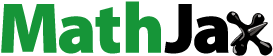
ABSTRACT
The monoclonal antibody against CTLA-4, Ipilimumab, is a first-in-class immune-checkpoint inhibitor approved for treatment of advanced melanoma in adults but not extensively studied in children. In light of the fact that the immune response early in life differs from that of adults, we have applied a human in vitro model stimulating CD4+ T-cells from neonates, children (1–5 years), and adults antigen-specifically with Staphylococcus aureus (S. aureus) for assessment of CTLA-4 blockade early in life. We show that T-cell proliferation as well as frequencies of antigen-specific T-cells (CD40L+CD4+) were enhanced in neonatal T-cells upon CTLA-4 blockade showing a larger variance within the group (F-test p < .0001). Using machine learning algorithm Random Forest, adult and neonatal T-cell responses can be unambiguously categorized (F1 score-0.75) on the basis of their cytokine (co-)expression. Blockade of CTLA-4 enhanced frequencies of IL-8, IFNγ, and IL-10 producers among CD40L+ T-cells. Of note, antigen-specific T-cells from neonates displayed higher cytokine coproduction at baseline, while T-cells from children caught up to neonates, and adults to baseline of children upon CTLA-4 blockade. These findings reveal that in neonatal T-cells blockade of CTLA-4 mainly unleashes the antigen-specific capacity by increasing the numbers of responding T-cells, whereas in children and adults it promotes the coexpression of cytokines by individual T-cells. Thus, CTLA-4 blockade boosts antitumor immunity through different mechanisms depending on the patients’ age. These data implicate a strong impact of the developmental stage of the T-cell compartment on the effects of immune-checkpoint therapy.
Introduction
Immune-checkpoint inhibitors (ICIs) are currently in use to treat malignant diseases and show long-term durable responses. Nevertheless, the promising therapeutic responses are limited to a minority of patients. Moreover, dramatic severe immune related adverse events (irAEs) often occur that are unpredictable, usually not even associated with therapeutic success.Citation1–4 A majority of clinical trials to date have evaluated ICIs in adult patients and still used cautiously in children.Citation5,Citation6 Given that the adaptive immune system acts very differently at the beginning of life compared to adultsCitation7,Citation8 and ICIs induce irAEs, it is a challenging task to transfer treatment protocols of immune-checkpoint therapies to neonates, toddlers and infants.
The immune system has developed sophisticated mechanisms to regulate, or more precisely to inhibit, the unintended activation of T-cells. Cytotoxic T-lymphocyte-associated Protein 4 (CTLA-4) is an inhibitory receptor on T-cells and its expression and function is intrinsically linked with T-cell activation. CTLA-4 is expressed on the surface of T-cells following TCR engagement, with its expression peaking 2–3 days after activation. The receptor counteracts TCR and CD28 mediated signals to suppress activation of T-cells.Citation9 Studies in in vitro and in vivo mouse models displayed that blockade of CTLA-4 signaling leads to an increase in T-cell proliferation and Th1-like cytokine production. Furthermore, it has been shown that CTLA-4 blockade affects in particular the proinflammatory cytokines IL-2 and IFNγ.Citation10,Citation11 Th2 responses are only impacted by CTLA-4 inhibition when not controlled by regulatory T-cells.Citation12 Ipilimumab a monoclonal antibody (MAb) against CTLA-4 was the first immune-checkpoint inhibitor to be approved for clinical use. It is applied to enhance immune responses in adult cancer patients.Citation13–15 Ipilimumab is used especially in immunogenic cancers such as advanced melanoma, and a pooled analysis of long-term follow up studies shows an overall survival rate of 22%.Citation16
While these results illustrate the contribution of the CTLA-4 axis to the downregulation of unwanted or terminal T-cell responses, and immune responses in adult cancer patients, its impact on antigen-specific T-cells early in life is unclear. As the adaptive immune responses differs substantially between adults and children, we hypothesize that in early life the effects of immune checkpoint blockade of CTLA-4 on T-cell biological functions, in terms of expansion and inflammatory cytokine production, may be much stronger than in adulthood. Hence, there is a serious need for the preclinical assessment of ICIs in early stages of life. This has to be performed with human in vitro models to avoid dramatic events, such as the devastating cytokine storm that occurred after the infusion of the CD28 superagonist antibody TGN1412 when no human in vitro model was used in advance.Citation17,Citation18 Therefore, to better understand the risk-benefit profile of ICIs across different age groups, we have analyzed the impact of CTLA-4 blockade on antigen-specific, human T-cell responses early in life and adults, and whether indeed age-dependent differences impact the efficacy of CTLA-4 blockade.
Results
Blockade of CTLA-4 during S. aureus-antigen specific T-cell stimulation shows enhanced proliferation in neonatal T-cells
Typically, T-cell responses early in life are thought to be aberrant in comparison to adult T-cell responses. Therefore, we have first adapted an antigen-specific stimulation model of human CD4+ T-cells to determine distinct characteristics of neonatal, children and adult T-cell responses.Citation19 To this purpose, CD4+ T-cells and CD14+ monocytes were isolated from adult blood (A), adenoids and blood from 1-5-year-old children (C), and umbilical cord blood (N), respectively, using MACS. Monocytes were matured overnight with h.i. S. aureus (SEBneg) and used to stimulate CD4+ T-cells. Monocytes from 5 neonates, 5 1- to 5-year-old children and 5 adults upon maturation with S. aureus expressed CD16 and cytokines IL-6 and IL-1β . No statistically significant differences in expression CD16 (A vs. C: p = .085; C vs. N p = .071; N vs. A p > .99), IL-6 (A vs. C: p > .99; C vs. N p > .99; N vs. A p > .99) and IL-1β (A vs. C: p = .967; C vs. N p > .99; N vs. A p > .99) () were observed, upon comparison of monocytes from adult, children and neonatal groups with each other. Upon coculture with S. aureus-loaded monocytes, also neonatal CD4+ T-cells could clearly be activated, which was determined by the expression of the activation marker CD25 (data not shown) and induced proliferation as indicated (). To test antigen-specificity of the S. aureus stimulation, HLA-DR was blocked using specific antibodies during maturation and stimulation, and CFSE was used to monitor T-cell proliferation (). Blockade of HLA-DR prevented the S. aureus induced T-cell proliferation of children by 96% and of neonates by 97% confirming antigen-specificity of the response.
Table 1. Summarized detailed data of monocyte maturation, T helper cell antigen specificity and CTLA-4 expression compared among different age groups
Figure 1. Antigen-specific T-cell responses of different age groups
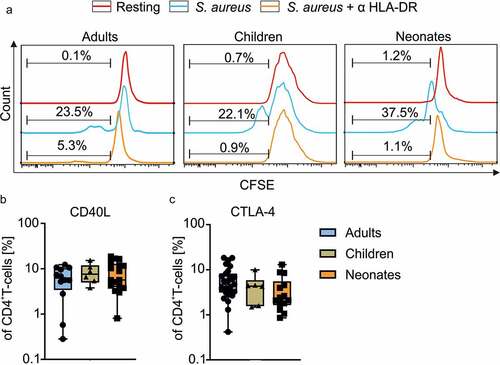
To identify antigen-specific responsiveness of neonatal T-cells in comparison to adults, CD4+ T-cells were activated for 4 d with S. aureus-matured monocytes and analyzed for the expression of CD40L by flow cytometry. Frequencies of CD40L expressing CD4+ T-cells of 12 adults, 6 children, and 17 neonates, did not significantly differ (all comparisons show p > .99 ()) (). To monitor whether activated T cells from neonates and children were able to express CTLA-4 at the cell surface, it´s expression was analyzed by flow cytometry (). In all age groups (A n = 27; C n = 6; N n = 14) examined, frequencies of CTLA-4 surface expressing cells were unambiguously detectable and similar between age groups (A: median 4.74 range 18.40–0.42; C: median 4.32 range 9.96–1.48; N: median 2.26 range 13.00–0.86) (A vs. C p > .99; C vs. N p > .99; N vs. A p = .103) (, ).
To investigate the age-related effects of CTLA-4 blockade on T-helper cells of neonates children and adults, CD4+ T-cells from respective 17 neonates, 6 children and 12 adults, were incubated with anti-CTLA-4 antibody or isotype control prior to priming them with autologous monocytes, which had been matured over night with h.i. S. aureus. Of note, comparing routinely stimulation of T cells with S. aureus-matured monocytes in the presence and absence of isotype control gave similar results (data not shown). On day 4, cells were briefly restimulated with h.i. S. aureus for 5 h to identify S. aureus-specific effector CD4+ T-cells. Flow cytometric analysis comparing controls to CTLA-4 blockade revealed enhanced frequencies of CD40L+ T-cells of neonates (median 5.64 range 18.60–0.80 vs. median 9.65 range 44.10–1.18, p < .049), but not of children (median 7.58 range 15.20–3.77 vs. median 4.88 range 16.40–1.31, p = .219) and adults (median 6.24 range 12.30–0.28 vs. median 5.27 range 8.12–0.52, p < .002), when CTLA-4 was blocked during stimulation (, Table S1). Importantly, significant differences in the deviations between the two age groups were determined using the F-test showing a significantly higher deviation in S. aureus-specific T-cells from neonates and children in comparison to adults when CTLA-4 was blocked (). To determine the impact of antigen-specific responsiveness, T-cell proliferation in response to S. aureus was determined using CFSE-labeling prior to stimulation. In comparison to T-cells from adults (n = 7) and children (n = 5), only T-cells of the neonates (n = 9) displayed upon CTLA-4 blockade an enhanced frequency of proliferating cells (CFSElow) (N: median 35.90 range 65.20–0.26 vs. median 44.70 range 76.50–14.60, p = .014) during antigen-specific stimulation for a period of 6 days () [(A: median 6.29 range 49.20–3.62 vs. median 12.50 range 44.30–3.92, p = .234) (C: median 8.96 range 25.40–1.42 vs. median 12.90 range 32.10–2.09, p = .094)] (Table S1). Of note, stimulation in the absence of CTLA-4 blockade did not show significant differences in proliferation among age groups (Table S2), whereas under CTLA-4 blockade, indeed, neonates vs. adults show to be significantly different (N: median 44.70 range 76.50–14.60, n = 9; A: median 12.50 range 44.30–3.92, n = 7; p = .041) (). Next, we wanted to know whether indeed more neonatal T-cells of the whole T-cell pool responded or rather similar original cells started in G0 but proliferated more vigorously. Therefore, original responders of G0 at time point 0 of total CD4+ T-cells were calculated for each generation (G1, G2, G3) separately and in total (Σ G1-G3) (). Blockade of CTLA-4 was found to significantly enhance frequencies (%) of total, original responding T-cells of neonates (median 2.90 range 6.50–0.16 vs. median 4.40 range 12.10–2.04, n = 9, p = .002), mainly by significantly enhancing slower responding T-cells of G1 (median 1.40 range 2.90–0.04 vs. median 2.50 range 6.20–1.06, p = .002) and G2 (median 0.90 range 2.00–0.10 vs. median 1.51 range 4.10–0.60, p = .004 (, Table S1). The ratio of original responding T-cells in G0 under CTLA-4 blockade to original responding T-cells without blockade (A: median 1.05 range 1.47–0.68, n = 7; N: median 1.86 range 13.09–1.06, n = 9; p = .019) supports this finding that more T cells in G0 responded with proliferation to CTLA-4 blockade in the neonatal than adult pool ().
Figure 2. Impact of CTLA-4 blockade on antigen-specific CD4+ T-cell responsiveness of adults, children and neonates
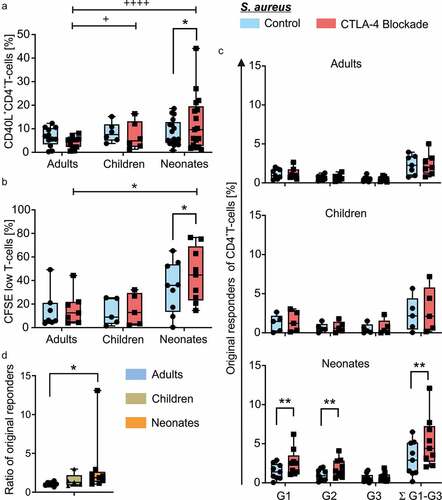
Blockade of CTLA-4 leads to increased frequencies of inflammatory cytokine producers in CD4+ T-helper cells from adults and children
We next wanted to know whether it is indeed possible to separate between neonates and adults on the basis of the observed frequencies of cytokine producers in the encountered CD4+ T-cell responses. To this purpose, CD4+ T-cells of 12 neonates and 12 adults were stimulated with S. aureus-matured monocytes as described in and the expression of CD40L and the cytokines TNFα, IL-8, IL-10, IFNγ, and IL-17a were determined by flow cytometry. Then, the machine learning classifier Random Forest (RF) was applied with fourfold stratified cross-validation, using CD40L and cytokine coexpression as features. To deal with the effects of the random data sampling for the construction of the fourfolds, 1000 runs were performed and the achieved F-scores were averaged. The average F1 score was 0.75 with a standard deviation (SD) of 0.065 (Supplemental Figure S1). The expected deviation from the true F1 score average is ± 0.00403 at a 95% confidence interval. This demonstrates that CD40L and cytokine-coexpression allow for a separation between neonatal and adult CD4+ T-cell responses.
In order to investigate the role of CTLA-4 in CD4+ T-cell responses at different stages of life, neonatal, children and adult CD4+ T-cells were stimulated with S. aureus-matured monocytes with and without blockade of CTLA-4 as described in . Responding antigen-specific CD40L+ T-cells (A: median 6.24 range 12.30–0.28, n = 12, C: median 7.58 range 15.20–3.77, n = 6, N: median 5.64 range 18.60–0.80, n = 17, all comparisons p > .99) revealed significantly enhanced frequencies of IL-8 and IFNγ cytokine producers by neonates and children compared with adults, in addition to IL-10 producers by neonates compared with adults (–e, median, range, n, and p values are summarized in Supplemental Table S2)). Under CTLA-4 blockade, enhanced frequencies of IL-8 producers were particularly observed in neonates compared to adults and children (N: median 15.30 range 92.70–1.93, n = 17; C: median 23.65 range 40.50–11.60, n = 6; A: median 6.85 range 18.70–1.27, n = 12; A vs. C p = .004; N vs. A p = .014). In addition IL-17 (C: median 16.90 range 40.50–8.85 n = 6; A: median 4.85 range 20.70–2.53, n = 12; p = .029), IL-10 (C: median 19.75 range 47.70–13.30, n = 6; A: median 5.87 range 22.30–2.13, n = 12; p = .023) and IFNγ (C: median 28.75 range 57.70–17.90, n = 6; A: median 6.83 range 20.40–3.78, n = 12; p = .003) producers were significantly enhanced by children compared to adults. Although having in general high frequencies of cytokine producers in neonates, blockade of CTLA-4 in comparison to controls, further enhanced the frequencies of cytokine producers IFNγ, IL-8, and IL-10 significantly in all three age groups. Importantly, whereas frequencies of each of these cytokine producers almost doubled especially in children upon CTLA-4 blockade (i.e. IL-10, C: median 11.95 range 18.10–3.98 vs. median 19.75 range 47.70–13.30, n = 6, p = .016), while neonatal frequencies enhanced only by about 10–15% (N: median 11.80 range 79.00–1.88 vs. median 14.50 range 88.80–3.48, n = 17, p = .028). While the IL-4 producers did not enhance significantly in any age group examined upon CTLA-4 blockade, enhanced frequencies were observed for IL-17 producers in children and neonates only (–e, Table S1). As activated T-cells of neonates and children express CTLA-4 at their cell surface () and the blockade of CTLA-4 was able to enhance their frequency of effector cytokine expression of IL-8, IL-10, IL-17, and IFNγ (), neonatal as well as children´s T-cell responses demonstrated to be controlled by CTLA-4.
Figure 3. Cytokine expression in antigen-specific T helper cells from neonates, children, and adults after blockade of CTLA-4
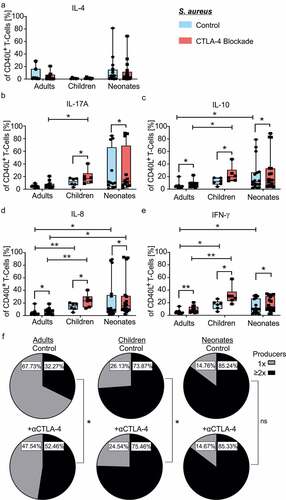
T-cells capable of simultaneous production of multiple cytokines are considered to be of high quality effector cells, providing potent and durable cellular immunity against infections or cancer.Citation20–22 Therefore, we next analyzed age-dependent diversity of the antigen-specific T-cell multifunctionality. The respective cytokine data of antigen-specific CD40L+ T-cells indicated that T-cell multifunctionality developed at any age. However, CD40L+ T-cells displayed a lower degree of multifunctionality with the increase in age (). Intriguingly, blockade of CTLA-4 significantly enhanced frequencies of multiple cytokine coproducing CD4+ T-cells specifically from adults and also children but not of those from neonates (). The maximum effect of blockade of CTLA-4 in adult CD4+ T-cells was observed in quadruple producers () but not on double or triple producers (data not shown). In neonatal and children CD4+ T-cells, quadruple cytokine producers were the dominant subpopulation among multifunctional T-cells, regardless of CTLA-4 blockade () and significantly enhanced in comparison to adults (A: median 1.14 range 5.79–0.51, C: median 8.898 range 12.87–2.37, N: median 5.81 range 57.42–1.03; A vs. C p = .008 N vs. A p = .015, ). Dissecting the individual functional T-cell subsets, we found that most of quadruple producers were IFNγ, IL-17, IL-10, and IL-8 coproducers in neonates, children and adults (, ). In contrast, the Th2-associated cytokine IL-4 was rarely observed in combination with other cytokines in neonates and almost not at all in children and adults (). Collectively, these results indicated that CD4+ T-cells from neonates in comparison to adults readily mount a vigorous response against antigens presented by autologous APCs (). Blockade of CTLA-4 boosts the efficiency of CD4+ T-cells from children to match to that of neonates, and from adults to almost match to that of children ().
Table 2. Specific detail data of 4x cytokine producers and IL-10 + 3× cytokine producers with and without blockade of CTLA-4
Table 3. Summarized detailed data of 4× cytokine producers and IL-10 + 3× cytokine producers compared among different age groups
Figure 4. Age-dependent diversity of CTLA-4 blockade in regulating high-quality cytokine coproducers
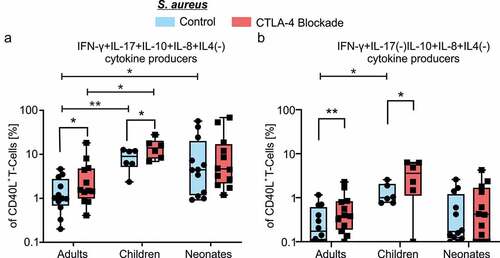
Enhanced IL-10 upon CTLA-4 blockade was coexpressed in Th1-like cells
Our data revealed that blockade of CTLA-4 enhanced the frequency of IL-10 producers significantly in all three age groups, adults, children and neonates resulting in significantly age-related enhanced frequencies in children compared to adults (, Table S1). IL-10 can act as an immune suppressor directly on T-cells by inhibiting proliferation and suppressing pro-inflammatory responses as a means to prevent collateral immune damage. But this mechanism may also limit effectiveness of immune response impairing the control of pathogens.Citation23 Therefore, we next asked if enhanced IL-10 expression upon CTLA-4 blockade is regulating multifunctionality of T-cells, especially in the triple producers. For this purpose, we have focused on IL-10 cytokine coproducers in all triple producers of antigen-specific CD40L+ T-cells, the most dominant triple-producers are Th1-like cells (IFNγ+IL-10+IL-8+) and are depicted in . These triple producers in comparison to controls were significantly enhanced upon CTLA-4 blockade in adults (A: median 0.17 range 1.16–0.00 vs. median 0.39 range 2.28–0.06, n = 12, p = .003) and children (C: median 1.00 range 2.57–0.76 vs. median 3.58 range 6.31–0.00, n = 6, p = .047) (, ). These results indicated that frequencies of IL-10-expressing triple producers significantly enhanced upon CTLA-4 blockade in adults and children is due to enhanced Th1 effector cytokines coexpression along with IL-10 (). CTLA-4 blockade of neonatal T-cells showed the highest frequency of IL-10+ triple producers coexpressing Th1 effector cytokines. CTLA-4 blockade significantly enhanced IL-10 coexpressing Th17-like cells too in neonates, however, these cells constituted only a small fraction of the IL-10+ triple producers ().
Discussion
In the present investigation, we have analyzed the potential of the CTLA-4-CD80/86- axis as a target for the specific intervention by CTLA-4 blockade for the treatment of patients early in life. We report the intriguing observation that – in contrast to T-cells from adults – blockade of CTLA-4 early in life strongly enhances antigen-specific CD4+ T-cell responses by triggering proliferation in a large number of original T-helper cells. In addition, many antigen-specific T-cells identified by CD40L expression are triggered to produce IL-10, IL-8, and IFNγ during treatment with anti-CTLA-4; which was more pronounced in neonates than in adults. We further demonstrate that multifunctional T-cells that are considered being high quality effector cells, providing potent and durable cellular immunity against infections or cancer, display an age dependent diversity in multifunctionality of antigen-specific responses (). Herein, CD4+ T-cells from neonates show the highest degree of multiple cytokine producers followed by children, whereas adult T-cell responses where characterized by a high degree of single cytokine producers. This is especially true at baseline, where the numbers of cytokine-coexpressing CD4+ T-cells from neonates already reach a plateau in response to antigen-specific stimulation alone. Blockade of CTLA-4 mainly affects cytokine-coexpressing CD4+ T-cells from adults, revealing an immune-checkpoint mediated loss of multifunctionality of CD4+ T-cell responses in an aged immune system (). Ultimately, this study provides new evidence that blockade of CTLA-4 acts age dependently, enhancing the numbers of responding T-cells in neonates and the frequency of multifunctional cytokine producers in children and adults at different levels.
We have demonstrated that blockade of CTLA-4 increases frequencies of activated CD40L+ T-cells and proliferation of original T-cells specifically in neonates rather than in children and adults (, b and c). Even though no difference in expression of CTLA-4 was found between samples (), the characteristic rapid activation by neonatal T-cellsCitation24,Citation25 is likely tightly controlled by CTLA-4 explaining their increased sensitivity to CTLA-4 blocking antibodies.Citation26 Furthermore, a lowered threshold for neonatal T-cell activation/stimulation due to increased sensitivity to CTLA-4 MAb might therefore allow expression of otherwise controlled genes or combinations.Citation27
Neonatal CD4+ T-cells are reported to display IL-8, IL-17, and IL-22 as signature cytokines.Citation28–30 In our model we extend this finding, that indeed IL-8 and IL-17 coexpressing cells are enhanced in early stages of life, and show that CTLA-4 blockade induces a similar phenomenon to a profound extent with the increase in age (). Thus, CTLA-4 strongly restricts coexpression of cytokines in adult T-cells.
Blockade of CTLA-4 had a similar impact on T-cells from neonates, children and adults in significantly enhancing frequencies of IL-10, IL-8, and IFNγ producers but not IL-4 producers (–e) as it has been reported in mice.Citation31 Effector T-cells producing multiple cytokines play an important role in defense against infections and cancer.Citation20–22 Accelerated terminal differentiation in neonatal T-cellsCitation25 might enhance the frequency of such high quality effector T-cells producing multiple cytokines to a near maximum which cannot be further increased by CTLA-4 blockade (). The capacity of T-cells from neonates to respond to antigen stimulation with multifunctional cytokine production, might bring them into a favorable position for defending the body. Whether this is another piece of the puzzle to explain, e.g. asymptomatic viral infections to Epstein-Barr virus or hepatitis B in neonates, toddlers, and young children needs to be proven.Citation32–35
Whereas quite some Phase I/II studies with ICI in pediatric tumors have been disappointing, it was shown to be promising in Hodgkin and non-Hodgkins lymphoma.Citation36–38 Of importance, initiated childhood cancers as they occur in neonates, toddlers, and infants are usually different than adult ones, they show less mutational load, which adult cells acquire gradually over time, making childhood cancers less likely to be recognized by the adaptive immune response. Pediatric tumors rather arise from embryonal cells than epithelial cells and others such as ALL are often associated with a compromised immune response.Citation39 An inherited risk factor is, e.g. trisomy 21. Due to the low levels of neoantigens of childhood tumors (in addition to other factors limiting antigen recognition), it has already been proposed to use combination therapy in pediatric cancers, aiming at enhancing other therapeutic approaches by ICIs.Citation36–38 The initial recognition of the tumor, is triggered for this purpose by other therapeutic approaches, such as radiotherapy, or for activating and directing polyclonal T-cell responses with bispecific antibodies (e.g. a fusion protein of anti-CD3 and a tumor antigen, e.g. anti-CD19) or CAR T-cells to the target cells.Citation37 Especially the enhanced coexpression of cytokines under CTLA-4 blockade in children and adults would eventually contribute to enhanced lysis of target cells when applied in this combinations.Citation22 Of concern, whether multifunctional memory T-cells are established, which could be a risk factor for self-sustaining, long-lasting autoimmunity, remains to be carefully controlled in children.Citation40 However, human in vitro studies suggest that the T-cells eventually undergo apoptosis when CTLA-4 is blocked which might counteract these unfavorable effects.Citation41,Citation42 In addition, several strategies like interruption of treatment and/or supportive therapy which include administration of immunosuppresants are adopted to manage irAEs.Citation43,Citation44
Even though our data show that blockade of CTLA-4 did not further increase the proportion of multifunctional neonatal T-cells, the checkpoint blockade of CTLA-4 still significantly enhanced the numbers of individual cytokine producers as well as the frequency of responding T-cells and their proliferation, indicating CTLA-4 impinges on more than one pathway.Citation10,Citation12,Citation27,Citation45,Citation46 The accelerated expansion of neonatal T-cells upon CTLA-4 blockade () could boost the relative numbers of multifunctional T-cells in neonates to a much higher level than in adults. Even though, children did not display accelerated expansion of T-cells upon CTLA-4 blockade, they show high proportion of multifunctional T-cells in comparison to those from adults, which are further increased upon CTLA-4 blockade (). This should be taken as a note of caution; pediatric patients getting an immune checkpoint therapy may only tolerate lower doses of Ipilimumab, whereas higher doses (that are tested in adults) could trigger massive proliferation of effector T-cells or increase in proportion of multifunctional T-cells. Both these events may lead to toxicities with all immune related adverse events as shown in a cohort of children with an average of 13 years, also including 2-year-oldsCitation6; more data of patients aged 1–5 are warranted. Further, the effects of anti-CTLA-4 showed a much higher variability for neonates than adults, which may reflect an increased risk of adverse effects of ICI. It would be important to assess responsiveness – low/nonresponders as well as strong responders – prior therapeutic manipulations with CTLA-4. In the same line, Hodgkin-lymphoma patients and pediatric melanoma patients – also being rare below the age of 6 – plus patients getting combinational therapies with CAR T-cells are at a risk of experiencing unpredictable irAEs upon Ipilimumab administration. Therefore, individual responses early in life could possibly be evaluated beforehand by testing their T-cell responses in in vitro using models as in this study to determine the safely administrable dose of Ipilimumab.
IL-10 expressing triple producers were low, but still enhanced upon CTLA-4 blockade in neonates, children and adults with the majority of these cells being Th1-like cytokine producers (). Therefore, an immunosuppressive effect of local IL-10 is unlikely to affect CTLA-4 blockade.Citation47–50 Th1-like IL-10 producers have been described as regulatory cells and might help in regulating exaggerated inflammatory response mitigating irAE-like tissue damage.Citation51
As we report here, with the increase in age T-cells seem to partially have lost coexpression of cytokines; however, the blockade of CTLA-4 apparently makes up for this acquired dysfunction of the aged immune system. It is also tempting to speculate that reduced exposure to microbes caused by urban life style may impair the generation of multifunctional T-cells during early childhood (Greaves hypothesis) compressing the memory pool with the increase in age. In this case, CTLA-4 blockade may “rejuvenate” the T-cells and thereby counteract this functional loss of the aged T-cell memory pool.
In conclusion, this study provides novel comprehensive insights into the CTLA-4 controlled diversity of antigen specific CD4+ T-cell responses in neonates, children, and adults. It shows the age dependency of responses to the blockade of such an immune checkpoint that contributes to evaluate the (combinational) application of this form of immunotherapy to younger patients.
Materials and methods
Patient material
Peripheral blood mononuclear cells (PBMCs) were prepared from leukocyte reduction filters (Sepacell RZ-2000; Asahi Kasaei Medical, Japan) of healthy adult donors (not matched of age and sex, age range 21.3–64 years, median age 43.5 years) supplied by the Institute of Transfusions Medicine and Immunohematology at the University Hospital of Magdeburg (Germany). Donors did not use antibiotics for the last 3 months, were nonsmokers, did not take any drugs, and had no chronic diseases. The blood and adenoids were obtained from children (age range 1–5 years, median age 3.4 years) suffering from adenoid hypertrophy through surgical excision, and supplied by the Department of Otorhinolaryngology of the University Hospital in Magdeburg. Cord blood (CB) samples taken from full-term births were obtained from umbilical cord veins immediately after birth from the Women’s Clinic of the University Hospital of Magdeburg. The study was approved by the Clinical Research Ethics Board of the University of Magdeburg (certificates 06/11, 79/07, and 26/12), and all donors and/or parents gave written informed consent in accordance with the declaration of Helsinki.
Cell purification and cell culture
PBMCs from peripheral blood of healthy donors (adults), cord blood (neonates), and of blood of children were purified by centrifugation using Ficoll-Hypaque density gradient. CD14+ monocytes were isolated from purified CBMCs and PBMCs by using CD14-microbeads (ref: 130–050-201), and autoMACS pro (both from Miltenyi Biotec, Germany) according to the manufacturer’s instructions. Isolated monocytes were matured with heat inactivated (h.i.) (1 h, 65°C and 3x shock frozen in liquid nitrogen) S. aureus (Staphylococcal enterotoxin (SEB)neg) overnight at 37°C in RPMI 1640 medium (ref: P04-18500, PAN-Biotec, Germany), supplemented with 10% Fetal Bovine Serum (ref: F7524, Sigma-Aldrich/Merck, Germany); and 10 U/ml penicillin; 10 µg/ml streptomycin (ref: 15140122, Life Technologies/Thermo Fischer Scientific, US). Monocytes were washed twice with complete RPMI medium prior to coculturing with autologous CD4+ T-cells. Single cell suspensions were generated by mechanical dissociation from surgically excised adenoids (of children suffering from adenoid hypertrophy) in PBS/0.2% BSA over a 70 µm cell strainer (BD Biosciences, US). Mononuclear cells (MCs) were purified by centrifugation using Ficoll-Hypaque density gradient.Citation52 CD4+ T-cells from purified MCs of PB of adults, CB of neonates and blood and surgically excised adenoids from children were enriched to a purity of >98% using human CD4-microbeads (ref: 130–045-101, Miltenyi Biotec, Germany) by magnetic beads separation with autoMACS-pro according to the manufacturer’s instructions.
A total of 2 × 105 enriched CD4+ T-cells were stimulated with the S. aureus-pulsed CD14+CD16+ mature monocytes at a ratio 2.5:1 for 4 or 6 days. For blockade of HLA-DR, monocytes were incubated for 30 min at 37°C in RPMI 1640 medium with anti-HLA-DR MAb (10 µg/ml, L249, purified from hybridoma supernatant, quality controlled by Western blotting and competitive FACS analysis) prior to their maturation with h.i. S. aureus. Once matured, monocytes were washed twice and were incubated once again with anti-HLA-DR MAb for 30 min at 37°C in RPMI 1640 medium and then cocultured with CD4+ T-cells as described above. CTLA-4 signaling on T-cells was blocked by the addition of anti-CTLA-4 (CD152) antibody (50 µg/ml, clone: BNI3) purchased from BD biosciences, US (ref: 555850) or purified from hybridoma supernatants using protein G and controlled using flow cytometry. Isotype control antibody (Clone: MG2a-53, ref: 401504) for CTLA-4 blockade was purchased from Biolegend, US. All T-cells isolated from adenoids were tested for resting by verifying that they did not express CD69 after 12 h in culture and that they did not proliferate spontaneously in the presence of only monocytes within 6 d (CFSE assay as described above).
Flow cytometric analysis
For surface marker analysis, cells were stained with indicated surface markers in PBS/0.2% BSA. Enriched and activated CD4+ T-cells were identified using surface staining by specific fluorescent labeled antibodies (PerCp conjugated anti-CD3 (clone: SK7, ref: 344814), PE/cyanine7 conjugated anti-CD4 (clone: RPA-T4, ref: 300512) and APC/cyanine7 conjugated anti-CD25 (clone: BC96, ref: 302614) (all from Biolegend, US)), classical and mature monocytes were identified using PE-Vio770 conjugated anti-CD14 (clone: REA-599, ref: 130–110-521), and APC-Vio770 conjugated anti-CD16 (clone: REA-423, ref: 130–106-707) (both from Miltenyi Biotec, Germany). T-cell proliferation was determined by labeling CD4+ T-cells with the vital dye carboxyfluorescein succinimidyl ester (CFSE, ref: C1157, Invitrogen/Thermo Fischer Scientific, US) and measuring CFSE dilution using flow cytometry. Using the sensitive CFSE assay as read out, CTLA-4 blockade using BNI3 was routinely controlled comparing the stimulation of T-cells in the presence and absence of Isotype control (50 µg/ml, Clone: MG2a-53, ref: 401504, Biolegend, US) with CTLA-4 blockade, which gave similar results.
Prior to intracellular cytokine analysis on day 4, T-cells were pulsed with h.i. S. aureus, monensin (ref: M5273, Sigma-Aldrich, US) 10 µg/ml and brefeldin A 5 µg/ml (ref: 9972, Cell Signaling, US) for 5 h. Intracellular staining was performed after the cells were surface stained with PerCp conjugated anti-CD3 (clone: SK7, ref: 344814, Biolegend, US) and APC- Vio770 conjugated anti-CD4 (clone: REA623, ref: 130–11-223, Miltenyi Biotec, Germany) antibodies. The surface stained cells were then fixed with 2% paraformaldehyde (ref: 11762.01000, Morphisto, Germany) in PBS for 20 min followed by permeabilization with 0.5% saponin (ref: S7900, Sigma-Aldrich, US) in PBS/0.2% BSA, and then incubation with following antibodies: PE/cyanine7 conjugated anti-IL-4 (clone: MP4-25D2, ref: 500824), BV421 conjugated anti-IL-10 (clone: JES3-9D7, ref: 501422), BV510 conjugated anti-IFNγ (clone: 4S.B3, ref: 502544), (all from Biolegend, US) FITC conjugated anti-IL-8 (clone: AS14, ref: 340509) (BD Biosciences, US), PE conjugated anti-IL-17a (clone: ebio64DEC17, ref: 12–7179-42) (Invitrogen/Thermo Fischer Scientific, US). To identify cytokine expression in recently activated antigen-specific CD4+ T-cells, APC conjugated anti-CD154 (CD40L) antibody (clone: 24–31, ref: 310810, Biolegend, US) was used to identify expression of CD40L. A Boolean gating strategy was used for analysis of single and multiple cytokine producers among all antigen-specific CD4+ CD40L+ T-cells. Monocyte maturation was routinely determined by intracellular measurement of cytokines using APC conjugated anti-IL-6 (clone: MQ2-13A5, ref: 501112, Biolegend, US), PE conjugated anti-IL-1β (clone: CRM56, ref: 12–7018-82, Invitrogen/Thermo Fischer Scientific, US) antibodies. All flow cytometric measurements were performed using FACS-Canto II (BD Biosciences, US) and analyzed with FlowJo software (FlowJoTM Software, LLC, US)
Machine learning
For the separation between CD4+ T-cell responses of neonatals and adults, we used as features: 36 cytokine coexpressions, CD40L, IFNγ/IL10 and the percentage of the CD4+ T-cells that did not produce any of the measured cytokines. On them, we trained a Random Forest (RF)Citation53 in a scikit-learn implementationFootnote1.Citation54 that uses an optimized version of the CART algorithm for decision tree induction. We set the number of decision trees to 100 and the maximum tree depth to 6 and we performed fourfold stratified cross-validation.
For the other RF parameters, we used the default values of the scikit-learn implementation, namely: each decision tree was allowed to use 50% of the 39 features and a bootstrapped sample of the training data (i.e. 18 instances in three folds for training and 6 instances in the test fold); the split criterion was Gini impurity; the minimum number of instances per node to allow for a split was 2; the minimum number of instances per leaf node was 1; no limit to the maximum number of leaf nodes; no pruning; label assignment through simple majority voting. As performance evaluation criterion we used F1 scoreCitation55 averaged over the fourfolds. The RF-model performance was affected by the placement of the instances in the folds, which was done randomly; to deal with this effect, we performed 1000 runs and computed the average F1 score over them.
Statistical analysis
Statistical analysis was performed using the Graph pad prism 8 software (GraphPad software Inc., US). The data are presented as median with data range [interquartile range (box) and upper limit to lower limit (whiskers)]. Comparison of distribution of two dependent samples within one age group were performed using Wilcoxon matched-pairs signed rank test. Variances between populations were compared using F-test (). Comparison between age groups were performed using Kruskal-Wallis test followed by Dunn’s multiple comparisons test. Statistical significance was reached when p < .05 (*), p < .01 (**), p < .001 (***) and p < .0001 (****).
Supplemental Material
Download ()Acknowledgments
The authors like to thank Noor Jamaludeen for discussions and Kathrin Kramer and Beatrix Kramer for excellent technical support.
Disclosure statement
The authors declare no financial or commercial conflict of interest.
Supplementary material
Supplemental data for this article can be accessed on the publisher’s website.
Additional information
Funding
Notes
1 XGB code from: https://xgboost.readthedocs.io/en/latest/python/index.html
References
- Feng Y, Roy A, Masson E, Chen TT, Humphrey R, Weber JS. Exposure-response relationships of the efficacy and safety of ipilimumab in patients with advanced melanoma. Clin Cancer Res. 2013;19(14):3977–12. doi:10.1158/1078-0432.CCR-12-3243.
- Sharma P, Allison JP. The future of immune checkpoint therapy. Science. 2015;348(6230):56–61. doi:10.1126/science.aaa8172.
- De Velasco G, Je Y, Bossé D, Awad MM, Ott PA, Moreira RB, Schutz F, Bellmunt J, Sonpavde GP, Hodi FS, et al. Comprehensive meta-analysis of key immune-related adverse events from CTLA-4 and PD-1/PD-L1 inhibitors in cancer patients. Cancer Immunol Res. 2017;5(4):312–318. doi:10.1158/2326-6066.CIR-16-0237.
- Shulgin B, Kosinsky Y, Omelchenko A, Chu L, Mugundu G, Aksenov S, Pimentel R, DeYulia G, Kim G, Peskov K, et al. Dose dependence of treatment-related adverse events for immune checkpoint inhibitor therapies: a model-based meta-analysis. OncoImmunology. 2020;9(1):1748982. doi:10.1080/2162402X.2020.1748982.
- Geoerger B, Bergeron C, Gore L, Sender L, Dunkel IJ, Herzog C, Brochez L, Cruz O, Nysom K, Berghorn E, et al. Phase II study of ipilimumab in adolescents with unresectable stage III or IV malignant melanoma. Eur J Cancer. 2017;86:358–363. doi:10.1016/j.ejca.2017.09.032.
- Merchant MS, Wright M, Baird K, Wexler LH, Rodriguez-Galindo C, Bernstein D, Delbrook C, Lodish M, Bishop R, Wolchok JD, et al. Phase I clinical trial of ipilimumab in pediatric patients with advanced solid tumors. Clin Cancer Res. 2016;22(6):1364–1370. doi:10.1158/1078-0432.CCR-15-0491.
- Adkins B, Leclerc C, Marshall-Clarke S. Neonatal adaptive immunity comes of age. Nat Rev Immunol. 2004;4(7):553–564. doi:10.1038/nri1394.
- Marchant A, Goldman M. T cell-mediated immune responses in human newborns: ready to learn? Clin Exp Immunol. 2005;141(1):10–18. doi:10.1111/j.1365-2249.2005.02799.x.
- Walunas TL, Lenschow DJ, Bakker CY, Linsley PS, Freeman GJ, Green JM, Thompson CB, Bluestone JA. CTLA-4 can function as a negative regulator of T cell activation. Immunity. 1994;1(5):405–413. doi:10.1016/1074-7613(94)90071-X.
- Brunner MC, Chambers CA, Chan FK, Hanke J, Winoto A, Allison JP. CTLA-4-Mediated inhibition of early events of T cell proliferation. J Immunol 1950;1999:5813–5820.
- Pandiyan P, Hegel JKE, Krueger M, Quandt D, Brunner-Weinzierl MC. High IFN-gamma production of individual CD8 T lymphocytes is controlled by CD152 (CTLA-4). J Immunol 2007;178(4):2132–2140. doi:10.4049/jimmunol.178.4.2132.
- Pierau M, Lingel H, Vogel K, Arra A, Brunner-Weinzierl MC. CTLA-4-competent conventional T cells back up regulatory T cells to restrain memory T-helper type 2 cell responses. Allergy. 2020;75(3):684–687. doi:10.1111/all.14042.
- Ascierto PA, Simeone E, Sileni VC, Pigozzo J, Maio M, Altomonte M. Clinical experience with ipilimumab 3 mg/kg: real-world efficacy and safety data from an expanded access programme cohort. J Transl Med. 2014;12:116.
- Hodi FS, O’Day SJ, McDermott DF, Weber RW, Sosman JA, Haanen JB, Gonzalez R, Robert C, Schadendorf D, Hassel JC, et al. Improved survival with ipilimumab in patients with metastatic melanoma. N Engl J Med. 2010;363(8):711–723. doi:10.1056/NEJMoa1003466.
- Wolchok JD, Neyns B, Linette G, Negrier S, Lutzky J, Thomas L, Waterfield W, Schadendorf D, Smylie M, Guthrie T, et al. Ipilimumab monotherapy in patients with pretreated advanced melanoma: a randomised, double-blind, multicentre, phase 2, dose-ranging study. Lancet Oncol. 2010;11(2):155–164. doi:10.1016/S1470-2045(09)70334-1.
- Schadendorf D, Hodi FS, Robert C, Weber JS, Margolin K, Hamid O, Patt D, Chen -T-T, Berman DM, Wolchok JD, et al. Pooled analysis of long-term survival data from phase II and phase III trials of ipilimumab in unresectable or metastatic melanoma. J Clin Oncol. 2015;33(17):1889–1894. doi:10.1200/JCO.2014.56.2736.
- Suntharalingam G, Perry MR, Ward S, Brett SJ, Castello-Cortes A, Brunner MD, Panoskaltsis N. Cytokine storm in a phase 1 trial of the anti-CD28 monoclonal antibody TGN1412. N Engl J Med. 2006;355(10):1018–1028. doi:10.1056/NEJMoa063842.
- Eastwood D, Findlay L, Poole S, Bird C, Wadhwa M, Moore M, Burns C, Thorpe R, Stebbings R. Monoclonal antibody TGN1412 trial failure explained by species differences in CD28 expression on CD4+ effector memory T-cells. Br J Pharmacol. 2010;161(3):512–526. doi:10.1111/j.1476-5381.2010.00922.x.
- Geiger R, Duhen T, Lanzavecchia A, Sallusto F. Human naive and memory CD4+ T cell repertoires specific for naturally processed antigens analyzed using libraries of amplified T cells. J Exp Med. 2009;206(7):1525–1534. doi:10.1084/jem.20090504.
- Darrah PA, Patel DT, De Luca PM, Lindsay RWB, Davey DF, Flynn BJ, Hoff ST, Andersen P, Reed SG, Morris SL, et al. Multifunctional TH1 cells define a correlate of vaccine-mediated protection against Leishmania major. Nat Med. 2007;13(7):843–850. doi:10.1038/nm1592.
- Ma C, Cheung AF, Chodon T, Koya RC, Wu Z, Ng C, Avramis E, Cochran AJ, Witte ON, Baltimore D, et al. Multifunctional T-cell analyses to study response and progression in adoptive cell transfer immunotherapy. Cancer Discov. 2013;3(4):418–429. doi:10.1158/2159-8290.CD-12-0383.
- Seder RA, Darrah PA, Roederer M. T-cell quality in memory and protection: implications for vaccine design (nature reviews immunology (2008) 8, (247-258)). Nat Rev Immunol. 2008;8(6):486. doi:10.1038/nri2355.
- Trinchieri G. Interleukin-10 production by effector T cells: th1 cells show self control. J Exp Med. 2007;204(2):239–243. https://pubmed.ncbi.nlm.nih.gov/17296790.
- Adkins B, Williamson T, Guevara P, Bu Y. Murine neonatal lymphocytes show rapid early cell cycle entry and cell division. J Immunol 1950;2003:4548–4556.
- Smith NL, Wissink E, Wang J, Pinello JF, Davenport MP, Grimson A, Rudd BD. Rapid proliferation and differentiation impairs the development of memory CD8+ T cells in early life. J Immunol. 2014;193(1):177–184. doi:10.4049/jimmunol.1400553.
- Krummey SM, Cheeseman JA, Conger JA, Jang PS, Mehta AK, Kirk AD, Larsen CP, Ford ML. High CTLA-4 expression on Th17 cells results in increased sensitivity to CTLA-4 coinhibition and resistance to belatacept. Am J Transplant. 2014;14(3):607–614. doi:10.1111/ajt.12600.
- Lingel H, Wissing J, Arra A, Schanze D, Lienenklaus S, Klawonn F, Pierau M, Zenker M, Jänsch L, Brunner-Weinzierl MC, et al. CTLA-4-mediated posttranslational modifications direct cytotoxic T-lymphocyte differentiation. Cell Death Differ. 2017;24(10):1739–1749. doi:10.1038/cdd.2017.102.
- Hebel K, Rudolph M, Kosak B, Chang H-D, Butzmann J, Brunner-Weinzierl MC. IL-1β and TGF-β act antagonistically in induction and differentially in propagation of human proinflammatory precursor CD4+ T cells. J Immunol 1950;2011:5627–5635.
- Kugelberg E. Babies‘ T cells can fight. Nat Rev Immunol. 2014;14(11):714–715. doi:10.1038/nri3758.
- Gibbons D, Fleming P, Virasami A, Michel M-L, Sebire NJ, Costeloe K, Carr R, Klein N, Hayday A. Interleukin-8 (CXCL8) production is a signatory T cell effector function of human newborn infants. Nat Med. 2014;20(10):1206–1210. doi:10.1038/nm.3670.
- Oosterwegel MA, Mandelbrot DA, Boyd SD, Lorsbach RB, Jarrett DY, Abbas AK, Sharpe AH. The role of CTLA-4 in regulating Th2 differentiation. J Immunol. 1999;163:2634–2639.
- Orbach D, Sarnacki S, Brisse HJ, Gauthier-Villars M, Jarreau P-H, Tsatsaris V, Baruchel A, Zerah M, Seigneur E, Peuchmaur M, et al. Neonatal cancer. Lancet Oncol. 2013;14(13):e609–20. doi:10.1016/S1470-2045(13)70236-5.
- Moore SW, Satgé D, Sasco AJ, Zimmermann A, Plaschkes J. The epidemiology of neonatal tumours. Report of an international working group. Pediatr Surg Int. 2003;19(7):509–519. doi:10.1007/s00383-003-1048-8.
- Ward E, DeSantis C, Robbins A, Kohler B, Jemal A. Childhood and adolescent cancer statistics, 2014. Cancer J Clin. 2014;64(2):83–103. doi:10.3322/caac.21219.
- Curtin SC, Minino AM, Anderson RN. Declines in cancer death rates among children and adolescents in the United States, 1999–2014. NCHS Data Brief. 2016;257:1–8.
- Hutzen B, Paudel SN, Naeimi Kararoudi M, Cassady KA, Lee DA, Cripe TP. Immunotherapies for pediatric cancer: current landscape and future perspectives. Cancer Metastasis Rev. 2019;38(4):573–594. doi:10.1007/s10555-019-09819-z.
- Hutzen B, Ghonime M, Lee J, Mardis ER, Wang R, Lee DA, Cairo MS, Roberts RD, Cripe TP, Cassady KA, et al. Immunotherapeutic challenges for pediatric cancers. Mol Ther Oncolytics. 2019;15:38–48. doi:10.1016/j.omto.2019.08.005.
- Park JA, Cheung N-KV. Limitations and opportunities for immune checkpoint inhibitors in pediatric malignancies. Cancer Treat Rev. 2017;58:22–33. doi:10.1016/j.ctrv.2017.05.006.
- Marshall GM, Carter DR, Cheung BB, Liu T, Mateos MK, Meyerowitz JG, Weiss WA. The prenatal origins of cancer. Nat Rev Cancer. 2014;14(4):277–289. doi:10.1038/nrc3679.
- Rudolph M, Hebel K, Miyamura Y, Maverakis E, Brunner-Weinzierl MC. Blockade of CTLA-4 decreases the generation of multifunctional memory CD4+ T cells in vivo. J Immunol. 2011;186(10):5580–5589. doi:10.4049/jimmunol.1003381.
- Hoff H, Knieke K, Cabail Z, Hirseland H, Vratsanos G, Burmester G-R. Surface CD152 (CTLA-4) expression and signaling dictates longevity of CD28 null T cells. J Immunol. 2009;182:5342–5351. doi:10.4049/jimmunol.0801624.
- Pandiyan P, Gärtner D, Soezeri O, Radbruch A, Schulze-Osthoff K, Brunner-Weinzierl MC. CD152 (CTLA-4) determines the unequal resistance of Th1 and Th2 cells against activation-induced cell death by a mechanism requiring PI3 kinase function. J Exp Med. 2004;199:831–842.
- Haanen JBAG, Carbonnel F, Robert C, Kerr KM, Peters S, Larkin J, Jordan K. Management of toxicities from immunotherapy: ESMO clinical practice guidelines for diagnosis, treatment and follow-up. Ann Oncol. 2017;28:iv119–iv142. doi:10.1093/annonc/mdx225.
- Morgado M, Plácido A, Morgado S, Roque F. Management of the adverse effects of immune checkpoint inhibitors. Vaccines. 2020;8(4):8. doi:10.3390/vaccines8040575.
- Arra A, Lingel H, Kuropka B, Pick J, Schnoeder T, Fischer T, Freund C, Pierau M, Brunner-Weinzierl MC. The differentiation and plasticity of Tc17 cells are regulated by CTLA-4-mediated effects on STATs. OncoImmunology. 2017;6(2):e1273300. doi:10.1080/2162402X.2016.1273300.
- Brunner-Weinzierl MC, Rudd CE. CTLA-4 and PD-1 control of T-cell motility and migration: implications for tumor immunotherapy. Front Immunol. 2018;9:2737. doi:10.3389/fimmu.2018.02737.
- Loevenich K, Ueffing K, Abel S, Hose M, Matuschewski K, Westendorf AM. DC-derived IL-10 modulates pro-inflammatory cytokine production and promotes induction of CD4+IL-10+ regulatory T cells during plasmodium yoelii infection. Front Immunol. 2017;8:152. doi:10.3389/fimmu.2017.00152.
- Smith LK, Boukhaled GM, Condotta SA, Mazouz S, Guthmiller JJ, Vijay R, Butler NS, Bruneau J, Shoukry NH, Krawczyk CM, et al. Interleukin-10 directly inhibits CD8+ T cell function by enhancing N-glycan branching to decrease antigen sensitivity. Immunity. 2018;48(299–312.e5):299–312.e5. doi:10.1016/j.immuni.2018.01.006.
- Ejrnaes M, Filippi CM, Martinic MM, Ling EM, Togher LM, Crotty S. Resolution of a chronic viral infection after interleukin-10 receptor blockade. J Exp Med. 2006;203:2461–2472. doi:10.1084/jem.20061462.
- Busse D, La Rosa De M, Hobiger K, Thurley K, Flossdorf M, Scheffold A, Hofer T. Competing feedback loops shape IL-2 signaling between helper and regulatory T lymphocytes in cellular microenvironments. Proc Natl Acad Sci U S A. 2010;107:3058–3063. doi:10.1073/pnas.0812851107.
- Häringer B, Lozza L, Steckel B, Geginat J. Identification and characterization of IL-10/IFN-γ–producing effector-like T cells with regulatory function in human blood. J Exp Med. 2009;206(5):1009–1017. doi:10.1084/jem.20082238.
- Vogel K, Pierau M, Arra A, Lampe K, Schlueter D, Arens C, Brunner-Weinzierl MC. Developmental induction of human T-cell responses against Candida albicans and Aspergillus fumigatus. Sci Rep. 2018;8(1):16904. doi:10.1038/s41598-018-35161-5.
- Breiman L. Random Forests. Mach Learn. 2001;45(1):5–32. doi:10.1023/A:1010933404324.
- Pedregosa F, Varoquaux G, Gramfort A, Michel V, Thirion B, Grisel O. Scikit-learn: machine Learning in Python; 2011. https://arxiv.org/abs/1201.0490 .
- Chinchor N. MUC-4 evaluation metrics. Proceedings of the 4th Conference on Message Understanding. MUC4 ’92; 1992. USA: Association for Computational Linguistics. 22–29.