ABSTRACT
The nasopharyngeal epithelium is highly susceptible to pathogenic infection. More than 95% of nasopharyngeal carcinomas (NPCs) are Epstein–Barr virus (EBV)-associated epithelial cancers densely infiltrated with EBV-free lymphocytes. It remains unknown whether the immune modulating effects of concurrent chemoradiotherapy (CCRT) on the tumor-infiltrating T-cell priming against EBV, tumor-associated antigens, and/or neoantigens can elicit systemic anti-tumor immunity and decrease recurrence or distant metastasis. Using matched EBV-associated NPCs, nasopharyngeal mucosal tissues, and longitudinal serial peripheral blood samples, we explored the spatiotemporal and quantitative changes in expansion and contraction of intratumoral T-cell clonotypes (ITCs) in peripheral blood samples from before, during, and after CCRT. The pre-treatment nasopharyngeal ITC repertoire contained unique mucosa-resident and commonly system-shared T-cell receptors (TCRs), portraying an individualized tumor-associated and/or metagenomic landscape. We found that the long-term disease-free patients had significantly more robust unique mucosa-resident ITCs that migrated into and expanded in the peripheral blood after CCRT than in the patients with recurrence or distant metastasis (Mann–Whitney U test, p = .0110). However, the system-shared productive ITC TCRs specific to the common viruses, such as EBV, cytomegalovirus, and influenzaA, in all the patients with and without recurrence demonstrated almost no expansion after CCRT. Thus, these findings underline the importance of determining the impact of unique intratumoral immune responses, reflected in the peripheral blood, on disease prognosis after treatment and challenge of mechanistically understanding the common systemic immune evasion of EBV in NPC patients.
Introduction
Nasopharyngeal carcinoma (NPC) originating from the epithelium of the nasopharynx is one of the most endemic malignant tumors in southern China, Southeast Asia, and Taiwan with an incidence of 3–25 per 100,000 people each year.Citation1,Citation2 The nasopharynx is a part of the Waldeyer’s ring of lymphoid tissue that is directly exposed to the external environment and, therefore, is highly susceptible to pathogenic infections.Citation3 More than 95% of NPCs are Epstein–Barr virus (EBV)-associated undifferentiated carcinomas.Citation2 Because the EBV-infected epithelial cancer cells of NPC are dispersed in a dense infiltration background of mature EBV-free lymphocytes, NPC is also called as lymphoepithelioma.
The role of EBV infection and host adaptive immunity in NPC oncogenesis and immune surveillance remain to be fully elucidated.Citation4–6 Although tumor-associated antigens, neoantigens, and/or EBV antigens on the NPC cells are likely the main sources driving the observed immune response,Citation7 the origin of the NPC-infiltrating lymphocytes is still unclear. However, the patient antigen specificity of T-cell receptor (TCR) repertoires in the nasopharynx-infiltrating lymphocytes of each EBV-associated NPC might be a result of the individual metagenomic and/or genomic landscape, reflecting the interactive tumor-host-virus immune microenvironment.Citation8
Persistent EBV infection can result in the chronic stimulation of T-cells, leading to T-cell exhaustion.Citation9 Whether the infiltrating lymphocytes around the EBV-infected NPC cells are anti-tumor and/or anti-EBV T-cells that can be primed to augment or induce anti-tumor immunity remains to be elucidated.Citation10 Successful adaptive mucosal immune responses may result in a functional state of immune memory, in which memory lymphocytes circulate through the whole body and migrate to micrometastatic deposits to prevent distant metastasis or subsequent recurrence after cancer treatment. Concurrent chemoradiotherapy (CCRT) is the mainstay treatment for locally advanced NPC.Citation11 In addition to directly killing tumor cells by inducing DNA damage, CCRT also induces indirect tumor cell death via immunomodulatory effects. In contrast to their immunosuppressive effects on inhibiting bone marrow precursor cell proliferation, both radiotherapy and chemotherapy can release immunostimulatory molecules, such as exogenous microbe- and damage-associated molecular patterns, from damaged virus-infected cells to activate dendritic cells, which then phagocyte the virus-related tumor cells to enhance tumor-associated antigen, neoantigen and/or viral antigen exposure and MHC class I presentation to favor specific CD8+ T-cells priming.Citation12–17 Furthermore, activated cytotoxic T-cells can kill not only the malignant cells in the irradiated area but also distant nonirradiated systemic tumors, a process termed abscopal effect.Citation18 However, it remains unknown whether CCRT differentially stimulates or ameliorates nasopharyngeal mucosal and defensive systemic immunity and whether CCRT elicits T-cell responses at the local mucosa and/or distal organs against tumor-associated antigens, neoantigens, and/or EBV antigens.
Radiation can not only activate T-cells but also kill T-cells inside the radiation field. CCRT effectively cleans the NPC cells and tumor-infiltrating lymphocytes in the irradiated nasopharynx and the adjacent neck lymphatic drainage space. Thus, because of rapid tumor regression by CCRT, the only chance to identify indicators of intrinsic tumor-infiltrating T-cell response after CCRT might be to study the serial changes of intratumoral T-cell clonotypes (ITCs) from before, during to after CCRT in the peripheral blood space that is outside the radiation field.
Peripheral expansion of ITCs in the systemic blood has been associated with the clinical response to PD-1/PD-L1 blockage immunotherapy.Citation19 Given the potential of CCRT to augment local and systemic anti-tumor immune responses, this study aims to characterize the intratumoral/nasopharyngeal mucosal infiltrating TCRβ clonotypes before, during, and after CCRT. With this approach, we seek to evaluate if the immune modulatory effects of CCRT on the EBV-associated NPC can induce proliferative ITC and/or anti-EBV-specific T-cell clone bursts in the peripheral blood and if the induced systemic immune responses is associated with the risk of recurrence or distant metastasis.
Materials and methods
Patient population
This study included 36 pathology-confirmed staged cT3-4N2-3M0 (stage III–IVb in 7th Edition of the American Joint Committee on Cancer) EBV-associated NPC patients with available tumor and blood TCRβ data received definitive concurrent chemotherapy and intensity-modulated radiotherapy of 74 Gy in 37 fractions at 2 Gy per fraction 5 days a week with weekly cisplatin (40 mg/m2) over 7 weeks at our center between January 01, 2007 and December 31, 2012.
This was a retrospective study. As shown in Supplemental Table S1, patient characteristics were similar between groups with (n = 11) and without (n = 25) recurrence, including gender, age, stage, T-category, N-category, T-cell clonality of the nasopharynx and blood space, and smoking status. The patients were of age of 26–73 years, exhibited Karnofsky scores of at least 70, and had adequate bone marrow, liver and renal function before treatment. No (neo)adjuvant chemotherapy was given. The cumulative radiation dose to the primary tumor and metastatic nodes of the neck was 70–74 Gy and 66–70 Gy, respectively. All possibly affected areas in bilateral cervical lymphatics down to the supraclavicular fossa were irradiated with a dose of 50–54 Gy.
All patients provided written informed consent for biopsy, pathological analysis, and treatment. They were regularly followed up at least four times per year according to the institutional protocol. Clinical characteristics, radiological evaluation findings, and laboratory results from the clinical and pathological database were retrospectively reviewed under approval of the Institutional Review Board.
In situ EBV hybridization
Tissue sections (4-μm-thick) from the paraffin blocks were deparaffinized, rehydrated with phosphate buffered saline (PBS), digested with pepsin (30 μg/mL) for 10 min at 37°C, fixed in 4% paraformaldehyde in PBS, and washed in 3 × saline solution citrate (SSC) buffer (0.15 M sodium chloride and 15 mM sodium citrate; pH 7.0). A probe encoding EBER-1, a small non-coding RNA expressed in all cells infected by EBV, was labeled with digoxigenin-dUTP by random priming with a DNA labeling kit (Boehringer Mannhim). The samples were pre-hybridized at 40°C for 2 h, and hybridization was performed overnight at 40°C with the EBV probe. After hybridization, excess probe was removed by sequential washing in 2 × SSC, 0.5 × SSC, and 0.2 × SSC at 37°C for 2 h. The tissue sections were incubated at 37°C for 1 h with biotinylated mouse anti-digoxigenin, followed by the addition of streptavidin-biotin-peroxidase complex for 20 min and a 5-min incubation with 3,3ʹ-diaminobenzidine/hydrogen peroxide as the substrate chromogen (Dako).
DNA and RNA extraction and high-throughput TCRβ sequencing
NPC and nasopharyngeal mucosa tissues were processed immediately after biopsy and embedded in formalin fixed paraffin blocks by certified medical pathologists. The formalin-fixed, paraffin-embedded tissue specimens were stored at room temperature and used for genomic DNA extraction. Once the matched serial blood samples were collected, the genomic DNA and RNA of blood lymphocytes were extracted immediately according to the mini kits from Qiagen following manufacturer’s instructions. RNA was reversely transcribed to cDNA. The genomic and cDNA were stored as a precipitate in ethanol at −164°C.
To generate the template library for analysis of the TCRβ immune repertoire, a multiplex PCR -based next-generation sequencing system was designed to amplify millions of rearranged TCRβ CDR3 regions from the genomic DNAs and cDNAs. As previously described elsewhere, 45 forward primers (each of them specific to a functional TCR Vβ segment), and 13 reverse primers (each of them specific to a TCR Jβ segment) were used.Citation20–22 With the selected primers, only the recombined V-D-J area was amplified. The forward and reverse primers contained an adaptor at their 5′-ends. The Illumina GA2 System generated reads of length (100–500 bp), which covered the entire range of TCRβ CDR3 lengths.
The detailed methodologies using Shannon entropy for comprehensive analysis of the number of T-cell clonotypes present (richness) and of their relative frequencies (evenness) of TCR repertoires have been described in our and other previous studies.Citation23–26 In brief, T-cell clonality was determined by using the sequence variance of the TCRβ CDR3, and each T-cell clonal frequency was calculated as the percentage of the total number of each unique sequence read divided by the overall reads of each sample. The abundance of each T-cell clonality was ranked according to its frequency as the top 1%, top 1% to 3%, 3% to 5%, and >5% most frequent clonotypes in each organ. T-cell occupied clonal space was defined as the summed frequency of clones relative to the total TCR β-chain repertoire in the respective organ space (blood or nasopharynx) of each patient.
Statistical analysis
The ITCs with an increase of occupied clonal space over time were defined as expanded clones, whereas those with decrease of abundance compared with previous time point were defined as contracted clones.Citation26 Identification of differentially expanded or contracted clones in the peripheral blood after CCRT was performed using Fisher exact test with multiple testing correction using the Benjamini-Hochberg procedure.Citation27 The Mann–Whitney U test was used for comparison of two-group data. A value of p < .05 was set as the threshold for significance. Statistical analysis was performed using the R software.
Results
Compartmental analysis of ITC dynamics after chemoradiotherapy
We have shown that the T-cell repertoire of T-cell infiltrating NPC is polyclonal and consists of locally mucosa-unique, patient-unique, tumor-associated, EBV- and other pathogen-specific, systemically blood-shared, and commonly patient-shared clones.Citation25,Citation28 We found a relatively high degree of overlap or homogeneity of the TCRβ repertoires between NPCs and their surrounding nonmalignant adjacent mucosa.Citation25 This portrays the tumor-associated and metagenomic landscape of individualized NPC/nasopharynx immune environment. The expansion of tumor-infiltrating TCRβ clonotypes in the peripheral blood during immunotherapy has been associated with the prognosis of patients.Citation19,Citation25 Hence, in this study we first assessed the synchronous cross-compartment dynamics of the intratumoral T-cell repertoires between the nasopharynx and the peripheral blood in EBV-associated NPC patients treated with CCRT before, during, and after treatment and investigated the relationship between the T-cell clonal dynamics and tumor recurrence or the appearance of distant metastasis (Supplemental Figure S1).
As shown in , the ITC population of NPC or the clonotypic composition of the tumor-infiltrating lymphocytes originally present in the tumor at the pre-treatment stage could be categorized into two groups: 1) local nasopharynx-, mucosa-, and tumor-resident ITCs; 2) systemic ITCs present in the nasopharynx and peripheral blood. Because positive and negative clonal selection might contribute to reshape the T-cell repertoire after CCRT, we used deep sequencing of TCRβ to investigate whether there were spatiotemporal or quantitative differences in the preexisting ITCs (mucosa-resident or system-shared) between patients with and without recurrence following CCRT.
Figure 1. Cross-compartment T-cell receptors (TCR) Vβ repertoire dynamics between the intratumoral/nasopharynx and the peripheral blood following concurrent chemoradiotherapy (CCRT) for Epstein–Barr virus (EBV)-associated nasopharyngeal carcinomas (NPCs). Each point on the scatter plots represents a single T-cell clonotype with normalized clonal size and fraction at pairwise nasopharynx/blood compartments from before, during, and after CCRT. The clonality and occupied clonal space fraction of the top frequency-ranked original tumor-infiltrating T-cells in each compartment are indicated. (a) A representative patient without recurrence 5 years after CCRT. (b) A representative patient with a newly developed bone metastasis 3 years after CCRT
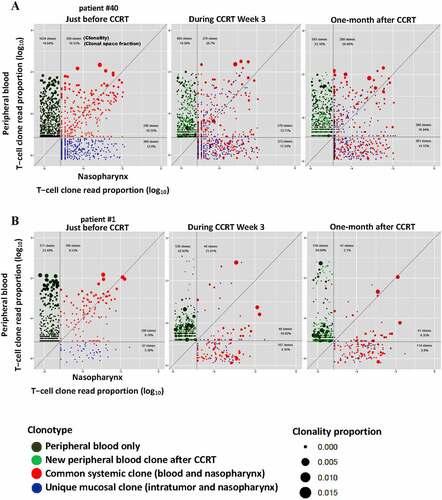
Unique clonal expansion of ITCs in peripheral blood correlates with clinical outcome after chemoradiotherapy
The T-cell clonalities of the pre-treatment intratumoral/nasopharynx and the peripheral blood seemed more diverse in the patients without recurrence than those in the patients with recurrence. Nonetheless, the total number of TCRβ clonotypes of the T-cell repertoire in each compartment in the patients with and without recurrence was not significantly different (). The dynamic changes in total lymphocyte count induced by CCRT were also similar among the patients with and without recurrence (). The total lymphocyte count fluctuated and declined gradually with time during CCRT, and then rapidly returned to normal levels within 2 weeks after CCRT. In line with the results of previous studies using low chemotherapy doses that were below the maximum-tolerated dose and were able to induce an immune response (12), the transient pronounced lymphodepletion by the metronomic schedule of CCRT used in this study enabled a global reset of the immune system to mount selective immune responses (Supplemental Figure S2). Although patients with recurrence presented a generalized low-reactive contractive patterns or loss of clonal expansion of ITC responses in the peripheral blood after CCRT, patients without recurrence presented a relatively increased expansive pattern of ITC reactions in the peripheral blood after CCRT. Moreover, following CCRT, the long-term disease-free patients had a significantly large proportion of initially mucosa-resident ITCs, but not commonly system-shared ITCs, that expanded in the peripheral blood relative to the patients with recurrence or distant metastasis (Mann–Whitney U test, p = .0110) (). The initially mucosa-resident ITCs expanded most in the peripheral blood immediately after CCRT in the patients without recurrence were unique to each patient and were not matched against any TCR in the known TCR database (). On the other hand, the commonly system- and patient-shared ITCs against EBV lytic and latent antigens, almost all of the cytomegalovirus (CMV) and influenzaA antigens in the patients with and without recurrence did not expanded substantially in the peripheral blood after CCRT. Nonetheless, some of them showed skewed expansion and contraction during the period of CCRT administration ().
Figure 2. Association of changes of intratumoral and blood T-cell clonality with clinical outcome in Epstein–Barr virus (EBV)-associated nasopharyngeal carcinoma (NPC) patients after concurrent chemoradiotherapy (CCRT) for the patients with recurrence (n = 11) and without recurrence (n = 25). (a) Clonality diversity of the T-cell receptor repertoires in the pre-treatment intratumor/nasopharynx and peripheral blood. A violin plot is used to visualize the data distribution and probability density (X-axis), including the median, interquartile range and 95% confidence interval in the central bar, and a wider or skinnier kernel density estimation on each side of the central bar to show the distribution shape of the higher or lower probability (Mann–Whitney U test). (b) The changes in lymphocyte count because of CCRT. (c) Correlation of the unique initially mucosa-resident and commonly system-shared intratumoral T-cell clonotype expansion in the peripheral blood with clinical outcome after CCRT. Note that a wider or skinnier kernel density estimation on each side of the central bar of the violin plot demonstrates the distribution shape of the higher or lower probability for the patients (Mann–Whitney U test)
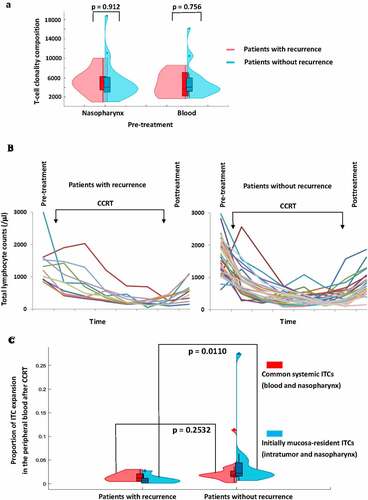
Figure 3. Temporal kinetic patterns of the initially mucosa-resident intratumoral T-cell clonotypes (ITCs) that migrated into the peripheral blood immediately following concurrent chemoradiotherapy (CCRT). Each line demonstrates the changes of occupied clonal space in the longitudinal serial peripheral blood samples of a single ITC with time. (a) The low-reactive, contractive, or exhausted ITC response patterns in 5 representative patients with recurrence. (b) The high-reactive and expansive ITC response patterns in 5 representative patients without recurrence. The antigen-binding site TCRβ CDR3 sequences of the initially mucosa-resident ITCs most expanded in the peripheral blood after CCRT are also shown. Note that these sequences are unique and not shared across the patients
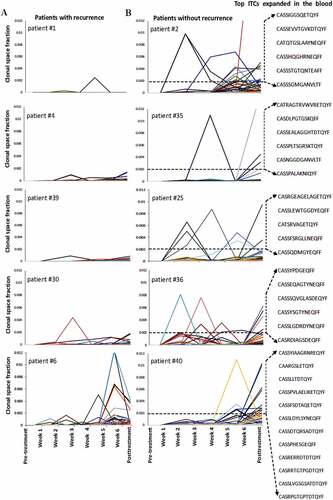
Figure 4. Acute systemic responses and temporal dynamics of the common anti-viral intratumoral T-cell clonotypes (ITCs) shared with the peripheral blood T-cell repertoires in the longitudinal serial peripheral blood samples following concurrent chemoradiotherapy (CCRT). Each line represents the total occupied clonal space in the blood of different ITCs against the same viral antigen over time in each patient with recurrence (n = 11) and without recurrence (n = 25). (a) Anti-Epstein Barr virus (EBV) lytic and latent antigens (BZLF 1, BRLF 1, BMLF 1, EBNA 1, EBNA 3A, EBNA 3B/4, LMP 1, and LMP 2A). (b) Anti-cytomegalovirus (CMV) antigens (IE1 and pp65). (c) Anti-influenzaA antigens (HA and M)
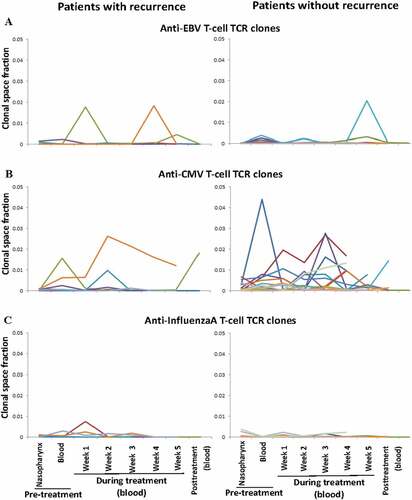
The fate of ITCs in the periphery after chemoradiotherapy
Because most recurrence occurred within 1–3 years after treatment, we continuously traced the fate of ITCs 1–3 years after CCRT. Despite complete remission of the primary EBV-associated NPCs in all treated patients, a subpopulation portion of ITCs following expansion, contraction, or exhaustion remodeling phases with time after CCRT were still detectable at high or low levels in the peripheral blood (). We then stratified the ITCs according to their baseline percentage in the tumor bed and evaluated the change in their abundance one year after CCRT. Patients without recurrence were found to have a higher proportion of the top 1% most frequent ITCs in the peripheral blood one year after CCRT than patients with recurrence (Mann–Whitney U test, p = .0466) (). Furthermore, the top 1% frequency-ranked ITCs in patients without recurrence occupied a significantly greater clonal space in the peripheral blood one year after CCRT than those in patients with recurrence (Mann–Whitney U test, p = .0033) (). These results indicated that peripheral expansion of the most frequent ITCs was correlated with better outcome after CCRT and that the most frequently expanded T-cell TCRβ clonotypes were among the most prevalent TCRs in the initially bulk tumor-resident T-cell population. The actively clonally-expanded ITCs (in-frame TCRβ CDR3 gene sequences with mRNA expression) that were long-lasting in the long-term disease-free patients might contain the tumor-infiltrating T-cells that underlie the patient-specific responses to CCRT. We further examined the productive intrinsic ITCs that reshaped the most in the peripheral blood 2 years after CCRT. While the frequency of the original ITC repertoire in the peripheral blood slightly fluctuated 2 years after CCRT, significantly more patients without recurrence still had a higher T-cell clonality and/or greater blood T-cell clonal space occupied by the actively producing ITCs as compared to patients with recurrence (). Almost all of the major remaining productive ITCs identified in the pairwise time point (pre-treatment vs. 2-year posttreatment) were still the originally top frequency-ranked uncharacterized mucosa- or tumor-resident lymphocyte products, and did not include anti-EBV clones despite the fact that all NPCs in this study were EBV-associated as established by in situ EBV hybridization during pathological diagnosis (Supplemental Tables S2A and S2B). In addition to significantly more unique expressing ITCs were enriched in the patient group without recurrence, a subset of the persistently active ITCs was identified to be shared only in the patient group without recurrence ( and Supplemental Table S3). In contrast, almost no expressing ITC (only 1 clonotype) was detectable to be shared only in the patient group with recurrence. Thus, the similarity and divergence of the posttreatment long-lasting active ITC TCRβ repertoires between patients with and without recurrence also correlated with the risk or prognostication. Moreover, 3 years after CCRT, 7 of the 11 patients with recurrence almost lost the originally mucosa-resident top 1–5% unique ITC TCRβ in the periphery, whereas this was only observed in 4 of the 25 patients without recurrence.
Figure 5. Peripheral intratumoral T-cell clonotype (ITC) repertoire remodeling 1 year after concurrent chemoradiotherapy (CCRT). A violin plot is used to visualize the data distribution and probability density, including the median, interquartile range and 95% confidence interval in the central bar, and a wider or skinnier kernel density estimation on each side of the central bar to show the distribution shape of the higher or lower probability for all of the patients with recurrence (n = 11, red) and without recurrence (n = 25, blue) (Mann–Whitney U test). (a) The percent of pre-treatment ITCs persistently detected in the 1-year post-treatment peripheral blood. (b) The percent of ITC composition in the 1-year post-treatment peripheral blood according to the initial frequency rank of the total occupied clonal space among all ITCs in the nasopharynx (top 1% ranked ITCs vs. top 1–3% vs. top 3–5% vs. non-top). (c) Comparison of the percent of 1-year post-treatment blood clonal space occupied by the frequency-ranked ITCs between the patients with and without recurrence
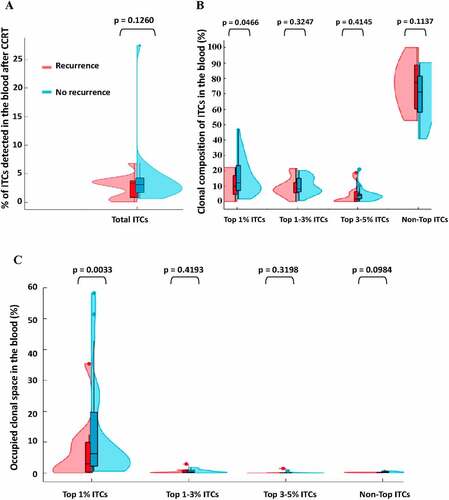
Figure 6. Comparison of the long-lasting expressing intratumor T-cell clonotypes (ITCs) in the peripheral blood 2 years after treatment between patients with and without recurrence. (a) The clonality and its corresponding occupied clonal space of persistently residual active original ITCs with in-frame TCRβ CDR3 gene sequences and mRNA expression in the peripheral blood 2 years after CCRT in patients recurrence (n = 11) and without recurrence (n = 25). (b) A violin plot is used to visualize the clonality and occupied clonal space fraction distribution and probability density, including the median, interquartile range and 95% confidence interval in the central bar, and a wider or skinnier kernel density estimation on each side of the central bar to show the distribution shape of the higher or lower probability (Mann–Whitney U test). (c) Comparison of the unique and interpatient shared expressing ITC composition between patients with and without recurrence. Note that a subset of expressing ITCs is shared only in patients without recurrence, which is in sharp contrast to almost no ITC (only 1 ITC) is shared only in patients with recurrence
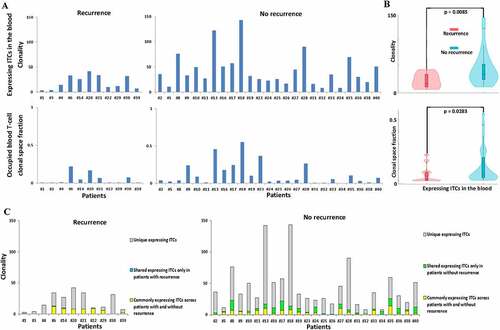
Low systemic T-cell response to EBV after chemoradiotherapy
Despite relatively low T-cell responses to EBV from before to after CCRT in all patients as compared to the unique mucosa-resident ITC expansion in the peripheral blood, we further studied whether patients with and without recurrence following CCRT presented differentiable or distinct systemic T-cell responses to respective EBV-specific antigens. For this purpose, we analyzed the occupied clonal space of EBV-specific ITCs in the pre-treatment nasopharynx () and the relative mRNA levels of active expression of anti-EBV respective antigen T-cell TCRs in the peripheral blood before and after CCRT between patients with and without recurrence (). No specifically expanded or productive TCRβ clonotypes recognizing EBV lytic or latent antigen epitopes were associated with the prognosis of EBV-associated NPC patients after CCRT. Moreover, both groups with and without recurrence had similarly no inductive or probably exhausted immune response to EBV after CCRT in near 30% of the patients (). Consistent with this result, all of the biopsy specimens from the primary, later recurrent, or distantly metastatic tumors showed strongly diffuse nuclear expression of the EBV non-coding small RNA, EBER-1, which is a molecular marker of immune escape ().Citation5,Citation6,Citation25 Collectively, our results implied that no or very low EBV-inducing anti-tumor T-cell reactivity or immunity was elicited before, during, or after CCRT despite unremitted, intense infiltration of lymphocytes around persistently EBV-associated everlasting NPC tumor cells.
Figure 7. The systemic T-cell repertoire responses to Epstein–Barr virus (EBV) antigens following concurrent chemoradiotherapy (CCRT). (a) Comparison of the summed occupied clonal space of pre-treatment intratumoral T-cell clonotypes (ITCs) against each EBV-specific lytic and latent antigen in the nasopharynx between the patients with (n = 11) and without recurrence (n = 25). (b, c) Comparison of the relative mRNA expression levels of the summed productive TCRβ clonalities against each anti-EBV-specific antigen in the pre-treatment and 1-year post-treatment peripheral blood between the patients with and without recurrence. (d) Representative examples of positron emission tomography–computed tomography (PET-CT) scanning, magnetic resonance imaging (MRI), hematoxylin and eosin (H&E) staining and the corresponding EBV EBER-1 in situ hybridization for the metastatic bone tumor from a nasopharyngeal carcinomas (NPC) patient 3 years after CCRT. Note that the dense EBV-free lymphocytes infiltrate around the diffusely strongly EBV-positive recurrent NPC tumor cells
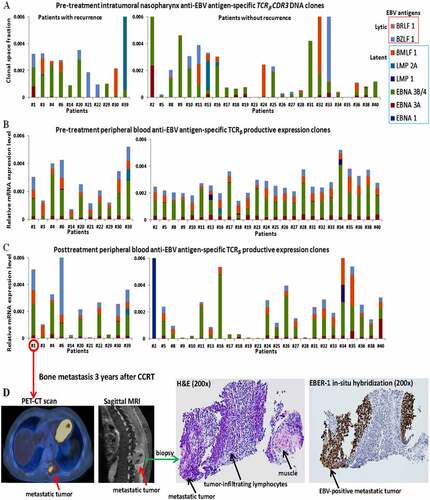
Discussion
NPC is heavily lymphocyte-infiltrated, in which the tumor cells, but not the tumor-infiltrating lymphocytes, are infected by EBV.Citation4 The clonal expansion and contraction of tumor-infiltrating T-cells might be essential for adaptive immune surveillance to prevent recurrence after treatment. To identify the predictive factors associated with clinical responses, as well as the mechanisms underlying therapeutic success, it is necessary to unravel the interactions between the environment, the immune system, and tumor cells, as well as their impact on CCRT treatment response. However, there is lack of quantitative information on the distribution and dynamics of individual clonotypes of the tumor-infiltrating T-cells, especially anti-tumor and anti-EBV T-cells, in relationship to clinical CCRT response,Citation2 and current understandings of the fate of tumor-infiltrating T-cells and their migration over the course of CCRT remain to be determined. The aim of the present study was to further elucidate the link between intratumoral and/or anti-EBV T-cell responses to CCRT with clinical outcomes. For this purpose, we sequenced T-cell TCR Vβ CDR3 antigen-binding site before, during, and after treatment to monitor changes in the clonotypic composition of ITCs in the peripheral blood. This approach permitted us to identify the unique, enriched, original mucosal ITCs, but not anti-EBV T-cells, with potent proliferative capacity, which might impact the systemic anti-tumor immune reactivity and imply immune evasion of EBV after CCRT.
We showed that more clonotypic expansion of unique intratumoral infiltrating T-cells in the peripheral blood was associated with a reduced risk of recurrence in patients after CCRT. This result is in line with previous studies reporting an association between specific tumor-infiltrating T-cell clonal expansion in the peripheral circulation with the anti-tumor response after PD-1 blockade immunotherapy in lung cancer patients.Citation19,Citation25 Tracking of the pre-treatment ITCs in the peripheral blood after CCRT revealed that some of the top 1% frequency-ranked unique mucosa-resident ITCs, but not the commonly system-shared anti-EBV-specific ITCs, emerged as the most critical immune response burst that could distinguish patients with or without recurrence after CCRT. Hence, reinvigoration of preexisting tumor-infiltrating mucosa-unique TCRβ clonotypes, but not instigation of the response of commonly system-shared anti-EBV T-cell, was associated with long lasting remissions in EBV-associated NPC patients. As shown in the , Supplemental Figure S2 and Supplemental Tables S2 and S3, our study indicated that in contrast to the low intratumoral T-cell response profile in patients experiencing recurrence, the active clonal expansion profile or pattern of originally uniquely mucosa-resident ITCs detected in the peripheral blood in the long-term disease-free patients might represent an effective intrinsic tumor-resident T-cell activation and serve as a personalized predictive biomarker of clinical benefit for NPC after CCRT.
Although the presence of uniquely enriched ITCs seemed to potentiate clinical efficacy, the present study was not sufficient to elucidate whether the prevailing or predominant CCRT-induced peripheral expansion of ITCs in patients without recurrence resulted from neoantigen-specific or tumor-reactive clonal selection. It also remained unsure whether the lower T-cell clonality of CCRT-induced expansion of ITCs in patients with recurrence than in patient without recurrence resulted just from nonspecific stimulation of lymphocytes present in tumors or the nasopharynx through clonal drifting happened by chance. In this and our previous study,Citation23 most of the T-cell TCRβ CDR3 antigen-binding site sequences from the tumor-infiltrating lymphocytes of EBV-associated NPCs were not discovered in the public databases. The common sequences against the widespread viral epitopes of EBV, CMV, and influenzaA antigens were easily detected in almost all of the patients. The low frequency of TCRβ clones responding to the shared neoantigens of BST2, MLANA, and TKT was found only in 24% of NPC patients. Interestingly, these known commonly patient-shared ITCs were not expanded following CCRT. By contrast, most of the CCRT-elicited top frequency ITCs were unique. The broad and heterogeneous landscape of unique ITCs between NPC patients could be shaped by ecological and evolutionary interactions between the tumor and its microenvironment and by host-specific immune-related HLA divergence combined with NPC-exclusive non-mutated cellular, viral antigen, and/or mutation-associated neoantigen generation. CCRT can trigger positive and negative ITC selection, which causes expansion and contraction of subclones. Moreover, some novel systemic TCRβ clonotypes expanded in the peripheral blood were also found in patients with and without recurrence after CCRT. Such kind of post-treatment novel clonal replacement might result from a newly developed neoantigen-specific T-cell response induced by in situ vaccination by CCRT. Nonetheless, it may also reflect a T-cell response from an evolution of environment-related, mucositis-induced, and/or pathogen-associated metagenomics, which is not relevant to the tumor response or clinical benefit. Therefore, the expanded ITC profiles could be the consequence of the overall immune challenge against tumors, irradiated microenvironment normal cells, self-antigens, tissue-specific antigens, and/or foreign antigens. Thus, to identify tumor-reactive lymphocytes and tumor targets, autologous tumor-specific recognition is not assessable by sequencing the TCRβ of expanded ITCs in the peripheral blood alone.
Moreover, patients with late recurrence after CCRT might have an immunosuppressive tumor–immune microenvironment with a deficiency in replenishment of T-cell repertoire and/or loss of the ability of the immune system to recognize tumor antigens, which could result in immune escape from the CCRT-activated immunosurveillance. Thus, to characterize the differences in anti-tumor immune responses to CCRT between patients with or without recurrence and to study the overall immune response, it is necessary to investigate not only the distinct tumor-infiltrating TCRβ profiles before and after CCRT and the CCRT-elicited peripheral expansion of the ITCs that are unique to individual patients, but also the detailed immunological function of tumor-associated T-cell reactivity and cytotoxicity before and after CCRT. Furthermore, it is also important to know the differential changes in the composition and quality of immune infiltrates in the tumor microenvironment during and after CCRT between the long-term disease-free patients and the patients with recurrence, and between the primary tumors and their corresponding metastatic tumors in the patients with recurrence after CCRT. These differential changes might include the effector and exhausted CD3/CD4/CD8/Treg T-cell subtype profiles and their ratios and densities, the IFNγ-associated gene expression, and the proximity of PD-1+-T-cell and PD-L1+-tumor cells.
In contrast to the striking interpatient specificity of the long-lasting, unique CCRT-induced, mucosa-resident ITC expansion and expression in the long-term disease-free patients, an inadequate T-cell proliferative burst to EBV in patients with and without recurrence after CCRT suggested that a general deficiency of local and systemic tumor-host-EBV immune reactions might represent a common mechanism of immune evasion in EBV-associated NPC patients. The non-coding RNAs expressed by EBV, including EBERs and miRNAs, have been found to mediate immune evasion.Citation5,Citation6 As EBER-1 is widely expressed in every EBV-positive primary, recurrent, or metastatic NPC tumor cell and chronic virus infection can induce a T-cell differentiated or exhausted state, an immunostimulation approach targeting EBER-1 by employing antisense oligonucleotides or small interfering RNAs and using an immune checkpoint blocker in combination with CCRT for EBV-associated NPC may be a suitable approach to convert an immune-cold into an immune-hot tumor.Citation5,Citation13,Citation29
In conclusion, our results suggested that although CCRT elicited the expansion of a subset of unique intratumoral mucosal TCRβ clonotypes that might imply a specific anti-tumor T-cell response, CCRT did not induce a mucosal EBV-specific vaccination in situ to exert a systemic immunity that allows abscopal effects on protection of EBV-associated NPC patients from recurrence and distant metastasis. As this is a retrospective study, large-scale prospective studies are warranted for validation.
Supplemental Material
Download ()Acknowledgments
This work was supported by the Ministry of Science and Technology of Taiwan under Grant number 106-2314-B-368-002 and 108-2320-B-368-001.
Disclosure statement
The authors report no conflict of interest.
Supplementary material
Supplemental data for this article can be accessed on the publisher’s website
Additional information
Funding
References
- Chang ET, Adami HO The enigmatic epidemiology of nasopharyngeal carcinoma. Cancer Epidemiol Biomarkers Prev. 2006 October;15(10):1765–11. doi:10.1158/1055-9965.EPI-06-0353.
- Chua MLK, Wee JTS, Hui EP, Chan ATC Nasopharyngeal carcinoma. Lancet. 2016 Mar 5;387(10022):1012–1024. doi:10.1016/S0140-6736(15)00055-0.
- Dubourg G, Edouard S, Raoult D Relationship between nasopharyngeal microbiota and patient’s susceptibility to viral infection. Expert Rev Anti Infect Ther. 2019 June;17(6):437–447. doi:10.1080/14787210.2019.1621168.
- Young LS, Yap LF, Murray PG Epstein-Barr virus: more than 50 years old and still providing surprises. Nat Rev Cancer. 2016 Dec;16(12):789–802.
- Münz C Latency and lytic replication in Epstein-Barr virus-associated oncogenesis. Nat Rev Microbiol. 2019 November;17(11):691–700. doi:10.1038/s41579-019-0249-7.
- Tsao SW, Tsang CM, To KF, Lo KW The role of Epstein-Barr virus in epithelial malignancies. J Pathol. 2015 January;235(2):323–333. doi:10.1002/path.4448.
- Choi I-K, Wang Z, Ke Q, Hong M, Paul DW, Fernandes SM, Hu Z, Stevens J, Guleria I, Kim H-J, et al. Mechanism of EBV inducing anti-tumour immunity and its therapeutic use. Nature. 2021 February;590(7844):157–162. doi:10.1038/s41586-020-03075-w.
- Edouard S, Million M, Bachar D, Dubourg G, Michelle C, Ninove L, Charrel R, Raoult D The nasopharyngeal microbiota in patients with viral respiratory tract infections is enriched in bacterial pathogens. Eur J Clin Microbiol Infect Dis. 2018 September;37(9):1725–1733. doi:10.1007/s10096-018-3305-8.
- PJ F Epstein-Barr Virus and Cancer. Annu Rev Pathol. 2019 Jan;24(14):29–53.
- Chow JC, Ngan RK, Cheung KM, Cho WC Immunotherapeutic approaches in nasopharyngeal carcinoma. Expert Opin Biol Ther. 2019 November;19(11):1165–1172. doi:10.1080/14712598.2019.1650910.
- Chen YP, Ismaila N, Chua MLK, Colevas AD, Haddad R, Huang SH, Wee JTS, Whitley AC, Yi JL, Yom SS, et al. Chemotherapy in Combination With Radiotherapy for Definitive-Intent Treatment of Stage II-IVA Nasopharyngeal Carcinoma: CSCO and ASCO Guideline. J Clin Oncol. 2021 Mar 1;39(7):840–859. doi:10.1200/JCO.20.03237.
- Galluzzi L, Humeau J, Buqué A, Zitvogel L, Kroemer G Immunostimulation with chemotherapy in the era of immune checkpoint inhibitors. Nat Rev Clin Oncol. 2020 December;17(12):725–741.
- Galon J, Bruni D Approaches to treat immune hot, altered and cold tumours with combination immunotherapies. Nat Rev Drug Discov. 2019 March;18(3):197–218.
- Jiang W, Chan CK, Weissman IL, Kim BYS, Hahn SM Immune Priming of the Tumor Microenvironment by Radiation. Trends Cancer. 2016 November;2(11):638–645. doi:10.1016/j.trecan.2016.09.007.
- Lhuillier C, Rudqvist NP, Elemento O, Formenti SC, Demaria S Radiation therapy and anti-tumor immunity: exposing immunogenic mutations to the immune system. Genome Med. 2019 June 20;11(1):40. doi:10.1186/s13073-019-0653-7.
- Demaria S, Golden EB, Formenti SC Role of Local Radiation Therapy in Cancer Immunotherapy. JAMA Oncol. 2015 December;1(9):1325–1332. doi:10.1001/jamaoncol.2015.2756.
- Rapoport BL, Anderson R Realizing the Clinical Potential of Immunogenic Cell Death in Cancer Chemotherapy and Radiotherapy. Int J Mol Sci. 2019 February 22;20(4):959. doi:10.3390/ijms20040959.
- Craig DJ, Nanavaty NS, Devanaboyina M, Stanbery L, Hamouda D, Edelman G, Dworkin L, Nemunaitis JJ The abscopal effect of radiation therapy. Future Oncol. 2021 May;17(13):1683–1694. doi:10.2217/fon-2020-0994.
- Wu TD, Madireddi S, de Almeida PE, Banchereau R, Chen YJ, Chitre AS, Chiang EY, Iftikhar H, O’Gorman WE, Au-Yeung A, et al. Peripheral T cell expansion predicts tumour infiltration and clinical response. Nature. 2020 March;579(7798):274–278. doi:10.1038/s41586-020-2056-8.
- Robins HS, Campregher PV, Srivastava SK, Wacher A, Turtle CJ, Kahsai O, Riddell SR, Warren EH, Carlson CS Comprehensive assessment of T-cell receptor beta-chain diversity in alphabeta T cells. Blood. 2009;114(19):4099–4107. doi:10.1182/blood-2009-04-217604.
- Kirsch I Immune monitoring technology primer: immunosequencing. J Immunotherapy Cancer. 2015;3(1):29–30. doi:10.1186/s40425-015-0076-y.
- Jang M, Yew PY, Hasegawa K, Ikeda Y, Fujiwara K, Fleming GF, Nakamura Y, Park JH Characterization of T cell repertoire of blood, tumor, and ascites in ovarian cancer patients using next generation sequencing. Oncoimmunology. 2015;4(11):e1030561. doi:10.1080/2162402X.2015.1030561.
- Aversa I, Malanga D, Fiume G, Palmieri C Molecular T-Cell Repertoire Analysis as Source of Prognostic and Predictive Biomarkers for Checkpoint Blockade Immunotherapy. Int J Mol Sci. 2020 March 30;21(7):2378. doi:10.3390/ijms21072378.
- DeWolf S, Grinshpun B Thomas Savage1, Sai Ping Lau, Aleksandar Obradovic, Brittany Shonts1, Suxiao Yang, Heather Morris, Julien Zuber, Robert Winchester, Megan Sykes, Yufeng Shen. Quantifying Size and Diversity of the Human T Cell Alloresponse. JCI Insight 2018 August 9;3(15):e121256.
- Chung YL, Wu ML Spatiotemporal homogeneity and distinctness of the T-cell receptor β-chain repertoires in Epstein-Barr virus-associated primary and metastatic nasopharyngeal carcinomas. Int J Cancer. 2018 August 1;143(3):610–620. doi:10.1002/ijc.31336.
- Zhang J, Ji Z, Caushi JX, El Asmar M, Anagnostou V, Cottrell TR, Chan HY, Suri P, Guo H, Merghoub T, et al. Compartmental Analysis of T-cell Clonal Dynamics as a Function of Pathologic Response to Neoadjuvant PD-1 Blockade in Resectable Non–Small Cell Lung Cancer. Clin Cancer Res. 2020 March 15;26(6):1327–1337. doi:10.1158/1078-0432.CCR-19-2931.
- Danilova L, Anagnostou V, Caushi JX, Sidhom JW, Guo H, Chan HY, Suri P, Tam A, Zhang J, Asmar ME, et al. The Mutation-Associated Neoantigen Functional Expansion of Specific T Cells (MANAFEST) Assay: a Sensitive Platform for Monitoring Antitumor Immunity. Cancer Immunol Res. 2018 August;6(8):888–899. doi:10.1158/2326-6066.CIR-18-0129.
- Wu L, Chung Y-L Tumor-Infiltrating T Cell Receptor-Beta Repertoires are Linked to the Risk of Late Chemoradiation-Induced Temporal Lobe Necrosis in Locally Advanced Nasopharyngeal Carcinoma. Int J Radiat Oncol Biol Phys. 2019 May 1;104(1):165–176. doi:10.1016/j.ijrobp.2019.01.002.
- Winkle M Sherien M El-Daly, Muller Fabbri, George A Calin. Noncoding RNA Therapeutics - Challenges and Potential Solutions. Nat Rev Drug Discov 2021 June;18:1–23.