ABSTRACT
Low response rates to certain tumor types remain a major challenge for immune checkpoint blockade therapy. In this study, we first conducted an integrated biomarker evaluation of bladder cancer patients from confirmatory cohorts (IMvigor210) and found that no significant differences exist between sexes before acceptance of anti-PD-L1 treatment, whereas male patients showed a better response. Thus, we then focused on sex-related changes post anti-PD-L1 treatment and found no obvious impact on the gut microbiota in male mice but a significant decrease in the sex hormone levels. Further, castration dramatically enhanced the antitumor efficacy against murine colon adenocarcinoma in male mice. Moreover, a narrow-spectrum antibiotic, colistin was innovatively used for deregulation of testosterone levels to enhance the immunotherapy efficiency in male mice. These findings indicate that the impact on the sex hormone levels in males may contribute to the sexual dimorphism in response and provide a promising way to enhance immunotherapy efficiency.
Introduction
Immunotherapy provides a revolutionary way to cancer treatment by harnessing the power of the patients’ own immune systems. Immune checkpoint blockade targeting the programmed cell death 1 (PD-1) or its ligand PD-L1, and the cytotoxic T lymphocyte–associated antigen 4 (CTLA-4) has been applied as a first-line treatment for several cancers.Citation1,Citation2 However, the response rate to immune checkpoint inhibitors (ICIs) is far from satisfactory for certain tumor types.Citation3 A number of factors, including the tumor intrinsic genetics, the immunity of patients, and environmental factors, are found to impact on the efficiency of immune checkpoint blockade therapy.Citation3 Factors such as the PD-L1 expression level, tumor mutational burden (TMB), and tumor infiltrating lymphocyte status are identified as the predictive biomarkers for patient selection.Citation4 Novel procedures such as regulating gut microbiota have been attempted to enhance the clinical responses of ICIs.Citation5 The sex factor in the ICIs treatment is, however, rarely discussed by far.
There are reports on differences in the male and female immune responses to self and foreign antigens.Citation6 Females usually have stronger immune responses with a lower frequency of infectious diseases but a higher risk of autoimmune diseases.Citation7 In contrast, males have a higher mortality rate across various cancer types.Citation8 In addition, females tend to produce higher levels of protective antibodies in response to viral vaccines but are more likely to develop adverse reactions than males.Citation9,Citation10 These sex-related differences may also influence the antitumor efficiency of ICIs. For instance, PD-L1 blockade has been shown to be more efficacious in female mice with B16 melanoma compared with their male counterparts.Citation11
Experimental data from clinical meta-analyses were controversial. A meta study involving 20 randomized controlled trials (mostly melanoma and non-small-cell lung cancer, NSCLC) by Conforti et al. showed that the pooled overall survival hazard ratio in male patients treated with anti-CTLA-4 or anti-PD-1 was obviously lower than in females.Citation12 Similar results were also obtained by other groups.Citation13–15 However, Wallis et al. found no difference in overall survival (OS) from ICIs treatments between different sexes.Citation16 Moreover, female patients derived more benefits from anti-PD-1/PD-L1 as compared with males when it was used in combination with chemotherapy in NSCLC.Citation17 While the controversy remains, understanding this sex dimorphism in response to anti-PD-L1 is essential to improve the immune checkpoint blockade efficacy.
The present study started with an integrated biomarker evaluation of the clinical data from bladder cancer patients treated with anti-PD-L1 reported in,Citation18 from which it was found that anti-PD-L1 treatment was more effective in male patients than in female patients but no significant differences were observed in the PD-L1 expression, CD8+ Teff signature score and TMB, etc. We determined possible changes after anti-PD-L1 treatment in mice and found that anti-PD-L1 administration significantly decreased the sex hormone levels in male mice possibly through induction of lipid disturbance and inflammation in the testes. We proposed and confirmed that downregulation of sex hormone levels, which can be attained by applying a narrow spectrum antibiotic colistin, dramatically enhances the antitumor efficacy of anti-PD-L1 treatment of murine colon adenocarcinoma in male mice.
Materials and methods
Data source and processing
A cohort of patients receiving anti-PD-L1 (atezolizumab) treatment with genomic, transcriptomic, and matched clinical data (n = 348) were downloaded from http://research-pub.gene.com/IMvigor210CoreBiologies under the Creative Commons 3.0 license.Citation18 IMvigor210 was a large phase 2 trials investigating the clinical activity of PD-L1 blockade with atezolizumab (1200 mg 3 weekly) in metastatic urothelial carcinoma patients (NCT02108652, NCT02951767). This trial enrolled two cohorts of patients, including those who had failed previous platinum-based chemotherapy and those who are first-line cisplatin-ineligible. Among which, bladder cancer (195 patients) accounts for most cases and thus is used in this study. Data from the Cancer Genome Atlas bladder cancer (TCGA-BLCA) was downloaded with the CGDSR R package. The survival analyses were performed using the R survival package and Kaplan–Meier plots were generated using the R survminer package.
Animals and treatments
C57BL/6 N mice (8-week old) were purchased from the Vital River Laboratory Animal Technology Co. Ltd (Beijing, China). All mice were maintained under specific pathogen-free conditions and performed in accordance with the guidelines of the Animal Care and Use Committee of Tsinghua University (No. 20-LZ1#). For the anti-PD-L1 treatment, mice were treated intraperitoneally with 200 μg αPD-L1 (BE0101, BioXCell) or IgG2b isotype (BE0090, BioXCell) twice at 3 days apart. For antibiotic treatment, 1 g/L colistin was given in the drinking water starting from the indicated time and throughout the experiment. For surgical orchidectomy, male mice underwent bilateral orchidectomy via scrotal incision under pentobarbital anesthesia as previously described.Citation19
MC38 colon tumor cells were from the American Type Culture Collection (ATCC), and were cultured in 5% CO2 and maintained in Dulbecco’s modified Eagle’s medium supplemented with 10% heat-inactivated fetal bovine serum. Mice were inoculated subcutaneously with 5 × 105 MC38 cells at the right flank. Tumor volumes were measured every 3–4 days with an electronic caliper and reported as volume using the formula (width2 × length)/2.
Detection of sex hormones, TNFα, and histamine
Serum samples were collected and examined for the concentration of estradiol (Abbkine), testosterone (Abbkine), FSH (Abnova), LH (Abnova), and TNFα (eBioscience) by ELISA. For the detection of histamine, fresh plasma samples were collected in EDTA (ethylenediaminetetraacetic acid) tubes and assayed by ELISA (Abnova).
Flow cytometric analysis
The immune infiltration in the testis was analyzed as previously described.Citation20 Briefly, testis tissues were collected, mechanically dissociated, and then digested in PBS containing 1 mg/mL Collagenase IV/0.15 mg/mL DNase I for 40 min at 37°C. After passing through a nylon mesh, cells were blocked with anti-CD16/32 antibody and then stained with conjugated antibodies to CD4-FITC, CD8a-APC, CD45-PE/Cy5, CD25-APC, CD117(c-kit)-APC, CD274(B7-H1)-PE, FcεRIα-FITC, CD34-PE, or FOXP3-PE (all from BioLegend). For intracellular staining, cells were fixed and permeabilized using the Foxp3/Transcription Factor Staining Buffer Set (eBioscience). The samples were assessed with BD Calibur flow cytometry, and the data were analyzed with FlowJo software (V. 10.6.2, Tree Star).
Histopathology
The collected testes were fixed in 4% formalin and embedded in paraffin, cut to 4 μm sections, and stained with hematoxylin and eosin. For oil red O staining, the fixed specimens were infiltrated with 30% sucrose, frozen in OCT, sectioned to 6 μm and then stained with oil red O (Sigma–Aldrich). Nuclei were counterstained with hematoxylin. Oil Red O staining intensity was quantified using the IHC toolbox plugin of the ImageJ software.
RNA sequence analysis
The total RNA extraction, whole transcriptome libraries preparation, and RNA sequencing were performed by the Majorbio Bio-pharm Technology Co., Ltd (Shanghai, China). Briefly, the total RNA was extracted from the tissue using TRIzol® Reagent according the manufacturer’s instructions (Invitrogen), and genomic DNA was removed using DNase I (TaKara). The RNA-seq transcriptome library was prepared following TruSeq™ RNA sample prep Kit (Illumina, San Diego, CA, USA), and was subsequently sequenced with the Illumina HiSeq xten. The principal component analysis (PCA) was conducted with the R package DESeq2, and the top 200 contributing genes in the direction of PC2 were then subjected to Gene Ontology (GO) analysis using the R package clusterprofiler. Genes with less than 30 read counts were removed from the subsequent analysis. Gene set enrichment analysis (GSEA) was also performed with a cluster profiler. The C5 gene sets from the Molecular Signature Database (MSigDB) was selected as the reference gene set, and P < .05 was chosen as the cutoff criteria. Gene set variation analysis (GSVA) was performed using the GSVA package.
16S rRNA gene sequence analysis
Genomic DNA was extracted from the fecal sample using the QIAamp DNA Stool Mini Kit (Qiagen, Germany) according to the manufacturer’s instructions. The V3–V4 hypervariable region of 16S rRNA gene was amplified with universal primers: 338 F (5′-ACTCCTACGGGAGGCAGCA-3′) and 806 R (5′-GGACTACHVGGGTWTCTAAT-3′) by PCR (ABI GeneAmp 9700) with a 20 μL reaction system (TransGen, China) containing 4 μL 5× FastPfu Buffer, 2 μL 2.5 mM dNTPs, 0.8 μL Forward Primer (5 μM), 0.8 μL Reverse Primer (5 μM), 0.4 μL FastPfu Polymerase, 0.2 µL BSA, and 10 ng template DNA. The procedure was as follows: 3 min of denaturation at 95°C, 27 cycles of 30 s at 95°C (denaturation), 30 s for annealing at 55°, 45 s at 72°C (elongation), and a final extension at 72°C for 10 min. PCR products were extracted from 2% agarose gel and purified using the AxyPrep DNA Gel Extraction Kit (Axygen Biosciences, USA). Then, the PCR products were quantified using Quantus™ Fluorometer (Promega, Madison, USA) and sequenced with an Illumina MiSeq platform according to the standard protocols (Shanghai Majorbio Bio-Pharm Technology Co. Ltd., Shanghai, China). The high-quality sequences were clustered into OTUs with 97% similarity cutoff using UPARSE (V. 7.1, http://drive5.com/uparse/). The taxonomy of each OTU representative sequence was analyzed by the RDP Classifier (V. 2.2) against the Silva Database (Release128, www.arb-silva.de) using a confidence threshold of 0.7. Alpha diversity was calculated for the observed OTUs, Shannon index, Simpson index, Chao1 index, and ACE index using the vegan package in R software. For beta-diversity, PCA was performed using the R package ade4. Analysis of similarity (ANOSIM) and permutational multivariate analysis of variance (PERMANOVA) of Bray–Curtis distances was performed to analyze the similarity between groups using the vegan package. Statistical analysis of taxonomic and functional profiles (STAMP) was conducted using the statistics software STAMP v2.1.3. The R package Tax4Fun was used to predict the functional changes in gut microbiota.
Statistical analysis
Statistical analyses were carried out using GraphPad Prism 8.0 (GraphPad Software Inc., USA). All continuous variables were presented as means ± SEM and compared between groups using Student’s t test unless otherwise indicated. P < .05 was considered to be statistically significant.
Results
Male patients appeared a better outcome but no preexisting determinants were identified
We first analyzed the clinical cancer data reported in,Citation18 in which cancer types with low numbers of tissue samples were excluded. The analysis included 195 bladder cancer patients (IMvigor210), of whom 42 (21.5%) are females and 153 (78.5%) are males. As shown in , male patients treated with anti-PD-L1 had a statistically significantly improved OS as compared to females (P < .05) and, moreover, male gender was significantly associated with OS in univariate Cox regression analyses (HR, 0.66; 95% CI, 0.45–0.98; P < .05). In contrast, the analysis of 406 patients from The Cancer Genome Atlas bladder cancer (TCGA-BLCA) cohort shows no significant differences in the OS between females (26.4%) and males (73.6%) according to the univariate analysis (HR, 0.87; 95% CI, 0.63–1.20; P < .05), hinting that the sex-related differences in tumor immunotherapy responses occurred after treatment. We then performed an integrated biomarker evaluation of the pre-treatment tumor samples from 195 bladder cancer patients, as reported in.Citation18 No significant difference was observed in the preexisting immunity as represented by PD-L1 gene expression in immune cells and CD8+ Teff signature score (). Similar results were also observed for TGF-β signal pathway, TMB and computationally predicted tumor neoantigen burden (). It is thus concluded that the anti-PD-L1 treatment achieved much better outcomes for male patients than for female patients and that this sex dimorphism in response to anti-PD-L1 might not be aroused by the preexisting determinants of response.
Figure 1. Sex dimorphism in response to anti-PD-L1. (a) Overall Survival (OS) between male and female bladder cancer patients with (left) or without (right) anti-PD-L1 treatment (log rank test). (b) Hazard ratio (HR) estimates for OS in Univariate Cox regression analysis. (c) PD-L1 protein expression on tumor cells (TC) or immune cells (IC) was not different between sex (Pearson’s Chi-squared test). There were no significant differences between male and female patients in neoantigen burden (d), TGFB1 gene expression (e), tumor mutation burden, TMB (f), or CD8+ Teff signature score (g). (Wilcoxon test). F, Female; M, Male
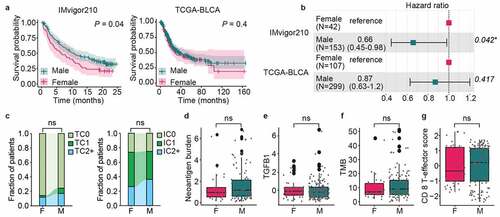
Immunotherapy decreased the sex hormone levels in male mice
We then examined the responses of male and female mice after anti-PD-L1 treatment. First, the serum sex hormone (testosterone and estradiol) concentrations were measured in both male and female mice 1 week later post-treatment with double intraperitoneal (IP) injection of 200 μg anti-PD-L1 or Isotype IgG. Treatment with anti-PD-L1 showed no obvious impact on the sex hormone levels of female mice (). In contrast, the serum levels of both testosterone and estradiol were significantly decreased in male mice receiving anti-PD-L1 treatment, as compared with IgG isotype control (). Considering the widely reported immune-related endocrine dysfunction following the use of immune checkpoint inhibitors especially such as hypophysitis,Citation21 the serum levels of luteinizing hormone (LH) and follicle-stimulating hormone (FSH) were also analyzed. As detailed in , anti-PD-L1 treatment has no effect on the secretion of gonadotropins in both male and female mice, suggesting that the decreased sex hormone levels in male mice are not due to the impact of anti-PD-L1 on the function of the pituitary. Mice feces were collected for 16S rRNA sequencing-based bacterial community analysis 1 week later after the anti-PD-L1 treatment. As shown in , no substantial effects of anti-PD-L1 on the alpha diversity index was observed in both male and female mice feces. However, the analysis of similarities (ANOSIM) and permutational multivariate analysis of variance (PERMANOVA) of Bray–Curtis distances reveals a significant impact of anti-PD-L1 on the composition of the gut microbiota in female mice (ANOSIM, R = 0.5, P = .025; PERMANOVA, pseudo-F = 2.456, P = .027) rather than in male mice (ANOSIM, R = −0.0625, P = .561; PERMANOVA, pseudo-F = 1.055, P = .416). Additionally, the principal component analysis (PCA) showed a similar result, i.e., an obvious difference in the distribution along the second principal component PC2 in female mice (PC1 and PC2 accounted for 30.27% and 22.77% of the total variance, respectively) (). Further analysis of the differential microbes at family level revealed that the abundance of Lachnospiraceae is significantly downregulated in the female mice after treatment with anti-PD-L1 (), which are associated with good response to ICIs.Citation22,Citation23 In conclusion, anti-PD-L1 administration reduces sex hormone levels in male mice, whereas reduces the richness of Lachnospiraceae in female mice.
Figure 2. Sex dimorphism in response to anti-PD-L1. Serum levels of testosterone and estradiol in female (a) or male (b) mice one week later after twice administration of anti-PD-L1 or Isotype IgG. Changes of serum LH and FSH in female (c) or male (d) mice post treatment with anti-PD-L1. (e) No differences were observed in alpha diversity of the gut microbiota between the treated group and the control group in either males or females. a-e, Mann–Whitney test. (f) Principal component analysis showed that gut microbiota composition was largely changed after anti-PD-L1 treatment in female mice at OTU level (ANOSIM, R = 0.323, P = .002; PERMANOVA, pseudo-F = 1.879, P = .001). (g) Statistical analysis of taxonomic and functional profiles (STAMP) showing differential families between FPD and FCT. P-values were derived from a White’s non-parametric t-test. Female IgG control group, FCT; Female anti-PD-L1 treated group, FPD; Male IgG control group, MCT; Male anti-PD-L1 treated group, MPD. All data were presented as means ± SEM. *P < .05
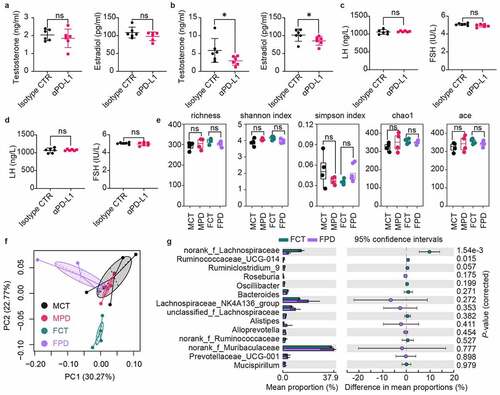
Lipid disturbance and inflammation in the testes of male mice treated with anti-PD-L1
The interaction between gut microbiota and tumor immunotherapy in female mice has been reported.Citation24,Citation25 Here, we focus on the impact of anti-PD-L1 on sex hormones in male mice. We first performed RNAseq transcriptome analysis of the testes of male mice 7 days after anti-PD-L1 treatment. The PCA plot revealed a good separation along the second principal component PC2 (). Therefore, the top 200 contributing genes in the direction of PC2 were subjected for Gene Ontology (GO) analysis, and the results show that fatty acid metabolic process is significantly affected (). Similarly, gene set enrichment analysis (GSEA) indicates a significant increase in lipid metabolic pathways in the group treated with anti-PD-L1 (). Furthermore, Gene Set Variation Analysis (GSVA) was used to score individual samples against C5 gene sets from Molecular Signature Database (MSigDB).Citation26 The results show that pathways involved in both lipid transportation and inflammation are affected by anti-PD-L1 treatment ().
Figure 3. RNAseq analysis of the testes collected one week later after twice administration of anti-PD-L1. (a) Principal component analysis of top 500 most variant genes. (b) Top 200 contributing genes in the direction of PC2 in (a) was subjected for Gene Ontology analysis. (c) Gene set enrichment analysis (GSEA) showed enrichment of lipid metabolic pathways in anti-PD-L1 treated group. (d) Gene Set Variation Analysis (GSVA) against C5 gene sets from the Molecular Signatures Database
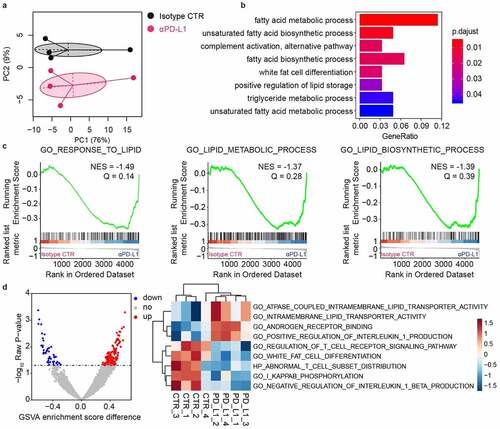
We then collected testis samples and performed tissue staining and immune infiltration analysis 1 week after double administration of anti-PD-L1 and IgG isotypes. Hematoxylin-eosin (HE) staining shows no obvious pathological changes in the group treated with anti-PD-L1 (). However, the results from oil red O staining show a significant accumulation of lipids in the testis interstitial tissue after exposure to anti-PD-L1 (). In addition, the expression of PD-L1 on both CD45+ and CD45− subpopulations is significantly downregulated in the anti-PD-L1 groups, hinting possible disruption of the inherent immunosuppressive circumstances (). Considering that IgG antibodies can trigger the activation of the mast cells and the resultant histamine release may affect the steroidogenesis in Leydig cells,Citation27,Citation28 both the infiltration of mast cells in the testes and the histamine levels in the blood was analyzed. As shown in , there is no substantial difference in the infiltration of mast cells after treatment with anti-PD-L1, while the blood histamine levels in the anti-PD-L1 group are significantly reduced. The infiltration of both CD45+ leukocytes and CD8+ T lymphocytes is not influenced by the anti-PD-L1 treatment (). In contrast, there is a significant increase in the infiltration of CD4+ T lymphocytes but not the CD4+CD25+Foxp3+ Treg cells in the group treated with anti-PD-L1 (). Moreover, a significant increase in the blood levels of TNFα appears in the anti-PD-L1 group (). Collectively, these results suggest that anti-PD-L1 treatment can lead to both disturbance of lipid metabolism and inflammation in the testes of male mice received anti-PD-L1 treatment.
Figure 4. Lipid accumulation and immune infiltration in the testes of male mice. The testes were collected for histology analysis and immune infiltration analysis one week later after twice administration of anti-PD-L1. HE staining (a) and Oil Red O staining (b) of the testis tissue showed increased inclusions of lipid droplets in the interstitial Leydig cells from anti-PD-L1 treated mice, scale bars are 100 μm. Mann–Whitney test. (c) PD-L1 protein expression on either CD45+ (Mann–Whitney test) or CD45− (t-test) cells in the testes. (d) Percentage of CD34+ c-Kit+ mast cells in total cells of the testes and serum histamine levels. Percentage of CD45+ (e), CD8+ (f), CD4+ (g), and CD4+ CD25+ Foxp3+ Treg cells (h) in total cells. (i) Serum TNFα levels were significantly increased in anti-PD-L1 treated group compared with the IgG isotype control. (d-f & h-i), Mann–Whitney test; (g), t-test. All data were presented as means ± SEM. *P < .05, **P < .01
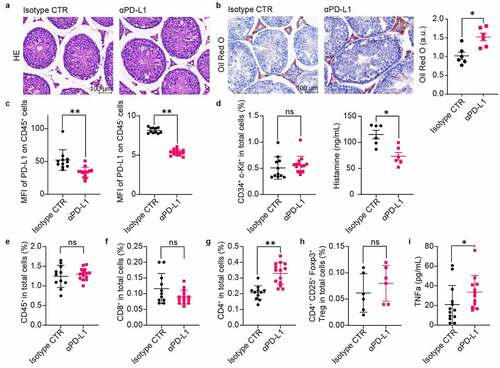
Downregulating sex hormone levels enhanced the antitumor efficiency of anti-PD-L1
We next asked whether the downregulated sex hormone levels caused by anti-PD-L1 could in turn enhance the antitumor efficiency. Male mice, after castration, were subcutaneously implanted with MC38 tumor cells and given two injections of anti-PD-L1 or isotype IgG on day 7 and day 10 post-tumor inoculations. As shown in , castration had no influence on the tumor growth but could significantly enhance the antitumor efficacy of anti-PD-L1, indicating that sex hormone levels play a vital role in tumor immunotherapy. In addition, the composition of the fecal microbiota was analyzed 2 weeks later after castration. Both the composition (ANOSIM, R = 0.656, P = .021; PERMANOVA, pseudo-F = 4.163, P = .015) and possible function of the gut microbiota showed obvious changes after castration ( and S1). Considering that changes in the commensal microbial community may in turn affect sex hormone levels,Citation29 we analyzed the serum sex hormone levels of mice received 1 week’s administration of colistin, a narrow spectrum antibiotic. In female mice, colistin did not influence the serum levels of either testosterone or estradiol, but it would induce a noticeable decline in the sex hormone levels when they were used during anti-PD-L1 treatment (). In contrast, colistin could markedly decrease the testosterone levels in male mice, while attenuated anti-PD-L1 aroused downregulation of the estradiol levels (). As a result, the ratio of estradiol to testosterone was substantially elevated by colistin. We examined the effect of colistin on the antitumor efficacy of anti-PD-L1 in both female and male mice. As shown in , the antitumor efficacy of anti-PD-L1 is decreased in female mice but significantly enhanced in male mice. We determined the serum sex hormone levels at the end of the experiment. In female mice, both testosterone and estradiol in anti-PD-L1 treated group was kept at relatively higher levels than others (). The anti-PD-L1 treatment induced increase in sex hormone levels was neutralized by colistin in female mice. In contrast, the anti-PD-L1 treatment could significantly downregulate the testosterone levels in male mice (). Moreover, colistin could further decrease the testosterone levels while increasing estradiol levels during the anti-PD-L1 treatment. To further reveal this sex-dependent interplay between sex hormones and gut microbiota, feces were collected at the end of the experiment and subjected to microbial community analysis. Results showed that both anti-PD-L1 and colistin could elicit more obvious changes in both diversity and function of the gut microbiota from tumor-bearing male mice than that from the females (Figure S2). Importantly, changes in the gut microbiota induced by anti-PD-L1 treatment were consistent with those inflicted by colistin. Collectively, these results reveal that sex hormones play a vital role during tumor immunotherapy and may serve as an important target to enhance the antitumor efficacy of anti-PD-L1.
Figure 5. Sex hormone levels affect the antitumor efficacy of anti-PD-L1. (a) C57BL/6 N male mice received castration or shamed operations on day −14 and were inoculated subcutaneously with 5 × 105 MC38 tumor cells on day 0, then treated with 200 μg of anti-PD-L1 or isotype IgG on day 7 and 10 (n = 6). (b) Feces were collected for 16S rRNA sequencing 2 weeks later after castration or shamed operations in male mice. Principal component analysis showed an obvious change in the gut microbiota composition at OTU level (ANOSIM, R = 0.656, P = .021; PERMANOVA, pseudo-F = 4.163, P = .015). Serum sex hormone levels in female (c) or male (d) mice after treatment with colistin and/or anti-PD-L1 were examined by ELISA. Female (e) or male (f) mice were inoculated subcutaneously with 5 × 105 MC38 cells and treated with 200 μg of αPD-L1 or isotype IgG on days 7 and 10 (n = 6). For colistin treated group, 1 mg/mL colistin was added to the sterile drinking water from day 7. Either female (g) or male (h) mice were sacrificed at the end of the experiment and blood were harvested for the assay of sex hormone levels. (d-f & h-i), Mann–Whitney test; (g), t-test. All data were presented as means ± SEM. *P < .05, **P < .01
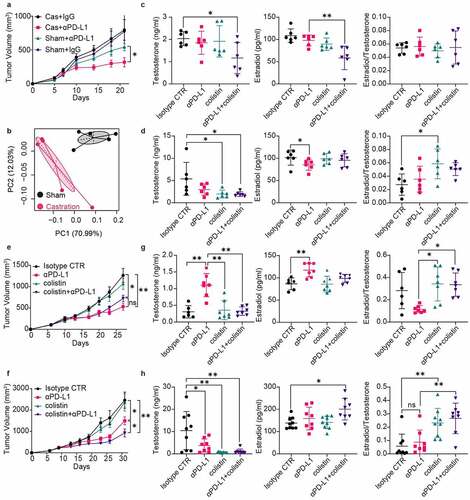
Discussion
While the effects of the sex hormones on immune responses have been well documented,Citation10,Citation30,Citation31 the mechanisms of the sex dimorphism in the response of ICIs are not adequately understood. Analysis of the anti-PD-L1 treatment in bladder cancer patients confirmed the better outcomes for male patients, and meanwhile, no significant difference in the currently identified biomarkers before the acceptance of immunotherapy. A significant reduction in sex hormone levels in male mice receiving anti-PD-L1 treatment was observed, which may be related to the induction of lipid disturbance and inflammation in the testes, while a decline in the richness of Lachnospiraceae was observed in female mice (). Moreover, we confirmed that the antitumor efficiency of anti-PD-L1 was affected by sex hormone levels and downregulated testosterone levels by, e.g., an antibiotic colistin, which was conductive to an improved antitumor outcome in male mice.
Figure 6. Sex dimorphism in the response to ICIs. (a) Anti-PD-L1 induces a significant decrease in sex hormone levels of male mice, possibly through induction of lipid disturbance and inflammation in the testes. The deceased testosterone levels, which can be attained by castration or oral administration of colistin, could dramatically enhance the antitumor efficiency of anti-PD-L1. (b) In normal female mice, anti-PD-L1 has no impact on sex hormone levels while reduces the richness of Lachnospiraceae in the gut. In tumor bearing female mice, anti-PD-L1 has a protection on the estradiol levels, which will be neutralized when used in combination with colistin. The antitumor efficiency of anti-PD-L1 is decreased by colistin
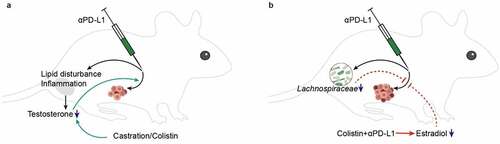
Both estradiol receptor (ER) and androgen receptor (AR) are extensively expressed in lymphocytes and myeloid-derived cells.Citation32,Citation33 Upon binding to the ligand, both of them can signal through either DNA-binding-dependent or DNA-binding-independent mechanisms to regulate the expression of downstream target genes or elicit the cytoplasmic signaling cascades.Citation32,Citation33 Thus, sex hormones can affect in various ways both innate and adaptive immune responses.Citation6 It has been reported that AR suppresses the transcription of PD-L1, while ER up-regulates PD-L1 protein expression in cancer cells, which may affect the response to immune checkpoint therapy.Citation34,Citation35 Our analysis of the clinical data from bladder cancer patients shows however, no differences in both PD-L1 expression and CD8+ Teff signature score between male and female bladder cancer patients before anti-PD-L1 treatment. Instead, both the serum levels of testosterone and estradiol are significantly decreased in male mice after anti-PD-L1 treatment, compared to the females. The enhanced antitumor efficacy of anti-PD-L1 in male mice after deprivation of sex hormones through castration indicates that sex hormones are not only involved in the sex dimorphism in response to immune checkpoint blockade but also a potential biomarker for the ICIs.
Generally, androgen tends to suppress immune function, while estradiol enhances both cellular and humoral immune responses.Citation6,Citation32 Such trends were also observed among the male mice in the present study (); however, the situation seemed different in female mice. Testosterone levels in tumor-bearing female mice were significantly increased by anti-PD-L1 as compared to the IgG control group, with an obvious decrease in the ratio of estradiol to testosterone (). Furthermore, the increased testosterone levels induced by anti-PD-L1 are attenuated by colistin (). Nonetheless, for female mice, the anti-PD-L1 treatment showed superior antitumor efficiency compared to the combined therapy with anti-PD-L1 and colistin (). It has been reported that testosterone could protect against type 1 diabetes upon reaching a certain concentration (less than 2 ng/mL).Citation36 Here, the testosterone levels in tumor-bearing female mice treated with anti-PD-L1 were higher than those of others with tumors, but were still lower than those from tumor-free mice. This indicates that there is a testosterone threshold for its inhibitory effects on the antitumor efficacy of anti-PD-L1. The detailed mechanisms for the effects of either testosterone or estradiol deserve further studies.
Endocrine dysfunction has emerged as one of the most common immune-related adverse events (irAEs) following the use of ICIs.Citation37 Among which, hypophysitis is common and often accompanied by low or inappropriately normal FSH and/or LH.Citation21 However, in this study, no obvious changes in either FSH or LH were observed in either male or female mice 1 week after double injection of anti-PD-L1. This might be attributed to the short treatment time since hypophysitis generally occurs 8–12 weeks after ICIs treatment (36). In contrast, both testosterone and estradiol levels are significantly decreased by anti-PD-L1 in male mice (). Further studies showed increased intracellular lipid droplets of Leydig cells and amplified inflammation in the testes of male mice receiving anti-PD-L1 treatment. The lipid droplets of Leydig cells serve as a large pool of cholesteryl ester, providing cholesterol as the precursor substrate for testosterone biosynthesis.Citation38 However, increased accumulation of cytoplasmic lipid droplets is associated with reduced testosterone production in Leydig cells.Citation39 In addition, increased accumulation of cytoplasmic lipid droplets in non-adipose cells is often observed under inflammatory conditions.Citation40 Here, we also observed an increased infiltration of CD4+ T lymphocytes in the testes and an elevation of TNFα in the blood of male mice treated with anti-PD-L1, indicating the occurrence of inflammation. It has been reported that both anti-PD-1Citation41 and anti-CTLA-4Citation42 triggered the production of TNFα that decreased the steroidogenesis in TM3 Leydig cells.Citation43 All these suggest that disturbances in lipid metabolism and inflammation in the testes of male mice elicited by anti-PD-L1 may contribute to the decreased testosterone secretion. More recently, Jung et al. reported that TNFα could promote lipid droplet formation in human endothelial cells through induction of acyl-CoA synthetase 3.Citation44 In the present study, we hypothesized that the increased TNFα induced by anti-PD-L1 may also inhibit the testosterone production in Leydig cells by promoting lipid droplet formation. Nevertheless, more efforts are needed to uncover the mechanistic links between PD-L1 blockade and reduced testosterone.
Disruption of gut microbiota with antibiotics, especially the broad-spectrum antibiotics has been associated with poor outcomes of tumor immunotherapy.Citation24,Citation45,Citation46 In the present study, a detrimental effect of the narrow spectrum antibiotic colistin also appeared in female mice (). However, the antitumor efficiency of anti-PD-L1 was significantly enhanced by colistin in male mice through downregulating the sex hormone levels (). Although the precise mechanisms remain to be investigated in the future, colistin can notably impact the sex hormone levels of male mice without severe destruction of gut microbial diversity (Figure S1A). The gut microbiota plays a vital role in the intestinal androgen reabsorption through deglucuronidation of testosterone and dihydrotestosterone,Citation47 thus serving as a new possible target for androgen regulation during tumor immunotherapy. Traditional androgen deprivation therapy can cause adverse effects including bone loss, metabolic changes, gynecomastia, increased cardiovascular events,Citation48 and suppress the efficiency of antitumor immunotherapy.Citation19 Thus, downregulation of testosterone levels through regulation of gut microbiota with certain antibiotics may present a novel way to enhance the efficiency of antitumor immunotherapy.
In conclusion, the present study shows that sex hormones play a vital role in the sexual dimorphism in the host’s response to anti-PD-L1 therapy. The analysis of literature data indicated that anti-PD-L1 treatment achieved a better outcome in male patients, whereas there was no difference in in CD8+ T lymphocytes infiltration, PD-L1 expression, TMB, etc., between male and female patients before ICIs treatment. A significant decrease in sex hormone levels in male mice was observed after anti-PD-L1 treatment, which was possibly mediated by disturbances in lipid metabolism or inflammation in the testes. Downregulation of sex hormones was then attempted, by applying appropriate antibiotics in the present study, and enhanced the antitumor efficiency of anti-PD-L1 in male mice bearing colon adenocarcinoma MC38. All these results suggest that sex hormone secretion may serve as novel paths toward enhancing the antitumor efficacy of PD-L1 blockade in male patients.
Ethics approval
All mice were performed in accordance with guidelines of the Animal Care and Use Committee of Tsinghua University (No. 20-LZ1#).
Author contributions
LW performed the formal analysis and wrote the manuscript. GJ and ZL were responsible for the funding acquisition. NJ, XL, HZ and WZ participated in the experiment. WL participated in the design of the study. GJ and ZL supervised the whole study and wrote the manuscript.
Supplemental Material
Download ()Acknowledgments
The authors would like to acknowledge their sincere thanks to Prof. Jianzhong Wu from the Dept. of Environmental and Chemical Engineering, University of California, Riverside, USA, for his valuable comments and help in preparing this manuscript.
Disclosure statement
The authors declare that they have no competing interests.
Availability of data and material
The RNA-Seq data have been deposited in NCBI Gene Expression Omnibus with the accession number GSE171229. The 16S rRNA sequencing data set has been deposited in the SRA database of NCBI with the Accession ID of SRP314123. All other data needed to evaluate the conclusions in this paper are presented in the paper and/or the Supplementary Materials. Additional data related to this paper may be requested by the authors.
Supplementary material
Supplemental data for this article can be accessed on the publisher’s website
Additional information
Funding
References
- Eroglu Z, Zaretsky JM, Hu-Lieskovan S, Kim DW, Algazi A, Johnson DB, et al. High response rate to PD-1 blockade in desmoplastic melanomas. Nature. 2018;553(7688):347–11. doi:10.1038/nature25187.
- Ribas A, Wolchok JD. Cancer immunotherapy using checkpoint blockade. Science. 2018;359(6382):1350–1355. doi:10.1126/science.aar4060.
- Tan S, Li D, Zhu X. Cancer immunotherapy: pros, cons and beyond. Biomed Pharmacother. 2020;124:109821. doi:10.1016/j.biopha.2020.109821.
- Gavrielatou N, Doumas S, Economopoulou P, Foukas PG, Psyrri A. Biomarkers for immunotherapy response in head and neck cancer. Cancer Treat Rev. 2020;84:101977. doi:10.1016/j.ctrv.2020.101977.
- Owens B. Gut bacteria link to immunotherapy sparks interest. Nat Biotechnol. 2018;36(2):121–123. doi:10.1038/nbt0218-121.
- Klein SL, Flanagan KL. Sex differences in immune responses. Nat Rev Immunol. 2016;16(10):626–638. doi:10.1038/nri.2016.90.
- Rubtsova K, Marrack P, Rubtsov AV. Sexual dimorphism in autoimmunity. J Clin Invest. 2015;125(6):2187–2193. doi:10.1172/JCI78082.
- Bray F, Ferlay J, Soerjomataram I, Siegel RL, Torre LA, Jemal A. Global cancer statistics 2018: GLOBOCAN estimates of incidence and mortality worldwide for 36 cancers in 185 countries. CA Cancer J Clin. 2018;68(6):394–424. doi:10.3322/caac.21492.
- Klein SL, Jedlicka A, Pekosz A. The Xs and Y of immune responses to viral vaccines. Lancet Infect Dis. 2010;10(5):338–349. doi:10.1016/S1473-3099(10)70049-9.
- Fischinger S, Boudreau CM, Butler AL, Streeck H, Alter G. Sex differences in vaccine-induced humoral immunity. Semin Immunopathol. 2019;41(2):239–249. doi:10.1007/s00281-018-0726-5.
- Lin PY, Sun L, Thibodeaux SR, Ludwig SM, Vadlamudi RK, Hurez VJ, et al. B7-H1-dependent sex-related differences in tumor immunity and immunotherapy responses. J Immunol. 2010;185(5):2747–2753. doi:10.4049/jimmunol.1000496.
- Conforti F, Pala L, Bagnardi V, De Pas T, Martinetti M, Viale G, et al. Cancer immunotherapy efficacy and patients’ sex: a systematic review and meta-analysis. Lancet Oncol. 2018;19(6):737–746. doi:10.1016/S1470-2045(18)30261-4.
- Wang C, Qiao W, Jiang Y, Zhu M, Shao J, Ren P, et al. Effect of sex on the efficacy of patients receiving immune checkpoint inhibitors in advanced non-small cell lung cancer. Cancer Med. 2019;8(8):4023–4031. doi:10.1002/cam4.2280.
- Wu Y, Ju Q, Jia K, Yu J, Shi H, Wu H, et al. Correlation between sex and efficacy of immune checkpoint inhibitors (PD-1 and CTLA-4 inhibitors). Int J Cancer. 2018;143(1):45–51. doi:10.1002/ijc.31301.
- Pinto JA, Vallejos CS, Raez LE, Mas LA, Ruiz R, Torres-Roman JS, et al. Gender and outcomes in non-small cell lung cancer: an old prognostic variable comes back for targeted therapy and immunotherapy? ESMO Open. 2018;3(3):e000344. doi:10.1136/esmoopen-2018-000344.
- Wallis CJD, Butaney M, Satkunasivam R, Freedland SJ, Patel SP, Hamid O, et al. Association of patient sex with efficacy of immune checkpoint inhibitors and overall survival in advanced cancers: a systematic review and meta-analysis. JAMA Oncol. 2019;5(4):529–536. doi:10.1001/jamaoncol.2018.5904.
- Conforti F, Pala L, Bagnardi V, Viale G, De Pas T, Pagan E, et al. Sex-based heterogeneity in response to lung cancer immunotherapy: a systematic review and meta-analysis. J Natl Cancer Inst. 2019;111(8):772–781. doi:10.1093/jnci/djz094.
- Mariathasan S, Turley SJ, Nickles D, Castiglioni A, Yuen K, Wang Y, et al. TGFbeta attenuates tumour response to PD-L1 blockade by contributing to exclusion of T cells. Nature. 2018;554(7693):544–548. doi:10.1038/nature25501.
- Pu Y, Xu M, Liang Y, Yang K, Guo Y, Yang X, et al. Androgen receptor antagonists compromise T cell response against prostate cancer leading to early tumor relapse. Sci Transl Med. 2016;8(333):333ra47. doi:10.1126/scitranslmed.aad5659.
- Mossadegh-Keller N, Sieweke MH. Characterization of mouse adult testicular macrophage populations by immunofluorescence imaging and flow cytometry. Bio Protoc. 2019;9(5):5. doi:10.21769/BioProtoc.3178.
- Chang LS, Barroso-Sousa R, Tolaney SM, Hodi FS, Kaiser UB, Min L. Endocrine toxicity of cancer immunotherapy targeting immune checkpoints. Endocr Rev. 2019;40:17–65.
- Peng Z, Cheng S, Kou Y, Wang Z, Jin R, Hu H, et al. The gut microbiome is associated with clinical response to anti-PD-1/PD-L1 immunotherapy in gastrointestinal cancer. Cancer Immunol Res. 2020;8(10):1251–1261. doi:10.1158/2326-6066.CIR-19-1014.
- Wang L, Jing N, Liu X, Jiang G, Liu Z. Nurturing and modulating gut microbiota with jujube powder to enhance anti-PD-L1 efficiency against murine colon cancer. J Funct Foods. 2020;64:103647. doi:10.1016/j.jff.2019.103647.
- Vetizou M, Pitt JM, Daillere R, Lepage P, Waldschmitt N, Flament C, et al. Anticancer immunotherapy by CTLA-4 blockade relies on the gut microbiota. Science. 2015;350(6264):1079–1084. doi:10.1126/science.aad1329.
- Viaud S, Saccheri F, Mignot G, Yamazaki T, Daillere R, Hannani D, et al. The intestinal microbiota modulates the anticancer immune effects of cyclophosphamide. Science. 2013;342(6161):971–976. doi:10.1126/science.1240537.
- Subramanian A, Tamayo P, Mootha VK, Mukherjee S, Ebert BL, Gillette MA, et al. Gene set enrichment analysis: a knowledge-based approach for interpreting genome-wide expression profiles. Proc Natl Acad Sci U S A. 2005;102(43):15545–15550. doi:10.1073/pnas.0506580102.
- Elieh Ali Komi D, Shafaghat F, Haidl G. Significance of mast cells in spermatogenesis, implantation, pregnancy, and abortion: cross talk and molecular mechanisms. Am J Reprod Immunol. 2020;83(5):e13228. doi:10.1111/aji.13228.
- Okayama Y, Kirshenbaum AS, Metcalfe DD. Expression of a functional high-affinity IgG receptor, Fc gamma RI, on human mast cells: up-regulation by IFN-gamma. J Immunol. 2000;164(8):4332–4339. doi:10.4049/jimmunol.164.8.4332.
- Markle JG, Frank DN, Mortin-Toth S, Robertson CE, Feazel LM, Rolle-Kampczyk U, et al. Sex differences in the gut microbiome drive hormone-dependent regulation of autoimmunity. Science. 2013;339(6123):1084–1088. doi:10.1126/science.1233521.
- Klein SL, Morgan R. The impact of sex and gender on immunotherapy outcomes. Biol Sex Differ. 2020;11(1):24. doi:10.1186/s13293-020-00301-y.
- Ozdemir BC, Dotto GP. Sex hormones and anticancer immunity. Clinical Cancer Research: An Official Journal of the American Association for Cancer Research. 2019;25(15):4603–4610. doi:10.1158/1078-0432.CCR-19-0137.
- Gubbels BMR, Tn J. Androgen-Induced Immunosuppression. Front Immunol. 2018;9:794. doi:10.3389/fimmu.2018.00794.
- Kovats S. Estrogen receptors regulate innate immune cells and signaling pathways. Cell Immunol. 2015;294:63–69.
- Jiang G, Shi L, Zheng X, Zhang X, Wu K, Liu B, et al. Androgen receptor affects the response to immune checkpoint therapy by suppressing PD-L1 in hepatocellular carcinoma. Aging (Albany NY). 2020;12(12):11466–11484. doi:10.18632/aging.103231.
- Yang L, Huang F, Mei J, Wang X, Zhang Q, Wang H, et al. Posttranscriptional Control of PD-L1 Expression by 17beta-Estradiol via PI3K/Akt Signaling Pathway in ERalpha-Positive Cancer Cell Lines. International Journal of Gynecological Cancer: Official Journal of the International Gynecological Cancer Society. 2017;27(2):196–205. doi:10.1097/IGC.0000000000000875.
- Yurkovetskiy L, Burrows M, Khan AA, Graham L, Volchkov P, Becker L, et al. Gender bias in autoimmunity is influenced by microbiota. Immunity. 2013;39(2):400–412. doi:10.1016/j.immuni.2013.08.013.
- Barroso-Sousa R, Barry WT, Garrido-Castro AC, Hodi FS, Min L, Krop IE, et al. Incidence of endocrine dysfunction following the use of different immune checkpoint inhibitor regimens: a systematic review and meta-analysis. JAMA Oncol. 2018;4(2):173–182. doi:10.1001/jamaoncol.2017.3064.
- Wang W, Wei S, Li L, Su X, Du C, Li F, et al. Proteomic analysis of murine testes lipid droplets. Sci Rep. 2015;5(1):12070. doi:10.1038/srep12070.
- Ma Y, Zhou Y, Zhu YC, Wang SQ, Ping P, Chen XF. Lipophagy contributes to testosterone biosynthesis in male rat leydig cells. Endocrinology. 2018;159(2):1119–1129. doi:10.1210/en.2017-03020.
- Pereira-Dutra FS, Teixeira L, de Souza Costa MF, Bozza PT. Fat, fight, and beyond: the multiple roles of lipid droplets in infections and inflammation. J Leukoc Biol. 2019;106(3):563–580. doi:10.1002/JLB.4MR0119-035R.
- Bertrand F, Montfort A, Marcheteau E, Imbert C, Gilhodes J, Filleron T, et al. TNFα blockade overcomes resistance to anti-PD-1 in experimental melanoma. Nat Commun. 2017;8(1):2256. doi:10.1038/s41467-017-02358-7.
- Hardy-Werbin M, Rocha P, Arpi O, Taus Á, Nonell L, Durán X, et al. Serum cytokine levels as predictive biomarkers of benefit from ipilimumab in small cell lung cancer. Oncoimmunology. 2019;8(6):e1593810–e. doi:10.1080/2162402X.2019.1593810.
- Leisegang K, Henkel R. The in vitro modulation of steroidogenesis by inflammatory cytokines and insulin in TM3 Leydig cells. Reprod Biol Endocrinol. 2018;16(1):26. doi:10.1186/s12958-018-0341-2.
- Jung HS, Shimizu-Albergine M, Shen X, Kramer F, Shao D, Vivekanandan-Giri A, et al. TNF-alpha induces acyl-CoA synthetase 3 to promote lipid droplet formation in human endothelial cells. J Lipid Res. 2020;61(1):33–44. doi:10.1194/jlr.RA119000256.
- Iida N, Dzutsev A, Stewart CA, Smith L, Bouladoux N, Weingarten RA, et al. Commensal bacteria control cancer response to therapy by modulating the tumor microenvironment. Science. 2013;342(6161):967–970. doi:10.1126/science.1240527.
- Pinato DJ, Gramenitskaya D, Altmann DM, Boyton RJ, Mullish BH, Marchesi JR, et al. Antibiotic therapy and outcome from immune-checkpoint inhibitors. J Immunother Cancer. 2019;7(1):287. doi:10.1186/s40425-019-0775-x.
- Collden H, Landin A, Wallenius V, Elebring E, Fandriks L, Nilsson ME, et al. The gut microbiota is a major regulator of androgen metabolism in intestinal contents. Am J Physiol Endocrinol Metab. 2019;317(6):E1182–E92. doi:10.1152/ajpendo.00338.2019.
- Nguyen PL, Alibhai SM, Basaria S, D’Amico AV, Kantoff PW, Keating NL, et al. Adverse effects of androgen deprivation therapy and strategies to mitigate them. Eur Urol. 2015;67(5):825–836. doi:10.1016/j.eururo.2014.07.010.