUT GBM.Citation9,Citation10 Thus, improving the immunotherapeutic response of IDH1WT GBM by modulating the immunosuppressive microenvironment is a promising strategy.
Microglia are resident macrophages and act as the first and foremost immune defense in the brain.Citation11 Under normal conditions, resting microglia continuously survey the surrounding environment. However, resting cells can rapidly polarize into either classic (immunostimulatory, tumor-restraining) or alternative (immunosuppressive, tumor-supportive) phenotypes in response to stimuli such as infection and damage.Citation12 An increased immunostimulatory/immunosuppressive phenotype ratio of microglia usually indicates better glioma patient prognosis.Citation13 The interaction between glioma cells and microglia is important in maintaining the immunosuppressive microenvironment. Therefore, blocking the communication between tumor-supportive microglia and glioma cells and thereby suppressing the immunosuppressive microenvironment is a promising strategy for the treatment of IDH1WT glioma.
The aberrantly activated Wnt/β-catenin signaling pathway is actively involved in carcinogenesis and the progression of multiple types of tumors.Citation14–16 A growing number of studies indicate that the β-catenin level in IDH1WT glioma cells is markedly increased.Citation17,Citation18 Interestingly, the Wnt/β-catenin signaling level is proportional to the immunosuppressive/immunostimulatory phenotype ratio of microglia.Citation19 Thus, we hypothesize that the Wnt pathway plays a pivotal role in establishing the immunosuppressive microenvironment in IDH1WT GBM by stimulating communication between cancer cells and immunosuppressive microglia.
In this study, we demonstrated that Wnt signaling was crucial in the development of an immunosuppressive microenvironment in IDH1WT GBM by promoting the interaction between immunosuppressive microglia and cancer cells. Blocking the Wnt/β-catenin pathway shifted the microglial phenotype from an immunosuppressive phenotype to an immunostimulatory phenotype, increased the infiltration of CD8+ T cells, and decreased the number of infiltrating regulatory T cells (Treg cells), leading to increased therapeutic responses. In summary, this work presents a strategy for refractory IDH1WT GBM therapy by abolishing the immunosuppressive microenvironment.
2. Materials and methods
2.1. Patient samples
Human specimens were obtained from patients who were newly diagnosed as IDH1WT GBM (n = 4) and IDH1MUT GBM (n = 4) at the Department of Neurosurgery, Huashan Hospital, from 2017 to 2019. Written informed consents were acquired from all participants, and the Institutional Review Board of Huashan Hospital approved the protocol.
2.2. Gene expression analysis
Kaplan-Meier survival analysis and gene expression array for IDH1WT and IDH1MUT glioma were performed in GBM using the Chinese Glioma Genome Atlas (CGGA; http://www.cgga.org.cn/). All searches were performed according to the online instructions of CGGA data portal.
2.3. Cell culture
Murine glioma GL261 cell line was kindly provided by Professor Zhou Liangfu (Department of Neurosurgery, Huashan Hospital in 2018). Murine glioma CT-2A cell line was purchased from Bluefbio (Shanghai) Biology Technology Development Co., Ltd. Human glioma IDH1WT U251 cell line was kindly provided by Professor Chen Liang (Department of Neurosurgery, Huashan Hospital in 2021). All cells were verified by negative mycoplasm tests and grown in DMEM (Meilunbio) with 10% FBS (Thermo-Fisher Scientific) at 37°C with 5% CO2.
2.4. Isolation of primary microglia
Murine primary microglia were obtained from ICR pups (<3 days old, raised in pathogen-free laboratory environment). Brains were excised and placed in 75% ethanol. The cerebellum was isolated before the brains were transferred into Hank’s Balanced Salt Solution (HBSS; Meilunbio). Cortices and meninges were removed. The remaining brains were washed by phosphate buffer saline (PBS; Meilunbio) and digested in 0.25% Trypsin/EDTA (Thermo Fisher Scientific) and 2.5 μg/mL DNase I (Beijing Dingguo Changsheng Biotechnology) for 5 min followed by gentle trituration. Tissue homogenates were passed through a sieve (75-µm mesh) and collected by horizontal centrifugation for 2 min at 2,000 rpm, then resuspended in 12% FBS-F12DMEM medium (Thermo Fisher Scientific) for plating. On the second day after isolation, primary microglia cultures were centrifuged for 2 min at 2,000 rpm. Half of the supernatant was transferred back to the plate, and an equal volume of fresh 12% FBS-F12DMEM was added. The medium for microglia culture was changed every 3 − 4 days. After 14 days, microglia cells were gently shaken off, and were re-plated in 12% FBS-F12DMEM for 2 − 3 days prior to further immunoblotting, immunofluorescence staining or flow cytometry analysis.
2.5. Cell proliferation assay
For determining the cell viability of IDH1WT GL261 cells, IDH1WT CT-2A cells, IDH1WT U251 cells or primary microglia cells after treated with Wnt-C59, the cells were seeded in 96-well plates and then cultured for 1 day, subsequently, exposed into completed DMEM media containing different concentrations of Wnt-C59 (0, 0.05, 0.1, 0.2, 0.4, 0.8, 1.6, 3.2, 6.4 µmol/L). Cancer cell viabilities were determined after culturing with media isolated from primary microglia, immunosuppressive phenotype microglia or Wnt-C59 (3 or 6 µmol/L) treated immunosuppressive microglia (the cells were cultured with Wnt-C59 for 1 day, then washed twice. The cells were cultured for another day in fresh medium). CCK-8 assay solution (10 μL/well, Yeasen Biotechnology) was added and the optical density was obtained after 2 h.
2.6. Wound healing assay
GL261 cells were cultured on 12-well plates (2 ×106/well). 80–90% confluence was usually achieved at 24 h post-seeding. The cells were scratched by a sterile 10 µL micropipette tip and the debris were carefully washed away by PBS. Then scratched cell monolayer was cultured with media isolated from immunosuppressive phenotype microglia or Wnt-C59 (5.2 µmol/L) treated immunosuppressive microglia (the cells were cultured with Wnt-C59 for 1 day, then incubated for another day in the fresh medium after washing twice) for 24 or 48 h.
2.7. Construction of IDH1R132H mutant lentivirus
The DNA plasmid and lentivirus were designed and constructed by Shanghai GeneChem. The vector was Ubi-MCS-3FLAG-CBh-gcGFP-IRES-puromycin, obtained by restriction endonuclease digestion. The following primers were designed by Snapgene software and were used to amplify the sequence by polymerase chain reaction (PCR): F:5ʹ-AGGTCGACTCTAGAGGATCCCGCCACCATGTCCAGAAAAATCCAAGGAGG-3ʹ; R:5ʹ-TCCTTGTAGTCCATACCAAGTTTGGCCTGAGCTAATTTGG-3ʹ. The products were inserted into GV492 plasmid vector in accordance with the manufacturer’s instructions (ClonExpressTM II One Step Cloning Kit, Vazyme, #C122). Then 10 μL reaction products were added to 100 μL competent cells, mixed under the number of elastic tube walls, and placed on the ice for 30 min. Heat shock at 42°C for 90 s, incubation in the ice water bath for 2 min. 500 μL Luria-Bertani (LB) medium was added and cultured in a shaking bed at 37°C for 1 h. The appropriate amount of bacterial solution was evenly coated on the plate containing the ampicillin antibiotics and cultured upside down for 16 h in a constant temperature incubator. The identified positive transformants by PCR were inoculated in LB medium containing ampicillin antibiotics, cultured at 37°C for 12 to 16 h, and sequenced. The correctly sequenced bacteria were transferred to 10 mL LB medium containing corresponding antibiotics and cultured overnight at 37°C. The plasmid was extracted with a small amount of internal toxin-free plasmid extraction kit. Blank GV492 plasmid vector was used as a negative control. IDH1 R132H plasmid, packaging plasmids Helper 1.0 and Helper 2.0 were transferred to 293 T cells for 72 h to lentivirus packaging. The harvested lentivirus was concentrated, purified and stored at −80°C.
2.8. Development of murine models bearing orthotopic glioma with IDH1WT or IDH1MUT genotype
All animal studies were authorized by the Institutional Animal Care and Use Committee (IACUC) of Fudan University. Male C57BL/6 mice (20 − 22 g) were obtained from Shanghai Lingchang Biological Technology and kept in pathogen-free conditions. Animal experiments were conducted according to the guidelines approved by the Ethics Committee of Fudan University.
Mice were anesthetized and then fixed. A small hole was drilled into the skull of the mouse, relative to the bregma (2 mm lateral). Through the hole, the syringe was injected 4.0 mm deep relative to the bregma. The syringe was withdrawn 0.5 mm before implanting with 5 μL of IDH1WT or IDH1MUT GL261 cell suspension (2 ×104 cells). The syringe was withdrawn slowly from the mouse brain, with a pause at Z = −2.5 mm deep for 1 min. Mouse models were checked twice a week and their body-weight were measured every week post-injection of the cancer cells.
2.9. T cell depletion
Subsets of T cells in C57BL/6 mouse models were depleted by intraperitoneal injection (i.p.) of 100 μL corresponding antibody at one day prior to IDH1WT glioma cell inoculation as indicated: anti-CD8α antibody (9.5 mg/kg; clone 2.43, BioXCell) and anti-CD4 antibody (10 mg/kg; clone GK1.5, BioXCell) every four days.Citation20,Citation21 Depletions of CD8+ T cells and CD4+ T cells were confirmed by flow cytometry.
2.10. In vivo magnetic resonance imaging (MRI) studies
C57 mouse models bearing IDH1WT GL261 glioma allograft were randomly assigned to 6 groups (n = 4) that received oral administration of vehicle (100 µL ddH2O containing 0.5% methylcellulose and 0.1% Tween-80) or Wnt-C59 (7.5 or 15 mg/kg) once daily for 7 or 14 days, respectively.Citation22 A detailed description of the MRI methods is provided in Supplementary Materials and Methods.
2.11. In vivo positron emission tomography (PET)/computed tomography (CT) studies
PET/CT imaging was carried out with a microPET/CT scanner (Siemens Inveon). The detailed procedures to acquire images were described in Supplementary Materials and Methods.
2.12. Flow cytometry studies
Brains were minced with scissors before mechanical homogenate and single cell suspensions were obtained through a 40-µm cell filter. The detailed procedures to flow cytometry studies were described in Supplementary Materials and Methods.
2.13. Immunoblotting assay, immunofluorescence and immunohistochemistry staining
The procedures for western blotting, immunofluorescence and immunohistochemistry staining were provided in Supplementary Materials and Methods.
2.14. RT- PCR studies
Total RNA was extracted with Trizol reagent (Life Technologies) from GBM patient tissues and mouse brain tumor tissues. Transcriptor First Strand cDNA Synthesis Kit (Roche) was used to reverse transcribe 1 μg RNA into cDNA. RT-PCR amplifications were accomplished in triplicates in a 25 µL reaction volume with the SYBR Premix Ex Taq Kit (Takara Bio). Primer sequences were provided in the Table. S1.
2.15. Statistical analysis
Results were presented as mean±SD or mean±SEM. The difference was evaluated with Mann-Whitney U-test (Student’s t-test), One-way ANOVA (GraphPad Prism 7.0). The correlation of multiple groups was measured by Pearson’s or Spearman’s correlation. p <.05 was considered statistically significant.
3. Results
3.1. The upregulation of immunosuppressive genes and β-catenin in IDH1WT glioma patients
CGGA data analysis demonstrated that the median survival of IDH1WT GBM was significantly shorter than that of the IDH1MUT subtype (Fig. S1) (p = .002). We hypothesize that this difference may be associated with the highly immunosuppressive microenvironment in IDH1WT GBM.Citation22 To verify this hypothesis, we compared the expression of immunosuppressive genes between IDH1WT GBM and IDH1MUT GBM. However, because only 5‒10% of primary GBM cases are IDH1MUT, we extended the cohort from the GBM (WHO-Grade IV) database to the high-grade glioma database containing WHO Grade III and IV cases, including 206 IDH1WT glioma cases and 183 IDH1MUT glioma cases. The workflow of the data filtering glioma case is shown in . The heat map of key genes involved in immune suppression is shown in . As expected, IDH1WT glioma showed significantly higher levels of immunosuppressive genes than IDH1MUT glioma (Table S2).Citation23,Citation24 Furthermore, the expression of CTNNB1, a pivotal gene in the Wnt/β-catenin pathway, was substantially higher in IDH1WT gliomas than in IDH1MUT gliomas (), suggesting that a correlation may exist between the β-catenin gene and immunosuppressive microenvironment.
Figure 1. The upregulation of immunosuppressive genes and β-catenin in IDH1WT glioma patients. (a) The workflow for filtering glioma cases in CGGA. (b) Heatmap depicting the expression levels of immunosuppressive genes in high-grade IDH1WT or IDH1MUT gliomas from patients in the CGGA cohort. (c) β-catenin gene (CTNNB1) expression levels in high-grade IDH1WT and IDH1MUT gliomas from patients in the CGGA cohort. CGGA: Chinese Glioma Genome Atlas
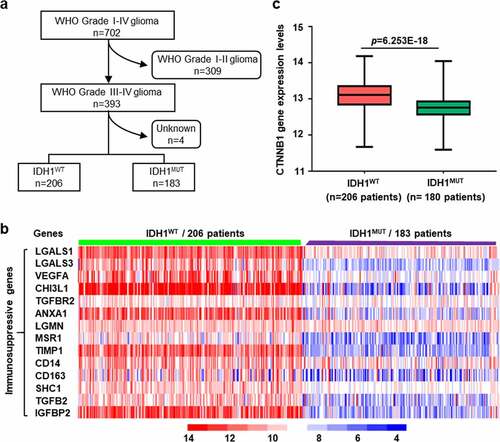
3.2. The upregulation of immunosuppressive microglia and β-catenin protein levels in IDH1WT GBM
Since immune-suppressive microglia is the prominent cell in the GBM microenvironment,Citation25,Citation26 we then investigated the relationship between microglia and β-catenin level in IDH1WT GBM. First, we confirmed that anti-inflammatory microglia were preferentially expressed in surgical specimens from IDH1WT GBM patients by determining the co-localization of transmembrane protein 119 (TMEM119, a specific biomarker for microglia) and CD163 (an anti-inflammatory microglia biomarker) (, Fig. S2a). Then, we found that β-catenin level in the above specimens was also significantly upregulated under immunofluorescence microscopy (, Fig. S2b). Immunoblotting further confirmed that the expression of CD163 and β-catenin was significantly upregulated in IDH1WT GBM patients (, Fig. S2c).
Figure 2. The upregulation of immunosuppressive microglia and β-catenin protein levels in IDH1WT GBM. (a) Representative immunofluorescence images of the microglial marker TMEM119 (green) and the anti-inflammatory microglia marker CD163 (red) in tissues from IDH1WT GBM patients (n = 4). Tissues from IDH1MUT GBM patients were used for control. (b) Representative immunofluorescence images of β-catenin (red) in the above tissues (n = 4). Immunoblots showing CD163, β-catenin and IDH1R132H protein expression in tissues isolated from the above GBM patients (n = 4) (c) and GL261 GBM allografts (n = 4) (d). (e) Strategy for discriminating microglia from tissue specimen with the gate of CD11b+ CD45+ Iba1+ CD49d−. (f) Flow cytometric analysis of the percentage of microglia with different phenotypes in IDH1WT GL261 GBM allografts. Tissues from IDH1MUT GL261 GBM allografts were used for control (n = 4, Student’s t-test). Scale bars: 50 μm at ×20 magnification. All values are shown as Mean±SD. ***p < .001
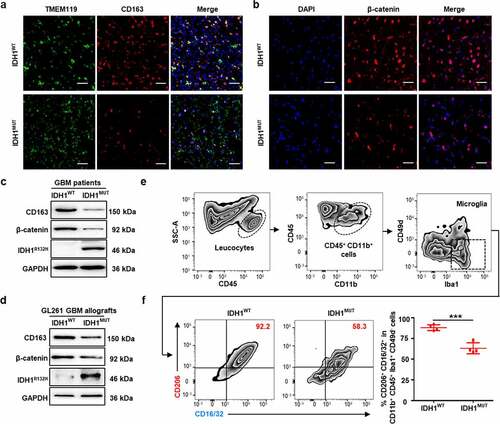
Considering the heterogeneity among the patient specimens,Citation23 we established an IDH1WT GBM allografts model and an IDH1MUT GBM allografts model as a control.Citation27,Citation28 First, the population of immunosuppressive microglia in the above allografts was analyzed by flow cytometry. The experimental results demonstrated that the immunosuppressive CD206+ CD16/32+ phenotype was the most dominant,Citation29,Citation30 making up 88.0% of all microglia (gated on CD11b+ CD45+ Iba1+ CD49d−) in the IDH1WT group, which was significantly higher than that in the IDH1MUT group (63.2%) (). Consistently, immunoblotting and immunofluorescence studies showed significant upregulation of CD163 and β-catenin in IDH1WT GBM allografts (, Fig. S2d-h). Furthermore, the intratumoral infiltrations of T cells and dendritic cells (DCs) in IDH1WT GBM and IDH1MUT GBM allografts were analyzed by flow cytometry. As shown in Suppl. Fig. 2i-n, though the total T cell (CD3+) infiltration in IDH1WT GL261 GBM allografts was comparable to that of IDH1MUT group, the infiltration degrees of CD8+ T cells and DCs were significantly decreased but the infiltration of Treg cells was significantly increased in IDH1WT GBM. These results confirmed the results of CGGA cohort, namely the upregulation of immunosuppressive genes and β-catenin in IDH1WT glioma patients. In addition, we established an IDH1WT CT-2A glioma cell and an IDH1MUT CT-2A glioma cell as controls. Immunoblotting studies showed significant upregulation of β-catenin protein in IDH1WT CT-2A glioma cells, which is consistent with results from IDH1WT GBM specimens from patients and IDH1WT GBM allograft. (Fig. S2o). Together, these data demonstrated that a positive correlation may exist between immunosuppressive microglia and β-catenin levels in IDH1WT GBM.
3.3. IDH1WT GBM-derived culture medium triggers immunosuppressive phenotypic transition in microglia by activating Wnt/β-catenin signaling
To interrogate the potential link between tumor-associated microglia and β-catenin, we isolated primary microglia from the hippocampus of newborn mice and then treated the microglia with normal medium (NM), IDH1WT GL261 GBM-derived conditioned media (CM-WT) or IDH1MUT GL261 GBM-derived conditioned media (CM-MUT) (). Immunoblotting revealed that the expression of CD163 increased 99.4% in the CM-WT group compared with the CM-MUT group (). Furthermore, the expression of Wnt3a and β-catenin increased 39.0% and 86.1%, respectively, in the CM-WT group compared with the CM-MUT group, whereas the expression of GSK-3β (a destruction complex leading to CTNNB1 proteasomal degradation) decreased 47.6% in the CM-WT group compared to the CM-MUT group (). Flow cytometry showed that the number of immunosuppressive microglia in the CM-WT group markedly increased 80.4% and 27.0-fold compared with that in the CM-MUT group and NM group, respectively (). Additionally, qRT-PCR analysis revealed that the mRNA level of signal transducer and activator of transcription 6 (STAT6) increased 1.4-fold in the CM-WT group compared with the CM-MUT group (Fig. S3a). Furthermore, confocal microscopic imaging demonstrated that while the immunofluorescence of CD163 and β-catenin remained low in the isolated primary microglia in the NM group and CM-MUT group, marked increases in the immunofluorescence intensity were observed in the CM-WT group (p <.001) (, Fig. S3b). These data suggest that IDH1WT glioma cells facilitate the transformation of primary microglia to an immunosuppressive phenotype by activating the Wnt pathway.
Figure 3. IDH1WT GBM cell-derived conditioned medium transforms primary microglia to an immunosuppressive phenotype by activating Wnt/β-catenin signaling. (a) Schematic illustration of the treatment of primary microglia with IDH1WT or IDH1MUT GL261 glioma cell-derived conditioned medium (CM). Primary microglia were cultured with CM from IDH1WT cells (CM-WT) or IDH1MUT cells (CM-MUT) for 24 h, while the control group was cultured in normal F12/DMEM (NM). (b) Immunoblots showing CD163, Wnt3a, GSK-3β and β-catenin in primary microglia treated with IDH1WT or IDH1MUT GL261 glioma cell-derived conditioned medium (CM). Primary microglia were cultured with CM from IDH1WT cells (CM-WT) or IDH1MUT cells (CM-MUT) for 24 h, while the control group was cultured in normal F12/DMEM (NM) (n = 4). Mean±SD are shown. (c) Flow cytometric analysis of CD11b+CD45low cells in primary microglia treated with CM (n = 4). Mean±SD are shown. (d) Representative white light and immunofluorescence images of primary microglia cultured in NM, CM-WT and CM-MUT for 24 h. CD163 and β-catenin are displayed in green and red, respectively. The nuclei were stained with DAPI (blue) (n = 4). Student’s t-test. Scale bar: 50 μm. ***p < .001. exp.: expression. NM: normal F12/DMEM medium; CM: conditioned medium; CM-WT: conditioned medium from IDH1WT cells; CM-MUT: conditioned medium from IDH1MUT cells
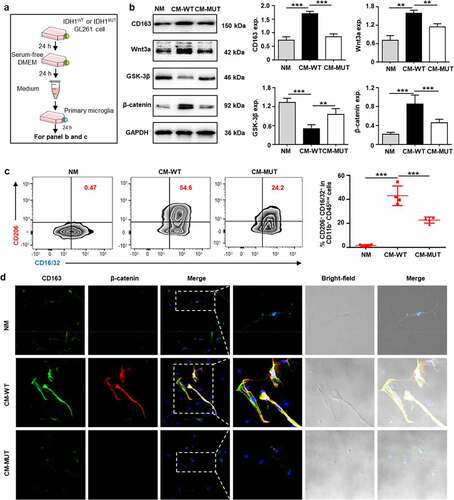
3.4. Wnt/β-catenin signaling blockade inhibits IDH1WT GBM cell migration and proliferation by abolishing immunosuppressive microglia
Wnt-C59, a porcupine (PORCN) inhibitor that prevents Wnt palmitoylation, secretion, and biological activity, was added to IDH1WT GL261 glioma cells at the half-maximal inhibitory concentration (IC50) (6.4 μmol/L) for 24 h (Fig. S4a).Citation31 After being washed and cultured in fresh medium for another 24 h, glioma-derived conditioned medium was added to the primary microglia (). Immunoblotting showed that the expression of Wnt3a and β-catenin in primary microglia treated with the CM (CM-WT-C59) was significantly lower than that in primary microglia treated with CM isolated from IDH1WT GL261 glioma cells without Wnt-C59 treatment (CM-WT) (, Fig. S4c). Interestingly, no difference in the degree of polarization was observed in primary microglia that were cultured with Wnt-59-treated IDH1MUT or Wnt-59-treated IDH1WT glioma-derived conditioned media (Fig. S4d). Furthermore, flow cytometry showed that the percentage of CD206+ immunosuppressive microglia and CD206+ CD16/32+ immunosuppressive microglia decreased 90.7% and 85.9%, respectively (), while the percentage of immunostimulatory microglia (CD16/32+) substantially increased 1.2-fold in the CM-WT-C59 group compared to the CM-WT group (Fig. S4e). These results suggested that inhibiting the Wnt pathway in IDH1WT glioma cells efficiently blocked the transition of microglia to a tumor-supportive phenotype. Similar experimental results were achieved when IDH1WT GL261 glioma cells were replaced by murine IDH1WT CT-2A glioma cells or human glioblastoma IDH1WT U251 cells (Fig. S4f-k).
Figure 4. Wnt/β-catenin signaling blockade inhibits IDH1WT GBM migration and proliferation by abolishing immunosuppressive microglia. (a) Schematic illustration showing the treatment of primary microglia with conditioned medium from IDH1WT GL261 cells (CM-WT) or medium from IDH1WT GL261 cells pretreated with Wnt-C59 (CM-WT-C59) for 24 h. Immunoblots of primary microglia after the corresponding treatments are shown in panel (b). (c) Flow cytometric analysis of CD11b+CD45low cells after the corresponding treatments (n = 4). Mean±SD are shown. (d) Schematic illustration of the treatment of IDH1WT GL261 cells with conditioned medium from immunosuppressive microglia (CM-IM) or Wnt-C59-pretreated immunosuppressive microglia (CM-C59-IM). (e) Immunoblots of Wnt-C59- or F12/DMEM-treated immunosuppressive microglia. (f) Wound healing assay showing IDH1WT GL261 cell monolayers at 24 h after treatment with CM-IM or CM-C59-IM. Scale bar, 100 μm. All values are shown as Mean±SD. Student’s t-test. ***p < .001. CM-WT: conditioned medium from IDH1WT GL261 cells; CM-WT-C59: conditioned medium from Wnt-59-treated IDH1WT GL261 cells; IM: immunosuppressive microglia; C59-IM: Wnt-59-treated immunosuppressive microglia; CM-IM: conditioned medium isolated from immunosuppressive microglia; CM-C59-IM: conditioned medium from Wnt-59-treated immunosuppressive microglia
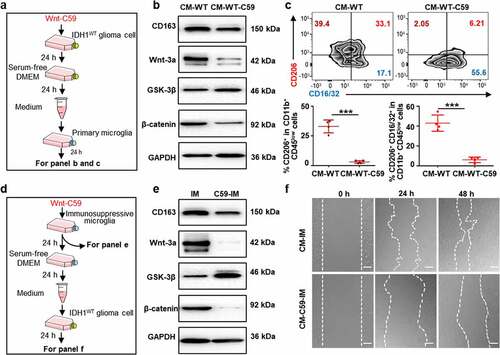
To investigate the effect of immunosuppressive microglia on IDH1WT glioma cells, GL261 cancer cells were treated with conditioned medium isolated from immunosuppressive microglia (CM-IM) or immunosuppressive microglia pretreated with the inhibitor Wnt-C59 (CM-C59-IM) (, Fig. S4b). Wnt-C59 treatment decreased the β-catenin level in immunosuppressive microglia by 92.3% and increased the GSK-3β level by 3.9-fold, suggesting that Wnt signaling in immunosuppressive microglia (IM) was quenched by the inhibitor Wnt-C59 (, Fig. S4i). Additionally, Wnt-C59 reduced the level of CD163 in immunosuppressive microglia (, Fig. S4i). The wound-healing assay showed that CM-C59-IM treatment decreased the GL261 cell migration rate, with markedly reduced migration distances of 34.8% and 65.2% in comparison to GL261 cells treated with CM-IM for 24 h and 48 h, respectively (). Cytotoxicity studies showed that the viability of IDH1WT GL261 tumor cells treated with CM-IM supplemented with 6 μmol/L Wnt-C59 (CM-6 C59-IM) for 24 h was markedly lower than those treated with MIM without Wnt-C59 treatment or pretreated with 3 μmol/L Wnt-C59 (CM-3 C59-IM) (Fig. S4m). These results suggest that blocking Wnt signaling in immunosuppressive microglia can inhibit the migration and proliferation of IDH1WT GBM cells.
3.5. Wnt/β-catenin signaling blockade reactivates the immune response in IDH1WT GBM allografts
Wnt signaling blockade rebalanced the tumor immunosuppressive microenvironment in IDH1WT GL261 GBM allografts. Translocator protein (TSPO), which is primarily expressed on immunostimulatory microglia,Citation32 was used as a biomarker to evaluate intratumoural neuroinflammation.Citation33 In vivo PET/CT imaging showed that the standardized uptake value (SUV) of [18F]DPA-714, a TSPO-targeted PET radiotracer, in IDH1WT gliomas at 14 days after Wnt-C59 treatment (15 mg/kg) was 5.2-fold higher than that in the control group (). Flow cytometric analysis of the dissociated tumor cells showed 8.7-fold increases in the immunostimulatory microglial population (CD16/32+ in the CD11b+ CD45+ Iba1+ CD49d− population) at 14 days after Wnt-C59 treatment (15 mg/kg) in comparison to that of control group. Moreover, the immunosuppressive microglial population (CD206+ CD16/32+ in the CD11b+ CD45+ Iba1+ CD49d− population) decreased by 63.8% (). Additionally, Wnt-C59 increased the number of tumor-infiltrating lymphocytes (TILs, CD3+) (Fig. S5a), especially cytotoxic T lymphocytes (CTLs, CD3+ CD8+) (). The cytotoxic T cell number at 14 days after 15 mg/kg Wnt-C59 treatment increased 18.7% and 193.7% in comparison to the value after treatment with 7.5 mg/kg Wnt-C59 and vehicle, respectively. Moreover, Treg cells (CD4+ Foxp3+ CD8− CD25+) decreased by 82.1% and 92.1% (). Immunoblot analysis indicated that compared with that of the control group, the expression of GSK-3β at 14 days after Wnt-C59 (15 mg/kg) treatment increased by 1.4-fold. Furthermore, the levels of CD163 and β-catenin decreased by 93.6% and 94.8%, respectively (, Fig. S5b). Moreover, compared with that of the control group, the mRNA expression of proinflammatory factors, including IFN-γ and iNOS, increased 2.1-fold and 21.3-fold, respectively, after 15 mg/kg Wnt-C59 treatment for 14 days. Notably, the mRNA levels of anti-inflammatory factors, including TGF-β and IL-10, decreased 46.0% and 31.2%, respectively, at 7 days after 15 mg/kg Wnt-C59 treatment compared with those of the control group (). In summary, these data indicated that blocking Wnt signaling stimulates the immune microenvironment in IDH1WT GBM by promoting the phenotypic transition of microglia, upregulating proinflammatory factors, increasing intratumoural cytotoxic CD8+ T cell infiltration and downregulating regulatory T cells.
Figure 5. Wnt inhibition reactivates immune responses in IDH1WT GBM allografts. (a) Representative [18F]DPA714-PET/CT images of mouse brains bearing IDH1WT GBM allografts at 7 and 14 days after treatment with the Wnt/β-catenin inhibitor Wnt-C59 (15 mg/kg). Right panel, intratumoral SUVmean at 30 min post [18F]DPA714 injection at 14 days (n = 4). Flow cytometric analysis of immunostimulatory (CD16/32+) and immunosuppressive (CD206+ CD16/32+) subtypes among CD11b+ CD45+ Iba1+ CD49d− microglia (b), CD8+ T cells and CD4+ T cells among CD45+ CD3+ cells (c), and CD4+ FOXP3+ T cells among CD45+ CD25+ CD8− cells (d) in untreated and Wnt-C59 (7.5 or 15 mg/kg)-treated model mice (n = 4). (e) Immunoblots showing CD163, Wnt3a, GSK-3β and β-catenin protein expression in tumor tissues isolated from untreated and treated model mice. (f) mRNA expression of IFN-γ, iNOS, TGF-β and IL-10 in the above samples (n = 3). In panel a, values are shown as Mean±SEM. In panel b, c, d and f, values are shown as Mean±SD. Student’s t-test. *p < .05; **p < .01 and ***p < .001
![Figure 5. Wnt inhibition reactivates immune responses in IDH1WT GBM allografts. (a) Representative [18F]DPA714-PET/CT images of mouse brains bearing IDH1WT GBM allografts at 7 and 14 days after treatment with the Wnt/β-catenin inhibitor Wnt-C59 (15 mg/kg). Right panel, intratumoral SUVmean at 30 min post [18F]DPA714 injection at 14 days (n = 4). Flow cytometric analysis of immunostimulatory (CD16/32+) and immunosuppressive (CD206+ CD16/32+) subtypes among CD11b+ CD45+ Iba1+ CD49d− microglia (b), CD8+ T cells and CD4+ T cells among CD45+ CD3+ cells (c), and CD4+ FOXP3+ T cells among CD45+ CD25+ CD8− cells (d) in untreated and Wnt-C59 (7.5 or 15 mg/kg)-treated model mice (n = 4). (e) Immunoblots showing CD163, Wnt3a, GSK-3β and β-catenin protein expression in tumor tissues isolated from untreated and treated model mice. (f) mRNA expression of IFN-γ, iNOS, TGF-β and IL-10 in the above samples (n = 3). In panel a, values are shown as Mean±SEM. In panel b, c, d and f, values are shown as Mean±SD. Student’s t-test. *p < .05; **p < .01 and ***p < .001](/cms/asset/c635b80e-2ca2-4080-88c3-4bee8606d105/koni_a_1932061_f0005_oc.jpg)
3.6. Wnt signaling blockade increases the survival of model mice bearing IDH1WT gliomas
The therapeutic efficacy of Wnt signaling blockade was evident in model mice bearing IDH1WT GBM tumor allografts. In vivo MRI analysis revealed that the tumor volume was reduced by 25.2% and 43.0% at day 7 after treatment with 7.5 mg/kg and 15 mg/kg Wnt-C59, respectively, in comparison with that of the control group. The corresponding values were 52.0% and 61.8%, respectively, at day 14 (). Moreover, 50% of the animals survived over 30 days after treatment with 15 mg/kg Wnt-C59 for 14 days, while none of the animals in the control group survived beyond 25 days (). Immunohistochemical analysis showed that Ki67 staining in tumors was reduced by 29.3% and 31.7% at day 7 post treatment with 7.5 mg/kg and 15 mg/kg Wnt-C59, respectively, in comparison with that of the control group. The corresponding values were 25.8% and 41.3%, respectively, at day 14, suggesting the reduced glioma cell proliferation rate after treatment with the Wnt inhibitor (, Fig. S6). Moreover, no obvious differences in mouse body weight gain were observed between the control and treatment groups.Citation22 Considering the increased cytotoxic CD8+ T cells and concomitant decreased Treg cells in glioma allograft after the treatment of Wnt-C59, we investigated whether depletion of CD4+ T cells or CD8+ T cells compromises the therapeutic efficacy of Wnt inhibitor. CD4+ T cells or CD8+ T cells depletion was conducted by intraperitoneal injection of anti-CD4 or anti-CD8α beginning at one day prior to IDH1WT glioma cell inoculation for every four days (). Successful eradication of CD4+ T cells and CD8+ T cells in glioma allografts were confirmed by flow cytometry (Fig. S7). As shown in , depletion of CD4+ T cells barely affected the survival benefit of Wnt/β-catenin inhibitor Wnt-C59 (15 mg/kg) in mouse models bearing IDH1WT GBM allografts, whereas CD8 antibody remarkably reduced the therapeutic response of Wnt inhibitor. These results indicted the CD8+ T cells played an important role in fulfilling the therapeutic efficacy of Wnt/β-catenin inhibitor.
Figure 6. Wnt/β-catenin signaling blockade shows therapeutic effects on IDH1WT glioma allografts. Representative T2-weighted MRI images (a) and the volumes (b) of IDH1WT GBM allografts after treatment with vehicle or Wnt-C59 (7.5 or 15 mg/kg) for 7 and 14 days (n = 4). Tumor margins are highlighted with yellow dashes. (c) Kaplan-Meier survival curves of IDH1WT model mice treated with 15 mg/kg Wnt-C59 (black line), 7.5 mg/kg Wnt-C59 (blue line) or control (purple line). Log-rank test p values for comparisons to controls are shown (n = 6, p = .003). (d) Ki67 staining in immunohistochemical images of IDH1WT gliomas after the above treatments. Scale bars, 50 μm; magnification 400 × . (e) Mouse models were treated with 100 μL CD4 antibody (Ab) (10 mg/kg) or CD8 Ab (9.5 mg/kg) at day −1, day 3 and day 7 post the IDH1WT glioma cells incubation. (f) Kaplan-Meier survival curves of IDH1WT glioma models treated with CD4 Ab plus 15 mg/kg Wnt-C59 (blue line), CD8 Ab plus 15 mg/kg Wnt-C59 (red line), 15 mg/kg Wnt-C59 (black line) or control (purple line). Log-rank test p values for comparisons to controls are shown (n = 6, CD4 Ab plus 15 mg/kg Wnt-C59 vs 15 mg/kg Wnt-C59 14 d, p = .782; CD4 Ab plus 15 mg/kg Wnt-C59 vs control, p < .001; CD8 Ab plus 15 mg/kg Wnt-C59 vs 15 mg/kg Wnt-C59 14 d, p = .007; CD8 Ab plus 15 mg/kg Wnt-C59 vs control; p = .04. In panel b, ***p < .001, control vs 7.5 mg/kg Wnt-C59 14 d or control vs 15 mg/kg Wnt-C59 14 d (one-way ANOVA)
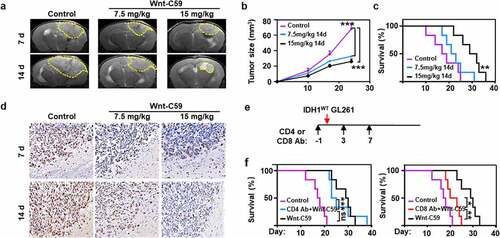
4. Discussion
We demonstrated the upregulation of β-catenin and immunosuppressive genes in IDH1WT GBMs from patients. Additionally, Wnt signaling activation was positively correlated with the degree of immunosuppressive polarization of microglia. Specifically, inhibiting Wnt signaling promoted the transition of microglia to an immunostimulatory phenotype, enhanced cytotoxic CD8+ T cell infiltration and reduced Treg cell numbers. Furthermore, gavage administration of a Wnt signaling inhibitor restrained glioma development and increased the survival of model mice bearing IDH1WT GBM allografts. Overall, the present work indicates that the crosstalk between IDH1WT cancer cells and immunosuppressive microglia plays an important role in maintaining the immunosuppressive microenvironment ().
Figure 7. Wnt/β-catenin signaling blockade activates the immunosuppressive microenvironment of IDH1WT GBM. (a) ① Wnt3a secreted by IDH1WT GBM cells triggers immunosuppressive microglial polarization by activating the Wnt/β-catenin pathway. ② Immunosuppressive microglia subsequently release Wnt3a stimulating tumor growth. ③ The positive feedback between IDH1WT GBM cells and immunosuppressive microglia exacerbates the immunosuppressive microenvironment. (b) Wnt-C59, an inhibitor of Wnt/β-catenin signaling, mitigates Wnt3a release, blocks immunosuppressive microglial polarization, and inhibits the co-stimulation of tumor-supportive microglia and cancer cells, consequently restraining glioma development
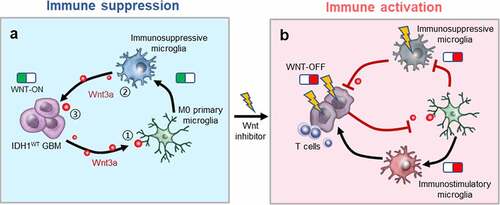
Microglia play a crucial role in regulating the glioma immune microenvironment. First, microglia initiate the immunosuppressive microenvironment in GBM. Glioma cells first secrete various cytokines, such as TGF-β and CCL2, to polarize microglia to the immunosuppressive phenotype.Citation34,Citation35 Tumor-supportive microglia then activate the transformation of astrocytes to an anti-inflammatory A2 phenotypeCitation36 and promote the growth of cancer cells by releasing a plethora of cytokines. The interaction of microglia, astrocytes and cancer cells results in the development of a glioma immunosuppressive environment. Second, microglia amplify the immunosuppressed microenvironment in GBM. Once stimulated by glioma cells, tumor-supportive microglia not only secrete a variety of cytokines, such as VEGF and IL-6, to stimulate GBM growthCitation37 but also accelerate the intracerebral entry of peripheral immunosuppressive cells, such as MDSCs and Treg cells, driving glioma progression.Citation38 Third, microglia modulate the immunosuppressive microenvironment. Xue et al demonstrated that chlorogenic acid effectively reduced glioma growth by skewing microglial polarization from the immunosuppressive to immunostimulatory phenotype by promoting STAT1 signaling and inhibiting STAT6 signaling.Citation39 Chen et al. reported that switching tumor-associated macrophages from an immunosuppressive to an immunostimulatory phenotype decreased the immunosuppressive infiltration of MDSCs and Treg cells.Citation40 Considering the crucial role of microglia in initiating, amplifying and modulating the immunosuppressive microenvironment in IDH1WT GBM,Citation41 the downregulation of immunosuppressive microglia is a promising strategy to ameliorate the hostile immune microenvironment in IDH1WT tumors.
Several approaches have been tested to eliminate immunosuppressive microglia.Citation42 For example, a colony stimulating factor 1 receptor inhibitor was used to ablate microglia.Citation43 However, no obvious therapeutic responses were reported in clinical trials.Citation44 Additionally, completely exhausting microglia in the brain could lead to deadly consequences.Citation45 A promising strategy for inhibiting glioma progression is rebalancing the ratio between immunostimulatory and immunosuppressive microglia. For example, Zhang et al. found that STAT3 inhibition resulted in the repolarization of microglia and abrogated glioma growth.Citation46 Sarkar et al. indicated that amphotericin B shifted microglia toward an immunostimulatory phenotype and reduced the development of glioblastoma stem cells.Citation47 Moreover, Kees et al showed that mediating microglial phenotypic transition led to reduced glioma cell invasiveness.Citation48 Thus, compared to microglial depletion, rebalancing the microglial phenotype is expected to be more effective in ameliorating the immunosuppressive microenvironment in glioma.
Wnt signaling actively regulates cell differentiation, proliferation and tissue homeostasis.Citation14 Abnormal activation of the Wnt/β-catenin pathway has been found in glioma, gastrointestinal cancer and lung cancer.Citation14,Citation49 Blocking Wnt/β-catenin signaling could lead to CD8+ T cell activation,Citation50 trigger macrophage polarizationCitation51,Citation52 and suppress cancer cell proliferation.Citation16,Citation53 The abovementioned observations prompted us to study the effect of Wnt signaling on the communication between IDH1WT cancer cells and immunosuppressive microglia. Our study first demonstrated that Wnt/β-catenin signaling was dramatically activated in both tumor cells and immunosuppressive microglia in IDH1WT GBM patients. Cancer cells trigger the polarization of primary microglia into an immunosuppressive phenotype by overproducing Wnt ligand. Blocking Wnt/β-catenin signaling in immunosuppressive microglia not only accelerated their conversion into an immunostimulatory phenotype but also markedly inhibited IDH1WT GBM cell growth. These findings showed that Wnt signaling in immunosuppressive microglia and IDH1WT cancer cells is crucial for maintaining the immunosuppressive microenvironment in IDH1WT GBM.Citation54 Interestingly, we found the increased cytotoxic CD8+ T cells infiltration in glioma allograft after the treatment of Wnt-C59, while depletion of CD8+ T cells remarkably reduced the therapeutic response of the Wnt inhibitor. The results suggested CD8+ T cells plays a key role in fulfilling the antitumor immunostimulatory responses of the Wnt/β-catenin inhibitor. Notably, our experimental data consisted with previous study that inhibiting Wnt/β-catenin signaling led to CD8+ T cell activation and restrained colorectal tumor growth.Citation55 Future studies will investigate the relationship between CD8+ T cell activation and microglia phenotype transition. Overall, above studies suggest that Wnt signaling is a promising target for IDH1WT glioma therapy.
In conclusion, this work demonstrated that the Wnt/β-catenin pathway was dramatically activated in both tumor cells and immunosuppressive microglia in IDH1WT glioma. Blocking Wnt signaling not only triggered the phenotypic transition of immunosuppressive microglia into an immunostimulatory phenotype but also markedly mitigated IDH1WT GBM cell growth. Importantly, Wnt signaling blockade is a promising strategy to modulate the glioma microenvironment by decreasing the ratio of immunosuppressive/immunostimulatory microglia and increasing T cell-mediated immune responses. This strategy provides an encouraging idea for IDH1WT GBM therapy by stimulating the immune microenvironment, which may improve the efficacies of chemoradiotherapy and immune treatments.
Conflicts of interest
The authors report no competing interests.
Author contributions
DF, QY, CW and CL conceived, designed the study. DF, QY, RY carried out cell biology experiments. JC, CW, DF, ZJ, CP synthesized [18F]DPA-714, performed PET experiments and analyzed the data. DF, JZ performed MRI experiments and analyzed the data. DF, ZJ, SY, QW, LC, XL and ZC carried out the rest of the animal experiments and analyzed the data. DF, RY, QY wrote the original draft. DF, QY, YM, RH, LC and CL reviewed the paper. LC, RH and CL supervised the studies and wrote the paper.
Supplemental Material
Download ()Supplementary material
Supplemental data for this article can be accessed on the publisher’s website.
Additional information
Funding
References
- Ostrom QT, Gittleman H, Fulop J, Liu M, Blanda R, Kromer C, Wolinsky Y, Kruchko C, Barnholtz-Sloan JS. CBTRUS statistical report: primary brain and central nervous system tumors diagnosed in the United States in 2008-2012. Neuro Oncol. 2015;17(Suppl 4):iv1–13. doi:10.1093/neuonc/nov189.
- Louis DN, Perry A, Reifenberger G, Von Deimling A, Figarella-Branger D, Cavenee WK, Ohgaki H, Wiestler OD, Kleihues P, Ellison DW, et al. The 2016 world health organization classification of tumors of the central nervous system: a summary. Acta Neuropathol. 2016;131:803–820. doi:10.1007/s00401-016-1545-1.
- Molinaro AM, Taylor JW, Wiencke JK, Wrensch MR. Genetic and molecular epidemiology of adult diffuse glioma. Nat Rev Neurol. 2019;15:405–417. doi:10.1038/s41582-019-0220-2.
- Khalil DN, Smith EL, Brentjens RJ, Wolchok JD. The future of cancer treatment: immunomodulation, CARs and combination immunotherapy. Nat Rev Clin Oncol. 2016;13:394. doi:10.1038/nrclinonc.2016.65.
- Filley AC, Henriquez M, Dey M. Recurrent glioma clinical trial, CheckMate-143: the game is not over yet. Oncotarget. 2017;8:91779–91794. doi:10.18632/oncotarget.21586.
- Lohr J, Ratliff T, Huppertz A, Ge Y, Dictus C, Ahmadi R, Grau S, Hiraoka N, Eckstein V, Ecker RC, et al. Effector T-cell infiltration positively impacts survival of glioblastoma patients and is impaired by tumor-derived TGF-beta. Clin Cancer Res. 2011;17:4296–4308. doi:10.1158/1078-0432.CCR-10-2557.
- Berghoff AS, Kiesel B, Widhalm G, Wilhelm D, Rajky O, Kurscheid S, Kresl P, Wöhrer A, Marosi C, Hegi ME, et al. Correlation of immune phenotype with IDH mutation in diffuse glioma. Neuro Oncol. 2017;19:1460–1468. doi:10.1093/neuonc/nox054.
- Richardson LG, Nieman LT, Stemmer-Rachamimov AO, Zheng XS, Stafford K, Nagashima H, Miller JJ, Kiyokawa J, Ting DT, Wakimoto H, et al. IDH-mutant gliomas harbor fewer regulatory T cells in humans and mice. Oncoimmunology. 2020;9:1806662. doi:10.1080/2162402X.2020.1806662.
- Amankulor NM, Kim Y, Arora S, Kargl J, Szulzewsky F, Hanke M, Margineantu DH, Rao A, Bolouri H, Delrow J, et al. Mutant IDH1 regulates the tumor-associated immune system in gliomas. Genes Dev. 2017;31:774–786. doi:10.1101/gad.294991.116.
- Molenaar RJ, Maciejewski JP, Wilmink JW, Van Noorden C. Wild-type and mutated IDH1/2 enzymes and therapy responses. Oncogene. 2018;37:1949–1960. doi:10.1038/s41388-017-0077-z.
- Davies LC, Jenkins SJ, Allen JE, Taylor PR. Tissue-resident macrophages. Nat Immunol. 2013;14:986–995. doi:10.1038/ni.2705.
- Ransohoff RM, Engelhardt B. The anatomical and cellular basis of immune surveillance in the central nervous system. Nat Rev Immunol. 2012;12:623–635. doi:10.1038/nri3265.
- Zeiner PS, Preusse C, Blank AE, Zachskorn C, Baumgarten P, Caspary L, Braczynski AK, Weissenberger J, Bratzke H, Reiß S, et al. MIF receptor CD74 is Restricted to microglia/ macrophages,associated with a M1-polarized immune milieu and prolonged patient survival in gliomas. Brain Pathol. 2015;25:491–504. doi:10.1111/bpa.12194.
- Galluzzi L, Spranger S, Fuchs E, López-Soto A. WNT signaling in cancer immunosurveillance. Trends Cell Biol. 2019;29:44–65. doi:10.1016/j.tcb.2018.08.005.
- Ji L, Qian W, Gui L, Ji Z, Yin P, Lin GN, Wang Y, Ma B, Gao WQ. Blockade of beta-Catenin-induced CCL28 suppresses gastric cancer progression via inhibition of treg cell infiltration. Cancer Res. 2004–16;80:2020.
- Spranger S, Bao R, Gajewski TF. Melanoma-intrinsic beta-catenin signalling prevents anti-tumour immunity. Nature. 2015;523:231–235. doi:10.1038/nature14404.
- Cui D, Ren J, Shi J, Feng L, Wang K, Zeng T, Jin Y, Gao L. R132H mutation in IDH1 gene reduces proliferation, cell survival and invasion of human glioma by downregulating Wnt/beta-catenin signaling. Int J Biochem Cell Biol. 2016;73:72–81. doi:10.1016/j.biocel.2016.02.007.
- Yao Q, Cai G, Yu Q, Shen J, Gu Z, Chen J, Shi W, Shi J. IDH1 mutation diminishes aggressive phenotype in glioma stem cells. Int J Oncol. 2018;52:270–278. doi:10.3892/ijo.2017.4186.
- Van Steenwinckel J, Schang A, Krishnan ML, Degos V, Delahaye-Duriez A, Bokobza C, Csaba Z, Verdonk F, Montané A, Sigaut S, et al. Decreased microglial Wnt/β-catenin signalling drives microglial pro-inflammatory activation in the developing brain. Brain. 2019;142:3806–3833. doi:10.1093/brain/awz319.
- Moriguchi T, Kaneumi S, Takeda S, Enomoto K, Mishra SK, Miki T, Koshimizu U, Kitamura H, Kondo T. Ecrg4 contributes to the anti-glioma immunosurveillance through type-I interferon signaling. Oncoimmunology. 2016;5:e1242547. doi:10.1080/2162402X.2016.1242547.
- Barberi T, Martin A, Suresh R, Barakat DJ, Harris-Bookman S, Drake CG, Lim M, Friedman AD. Absence of host NF-kappaB p50 induces murine glioblastoma tumor regression, increases survival, and decreases T-cell induction of tumor-associated macrophage M2 polarization. Cancer Immunol Immunother. 2018;67:1491–1503. doi:10.1007/s00262-018-2184-2.
- Proffitt KD, Madan B, Ke Z, Pendharkar V, Ding L, Lee MA, Hannoush RN, Virshup DM. Pharmacological inhibition of the Wnt acyltransferase PORCN prevents growth of WNT-driven mammary cancer. Cancer Res. 2013;73:502–507. doi:10.1158/0008-5472.CAN-12-2258.
- Cai J, Chen Q, Cui Y, Dong J, Chen M, Wu P, Jiang C. Immune heterogeneity and clinicopathologic characterization of IGFBP2 in 2447 glioma samples. Oncoimmunology. 2018;7(5):e1426516. doi:10.1080/2162402X.2018.1426516.
- Zeiner PS, Preusse C, Golebiewska A, Zinke J, Iriondo A, Muller A, Kaoma T, Filipski K, Müller-Eschner M, Bernatz S, et al. Distribution and prognostic impact of microglia/macrophage subpopulations in gliomas. Brain Pathol. 2019;29(4):513–529. doi:10.1111/bpa.12690.
- Komohara Y, Ohnishi K, Kuratsu J, Takeya M. Possible involvement of the M2 anti-inflammatory macrophage phenotype in growth of human gliomas. J Pathol. 2008;216(1):15–24. doi:10.1002/path.2370.
- Zhou W, Ke SQ, Huang Z, Flavahan W, Fang X, Paul J, Wu L, Sloan AE, McLendon RE, Li X, et al. Periostin secreted by glioblastoma stem cells recruits M2 tumour-associated macrophages and promotes malignant growth. Nat Cell Biol. 2015;17(2):170–182. doi:10.1038/ncb3090.
- Maes W, Van Gool SW. Experimental immunotherapy for malignant glioma: lessons from two decades of research in the GL261 model. Cancer Immunol Immunother. 2011;60:153–160. doi:10.1007/s00262-010-0946-6.
- Qian J, Luo F, Yang J, Liu J, Liu R, Wang L, Wang C, Deng Y, Lu Z, Wang Y, et al. TLR2 promotes glioma immune evasion by downregulating MHC class II molecules in Microglia. Cancer Immunol Res. 2018;6:1220–1233. doi:10.1158/2326-6066.CIR-18-0020.
- Bowman RL, Klemm F, Akkari L, Pyonteck SM, Sevenich L, Quail DF, Dhara S, Simpson K, Gardner EE, Iacobuzio-Donahue CA, et al. Macrophage ontogeny underlies differences in tumor-specific education in brain malignancies. Cell Rep. 2016;17(9):2445–2459. doi:10.1016/j.celrep.2016.10.052.
- Klemm F, Maas RR, Bowman RL, Kornete M, Soukup K, Nassiri S, Brouland J-P, Iacobuzio-Donahue CA, Brennan C, Tabar V, et al. Interrogation of the microenvironmental landscape in brain tumors reveals disease-specific alterations of immune cells. Cell. 2020;181(7):1643–1660.e17. doi:10.1016/j.cell.2020.05.007.
- Bengoa-Vergniory N, Gorrono-Etxebarria I, Gonzalez-Salazar I, Kypta RM. A switch from canonical to noncanonical Wnt signaling mediates early differentiation of human neural stem cells. Stem Cells. 2014;32:3196–3208. doi:10.1002/stem.1807.
- Cosenza-Nashat M, Zhao ML, Suh HS, Morgan J, Natividad R, Morgello S, Lee SC. Expression of the translocator protein of 18 kDa by microglia, macrophages and astrocytes based on immunohistochemical localization in abnormal human brain. Neuropathol Appl Neurobiol. 2009;35:306–328. doi:10.1111/j.1365-2990.2008.01006.x.
- Beckers L, Ory D, Geric I, Declercq L, Koole M, Kassiou M, Bormans G, Baes M. Increased expression of translocator protein (TSPO) marks pro-inflammatory microglia but does not predict neurodegeneration. Mol Imaging Biol. 2018;20:94–102. doi:10.1007/s11307-017-1099-1.
- Hu F, Ku MC, Markovic D, Dzaye O, Lehnardt S, Synowitz M, Wolf SA, Kettenmann H. Glioma-associated microglial MMP9 expression is upregulated by TLR2 signaling and sensitive to minocycline. Int J Cancer. 2014;135:2569–2578. doi:10.1002/ijc.28908.
- Matias D, Predes D, Niemeyer FP, Lopes MC, Abreu JG, Lima F, Moura Neto V. Microglia-glioblastoma interactions: new role for Wnt signaling. Biochim Biophys Acta Rev Cancer. 2017;1868:333–340. doi:10.1016/j.bbcan.2017.05.007.
- Henrik HD, Ravi VM, Behringer SP, Frenking JH, Wurm J, Joseph K, Garrelfs NWC, Strähle J, Heynckes S, Grauvogel J, et al. Tumor-associated reactive astrocytes aid the evolution of immunosuppressive environment in glioblastoma. Nat Commun. 2019;10(1):2541. doi:10.1038/s41467-019-10493-6.
- Li W, Graeber MB. The molecular profile of microglia under the influence of glioma. Neuro Oncol. 2012;14:958–978.
- Chang AL, Miska J, Wainwright DA, Dey M, Rivetta CV, Yu D, Kanojia D, Pituch KC, Qiao J, Pytel P, et al. CCL2 produced by the glioma microenvironment is essential for the recruitment of regulatory T cells and myeloid-derived suppressor cells. Cancer Res. 2016;76(19):5671–5682. doi:10.1158/0008-5472.CAN-16-0144.
- Xue N, Zhou Q, Ji M, Jin J, Lai F, Chen J, Zhang M, Jia J, Yang H, Zhang J, et al. Chlorogenic acid inhibits glioblastoma growth through repolarizating macrophage from M2 to M1 phenotype. Sci Rep. 2017;7(1):39011. doi:10.1038/srep39011.
- Chen D, Xie J, Fiskesund R, Dong W, Liang X, Lv J, Jin X, Liu J, Mo S, Zhang T, et al. Chloroquine modulates antitumor immune response by resetting tumor-associated macrophages toward M1 phenotype. Nat Commun. 2018;9(1):873. doi:10.1038/s41467-018-03225-9.
- Komohara Y, Horlad H, Ohnishi K, Fujiwara Y, Bai B, Nakagawa T, Suzu S, Nakamura H, Kuratsu J-I, Takeya M, et al. Importance of direct macrophage-tumor cell interaction on progression of human glioma. Cancer Sci. 2012;103:2165–2172. doi:10.1111/cas.12015.
- Hodges TR, Ferguson SD, Caruso HG, Kohanbash G, Zhou S, Cloughesy TF, Berger MS, Poste GH, Khasraw M, Ba S, et al. Prioritization schema for immunotherapy clinical trials in glioblastoma. Oncoimmunology. 2016;5(6):e1145332. doi:10.1080/2162402X.2016.1145332.
- Pyonteck SM, Akkari L, Schuhmacher AJ, Bowman RL, Sevenich L, Quail DF, Olson OC, Quick ML, Huse JT, Teijeiro V, et al. CSF-1R inhibition alters macrophage polarization and blocks glioma progression. Nat Med. 2013;19(10):1264–1272. doi:10.1038/nm.3337.
- Butowski N, Colman H, De Groot JF, Omuro AM, Nayak L, Wen PY, Cloughesy TF, Marimuthu A, Haidar S, Perry A. Orally administered colony stimulating factor 1 receptor inhibitor PLX3397 in recurrent glioblastoma: an Ivy foundation early phase clinical trials consortium phase II study. Neuro Oncol. 2016;18(4):557–564. doi:10.1093/neuonc/nov245.
- Kierdorf K, Prinz M. Microglia: same same, but different. J Exp Med. 2019;216:2223–2225. doi:10.1084/jem.20191535.
- Zhang L, Alizadeh D, Van Handel M, Kortylewski M, Yu H, Badie B. Stat3 inhibition activates tumor macrophages and abrogates glioma growth in mice. Glia. 2009;57:1458–1467.
- Sarkar S, Doring A, Zemp FJ, Silva C, Lun X, Wang X, Kelly J, Hader W, Hamilton M, Mercier P, et al. Therapeutic activation of macrophages and microglia to suppress brain tumor-initiating cells. Nat Neurosci. 2014;17(1):46–55. doi:10.1038/nn.3597.
- Kees T, Lohr J, Noack J, Mora R, Gdynia G, Todt G, Ernst A, Radlwimmer B, Falk CS, Herold-Mende C, et al. Microglia isolated from patients with glioma gain antitumor activities on poly (I:C) stimulation. Neuro Oncol. 2012;14(1):64–78. doi:10.1093/neuonc/nor182.
- Lee Y, Lee JK, Ahn SH, Lee J, Nam DH. WNT signaling in glioblastoma and therapeutic opportunities. Lab Invest. 2016;96:137–150. doi:10.1038/labinvest.2015.140.
- Gattinoni L, Zhong XS, Palmer DC, Ji Y, Hinrichs CS, Yu Z, Wrzesinski C, Boni A, Cassard L, Garvin LM, et al. Wnt signaling arrests effector T cell differentiation and generates CD8+ memory stem cells. Nat Med. 2009;15:808–813.
- Zhang D, Lu Z, Man J, Cui K, Fu X, Yu L, Gao Y, Liao L, Xiao Q, Guo R, et al. Wnt-3a alleviates neuroinflammation after ischemic stroke by modulating the responses of microglia/macrophages and astrocytes. Int Immunopharmacol. 2019;75:105760. doi:10.1016/j.intimp.2019.105760.
- Yang Y, Ye YC, Chen Y, Zhao JL, Gao CC, Han H, Liu W-C, Qin H-Y. Crosstalk between hepatic tumor cells and macrophages via Wnt/beta-catenin signaling promotes M2-like macrophage polarization and reinforces tumor malignant behaviors. Cell Death Dis. 2018;9:793. doi:10.1038/s41419-018-0818-0.
- Matias D, Dubois LG, Pontes B, Rosário L, Ferrer VP, Balça-Silva J, Fonseca AC, Macharia LW, Romão L, Chimelli L, et al. GBM-derived Wnt3a induces M2-like phenotype in microglial cells through Wnt/β-catenin signaling. Mol Neurobiol. 2018;56:1517–1530. doi:10.1007/s12035-018-1150-5.
- Li Z, Zhang J, Zheng H, Li C, Xiong J, Wang W, Bao H, Jin H, Liang P, Matias D. Modulating lncRNA SNHG15/CDK6/miR-627 circuit by palbociclib, overcomes temozolomide resistance and reduces M2-polarization of glioma associated microglia in glioblastoma multiforme. J Exp Clin Cancer Res. 2019;38(1):380. doi:10.1186/s13046-019-1371-0.
- Pacella I, Cammarata I, Focaccetti C, Miacci S, Gulino A, Tripodo C, Ravà M, Barnaba V, Piconese S. Wnt3a neutralization enhances T-cell responses through indirect mechanisms and restrains tumor growth. Cancer Immunol Res. 2018;6:953–964. doi:10.1158/2326-6066.CIR-17-0713.