ABSTRACT
Cancer immunotherapies have induced long-lasting responses in cancer patients including those with melanoma and head and neck squamous cell carcinoma (HNSCC). However, the majority of treated patients does not achieve clinical benefit from immunotherapy because of systemic tumor-induced immunosuppression. Monocytic myeloid-derived suppressor cells (M-MDSCs) are implicated as key players in inhibiting anti-tumor immune responses and their frequencies are closely associated with tumor progression. Tumor-derived signals, including signaling via STAT3-COX-2, induce the transformation of monocytic precursors into suppressive M-MDSCs. In a retrospective assessment, we observed that survival of melanoma patients undergoing dendritic cell vaccination was negatively associated with blood M-MDSC levels. Previously, it was shown that platinum-based chemotherapeutics inhibit STAT signaling. Here, we show that cisplatin and oxaliplatin treatment interfere with the development of M-MDSCs, potentially synergizing with cancer immunotherapy. In vitro, subclinical doses of platinum-based drugs prevented the generation of COX-2+ M-MDSCs induced by tumor cells from melanoma patients. This was confirmed in HNSCC patients where intravenous cisplatin treatment drastically lowered M-MDSC frequency while monocyte levels remained stable. In treated patients, expression of COX-2 and arginase-1 in M-MDSCs was significantly decreased after two rounds of cisplatin, indicating inhibition of STAT3 signaling. In line, the capacity of M-MDSCs to inhibit activated T cell responses ex vivo was significantly decreased after patients received cisplatin. These results show that platinum-based chemotherapeutics inhibit the expansion and suppressive activity of M-MDSCs in vitro and in cancer patients. Therefore, platinum-based drugs have the potential to enhance response rates of immunotherapy by overcoming M-MDSC-mediated immunosuppression.
Introduction
Immunotherapy is a promising and proven therapeutic strategy to treat a number of cancer types including melanoma, non-small cell lung cancer (NSCLC) and head and neck squamous cell carcinoma (HNSCC).Citation1 In particular, the administration of immune checkpoint inhibitors against programmed cell death protein 1 (PD-1), PD-1 ligand (PD-L1) and cytotoxic T-lymphocyte associated protein 4 (CTLA-4) has greatly increased the survival of patients with melanoma and lung cancer.Citation2 Unfortunately, the reality is that the majority of cancer patients do not respond to currently available immunotherapies.Citation3 Several mechanisms exploited by advanced tumors to resist against therapeutic immune responses have been identified. These include downregulation of MHC class I molecules, expression of co-inhibitory molecules and recruitment of immunosuppressive cells.Citation4 Monocytic myeloid-derived suppressor cells (M-MDSCs) are immature myeloid cells capable of inhibiting anti-tumor immunity. The frequency of these cells is expanded during cancer progression driven by tumor-derived signals such as GM-CSF, IL-6, and PGE-2.Citation5,Citation6 The ability to suppress T cells is the main defining feature of M-MDSCs and phenotypically they are defined by high expression of CD11b, CD33, and CD14 plus low to no expression of the MHC class II molecule HLA-DR.Citation7,Citation8 M-MDSC levels are elevated in cancer patients and correlate to advanced disease and impaired efficacy of immunotherapies such as immune checkpoint inhibitors, DC vaccination, and adoptive cell transfer therapy.Citation9–12 Therefore, M-MDSCs are a prime target for drugs to overcome immunosuppression in the context of cancer immunotherapy. In this regard, signal transducer and activator of transcription 3 (STAT3) is an attractive target protein for inhibition because its signaling function plays a central role in M-MDSC expansion and suppressive activity.Citation5,Citation13 Direct transcriptional targets of STAT3 are the enzymes NADPH oxidase 2 (NOX2), arginase-1 (ARG-1) and cyclo-oxygenase 2 (COX-2).Citation14–16 These enzymes play key roles in the ability to inhibit T cell function. The first two enzymes function to actively inhibit T cell proliferation and activation, whereas COX-2 produces inflammatory mediator PGE-2 which is involved in inflammation and in development of MDSCs.Citation5,Citation17,Citation18 Previously, it was reported that platinum-based chemotherapeutics such as cisplatin, oxaliplatin, and carboplatin can inhibit STAT signaling by binding to the SH2 domain in STAT proteins.Citation19 This observation was validated in HNSCC patients, where cisplatin-based concomitant chemo-radiotherapy had increased patient survival in STAT3 and STAT6 positive tumors compared to patients with STAT3/6 negative tumors.Citation19 In addition, platinum-based compounds were found to have immunostimulatory effects through the induction of immunogenic cancer cell death, sensitizing tumors to immune attack and promoting effector immune functions.Citation20,Citation21 In particular, the blocking of STAT6-dependent PD-L2 expression in platinum-treated tumor cells and dendritic cells contributed to increased T cell responses.Citation22
Considering the importance of STAT signaling during expansion and activation of M-MDSCs in cancer patients,Citation13 we hypothesized that platinum drugs would modulate these suppressive myeloid cells thereby potentially contributing to the effect of these drugs on immune responses. Here, we report for the first time that platinum drugs specifically inhibit the generation and suppressive activity of human M-MDSCs in vitro and in HNSCC patients. These results support the use of platinum drugs to overcome immunosuppressive mechanisms in cancer patients to increase clinical benefit of cancer immunotherapy.
Material and methods
Healthy donor blood and patient blood
Healthy donor buffy coats were obtained from the Karolinska University Hospital blood center (ethical permit: #20010305,01–50) and Sanquin, Nijmegen, the Netherlands. Blood collection of patients was approved by the local Institutional Review Board (Regional Ethical Review Board in Stockholm #20010305,01–50 and Committee on Research involving Human Subjects Arnhem-Nijmegen #2014-083) and in accordance with the declaration of Helsinki. Written informed consent was obtained from all patients. Peripheral Blood Mononuclear Cells (PBMCs) were isolated from blood by density gradient centrifugation using Ficoll-Paque PLUS (GE Healthcare Life Sciences) or lymphoprep (Axis-Shield).
Figure 1. M-MDSC frequencies are increased in metastatic melanoma patients and associated to short survival after DC vaccination. Metastatic melanoma patient enrolled in clinical trials of DC vaccination were divided into short (<12 months) and long survivors (>24 months) based on overall survival. (a) Quantified frequency of M-MDSCs among freshly isolated total PBMCs in healthy donors (n = 10) and metastatic melanoma patients (n = 28) before DC vaccination. (b) Quantified frequency of M-MDSCs among frozen PBMCs in short (n = 14) and long (n = 8) survivors after DC vaccination. (c-d) Patients were divided into quartiles based on M-MDSCs frequency (low to high) and (e-f) divided into 2 groups based on above and below average M-MDSC frequency (12,5%). Increased M-MDSC frequencies correlated with significantly poor OS and PFS in 22 patients. Mann–Whitney test in panel b, unpaired t-test in panel c and log-rank (Mantel-Cox) test in panel c-f. Mean + SEM in panel a-b
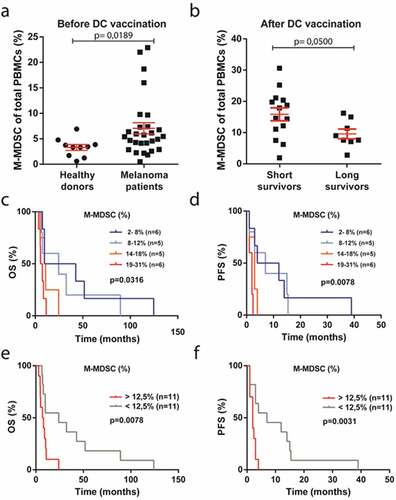
Figure 2. Platinum-based drugs inhibit the development and suppressive activity of melanoma-induced M-MDSCs in vitro.(a) Schematic overview of M-MDSC induction by THFR primary melanoma cells in the presence and absence of platinum drugs followed by autologous T cell co-culture. (b) Percentage of proliferated CD3 + T cell based on CFSE signal after 4 day co-culture with conditioned monocytes (n = 4). (c) Intracellular COX-2 expression by conditioned monocytes was quantified by flow cytometry. Data are depicted as fold increase of COX-2 MFI compared to isotype control (n = 4). One way ANOVA was used to test significance. Mean + SEM in both graphs *** p = <0.001, ** p = <0.01, * p = <0.05
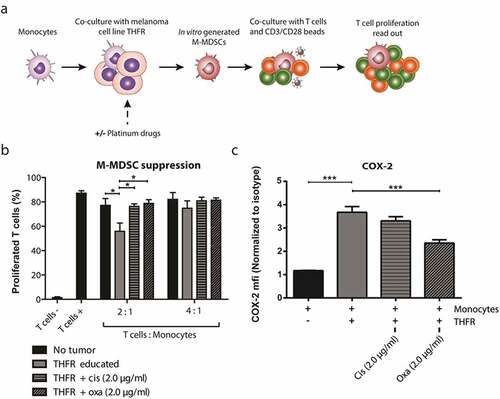
Figure 3. Cisplatin inhibits M-MDSC frequency and expression of STAT3-controlled enzymes in HNSCC patients. (a) Overview of treatment schedule for HNSCC patients undergoing cisplatin-based chemoradiotherapy and timepoints of blood collection for assessment of PBMCs by flow cytometry. Shown are (b) quantified frequency of M-MDSCs (n = 8–19) and (c) quantified frequency of monocytes (n = 8–19) of total viable PBMCs plus (d) a representative plot and quantified COX-2 positivity (n = 3–4) and (e) a representative plot and quantified ARG-1 positivity (n = 3–5) both within M-MDSCs and monocytes. One way ANOVA was used to test significance. Mean + SD in all graphs ****p = <0.0001 *** p = <0.001, ** p = <0.01, * p = <0.05, ns = non-significant
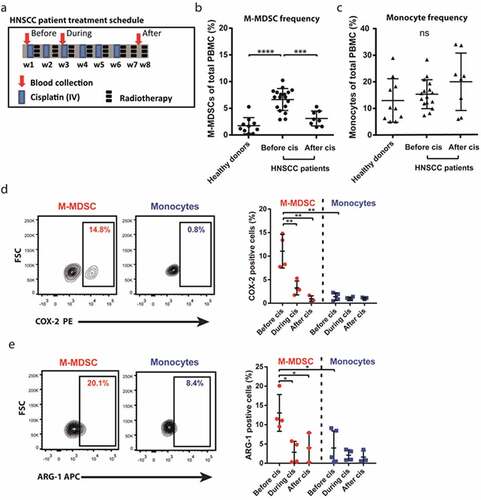
Metastatic melanoma patients
Frozen PBMC fractions of metastatic melanoma patients were collected during clinical trials of DC cancer vaccination (). Patients were categorized as short or long survivors based on overall survival of <12 months or >24 months after start of DC vaccination, respectively. PBMCs were thawed and stained with viability dye eFluor® 780 followed by an antibody panel (Sup. ). Samples were acquired on CyAn ADP analyzer using Summit Software (both Beckman Coulter) and analyzed with FlowJo software (TreeStar V10).
Table 1. Metastatic melanoma patients characteristics
Figure 4. Cisplatin inhibits suppressive activity of M-MDSCs in HNSCC patients. (a) Overview of treatment schedule for HNSCC patients undergoing cisplatin-based chemoradiotherapy and timepoints of blood collection for assessment of suppressive capacity by M-MDSCs. Subpanel indicates two experimental setups of suppression assays performed at each time point. (b) Absolute proliferation of activated T cells in counts per minute after 3 days of culture followed by 16 h thymidine incorporation. T cells from a paired HD were thawed and stimulated for a suppression assay at three timepoints for each patient isolation (in panel b) ensuring similar T cell responses. (c) Proliferation of activated HD T cells was measured by thymidine incorporation after 3 day co-culture with M-MDSCs isolated from patients before, during and after intravenous cisplatin treatment. Proliferation was normalized to monocultured activated HD T cells at every timepoint. (d) Absolute proliferation of activated T cells in counts per minute after 3 days of culture followed by 16 h thymidine incorporation. Autologous T cells were isolated in parallel with M-MDSCs for a suppression assay at three timepoints for each patient (in panel e) simulating in vivo T cell responses. (e) Proliferation of activated autologous T cells was measured by thymidine incorporation after 3 day co-culture with M-MDSCs isolated from patients before, during and after intravenous cisplatin treatment. Proliferation was normalized to monocultured activated autologous T cells at every timepoint. (f) Secreted IFNγ after 3 day co-culture of activated HD T cells and M-MDSCs (from panel C). (g) Secreted IFNγ after 3 day co-culture of activated autologous T cells and M-MDSCs (from panel E). One way ANOVA was used to test significance. Mean + SD in all graphs. ****p = <0.0001 *** p = <0.001, ** p = <0.01, * p = <0.05, ns = non-significant, na = not available, nd = not detected, value below detection limit ELISA, nd* = IFNγ could not be detected in any sample including monocultured activated T cells
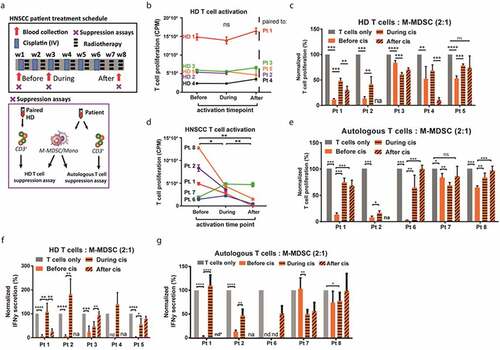
Melanoma-induced in vitro M-MDSCs
The melanoma cell line THFR was established from a metastatic lesion of a patient at the oncology clinic of the Karolinska University Hospital, as approved by the local Ethics Committee and after signing a written informed consent in accordance with the Declaration of Helsinki. To exclude the influence of long-term in vitro culture only fourth passage melanoma cells were used. Cells were thawed to be grown confluent in T75 flasks (Corning Life Sciences) containing IMDM medium (Gibco) + 10% heat-inactivated fetal bovine serum (FBS). Upon harvest cells were trypsinized and used for tumor-monocyte co-cultures. Monocytes were purified from healthy donor PBMCs using CD14 microbeads and LS columns (Miltenyi Biotec) and subjected to the melanoma-based M-MDSC induction protocol as previously published.Citation23 In brief, 4×105 THFR melanoma cells were seeded in a 6-well plate and 106 CD14+ monocytes were added in a total volume of 3 ml IMDM medium + 10% human serum (HS). Monocytes cultured without tumor cells were used as control cells. CD14-PBMCs were cultured using the same medium in a separate 6-well plate in a density of 5×106 cells per ml. After 3 days, co-cultured monocytes (in vitro M-MDSCs) and control monocytes were both harvested and purified from the tumor cells using HLA-DR microbeads (Miltenyi Biotec) according to manufacturer’s instructions. Autologous T cells were purified from the CD14-PBMCs using the Pan T cell Isolation Kit (Miltenyi Biotec) according to manufacturer’s instructions. To evaluate the effects of platinum-based drugs on M-MDSC induction by melanoma cells, subclinical dosages (concentrations indicated in figures) of oxaliplatin (Fresenius Kabi) or cisplatin (Teva Pharmaceuticals) were added to the tumor-monocyte co-cultures. Suppression assays with purified monocytes were performed as described below.
Cell isolation from HNSCC patients
Nineteen HNSCC patients with lymph node metastases, but without distant metastases, were included. Treatment consisted of concomitant chemo-radiotherapy with weekly cisplatin (40 mg/m2) for 6 weeks in the Radboudumc, Nijmegen (). Per patient, blood was collected at three time points being before the first round of treatment (before cisplatin), before the third round of treatment (during cisplatin), and two weeks after the 6th round of cisplatin (after cisplatin). Isolated PBMCs were stored overnight at 4°C. M-MDSCs from nine HNSCC patients were assessed in ex vivo suppression assays (). Therefore, T cells were isolated using the Pan T cell Isolation Kit and an LS column (Miltenyi Biotec) according to manufacturer’s instructions. The T cell negative fraction was stained with antibodies against CD14 and HLA-DR (Sup. ) in PBS with 0,1% bovine serum albumin (BSA) and 0,4% ethylenediaminetetraacetic acid (EDTA) for 30 minutes. CD14+ HLA-DRneg/low (M-MDSCs) were sorted with a FACSAria (BD Biosciences) using BD FACSDiva software.
Table 2. HNSCC patient characteristics (flow cytometry)
Table 3. HNSCC patient characteristics (functional assays)
T cell suppression assays
To assess the suppressive activity of melanoma-induced M-MDSCs, 105 autologous T cells, labeled with 1.4 µM carboxyfluorescein succinimidyl ester (CFSE; Biolegend), were co-cultured with M-MDSCs in ratios 1:2 and 1:4 (M-MDSCs: T cells) in a U-bottom 96-wells plate in IMDM medium supplemented with 10% HS. T cells were activated with 1 µl (4×104) beads CD3/CD28 Dynabeads® (Gibco) per 105 T cells in a total volume of 200 µl. After 4 days of incubation, cells were harvested and analyzed by flow cytometry as described below.
To assess the suppressive activity of M-MDSCs isolated from HNSCC patients undergoing cisplatin-based concomitant chemo-radiotherapy, two assay setups were performed defined by the origin of T cells (). In both setups 2.5×104 T cells were co-cultured with sorted M-MDSCs in ratios of 1:2 or 1:4 (M-MDSC: T cells) in X–VIVO 15 (Lonza) + 2% human serum (Sigma) in 96-well u-bottom plates in 3–6 technical replicates. T cells were activated with 5×103 CD3/CD28 Dynabeads® per 2.5×104 T cells (1 bead: 5 T cells). After 3 days, proliferation of T cells was assessed by incubating cells with 3 H-thymidine for 16 hours and incorporation was measured with a β-counter. 75 µl of supernatant was stored at −20°C for determining IFNγ content by ELISA.
Flow cytometry
To determine the phenotype of melanoma-induced M-MDSCs compared to control cells, monocytes were stained with LIVE/DEAD™ Fixable Near-IR Dead Cell Stain Kit (Thermo Fisher Scientific) followed by a panel of antibodies (Sup. ). Intracellular staining was performed to quantify the expression of COX-2 in melanoma-induced M-MDSCs compared to control monocytes. Cells were first stained for viability with LIVE/DEAD™ Fixable Near-IR Dead Cell Stain Kit (Thermo Fisher Scientific), then fixed with BD Cytofix fixation buffer and subsequently permeabilized using BD Phosflow Perm Buffer III (BD Biosciences) according to manufacturer’s instructions, before staining with COX2-PE antibody (Santa Cruz Biotechnology). Additionally, T cell proliferation after co-culture with melanoma-induced M-MDSCs was measured by flow cytometry based on CFSE intensities 7-AAD Viability Staining Solution (BioLegend) was used to exclude dead cells and CD3 was used to gate on T cells (Sup. ).
PBMC fractions of healthy donors and HNSCC patients were blocked with PBA + 2% HS, stained with viability dye eFluor®506 (eBioscience) followed by separate antibody panels (Sup. ). For intracellular staining cells were permeabilized using Fixation/Permeabilization Solution Kit (BD Biosciences) before staining for intracellular markers. Samples were acquired on a LSR II flow cytometer or FACSVerse (BD Biosciences) and analyzed with FlowJo software (Treestar V10).
Enzyme-linked immunosorbent assay for IFNγ secretion
Supernatant of suppression assays were taken after 3 days of co-culture and IFNγ content was quantified by a sandwich ELISA using the IFNγ human uncoated ELISA kit (Invitrogen) according to manufacturer’s instructions. Absorption was measured using an iMark Microplate Reader (Bio-Rad).
Statistical analyses
Data were analyzed using Prism software version 5.0 (GraphPad Software). Significant differences between two means were analyzed by two-tailed student’s t-tests or Mann–Whitney test in case of unequally distributed populations. Differences between three or more means were tested by one way-ANOVA. Error bars and sample sizes are stated in the figure legends. Overall survival (OS) was calculated from the time of diagnosis to death from any cause or last follow-up. Progression-free survival (PFS) was calculated from the time of diagnosis to disease progression, relapse, or death from any cause. Patients who remained alive or progression free were censored at last follow-up. Survival analysis was performed using the Kaplan–Meier method, and differences were compared using the log-rank (Mantel-Cox) test.
Compliance with ethical standards
Ethical approval: All procedures performed in studies involving human participants were in accordance with the ethical standards of the institutional research committees (Regional Ethical Review Board in Stockholm and Committee on Research involving Human Subjects Arnhem-Nijmegen) and with the 1964 Helsinki declaration and its later amendments or comparable ethical standards.
Informed consent: Informed consent was obtained from all individual participants included in the study.
Results
M-MDSC frequency is associated with short survival in metastatic melanoma patients after DC vaccination
To evaluate the relevance of M-MDSCs in the context of cancer immunotherapy, we assessed melanoma patients enrolled in clinical trials for DC vaccination to treat advanced stage melanoma (). M-MDSC frequencies were quantified by flow cytometry in blood samples from patients and healthy donors (Sup. ). Before DC vaccination metastatic melanoma patients had increased levels of M-MDSCs among freshly isolated PBMCs (mean 7%) as compared to healthy donors (mean 3%), in line with previous studiesCitation9,Citation24,Citation25 (). Next, we retrospectively divided patients after DC vaccination into short survivors (n = 14) and long survivors (n = 8) based on overall survival (OS) (less than 12 months versus more than 24 months) and thawed patient PBMCs for analysis. Although freezing and thawing of PBMCs led to an increase in absolute M-MDSC levels (Sup. ), we observed after DC vaccination, that M-MDSCs were more frequent in short survivors (16%) relative to long survivors (10%) (). In line, when the OS and progression-free survival (PFS) of these 22 enrolled patients were stratified by M-MDSC frequency there was a significant correlation between increased M-MDSC frequencies and poor survival after DC vaccination (–f). These observations suggest that M-MDSCs counteract DC-induced anti-tumor immune responses thereby impairing clinical benefit of immunotherapy for patients. Overall, these data show that M-MDSCs are expanded in metastatic melanoma patients and associated with short survival after immunotherapy.
Platinum-based drugs inhibit the development and suppressive activity of melanoma-induced M-MDSCs in vitro
Previously, the role of melanoma tumor cells in generating M-MDSCs was demonstrated by co-culturing healthy donor monocytes with a melanoma patient-derived tumor cell line, THFR.Citation23 Here, we investigated the effect of platinum-based drugs on the development of melanoma-induced M-MDSCs in vitro (, Sup. ). Cisplatin and oxaliplatin were added during the co-culture of monocytes and tumor cells in a dose that is well below the maximum concentration observed in patients after intravenous administration (Cmax: 5 µg/ml, both).Citation26,Citation27 These subclinical doses of platinum drugs did not affect the viability of monocytes and melanoma cells in the co-cultures (Sup. ). Subsequently, monocytes were harvested and co-cultured with CD3/CD28 stimulated autologous T cells in a 2:1 or 4:1 ratio (T cell: Monocyte) for 4 days to assess their suppressive capacity. At a 2:1 ratio, monocytes co-cultured with melanoma cell-line THFR were able to significantly suppress T cell proliferation compared to mono-cultured control monocytes, indicating successful induction of M-MDSCs in vitro by the melanoma cell-line THFR (). Treatment of monocytes with low-dose cisplatin and oxaliplatin during the co-culture with melanoma cell-line THFR completely abrogated the suppressive activity of in vitro generated M-MDSCs to the level of control monocytes. Melanoma-induced M-MDSCs did not significantly inhibit the proliferation of autologous T cells in a 4:1 ratio, hence effects of platinum drugs could not be measured. It was previously shown that the development and suppressive activity of melanoma-induced M-MDSCs is dependent on a positive feedback loop of STAT3-COX-2 signaling.Citation23 In brief, melanoma-derived PGE-2 induces STAT3 signaling in monocytes that activates the expression of suppressive machinery and COX-2, which in turn leads to more PGE-2 production. To explore whether STAT3 inhibition by platinum-based drugs could explain their inhibitory effect on M-MDSCs in vitro, intracellular COX-2 expression was measured in melanoma-induced M-MDSCs. Indeed, the acquired suppressive capacity of these M-MDSCs coincided with an increased expression of intracellular COX-2 as compared to control monocytes, indicative of active STAT3 signaling (). In addition, treatment of monocytes with oxaliplatin during melanoma co-culture significantly reduced COX-2 expression suggesting inhibition of STAT3 signaling. Taken together, our observations show that subclinical doses of cisplatin and oxaliplatin prevent the transformation of monocytes into melanoma-induced M-MDSCs in vitro.
Cisplatin diminishes M-MDSC frequency and lowers the expression of STAT3-controlled enzymes in M-MDSCs in HNSCC patients
Melanoma patients are not treated with platinum-based chemotherapy; therefore, we selected patients with HNSCC to investigate the ability of platinum-based drugs to deplete M-MDSC numbers in vivo. To this end, blood from 19 HNSCC patients undergoing chemo-radiotherapy with weekly cisplatin as the sole chemotherapeutic agent was collected (). illustrates the treatment schedule according to which blood samples from patients were collected. Blood was taken at three time points per patient: Before start, during week 3 (prior to third administration) and 2 weeks after six rounds of cisplatin administration. Of note, not all time points could be collected for each patient. The frequency of M-MDSCs and monocytes of PBMCs isolated from these HNSCC patients and 10 healthy donors was determined by flow cytometry (Sup. ). There was an increased frequency of M-MDSCs in patients before treatment as compared to healthy donor PBMCs (mean 8% vs 2%) (). Interestingly, this M-MDSC frequency was drastically lowered in HNSCC patients after receiving six rounds of cisplatin treatment (mean 4%). In contrast, monocyte frequencies were similar between untreated HNSCC patients and healthy donors (mean 12% vs 14%) and did not significantly change after cisplatin treatment (mean 20%) (). These observations indicate that cisplatin treatment specifically impairs M-MDSC survival or the development of new M-MDSCs. To investigate whether STAT3-COX-2-dependent induction of M-MDSCs was inhibited by cisplatin, intracellular COX-2 expression was measured in M-MDSCs and monocytes from 4 HNSCC patients. COX-2 expression was highest in M-MDSC compared to monocytes before treatment (mean 11% vs 1% positive), suggestive of increased STAT3 signaling (). Strikingly, COX-2 expression in M-MDSCs was significantly decreased during treatment after two rounds of cisplatin (3% positive) and after six rounds of cisplatin (1% positive). In addition, intracellular expression of a second STAT3-controlled enzyme, ARG-1, followed the same pattern as COX-2 in M-MDSCs and monocytes during treatment. ARG-1 expression was highest in M-MDSCs before treatment as compared to monocytes (13% vs 4% positive) and significantly decreased in M-MDSCs during and after cisplatin treatment (3% and 4% positive) (). Collectively, our data show that cisplatin treatment of HNSCC patients specifically depletes M-MDSCs, but not monocytes, which could be explained by the inhibition of STAT3 signaling as indirectly evidenced by decreased expression of COX-2 and ARG-1 in platinum-treated M-MDSCs in vivo.
Cisplatin inhibits the suppressive activity of M-MDSCs in HNSCC patients
Next, we examined the suppressive capacity of M-MDSCs isolated from HNSCC patients undergoing cisplatin-based chemotherapy with concomitant radiotherapy (, Sup. ). M-MDSCs were FACS-sorted from 5 HNSCC patients at three time points during cisplatin treatment and co-cultured with stimulated T cells from a healthy donor (HD) (). To minimize donor–donor variation, the same combination of patient-donor (M-MDSC-T cell) was used at each time point. This HD T cell suppression assay was set up to observe effects of cisplatin-treated M-MDSCs on T cells, not affected by tumor-induced or therapy-induced alterations in T cells. In line, the amount of proliferation by CD3/CD28 stimulated T cells derived from the paired HD was similar at every time point when M-MDSCs were isolated from the patient (). Before cisplatin treatment, M-MDSCs from all five patients had the capacity to inhibit stimulated HD T cells in a 2:1 ratio (T cell: M-MDSC) ex vivo (). Decreased to no suppressive activity by isolated M-MDSCs was observed in three out of five patients after two rounds of cisplatin and in two out of four patients after six rounds of cisplatin. Similar results were seen for 4:1 ratio (T cell: M-MDSC) co-cultures (Sup. ). Next, M-MDSCs were FACS-sorted from 5 HNSCC patients and co-cultured with stimulated autologous T cells that were isolated in parallel with M-MDSCs from the patient at the 3 time points (). This autologous T cell suppression assay was setup to be more clinically relevant by assessing whether the effect of cisplatin-treated M-MDSCs toward T cells is conserved in the context of chemotherapy and active disease. Notably, the amount of T cell proliferation induced by CD3/CD28 stimulation was significantly decreased after two and six rounds of cisplatin illustrating cisplatin-induced inhibition of T cell responses (, Sup. ). M-MDSCs co-cultured with autologous stimulated T cells in a 2:1 ratio (T cell: M-MDSC) were suppressive in all five patients (). In addition, M-MDSCs were significantly less or non-suppressive when isolated after two rounds of cisplatin in four out of five patients and after six rounds of cisplatin in four out of four patients. A similar pattern was observed in 4:1 ratio co-cultures (Sup. ) and when untreated autologous T cells (before cisplatin) were used at every time point (Sup. ). Whether the rescue of T cell proliferation by cisplatin-treated M-MDSCs corresponds with improved T cell activation was assessed by measuring IFNγ secretion in the T cell – M-MDSC co-cultures. In line with the proliferation data, IFNγ secretion by both HD and autologous T cells was suppressed by M-MDSC ex vivo before cisplatin treatment and increased after treatment in 4 out of 5 patients (). Overall, cisplatin treatment significantly impaired the suppressive capacity of M-MDSCs as evidenced by the rescue of proliferation and activation of healthy donor and autologous T cells after ex vivo co-culture in 60–80% of treated HNSCC-patients.
Discussion
In this study, we investigated the effects of platinum-based chemotherapeutics on the development and suppressive capacity of M-MDSCs in vitro and in cancer patients. First, we observed that M-MDSCs frequency in metastatic melanoma patients was associated with short survival after DC vaccination, which was previously also shown for patients treated with adoptive cell therapy and immune checkpoint inhibitors.Citation9,Citation11,Citation12,Citation28 Subsequently, we made use of a clinically relevant in vitro model that differentiates monocytes into suppressive cells that closely resemble human M-MDSCs through co-culture with a patient-derived melanoma cell line.Citation23,Citation29 We showed that treatment of monocytes with non-cytotoxic doses of cisplatin and oxaliplatin during these co-cultures completely prevented the development of suppressive melanoma-induced M-MDSCs. This is in line with a recent study performed in a murine colon cancer model, where in vitro treatment with non-cytotoxic doses of oxaliplatin impaired the suppressive activity of splenic M-MDSCs.Citation30 We found that the diminished suppressive activity in oxaliplatin-treated M-MDSCs coincided with decreased STAT3-COX2 signaling as indicated by impaired COX-2 expression. However, cisplatin also blocked suppressive function but did not clearly lower COX-2 expression in melanoma-induced M-MDSCs in vitro as it did in M-MDSCs in vivo. This suggests that M-MDSC-targeting effects by platinum compounds are not limited to STAT3-COX-2 inhibition but likely also affects other pathways through which M-MDSCs exert their suppressive functions, such as production of NO.Citation7 In this regard, platinum drugs have different effects on multiple STAT-regulated pathways including STAT1, STAT5, or STAT6, which are also involved in M-MDSC suppressive function.Citation13 Future studies are needed to establish which additional signaling pathways are inhibited by platinum drugs in human M-MDSCs and how these contribute to the impaired immunosuppressive function.
To validate the effect of platinum-based chemotherapeutics on M-MDSCs in vivo, we identified that patients with HNSCC are the most suitable patient population because these are, unlike most tumor types, treated with platinum drugs as single chemotherapeutic modality. M-MDSC frequency and suppressive function were determined in blood samples of patients undergoing cisplatin-based chemo-radiotherapy. We showed for the first time that chemo-radiotherapy with cisplatin as the sole chemotherapeutic agent reduces the frequency and suppressive activity of M-MDSCs in cancer patients. So far, in mice both MDSC-depleting and -promoting effects of platinum drugs have been described. This discrepancy is likely due to differences in drug concentrations, treatment intervals, MDSC localization, timing of MDSC sampling and tumor models.Citation31 Nevertheless, studies that examined the functionality of MDSCs showed that a single dose of 7.5 mg/kg cisplatin inhibited the frequencies and suppressive activity of intratumoral and splenic MDSCs, but not peripheral blood-borne MDSCs from B16 melanoma-bearing mice.Citation32 A second study, using a murine CT26 colon cancer model, demonstrated that one intraperitoneal injection of 10 mg/kg oxaliplatin depleted MDSCs and in particular M-MDSCs in the spleen but not in the tumor. These studies suggest that assessment 2–3 days after platinum treatment is too early to detect systemic changes in the myeloid compartment or that single doses are insufficient in inducing these systemic effects.
In our study, cisplatin-treated patients underwent a 6 week course of radiotherapy concomitant to the chemotherapy. In this regard, it was previously shown that a direct immunological effect on myeloid cells by radiotherapy is unlikely as evidenced by unchanged STAT phosphorylation in monocyte-derived dendritic cells after in vitro irradiation.Citation22 Moreover, the patients were given a single dose of 8 mg dexamethasone for anti-emetic purpose prior to each cisplatin administration in order to tolerate clinical doses. As dexamethasone is also widely used as immunosuppressant this has been a relevant concern in recent chemo-immunotherapy studies. There, it was observed that anti-emetic doses of dexamethasone did not constrain immune responses in mice and in melanoma patients, including anti-tumor immunity induced by immunotherapy.Citation33–35 In accordance, we found that the frequency of monocytes in cisplatin-treated patients remained unaltered, which argues in favor of minimal immunological interference by dexamethasone in the monocytic compartment.
Our in vitro observations clearly demonstrate an immunostimulatory effect of platinum drugs via the direct inhibition of M-MDSCs. This was complemented by the drastically decreased expression of COX-2 and ARG1 observed in M-MDSCs in vivo after only two rounds of cisplatin treatment, indicative of a direct effect on STAT3 signaling. Furthermore, in vivo, M-MDSCs are possibly affected by additional indirect effects of platinum drugs. For example, reduced tumor burden as result of the platinum-based chemo-radiotherapy could indirectly contribute to M-MDSC depletion by affecting tumor-derived signals in patients.Citation28 This supports the administration of platinum drugs to overcome M-MDSC-mediated resistance to immunotherapy in order to improve clinical benefit for patients.
A recent clinical study of HPV+ oropharyngeal cancer patients undergoing radical therapy consisting of mixed strategies including platinum-based chemo-radiotherapy demonstrated that patients had decreased HPV-specific T cell responses 6 to 16 weeks post-treatment.Citation36 This suggests that the potential to elicit anti-tumor immunity in HNSCC patients after such a period post-radical therapy is unfavorable for immunotherapies. The importance of timing when combining chemo- with immunotherapy was also evidenced in a recent clinical trial where administering cisplatin 1–2 hours prior to DC-based cancer vaccines had no added benefit over DC vaccination alone in melanoma patients.Citation33 However, when an HPV cancer vaccine was administered 2 weeks after the second round of carboplatin treatment combined with alkaloid chemotherapeutic paclitaxel, it resulted in unusually strong T cell responses in cervical cancer patients, which remained during the course of the chemotherapy.Citation35 It was shown that the cancer-induced expansion of the myeloid compartment including MDSC frequencies were normalized during this window starting between the second and third round of chemotherapy. In line, our observations also show that the suppressive activity of M-MDSC in HNSCC patients was decreased between the second and third round of cisplatin treatment and that this effect lasted until at least 2 weeks after the sixth round. Combined, these data emphasize the contribution of M-MDSC-mediated immunosuppression to the refractoriness of immunotherapy and the potential that platinum-based chemotherapy has to overcome this obstruction. Whether two rounds of chemotherapy prior to immunotherapy is sufficient to achieve optimal T cell responses or what time point in the now proposed window is most effective to start immunotherapeutic intervention still needs to be investigated. Lastly, our in vitro data suggest that M-MDSC-targeting effects by cisplatin and oxaliplatin could already be achieved by sub-clinical doses in patients. This could potentially translate into lowering chemotherapeutic doses strictly needed for combined chemo-immunotherapy, which in turn could alleviate therapy burden for cancer patients.
In conclusion, we have shown that platinum-based drugs impair the suppressive activity of M-MDSCs both in vitro and in cancer patients, likely by the inhibition of STAT3 and its downstream targets including COX-2 and ARG-1. Due to the broad relevance of M-MDSCs in cancer these novel findings support the application of platinum-based combined chemo-immunotherapeutic strategies across several tumor types. Additional studies will have to demonstrate whether the combination of platinum drugs and properly timed immunotherapy results in added clinical benefit.
Author contributions
G.F.W., N.H., T.A.M., Y.M., S.V.H. and I.J.M.V. designed and performed experiments and analyzed the data. M.B. and S.HB. provided clinical patient information and contributed to data interpretation. G.F.W. and N.H. were responsible for writing the manuscript. R.K.´s laboratory provided the model for in vitro induction of MDSCs and established the melanoma tumor line. S.K.H., R.K., C.M.L.H., G.FG., S.V.H., and I.J.M.V substantially revised the manuscript.
Supplemental Material
Download ()Acknowledgments
The authors would like to thank Rob Woestenenk and the Radboud Technology Center Flow Cytometry for their assistance in FACS sorting and Michiel Kerkkamp for patient inclusion and patient care. Nicole Scharenborg, Annemiek de Boer, Tjitske Duiveman, Tom van Oorschot, and Anouk Becker are acknowledged for their assistance.
Disclosure statement
The authors declare that they have no conflict of interest.
Supplementary material
Supplemental data for this article can be accessed on the publisher’s website
Additional information
Funding
References
- Waldman AD, Fritz JM, Lenardo MJ. A guide to cancer immunotherapy: from T cell basic science to clinical practice. Nat Rev Immunol. 2020;20:651–12.
- Robert C. A decade of immune-checkpoint inhibitors in cancer therapy. Nat Commun. 2020;11(1). doi:10.1038/s41467-020-17670-y.
- Luke JJ, Flaherty KT, Ribas A, Long GV. Targeted agents and immunotherapies: optimizing outcomes in melanoma. Nat Rev Clin Oncol. 2017;14(8):463–482. doi:10.1038/nrclinonc.2017.43.
- Van Der Burg SH, Arens R, Ossendorp F, Van Hall T, Melief CJM. Vaccines for established cancer: overcoming the challenges posed by immune evasion. Nat Rev Cancer. 2016;16(4):219–233. doi:10.1038/nrc.2016.16.
- Weber R, Riester Z, Hüser L, Sticht C, Siebenmorgen A, Groth C, Hu X, Altevogt P, Utikal JS, Umansky V. IL-6 regulates CCR5 expression and immunosuppressive capacity of MDSC in murine melanoma. J Immunother Cancer. 2020;8(2):e000949. doi:10.1136/jitc-2020-000949.
- Porta C, Consonni FM, Morlacchi S, Sangaletti S, Bleve A, Totaro MG, Larghi P, Rimoldi M, Tripodo C, Strauss L, et al. Tumor-derived prostaglandin E2 promotes p50 NF-kB-dependent differentiation of monocytic MDSCs. Cancer Res. 2020;80(13):2874–2888. doi:10.1158/0008-5472.CAN-19-2843.
- Bronte V, Brandau S, Chen SH, Colombo MP, Frey AB, Greten TF, Mandruzzato S, Murray PJ, Ochoa A, Ostrand-Rosenberg S, et al. Recommendations for myeloid-derived suppressor cell nomenclature and characterization standards. Nat Commun. 2016;7(12150). doi:10.1038/ncomms12150.
- Veglia F, Sanseviero E, Gabrilovich DI. Myeloid-derived suppressor cells in the era of increasing myeloid cell diversity. Nat Rev Immunol. 2021. doi:10.1038/s41577-020-00490-y.
- Meyer C, Cagnon L, Costa-Nunes CM, Baumgaertner P, Montandon N, Leyvraz L, Michielin O, Romano E, Speiser DE. Frequencies of circulating MDSC correlate with clinical outcome of melanoma patients treated with ipilimumab. Cancer Immunol Immunother. 2014;63(3):247–257. doi:10.1007/s00262-013-1508-5.
- Chesney JA, Mitchell RA, Yaddanapudi K. Myeloid‐derived suppressor cells—a new therapeutic target to overcome resistance to cancer immunotherapy. J Leukoc Biol. 2017;102(3):727–740. doi:10.1189/jlb.5VMR1116-458RRR.
- Krieg C, Nowicka M, Guglietta S, Schindler S, Hartmann FJ, Weber LM, Dummer R, Robinson MD, Levesque MP, Becher B. High-dimensional single-cell analysis predicts response to anti-PD-1 immunotherapy. Nat Med. 2018;24(2):144–153. doi:10.1038/nm.4466.
- Pico de Coaña Y, Wolodarski M, Van Der Haar Àvila I, Nakajima T, Rentouli S, Lundqvist A, Masucci G, Hansson J, Kiessling R. PD-1 checkpoint blockade in advanced melanoma patients: NK cells, monocytic subsets and host PD-L1 expression as predictive biomarker candidates. Oncoimmunology. 2020;9(1):1786888. doi:10.1080/2162402X.2020.1786888.
- De Haas N, de Koning C, Spilgies L, De Vries IJM, Hato SV. Improving cancer immunotherapy by targeting the STATe of MDSCs. Oncoimmunology. 2016;5(7):e1196312. doi:10.1080/2162402X.2016.1196312.
- Corzo CA, Cotter MJ, Cheng P, Cheng F, Kusmartsev S, Sotomayor E, Padhya T, McCaffrey TV, McCaffrey JC, Gabrilovich DI. Mechanism regulating reactive oxygen species in tumor-induced myeloid-derived suppressor cells. J Immunol. 2009;182(9):5693–5701. doi:10.4049/jimmunol.0900092.
- Vasquez-Dunddel D, Pan F, Zeng Q, Gorbounov M, Albesiano E, Fu J, Blosser RL, Tam AJ, Bruno T, Zhang H, et al. STAT3 regulates arginase-i in myeloid-derived suppressor cells from cancer patients. J Clin Invest. 2013;123(4):1580–1589. doi:10.1172/JCI60083.
- Lo HW, Cao X, Zhu H, Ali-Osman F. Cyclooxygenase-2 is a novel transcriptional target of the nuclear EGFR-STAT3 and EGFRvIII-STAT3 signaling axes. Mol Cancer Res. 2010;8(2):232–245. doi:10.1158/1541-7786.MCR-09-0391.
- Mao Y, Sarhan D, Steven A, Seliger B, Kiessling R, Lundqvist A. Inhibition of tumor-derived prostaglandin-E2 blocks the induction of myeloid-derived suppressor cells and recovers natural killer cell activity. Clin Cancer Res. 2014;20(15):4096–4106. doi:10.1158/1078-0432.CCR-14-0635.
- Beury DW, Carter KA, Nelson C, Sinha P, Hanson E, Nyandjo M, Fitzgerald PJ, Majeed A, Wali N, Ostrand-Rosenberg S. Myeloid-derived suppressor cell survival and function are regulated by the transcription factor Nrf2. J Immunol. 2016;196(8):3470–3478. doi:10.4049/jimmunol.1501785.
- Hato SV, Figdor CG, Takahashi S, Pen AE, Halilovic A, Bol KF, Vasaturo A, Inoue Y, De Haas N, Verweij D, et al. Direct inhibition of STAT signaling by platinum drugs contributes to their anti-cancer activity. Oncotarget. 2017;8(33):54434–54443. doi:10.18632/oncotarget.17661.
- Hato SV, Khong A, De Vries IJM, Lesterhuis WJ. Molecular pathways: the immunogenic effects of platinum-based chemotherapeutics. Clin Cancer Res. 2014;20:2831–2837. doi:10.1158/1078-0432.CCR-13-3141.
- De Biasi AR, Villena-Vargas J, Adusumilli PS. Cisplatin-induced antitumor immunomodulation: a review of preclinical and clinical evidence. Clin Cancer Res. 2014;20(21):5384–5391. doi:10.1158/1078-0432.CCR-14-1298.
- Lesterhuis WJ, Punt CJA, Hato SV, Eleveld-Trancikova D, Jansen BJH, Nierkens S, Schreibelt G, de Boer A, Van Herpen CML, Kaanders JH, et al. Platinum-based drugs disrupt STAT6-mediated suppression of immune responses against cancer in humans and mice. J Clin Invest. 2011;121(8):3100–3108. doi:10.1172/JCI43656.
- Mao Y, Poschke I, Wennerberg E, Pico de Coaña Y, Egyhazi Brage S, Schultz I, Hansson J, Masucci G, Lundqvist A, Kiessling R. Melanoma-educated CD14+cells acquire a myeloid-derived suppressor cell phenotype through COX-2–dependent mechanisms. Cancer Res. 2013;73(13):3877–3887. doi:10.1158/0008-5472.CAN-12-4115.
- Filipazzi P, Valenti R, Huber V, Pilla L, Canese P, Iero M, Castelli C, Mariani L, Parmiani G, Rivoltini L. Identification of a new subset of myeloid suppressor cells in peripheral blood of melanoma patients with modulation by a granulocyte-macrophage colony-stimulation factor-based antitumor vaccine. J Clin Oncol. 2007;25(18):2546–2553. doi:10.1200/JCO.2006.08.5829.
- Poschke I, Mougiakakos D, Hansson J, Masucci GV, Kiessling R. Immature immunosuppressive CD14+HLA-DR-/low cells in melanoma patients are Stat3hi and overexpress CD80, CD83, and DC-sign. Cancer Res. 2010;70:4335–4345. doi:10.1158/0008-5472.CAN-09-3767.
- Gullo JJ, Litterst CL, Maguire PJ, Sikic BI, Hoth DF, Woolley PV. Pharmacokinetics and protein binding of cis-dichlorodiammine platinum (II) administered as a one hour or as a twenty hour infusion. Cancer Chemother Pharmacol. 1980;5(1):21–26. doi:10.1007/BF00578558.
- Gamelin E, Bouil AL, Boisdron-Celle M, Turcant A, Delva R, Cailleux A, Krikorian A, Brienza S, Cvitkovic E, Robert J, et al. Cumulative pharmacokinetic study of oxaliplatin, administered every three weeks, combined with 5-fluorouracil in colorectal cancer patients. Clin Cancer Res. 1997;3(6):891–899.
- Shipp C, Speigl L, Janssen N, Martens A, Pawelec G. A clinical and biological perspective of human myeloid-derived suppressor cells in cancer. Cell Mol Life Sci. 2016;73(21):4043–4061. doi:10.1007/s00018-016-2278-y.
- Lechner MG, Megiel C, Russell SM, Bingham B, Arger N, Woo T, Epstein AL. Functional characterization of human Cd33+And Cd11b+myeloid-derived suppressor cell subsets induced from peripheral blood mononuclear cells co-cultured with a diverse set of human tumor cell lines. J Transl Med. 2011;9(1):90. doi:10.1186/1479-5876-9-90.
- Kim NR, Kim YJ. Oxaliplatin regulates myeloid-derived suppressor cell-mediated immunosuppression via downregulation of nuclear factor-κB signaling. Cancer Med. 2019;8(1):276–288. doi:10.1002/cam4.1878.
- Wang Z, Till B, Gao Q. Chemotherapeutic agent-mediated elimination of myeloid-derived suppressor cells. Oncoimmunology. 2017;6(7):e1331807. doi: 10.1080/2162402X.2017.1331807.
- Huang X, Cui S, Shu Y. Cisplatin selectively downregulated the frequency and immunoinhibitory function of myeloid-derived suppressor cells in a murine B16 melanoma model. Immunol Res. 2016;64(1):160–170. doi:10.1007/s12026-015-8734-1.
- Boudewijns S, Bloemendal M, de Haas N, Westdorp H, Bol KF, Schreibelt G, Aarntzen EHJG, Lesterhuis WJ, Gorris MAJ, Croockewit A, et al. Autologous monocyte-derived DC vaccination combined with cisplatin in stage III and IV melanoma patients: a prospective, randomized phase 2 trial. Cancer Immunol Immunother. 2020;69(3):477–488. doi:10.1007/s00262-019-02466-x.
- Aston WJ, Hope DE, Cook AM, Boon L, Dick I, Nowak AK, Lake RA, Lesterhuis WJ. Dexamethasone differentially depletes tumour and peripheral blood lymphocytes and can impact the efficacy of chemotherapy/checkpoint blockade combination treatment. Oncoimmunology. 2019;8(11):e1641390. doi:10.1080/2162402X.2019.1641390.
- Welters MJ, van der Sluis TC, Van Meir H, Loof NM, Van Ham VJ, Van Duikeren S, Santegoets SJ, Arens R, de Kam ML, Cohen AF, et al. Vaccination during myeloid cell depletion by cancer chemotherapy fosters robust T cell responses. Sci Transl Med. 2016;8(334):334ra52–334ra52. doi:10.1126/scitranslmed.aad8307.
- Al-Taei S, Banner R, Powell N, Evans M, Palaniappan N, Tabi Z, Man S. Decreased HPV-specific T cell responses and accumulation of immunosuppressive influences in oropharyngeal cancer patients following radical therapy. Cancer Immunol Immunother. 2013;62(12):1821–1830. doi:10.1007/s00262-013-1488-5.