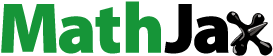
ABSTRACT
Interleukin-1 beta (IL-1β), a pro-inflammatory cytokine, has been ascribed a role in the expansion of myeloid progenitors in acute myeloid leukemia (AML) and in promoting myeloid cell-induced suppression of lymphocyte-mediated immunity against malignant cells. This study aimed at defining the potential impact of IL-1β in the post-remission phase of AML patients receiving immunotherapy for relapse prevention in an international phase IV trial of 84 patients (ClinicalTrials.gov; NCT01347996). Consecutive serum samples were collected from AML patients in first complete remission (CR) who received cycles of relapse-preventive immunotherapy with histamine dihydrochloride (HDC) and low-dose interleukin-2 (IL-2). Low IL-1β serum levels before and after the first HDC/IL-2 treatment cycle favorably prognosticated leukemia-free survival and overall survival. Serum levels of IL-1β were significantly reduced in patients receiving HDC/IL-2. HDC also reduced the formation of IL-1β from activated human PBMCs in vitro. Additionally, high serum levels of the IL-1 receptor antagonist IL-1RA were associated with favorable outcome, and AML patients with low IL-1β along with high IL-1RA levels were strikingly protected against leukemic relapse. Our results suggest that strategies to target IL-1β might impact on relapse risk and survival in AML.
Introduction
Acute myeloid leukemia (AML) is characterized by a rapid accumulation of immature myeloid cells in bone marrow. Patients initially receive cycles of induction chemotherapy aiming at attaining microscopic disappearance of leukemic cells and the subsequent re-appearance of normal hematopoiesis, defined as complete remission (CR). Despite ensuing consolidation chemotherapy, the majority of patients who have achieved CR will experience relapse of leukemia within 2–3 years, which significantly explains why the long-term survival for adult AML patients remains in the range of 20–40%.Citation1
The shortage of efficacious strategies to prevent relapse in the post-remission phase remains a clinical challenge. AML patients with disease features that herald poor prognosis may be offered allogeneic stem cell transplantation, which prevents relapse but entails significant morbidity and mortality.Citation2 Non-transplanted older AML patients benefit from post-remission therapy with DNA methylation inhibitors.Citation3,Citation4 Furthermore, immunotherapy with the NOX2 inhibitor histamine dihydrochloride (HDC) in conjunction with low doses of the NK cell and T cell stimulatory cytokine interleukin-2 (IL-2) reduces the relapse risk in younger AML patients.Citation5,Citation6,Citation7 and is approved for relapse prevention throughout the European Union (EU).
Cytokines and growth factors secreted in the bone marrow microenvironment modulate leukemic cell survival, proliferation, and differentiation.Citation8 Accordingly, the pro-inflammatory cytokine IL-1β has been implicated in the proliferation of AML cells and in causing resistance of AML cells to apoptosis.Citation9,Citation10,Citation11,Citation12 Carey et al. utilized primary AML patient samples and identified IL-1β as a significant enhancer of leukemic cell growth 11. IL-1β was also reported to enhance the clonogenic activity of AML cells, but to inhibit the self-renewal capacity of normal hematopoietic stem cells Citation11 Citation12. Furthermore, IL-1β stimulates the formation of stem cell factor (SCF) by epithelial cells and bone marrow stromal cells, which promotes expansion of leukemic cells.Citation13,Citation14,Citation15 AML patients show increased levels of IL-1β in peripheral blood and in bone marrow cells.Citation11,Citation16
IL-1β is produced by myeloid cells after activation of Toll-like receptors or retinoic acid-inducible gene-like receptors.Citation17,Citation18,Citation19 It is synthesized as a precursor protein, pro-IL-1β, that may be cleaved into mature IL-1β by caspase-1 within an activated inflammasome.Citation20 Mature IL-1β exerts pro-inflammatory effects by binding to the IL-1 receptor type 1 (IL-1R1) that allows its interaction with the co-receptor IL-1R3 with subsequent transcription of inflammatory target genes.Citation21 The downstream effects of IL-1β are modulated by the IL-1 receptor antagonist (IL-1RA), which is an endogenous antagonist to IL-1β that competitively binds IL-1R1 and prevents downstream signaling.Citation21
The interest in IL-1β in the context of cancer immunology and immunotherapy was sparked by the CANTOS study, which was originally designed to uncover the potential influence of IL-1β for the clinical course of atherosclerosis-related morbidity and mortality.Citation22 In the CANTOS study, the administration of a mAb against IL-1β caused a pronounced reduction of the incidence of lung cancer.Citation22 These and other results in clinical and preclinical settings support that IL-1β promotes tumor progression, presumably by promoting inflammation and by sustaining and enhancing immunosuppressive functions of myeloid cells.Citation23,Citation24 The actions of IL-1β in immune surveillance against malignant cells are, however, context-dependentCitation25,Citation26 and the influence of IL-1β and its interaction with IL-1RA on relapse risk and survival in AML is not known.
With this background, we sought to define the potential impact of IL-1β and IL-1RA on the course of disease in AML patients receiving combinatorial relapse-preventive immunotherapy with the NOX2-inhibitor HDC and low-dose IL-2, an activator of T cell and NK cell antitumor immunity. Our results suggest that IL-1β may be targeted for relapse prevention and improved survival in AML.
Materials and methods
Patients, study design, and objectives
Eighty-four adult AML patients in first CR were enrolled to this single-armed multicenter phase IV study (Re:Mission, NCT01347996, registered at www.clinicaltrials.gov). The patients received 10 consecutive three-week cycles of HDC (Ceplene R, 0.5 mg bid s.c.) in conjunction with low-dose IL-2 (1MIU bid s.c.), with three- or six-week rest periods in-between cycles. Patients were monitored for at least two years, or until relapse or death. Relapse was defined as at least 5% blast cells in the bone marrow or the presence of extramedullary leukemia. The dosage, route of administration, exclusion criteria etc. were identical to those described for a previous phase III trial 5. Three patients withdrew consent and were not included in any analysis. Serum samples were available from 78 patients prior to immunotherapy, 73 patients after the first cycle of immunotherapy, 55 patients prior to and 53 patients after the third cycle of immunotherapy. The herein reported analyses of IL-1β and IL-1RA vs. leukemia-free survival (LFS) and overall survival (OS) were performed post-hoc. LFS was defined as the time in days from start of immunotherapy with HDC/IL-2 to relapse or death from any cause, and OS was defined as the corresponding time to death. summarizes patient characteristics. More details regarding the patients, including previous induction and consolidation therapy are accounted for in preceding reports.Citation27,Citation28,Citation29,Citation30 This study was conducted according to the Declaration of Helsinki principles. The trial was approved by the Ethical Committees of each participating institution, approval number 267–09 by the regional ethics committee in Gothenburg. All patients gave written informed consent before enrollment.
Table 1. Patient characteristics
Serum collection and cytokine analysis
Peripheral blood from patients was drawn before and after the first and third three-week HDC/IL-2 treatment cycles, i.e. cycle one, day one (C1D1), cycle one, day 21 (C1D21), cycle three, day one (C3D1) and cycle three, day 21 (C3D21). The peripheral blood was collected into Vacuette® tubes (Greiner Bio-One, #455,009) that were centrifuged within 2 h for 10 min at 2000 g. Serum was then recovered and immediately frozen at local sites. The serum samples were shipped on dry ice to the Tumor Immunology Laboratory in Gothenburg for analysis. Serum was recovered from peripheral blood of seven healthy donors using the same method. The sera were analyzed with a 27-plex cytokine panel (Bio-Plex™ Pro Human Cytokine Standard 27-Plex Group 1, Bio-Rad, #M500KCAF0Y) utilizing Luminex technology according to the manufacturer’s protocol. In brief, magnetic color-coded beads coated with antibodies against the cytokines were added to each well of the assay plates. After washing, samples and standards were added to the wells and plates were incubated for 30 minutes, washed and incubated for another 30 minutes with detection antibodies. After washing, plates were incubated with streptavidin-phycoerythrin for 10 min followed by washing and addition of 125µl assay buffer/well. The content of cytokines was measured using a Bio-Plex™ 200 System (Bio-Rad).
Preparation of PBMC
Buffy coats obtained from healthy blood donors were mixed 1:1 with 2% dextran. After sedimentation of erythrocytes, peripheral blood mononuclear cells (PBMCs) were separated by Ficoll-Paque (Lymphoprep, Nycomed, Oslo, Norway) density centrifugation. PBMCs were cultured overnight in Iscoves’ medium supplemented with 10% human AB serum, in the presence or absence of 0.5 µg/ml LPS (Sigma-Aldrich), 100 µM HDC (Sigma-Aldrich), 100 nM diphenylene iodonium (DPI; Sigma-Aldrich) or 500 U/ml IL-2 (Proleukin, Chiron). After 24 h, supernatants were collected and assayed for IL-1β and IL-1RA using ELISA (R&D systems, DY201, and R&D systems, DY280-05, respectively) according to the manufacturer’s instructions.
Statistics
Changes in IL-1β and IL-1RA serum levels before and after HDC/IL-2 therapy were analyzed using Wilcoxon matched pairs test, and by the Mann–Whitney test for levels of these mediators in serum from patients and healthy donors. The impact of HDC, IL-2 and DPI on LPS-induced IL-1β and IL-1RA production from PBMCs, was determined by Mixed-effects analysis followed by Holm-Sidak´s multiple comparisons test. Student’s paired t-test was used to determine direct effects of HDC or IL-2 on cytokine production from PBMCs. Asterisks are used as follows: * p < .05; **, p < .01; and ***, p < .001. All indicated P values are two-sided. The logrank test was utilized to determine the impact of IL-1β and IL-1RA levels in serum, before or after immunotherapy, on LFS and OS. Logrank test for trend was used to analyze the combined impact of low IL-1β and high IL-1RA serum levels on LFS and OS. The impact of cytokine levels in serum on LFS and OS was further analyzed using Cox univariate and multivariable regression analyses. Cox univariable regression analyses of the impact of age, risk group classification, number of induction courses required to achieve CR, and number of consolidation courses given on LFS and OS, defined age and number of induction courses as prognostic factors achieving a p value of below 0.1. These were included as potential confounders in the Cox multivariable regression analysis.
Results
Serum levels of IL-1β are reduced in AML patients during HDC/IL-2 immunotherapy
Serum samples, obtained from the peripheral blood of AML patients in first CR before and after the first and the third HDC/IL-2 treatment cycle, were analyzed for content of IL-1β and IL-1RA. Levels of IL-1β were significantly reduced during the first treatment cycle and remained reduced at later time points ()). No significant changes were observed in the serum levels of IL-1RA following treatment with HDC/IL-2 ()). Serum levels of IL-1β and IL-1RA were also analyzed in seven healthy donors. Levels of IL-1β were lower in healthy donors compared with levels in AML patients in the post-consolidation phase immediately prior to onset of treatment with HDC/IL-2 (median 0 pg/ml, range 0–0.38 vs median 0.58, range 0–21.3; p = .014, Mann–Whitney test). In contrast, the serum levels of IL-1RA were higher in healthy donors compared with pre-treatment AML patients in CR (median 307 pg/ml, range 176–789 vs median 67, range 3–4366; p < .001, Mann–Whitney test).
Figure 1. Serum IL-1βlevels are reduced following HDC/IL-2 immunotherapy. Serum levels of IL-1β (a) and IL-1RA (b) before (D1) and after (D21) cycles 1 (C1) and 3 (C3) of HDC/IL-2 immunotherapy (C1D1 n = 78, C1D21 n = 73, C3D1 n = 55, C3D21 n = 53; Wilcoxon-matched pairs test; * p < .05, ** p < .01). The production of IL-1β (c) and IL-1RA (d) by PBMCs stimulated with 0.5 µg/ml LPS in the presence or absence of HDC (100 µM), DPI (100 nM) or IL-2 (500 U/ml). Ctrl, LPS, LPS+HDC; n = 7, LPS+DPI and LPS+IL-2; n = 5; Mixed-effects analysis followed by Holm-Sidak’s multiple comparisons test; * p < .05, ** p < .01, *** p < .001
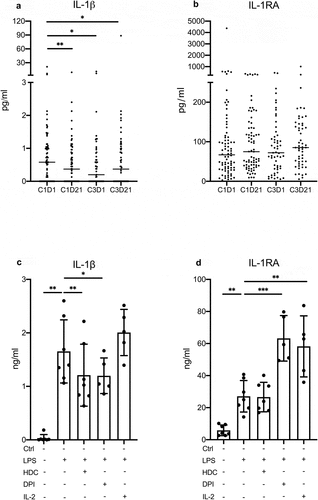
HDC suppresses LPS-induced production of IL-1β from PBMCs
To determine if HDC or IL-2 affected IL-1β production from myeloid cells in vitro, human PBMCs were stimulated with the Toll-like receptor 4 agonist LPS in the presence or absence of HDC or IL-2. HDC, but not IL-2, was found to significantly suppress LPS-induced IL-1β production ()). Reactive oxygen species (ROS) have been implicated as key signaling molecules downstream of the inflammasome.Citation31,Citation32 As HDC is a NOX2-inhibitor that reduces ROS formation in myeloid cells,Citation33 we also determined effects of the NOX-inhibitor DPI on IL-1β production from PBMC. DPI significantly inhibited LPS-induced IL-1β production to a similar degree as did HDC ()). We also analyzed the effect of HDC, IL-2 and DPI on LPS-induced IL-1RA production. HDC did not alter the production of IL-1RA from PBMCs, while both IL-2 and DPI enhanced LPS-induced IL-1RA levels ()). Unstimulated PBMCs produced very low levels of IL-1β and IL-1RA that were not altered by the addition of HDC (data not shown). IL-2 did not affect IL-1β production, but triggered a slight induction of IL-1RA from unstimulated PBMC (Ctrl 5,8 1,2 ng/ml vs IL-2 12
1,9 ng/ml (mean
SEM), p = .013, n = 5, Student’s paired t-test).
Low IL-1β and high IL-1RA levels in serum are associated with favorable outcome of AML
While IL-1β has been implicated in clonal expansion of AML cells,Citation9,Citation10,Citation11,Citation12 the impact of IL-1β in the post-chemotherapy phase of AML is not known. We asked if IL-1β serum levels impacted on relapse risk and survival in this patient cohort of AML patients receiving relapse-preventive immunotherapy. Low IL-1β levels, both before and after the first HDC/IL-2 treatment cycle, predicted prolonged LFS and OS (), Supplementary Figure 1a-b). The favorable impact of low IL-1β levels on LFS remained significant in multivariable Cox regression analysis (C1D1 LFS: HR = 1.09: CI = 1.01–1.17, p = .035; C1D21 LFS: HR = 1.27: CI = 1.06–1.53, p = .011). Furthermore, patients with high levels of the natural IL-1β antagonist, IL-1RA, after the first treatment cycle displayed prolonged LFS and OS () with similar trend was observed prior to immunotherapy (Supplementary Figure 1c-d). Patients with low IL-1β serum levels in conjunction with high IL-1RA levels showed the most favorable outcome. Patients with either low IL-1β or high IL-1RA levels showed intermediate outcome, and patients with high IL-1β and low IL-1RA levels showed the poorest outcome (), Supplementary Figure 1e-f).
Figure 2. Low IL-1βand high IL-1RA serum levels after immunotherapy are associated with a favorable outcome in AML. (a-d) Patients dichotomized based on below or above 0.6 pg/ml of IL-1β (a, b) or 66 pg/ml IL-1RA (c-d) in serum after the first cycle of immunotherapy were analyzed for leukemia-free survival (a, c) or overall survival (b, d) by the log-rank test. (e-f) Patients were separated into three groups where the first constituted patients with low (<0.6 pg/ml) IL-1β levels along with high (>66 pg/ml) IL-1RA levels in serum after the first cycle of immunotherapy (IL-1β low and IL-1RA high, black line, n = 30), the second constituted patients with high IL-1β levels along with low IL-1RA levels (IL-1β high and IL-1RA low, blue line, n = 24), and the remaining patients were in the third group (IL-1β low or IL-1RA high, red line, n = 19). The leukemia-free survival (e) and overall survival (f) of patients in the three groups were analyzed by the log-rank test for trend
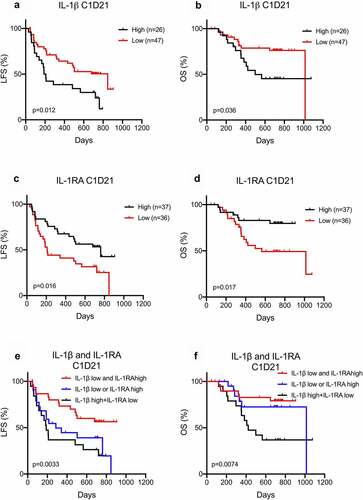
Discussion
The majority of AML patients achieve CR after induction chemotherapy. Despite ensuing rounds of consolidation chemotherapy, relapse in the post-consolidation phase is common and relapse-preventive strategies are highly warranted. For older AML patients, post-remission therapy with azacitidine is a viable option.Citation3,Citation4 In addition, post-consolidation immunotherapy with HDC/IL-2 reduces the risk of leukemic relapse, in particular in younger adults (<60 years old).Citation5 The proposed mechanism of action of the HDC component of this regimen is to reduce the formation of immunosuppressive NOX2-derived ROS from myeloid cells to allow for optimal induction of anti-leukemic properties of NK cells and cytotoxic T cells by the IL-2 component. As NK cells and T cells are highly sensitive to the immunosuppressive actions of ROS, HDC and IL-2 are assumed to act in synergy to enhance the ability of cytotoxic lymphocytes to eradicate residual leukemic cells.Citation6,Citation7,Citation34,Citation35 Administration of HDC/IL-2 activates NK cell- and T cell-mediated immunity in AML patients and correlative analyses support that these aspects of immune regulation are critical for the reduction of relapse risk.Citation27,Citation28,Citation29,Citation30,Citation36,Citation37 Further understanding of the immunomodulatory effects of HDC/IL-2 may translate into alternative or supplementary strategies for improved immunotherapy in AML.
Histamine, which is the endogenous counterpart to HDC, has been ascribed multiple effects on the production of cytokines from immune and inflammatory cells (reviewed in[38]). Histamine has thus been shown to modulate the formation of tumor necrosis factor (TNF), IL-1, IL-6, IL-10, IL-12 and IL-17 by leukocytes.Citation38,Citation39 Effects of histamine on cytokine production are determined by the cellular distribution and expression of subtypes of histamine receptors and may additionally encompass indirect effects on receptor expression with ensuing effects of cytokine production.Citation38,Citation40 Although histamine is well known as a mediator of allergic inflammation, mainly via its vascular effects downstream of the histamine type 1 receptor, several studies also shown that histamine may dampen myeloid cell-induced inflammation.Citation34,Citation41,Citation42 These anti-inflammatory actions of histamine, including reduced NOX2-dependent ROS formation and reduced production of IL-12, TNF and IL-1 by myeloid cells, are typically mediated via histamine-type 2 receptors.Citation34,Citation38,Citation39,Citation43 The suggested anti-inflammatory role of histamine is bolstered by results achieved in genetically histamine-deficient mice, which are characterized by markedly increased myeloid cell-related inflammation along with increased growth of inflammation-induced cancer.Citation44
IL-1β is a central mediator of inflammation with a myriad of effects on different tissues and immune cells. It is considered to promote myeloid cell-induced inflammation,Citation23,Citation24 trigger the expression of adhesion molecules on endothelial cells,Citation45 induce the production of IL-17 by γδT cells, polarize T cells to the Th17 phenotype, and to induce the acute-phase reaction.Citation46,Citation47,Citation48 In an acute setting, many of these effects are associated with an adequate immune response. However, long-term chronic IL-1β signaling may contribute to cancer-associated inflammation and immune suppression. Our finding that systemic levels of IL-1β were sustainably reduced during treatment of AML patients with HDC/IL-2 may thus reflect a potentially important aspect of histamine-mediated immunomodulation. Of note, hypomethylating agents (HMA), that are approved for relapse prevention in older AML patients in the post-consolidation phase, enhance the expression of several genes by removal of methyl groups in promotor regions.Citation49,Citation50 Hypomethylation of the promotor region of IL-1β as well as IL-1RA is reported to enhance the expression of these mediators.Citation36,Citation51 Treatment of MDS patients with azacitidine did, however, not trigger significant induction of IL-1β Citation50, and it remains to be defined if HMA therapy affects IL-1β and IL-1RA levels in AML patients, or if levels of these mediators correlate to clinical outcome in HMA-treated patients.
ROS are pivotal second messengers in inflammasome activation, and thus the formation of functional IL-1β.Citation31,Citation32 The NOX2 enzyme constitutes a major source of ROS in myeloid cells.Citation52 We observed an inhibition of activation-induced IL-1β production from PBMC by HDC or DPI, which is in accordance with previous studies that suggest a role for NOX2-derived ROS in regulating IL-1β.Citation53 Our in vitro results thus support that the HDC component accounted for the observed reduction of IL-1β during HDC/IL-2 treatment in vivo. The details of histaminergic regulation of IL-1β remain to be defined, but our results imply that NOX2-related inflammation encompasses the formation and/or release of IL-1β.Citation54,Citation55 that may be targeted by HDC. In our in vitro experiments, IL-1β expression was triggered by the TLR-4 agonist LPS. Notably, other TLR ligands such as high mobility group box 1 (HMGB1) are increased in the serum of AML patients and have been proposed to stimulate inflammasome activation in AML.Citation56
IL-1β exerts its immunoregulatory and pro-inflammatory functions via IL-1R1 to stimulate MyD88-mediated induction of NF-κB and expression of multiple target genes including cytokines, chemokines and adhesion molecules.Citation57 IL-1β signaling is competitively inhibited by IL-1RA, which is a natural antagonist at IL-1R1 21. IL-2, but not HDC, was found to boost IL-1RA production from in vitro stimulated PBMCs. The levels of IL-1RA were, however, not significantly modulated in patients receiving HDC/IL-2 treatment. We speculate that the systemic levels of IL-2 achieved using the low-dose regiment of IL-2 were below those required to trigger measurable IL-1RA production in vivo. It was also observed that the NOX inhibitor DPI boosted IL-1RA formation in myeloid cells. As this effect was not shared by the NOX2 inhibitor HDC, we assume that effects of DPI that are unrelated to NOX2.Citation58,Citation59 may be responsible for the observed IL-1RA induction.
A main outcome of this study was that systemic levels of IL-1β prognosticated relapse and overall survival in patients receiving relapse-preventive immunotherapy. This novel finding coheres with earlier studies suggesting that the targeting of IL-1β, by use of monoclonal antibodies, reduces the incidence of lung cancer in high-risk patients.Citation60 Our results merit studies to explore the potential impact of IL-1β-reductive therapies for relapse prevention in AML. Additionally, high serum levels of IL-1RA were associated with prolonged survival. We also found that AML patients in CR had higher IL-1β serum levels, but lower IL-1RA serum levels compared with healthy donors. AML patients with low serum levels of IL-1β in conjunction with high levels of IL-1RA showed very favorable outcome, indicating that signaling via IL-1R1 may be associated with unfavorable outcome in the post-consolidation phase of AML. We recognize that further studies are warranted to confirm this hypothesis. We also envisage future studies to broadly clarify the impact of IL-1β signaling for relapse-free survival in AML in the absence of immunotherapy.
Disclosure Statement
Authors HGW, KH and AM hold issued or pending patents protecting the use of NOX2 inhibitors in cancer. The other authors declare no potential conflicts of interest.
Supplementary material
Supplemental data for this article can be accessed on the publisher’s website.
Additional information
Funding
References
- Bertoli S, Tavitian S, Huynh A, Borel C, Guenounou S, Luquet I, Delabesse E, Sarry A, Laurent G, Attal M, et al Improved outcome for AML patients over the years 2000-2014. Blood Cancer J. 2017;7(12):p. 635. doi:10.1038/s41408-017-0011-1.
- Döhner H, et al.. Diagnosis and management of AML in adults: 2017 ELN recommendations from an international expert panel. Blood. 2017;129(4):p. 424–9.
- Huls G, Chitu DA, Havelange V, Jongen-Lavrencic M, van de Loosdrecht AA, Biemond BJ, Sinnige H, Hodossy B, Graux C, Kooy RVM, et al.. Azacitidine maintenance after intensive chemotherapy improves DFS in older AML patients. Blood. 2019;133(13):1457–1464. doi:10.1182/blood-2018-10-879866.
- Wei AH, et al.. The QUAZAR AML-001 Maintenance trial: results of a phase III international, randomized, double-blind, placebo-controlled study of CC-486 (oral formulation of azacitidine) in patients with acute myeloid leukemia (AML) in first remission. Washington (DC): American Society of Hematology; 2019
- Brune M, et al.. Improved leukemia-free survival after postconsolidation immunotherapy with histamine dihydrochloride and interleukin-2 in acute myeloid leukemia: results of a randomized phase 3 trial. Blood. 2006;108(1):88–96. doi:10.1182/blood-2005-10-4073.
- Martner A, Thorén FB, Aurelius J, Söderholm J, Brune M, Hellstrand K. Immunotherapy with histamine dihydrochloride for the prevention of relapse in acute myeloid leukemia. Expert Rev Hematol. 2010;3(4):381–391. doi:10.1586/ehm.10.30.
- Martner A, Thorén FB, Aurelius J, Hellstrand K. Immunotherapeutic strategies for relapse control in acute myeloid leukemia. Blood Rev. 2013;27(5):209–216. doi:10.1016/j.blre.2013.06.006.
- Binder S, Luciano M, Horejs-Hoeck J. The cytokine network in acute myeloid leukemia (AML): a focus on pro- and anti-inflammatory mediators. Cytokine Growth Factor Rev. 2018;43:8–15. doi:10.1016/j.cytogfr.2018.08.004.
- Turzanski J, et al.. Interleukin-1beta maintains an apoptosis-resistant phenotype in the blast cells of acute myeloid leukaemia via multiple pathways. Leukemia. 2004;18(10):1662–1670. doi:10.1038/sj.leu.2403457.
- Bruserud O. Effects of endogenous interleukin 1 on blast cells derived from acute myelogenous leukemia patients. Leuk Res. 1996;20(1):65–73. doi:10.1016/0145-2126(95)00112-3.
- Carey A, Edwards DK, Eide CA, Newell L, Traer E, Medeiros BC, Pollyea DA, Deininger MW, Collins RH, Tyner JW, et al.. Identification of interleukin-1 by functional screening as a key mediator of cellular expansion and disease progression in acute myeloid leukemia. Cell Rep. 2017;18(13):3204–3218. doi:10.1016/j.celrep.2017.03.018.
- Wang Y, Sun X, Yuan S, Hou S, Guo T, Chu Y, Pang T, Luo HR, Yuan W, Wang X, et al.. Interleukin-1β inhibits normal hematopoietic expansion and promotes acute myeloid leukemia progression via the bone marrow niche. Cytotherapy. 2020;22(3):127–134. doi:10.1016/j.jcyt.2020.01.001.
- Broudy VC. Stem cell factor and hematopoiesis. Blood. 1997;90:1345–1364.
- Wyszynski RW, Gibbs BF, Varani L, Iannotta D, Sumbayev VV. Interleukin-1 beta induces the expression and production of stem cell factor by epithelial cells: crucial involvement of the PI-3K/mTOR pathway and HIF-1 transcription complex. Cell Mol Immunol. 2016;13(1):47–56. doi:10.1038/cmi.2014.113.
- Lee SJ, Yoon JH, Song KS. Chrysin inhibited stem cell factor (SCF)/c-Kit complex-induced cell proliferation in human myeloid leukemia cells. Biochem Pharmacol. 2007;74(2):215–225. doi:10.1016/j.bcp.2007.04.011.
- Tao M, et al.. SCF, IL-1beta, IL-1ra and GM-CSF in the bone marrow and serum of normal individuals and of AML and CML patients. Cytokine. 2000;12(6):699–707. doi:10.1006/cyto.2000.0666.
- Fields JK, Günther S, Sundberg EJ. Structural basis of IL-1 family cytokine signaling. Front Immunol. 2019;10:1412. doi:10.3389/fimmu.2019.01412.
- Dinarello CA. Overview of the IL-1 family in innate inflammation and acquired immunity. Immunol Rev. 2018;281:8–27.
- Loo YM, Gale M Jr.. Immune signaling by RIG-I-like receptors. Immunity. 2011;34(5):680–692. doi:10.1016/j.immuni.2011.05.003.
- Franchi L, Eigenbrod T, Muñoz-Planillo R, Nuñez G. The inflammasome: a caspase-1-activation platform that regulates immune responses and disease pathogenesis. Nat Immunol. 2009;10(3):241–247. doi:10.1038/ni.1703.
- Schreuder H, Tardif C, Trump-Kallmeyer S, Soffientini A, Sarubbi E, Akeson A, Bowlin T, Yanofsky S, Barrett RW. A new cytokine-receptor binding mode revealed by the crystal structure of the IL-1 receptor with an antagonist. Nature. 1997;386(6621):194–200. doi:10.1038/386194a0.
- Ridker PM, Everett BM, Thuren T, MacFadyen JG, Chang WH, Ballantyne C, Fonseca F, Nicolau J, Koenig W, Anker SD, et al.. Antiinflammatory therapy with canakinumab for atherosclerotic disease. N Engl J Med. 2017;377(12):1119–1131. doi:10.1056/NEJMoa1707914.
- Kaplanov I, Carmi Y, Kornetsky R, Shemesh A, Shurin GV, Shurin MR, Dinarello CA, Voronov E, Apte RN. Blocking IL-1β reverses the immunosuppression in mouse breast cancer and synergizes with anti–PD-1 for tumor abrogation. Proc Natl Acad Sci U S A. 2019;116(4):1361–1369. doi:10.1073/pnas.1812266115.
- Mantovani A, Dinarello CA, Molgora M, Garlanda C. Interleukin-1 and related cytokines in the regulation of inflammation and immunity. Immunity. 2019;50(4):778–795. doi:10.1016/j.immuni.2019.03.012.
- Lee P-H, Yamamoto TN, Gurusamy D, Sukumar M, Yu Z, Hu-Li J, Kawabe T, Gangaplara A, Kishton RJ, Henning AN, et al.. Host conditioning with IL-1β improves the antitumor function of adoptively transferred T cells. J Exp Med. 2019;216(11):2619–2634. doi:10.1084/jem.20181218.
- Aggen DH, Ager CR, Obradovic AZ, Chowdhury N, Ghasemzadeh A, Mao W, Chaimowitz MG, Lopez-Bujanda ZA, Spina CS, Hawley JE, et al.. Blocking IL1 beta promotes tumor regression and remodeling of the myeloid compartment in a renal cell carcinoma model: multidimensional analyses. Clin Cancer Res. 2021;27(2):608–621. doi:10.1158/1078-0432.CCR-20-1610.
- Sander FE, Rydström A, Bernson E, Kiffin R, Riise R, Aurelius J, Anderson H, Brune M, Foà R, Hellstrand K, et al.. Dynamics of cytotoxic T cell subsets during immunotherapy predicts outcome in acute myeloid leukemia. Oncotarget. 2016;7(7):7586–7596. doi:10.18632/oncotarget.7210.
- Martner A, Rydström A, Riise RE, Aurelius J, Anderson H, Brune M, Foà R, Hellstrand K, Thorén FB. Role of natural killer cell subsets and natural cytotoxicity receptors for the outcome of immunotherapy in acute myeloid leukemia. Oncoimmunology. 2016;5(1):e1041701. doi:10.1080/2162402X.2015.1041701.
- Martner A, Rydström A, Riise RE, Aurelius J, Brune M, Foà R, Hellstrand K, Thorén FB. NK cell expression of natural cytotoxicity receptors may determine relapse risk in older AML patients undergoing immunotherapy for remission maintenance. Oncotarget. 2015;6(40):42569–42574. doi:10.18632/oncotarget.5559.
- Sander FE, et al.. Role of regulatory T cells in acute myeloid leukemia patients undergoing relapse-preventive immunotherapy. Cancer Immunol Immunother. 2017;66(4):1473–1484. doi:10.1007/s00262-017-2040-9.
- Bai B, Yang Y, Wang Q, Li M, Tian C, Liu Y, Aung LHH, Li P-F, Yu T, Chu X-M, et al.. NLRP3 inflammasome in endothelial dysfunction. Cell Death Dis. 2020;11(9):776. doi:10.1038/s41419-020-02985-x.
- Tschopp J, Schroder K. NLRP3 inflammasome activation: the convergence of multiple signalling pathways on ROS production? Nat Rev Immunol. 2010;11(9):210–215. doi:10.1038/nri2725.
- Martner A, Wiktorin HG, Lenox B, Ewald Sander F, Aydin E, Aurelius J, Thorén FB, Ståhlberg A, Hermodsson S, Hellstrand K, et al.. Histamine promotes the development of monocyte-derived dendritic cells and reduces tumor growth by targeting the myeloid NADPH oxidase. J Immunol. 2015;194(10):5014–5021. doi:10.4049/jimmunol.1402991.
- Hellstrand K, Asea A, Dahlgren C, Hermodsson S. Histaminergic regulation of NK cells. Role of monocyte-derived reactive oxygen metabolites. J Immunol. 1994;153:4940–4947.
- Asea A, Hermodsson S, Hellstrand K. Histaminergic regulation of natural killer cell-mediated clearance of tumour cells in mice. Scand J Immunol. 1996;43(1):9–15. doi:10.1046/j.1365-3083.1996.d01-14.x.
- Hallner A, Bernson E, Hussein BA, Ewald Sander F, Brune M, Aurelius J, Martner A, Hellstrand K, Thorén FB. The HLA-B −21 dimorphism impacts on NK cell education and clinical outcome of immunotherapy in acute myeloid leukemia. Blood. 2019;133(13):1479–1488. doi:10.1182/blood-2018-09-874990.
- Bernson E, Hallner A, Sander FE, Nicklasson M, Nilsson MS, Christenson K, Aydin E, Liljeqvist J-Å, Brune M, Foà R, et al.. Cytomegalovirus serostatus affects autoreactive NK cells and outcomes of IL2-based immunotherapy in acute myeloid leukemia. Cancer Immunol Res. 2018;6(9):1110–1119. doi:10.1158/2326-6066.CIR-17-0711.
- Branco A, Yoshikawa FSY, et al.. Role of histamine in modulating the immune response and inflammation. Mediators Inflamm. 2018;2018:9524075. Pietrobon, A J., Sato, M N.. doi:10.1155/2018/9524075.
- Dohlsten M, et al.. Histamine inhibits interleukin 1 production by lipopolysaccharide-stimulated human peripheral blood monocytes. Scand J Immunol. 1988;27(5):527–532. doi:10.1111/j.1365-3083.1988.tb02379.x.
- Nishibori M, Takahashi HK, Mori S. The regulation of ICAM-1 and LFA-1 interaction by autacoids and statins: a novel strategy for controlling inflammation and immune responses. J Pharmacol Sci. 2003;92(1):7–12. doi:10.1254/jphs.92.7.
- Mellqvist UH, Hansson M, Brune M, Dahlgren C, Hermodsson S, Hellstrand K. Natural killer cell dysfunction and apoptosis induced by chronic myelogenous leukemia cells: role of reactive oxygen species and regulation by histamine. Blood. 2000;96(5):1961–1968. doi:10.1182/blood.V96.5.1961.
- Aydin E, Johansson J, Nazir FH, Hellstrand K, Martner A. Role of NOX2-derived reactive oxygen species in NK cell-mediated control of murine melanoma metastasis. Cancer Immunol Res. 2017;5(9):804–811. doi:10.1158/2326-6066.CIR-16-0382.
- Frei R, Ferstl R, Konieczna P, Ziegler M, Simon T, Rugeles TM, Mailand S, Watanabe T, Lauener R, Akdis CA, et al.. Histamine receptor 2 modifies dendritic cell responses to microbial ligands. J Allergy Clin Immunol. 2013;132(1):194–204. doi:10.1016/j.jaci.2013.01.013.
- Yang XD, Ai W, Asfaha S, Bhagat G, Friedman RA, Jin G, Park H, Shykind B, Diacovo TG, Falus A, et al.. Histamine deficiency promotes inflammation-associated carcinogenesis through reduced myeloid maturation and accumulation of CD11b+Ly6G+ immature myeloid cells. Nat Med. 2011;17(1):87–95. doi:10.1038/nm.2278.
- Haraldsen G, Kvale D, Lien B, Farstad IN, Brandtzaeg P. Cytokine-regulated expression of E-selectin, intercellular adhesion molecule-1 (ICAM-1), and vascular cell adhesion molecule-1 (VCAM-1) in human microvascular endothelial cells. J Immunol. 1996;156:2558–2565.
- Sutton CE, Lalor SJ, Sweeney CM, Brereton CF, Lavelle EC, Mills KHG. Interleukin-1 and IL-23 induce innate IL-17 production from gammadelta T cells, amplifying Th17 responses and autoimmunity. Immunity. 2009;31(2):331–341. doi:10.1016/j.immuni.2009.08.001.
- Veldhoen M, Hocking RJ, Atkins CJ, Locksley RM, Stockinger B. TGFbeta in the context of an inflammatory cytokine milieu supports de novo differentiation of IL-17-producing T cells. Immunity. 2006;24(2):179–189. doi:10.1016/j.immuni.2006.01.001.
- Kramer F, Torzewski J, Kamenz J, Veit K, Hombach V, Dedio J, Ivashchenko Y. Interleukin-1beta stimulates acute phase response and C-reactive protein synthesis by inducing an NFkappaB- and C/EBPbeta-dependent autocrine interleukin-6 loop. Mol Immunol. 2008;45(9):2678–2689. doi:10.1016/j.molimm.2007.12.017.
- Kordella C, Lamprianidou E, Kotsianidis I. Mechanisms of action of hypomethylating agents: endogenous retroelements at the epicenter. Front Oncol. 2021;11:650473. doi:10.3389/fonc.2021.650473.
- Moudra A, Hubackova S, Machalova V, Vancurova M, Bartek J, Reinis M, Hodny Z, Jonasova A. Dynamic alterations of bone marrow cytokine landscape of myelodysplastic syndromes patients treated with 5-azacytidine. Oncoimmunology. 2016;5(10):e1183860. doi:10.1080/2162402X.2016.1183860.
- Hashimoto K, Oreffo ROC, Gibson MB, Goldring MB, Roach HI. DNA demethylation at specific CpG sites in the IL1B promoter in response to inflammatory cytokines in human articular chondrocytes. Arthritis Rheum. 2009;60(11):3303–3313. doi:10.1002/art.24882.
- Martner A, Aydin E, Hellstrand K. NOX2 in autoimmunity tumor growth and metastasis. J Pathol. 2019;247(2):151–154. doi:10.1002/path.5175.
- Singh A, Singh V, Tiwari RL, Chandra T, Kumar A, Dikshit M, Barthwal MK. The IRAK-ERK-p67phox-Nox-2 axis mediates TLR4, 2-induced ROS production for IL-1β transcription and processing in monocytes. Cell Mol Immunol. 2016;13(6):745–763. doi:10.1038/cmi.2015.62.
- Dostert C, Petrilli V, Van Bruggen R, Steele C, Mossman BT, Tschopp J. Innate immune activation through Nalp3 inflammasome sensing of asbestos and silica. Science. 2008;320(5876):674–677. doi:10.1126/science.1156995.
- Gabelloni ML, et al.. NADPH oxidase derived reactive oxygen species are involved in human neutrophil IL-1β secretion but not in inflammasome activation. Eur J Immunol. 2013;43(12):3324–3335. doi:10.1002/eji.201243089.
- Liu N, Wu Y, Wen X, Li P, Lu F, Shang H. Chronic stress promotes acute myeloid leukemia progression through HMGB1/NLRP3/IL-1β signaling pathway. J Mol Med (Berl). 2021;99(3):403–414. doi:10.1007/s00109-020-02011-9.
- Dinarello CA. Interleukin-1 in the pathogenesis and treatment of inflammatory diseases. Blood. 2011;117(14):3720–3732. doi:10.1182/blood-2010-07-273417.
- Aldieri E, Riganti C, Polimeni M, Gazzano E, Lussiana C, Campia I, Ghigo D. Classical inhibitors of NOX NAD(P)H oxidases are not specific. Curr Drug Metab. 2008;9(8):686–696. doi:10.2174/138920008786049285.
- Massart C, Giusti N, Beauwens R, Dumont JE, Miot F, Sande JV. Diphenyleneiodonium, an inhibitor of NOXes and DUOXes, is also an iodide-specific transporter. FEBS Open Bio. 2013;4(1):55–59. doi:10.1016/j.fob.2013.11.007.
- Ridker PM, MacFadyen JG, Thuren T, Everett BM, Libby P, Glynn RJ, Ridker P, Lorenzatti A, Krum H, Varigos J, et al.. Effect of interleukin-1β inhibition with canakinumab on incident lung cancer in patients with atherosclerosis: exploratory results from a randomised, double-blind, placebo-controlled trial. Lancet. 2017;390(10105):1833–1842. doi:10.1016/S0140-6736(17)32247-X.