ABSTRACT
While chemotherapy and radiotherapy remain the first-line approaches for the management of most unresectable tumors, immunotherapy has emerged in the past two decades as a game-changing treatment, notably with the clinical success of immune checkpoint inhibitors. Immunotherapies aim at (re)activating anticancer immune responses which occur in two main steps: (1) the activation and expansion of tumor-specific T cells following cross-presentation of tumor antigens by specialized myeloid cells (priming phase); and (2) the immunological clearance of malignant cells by these antitumor T lymphocytes (effector phase). Therapeutic vaccines, adjuvants, monoclonal antibodies, cytokines, immunogenic cell death-inducing agents including oncolytic viruses, anthracycline-based chemotherapy and radiotherapy, as well as adoptive cell transfer, all act at different levels of this cascade to (re)instate cancer immunosurveillance. Intratumoral delivery of these immunotherapeutics is being tested in clinical trials to promote superior antitumor immune activity in the context of limited systemic toxicity.
Introduction
Brief history of anticancer immunity
In 1890, William B. Coley injected dying bacterial cultures (called Coley toxins) as a treatment for various cancers, including soft tissue sarcoma.Citation1 In spite of mixed success, this bacterial immunotherapy inspired the application of Bacillus Calmette–Guérin (BCG), an attenuated form of the tuberculosis-causing bacteria, for malignant indications. In 1990, BCG became among the first antitumoral immunotherapy approved by the Food and Drug Administration (FDA) as an adjuvant treatment of superficial bladder carcinoma for which it remains a standard of care to date.Citation2 In 1994, it was shown that the immune system not only recognizes tumor antigens but also danger signals emitted by cells undergoing stress or abnormal differentiation.Citation3–5 Despite these elements, the role of the immune system against cancer has long been debated, in part due to the evidence that tumor cells could benefit from a proinflammatory environment, including proliferative and proangiogenic signaling.Citation6,Citation7 In the early 2000s, the group of Robert Schreiber demonstrated that interferon gamma (IFNG, best known as IFNγ) plays a crucial role in cancer immunosurveillance and that tumors coming from immunodeficient mice are more immunogenic than tumors arising on immunocompetent ones, giving birth to the concept of immunoediting.Citation8–10 These two fundamental findings led to a regain of interest for cancer immunotherapy, which aims at (re)activating an anticancer immune response.Citation11,Citation12 At the same time, the evidence came up that some conventional treatments, like anthracycline-based chemotherapy or radiotherapy,Citation13,Citation14 which were initially used to impair cancer cell proliferation, were also able to induce the release of damage-associated molecular patterns (DAMPs) and antigens, leading to the activation of an adaptive anticancer immune response.Citation15–18 Nowadays, several immunotherapies are being investigated in oncology: vaccines,Citation19,Citation20 adjuvants,Citation21,Citation22 monoclonal antibodies (mAbs),Citation23,Citation24 virotherapy,Citation25 cytokines,Citation26 and adoptive cell transfer.Citation27,Citation28 Immune checkpoint inhibitors (ICIs) are a subclass of mAbs neutralizing inhibitory immune signals such as programmed cell death 1 (PDCD1, best known as PD1), CD174 (best known as PDL1), or cytotoxic T lymphocyte-associated protein 4 (CTLA4).Citation29,Citation30 Eight ICIs are currently on the market after demonstrating remarkable antineoplastic efficacy first in melanoma, then in a still growing number of cancer histotypes ().Citation31–33
Table 1. Cancer immunotherapeutics approved by the FDA
Mechanisms of anticancer immunity
During tumorigenesis, tumor cells require sustained blood influx and stromal reorganization to support their expansion.Citation34 This environment promotes proinflammatory cytokine release by malignant and stromal cells.Citation35,Citation36 Such cytokines include tumor necrosis factor (TNF), transforming growth factor beta 1 (TGFB1, best known as TGFβ), interleukin 1 beta (IL1B, best known as IL1β), IL6 and IL10, altogether leading to the recruitment of natural killer (NK) cells, NKT cells, macrophages and dendritic cells (DCs) at the tumor site.Citation3,Citation37–39 In turn, recruited immune cells secrete proinflammatory cytokines, like IL12 and IFNγ.Citation40 Tumor-infiltrating NK cells mediate cytotoxic effect on neoplastic cells promoting the release of tumor antigens.Citation41–44 Dying cancer cells and released antigens are ingested by DCs, which undergo activation upon sensing of DAMPs via their pattern recognition receptors (PRRs).Citation45,Citation46 Ultimately, these signals promote the maturation of DCs, in particular the XCR1+CLEC9a+ conventional DC subtype 1 (cDC1),Citation47 which is characterized by the cross-presentation of tumor antigens as well as the expression of co-stimulatory molecules and the secretion of proinflammatory cytokines including IL12, IL6, TNF, and type I IFNs (IFN-I).Citation48–50 Activated DCs migrate to the draining lymph node where they drive naïve CD4+ T cells into the type 1 helper T (TH1) cell lineage.Citation51–53 DCs also prime naïve CD8+ T cells and promote their differentiation into cytotoxic T lymphocytes (CTLs).Citation51,Citation52,Citation54 Similar to NK cells, expanded CTLs can reach the tumor bed via the bloodstream.Citation43 There, they elicit cytotoxicity against the cancer cells which harbor their cognate antigen, mostly through secretion of perforins, granzymes, and granulysins, as well as via the expression of pro-apoptotic receptors like TNF-related apoptosis inducing ligand (TRAIL) or Fas ligand (FASLG).Citation55–58
When tumors have undergone immunoediting and escaped from these anticancer immune mechanisms, a clinically detectable neoplastic mass arises.Citation59 At this stage, the composition of the tumor immune infiltrate influences the outcome of the disease, with the presence of CTLs, among others, as good prognostic factors.Citation60–62 Importantly, the nature and/or density of the tumor-infiltrating populations of leukocytes can be modified by immunotherapy. Immunotherapeutic approaches aim at (re)activating an anticancer immune response, either by enhancing cancer adjuvanticity and/or antigenicity, or by providing some immune cells which harbor cytotoxic functions. Immunotherapeutic interventions that have been approved or which are under clinical evaluation for oncological indications include: [i] some agonists of PRRs like Toll-like receptors (TLRs),Citation63,Citation64 or of their adapters like stimulator of interferon response cGAMP interactor 1 (STING1),Citation65–67 [ii] some agents inducing cancer immunogenic cell death (ICD) like oncolytic viruses (OVs) and some chemotherapeutic agents,Citation68–70 [iii] a plethora of mAbs which targets surface tumor antigens and mediates cytotoxicity,Citation71 [iv] other mAbs belong to the subclass of immune checkpoint modulators and support CTL activation either by blocking co-inhibitory immune signals, or by stimulating co-stimulatory ones at the surface of malignant and/or stromal entities,Citation72 [v] vaccines which aim at feeding DCs with enlarged amounts of tumor antigens to further stimulate T cell priming,Citation73 [vi] adoptive transfer of lymphocytes with tumor killing activity (e.g. NK cells, CTLs),Citation74 and finally [vii] cytokines which can promote an inflammatory environment and participate in immune cell recruitment and activation (; ).Citation75,Citation76
Figure 1. Types of immunotherapies and their targets in the cancer-immunity cycle. Upon immunogenic cell death of cancer cells, dendritic cells (DCs) are recruited to the lesion where they uptake and present tumor antigens to naïve CD8+ T cells, triggering their differentiation into cytotoxic CD8+ T cells which, together with natural killer (NK) cells, eliminate cancer cells. Memory T cells are also generated during this process. Cancer cells may express immune checkpoint ligands contributing to T cell exhaustion. Immunotherapeutics (in red) can act at different levels of the (re)establishment of this anticancer immune response. DAMP, damage-associated molecular pattern; DC, dendritic cell; ICD, immunogenic cell death; ICI, immune checkpoint inhibitor; IFN, interferon; IFN-I, type 1 interferons; IL, interleukin; mAb, monoclonal antibody; TNF, tumor necrosis factor
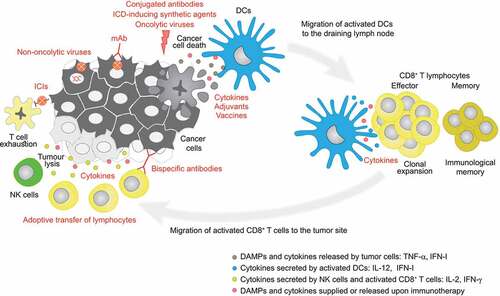
Intratumoral versus systemic injection
Systemic immunotherapies have demonstrated their efficacy to induce durable antitumor immune responses and to increase overall survival (OS) in several solid cancers.Citation77 Yet, a majority of patients experience adverse events which can lead to treatment disruption. Moreover, the immunosuppressive tumor microenvironment efficiently shields off infiltration by cellular mediators of cancer immunosurveillance, thus frequently resulting in the inefficiency of systemic immunotherapies. Preclinical studies have demonstrated that local delivery of immunostimulatory products, such as OVs, cytokines, and PRR agonists could overcome the resistance to systemic immune checkpoint blockade therapies.Citation78–81 These observations have precipitated the evaluation of intratumoral (i.t.) immunotherapy in the clinic.Citation82,Citation83 This dynamic has been supported in 2015 by the FDA approval of talimogene laherparepvec (T-VEC) for i.t. oncolytic virotherapy of melanoma.Citation84,Citation85
The intralesional route offers several advantages over systemic infusion. First, local administration translates into an immediate effect of the drugs on targeted cancer cells. Consequently, lower dosages could be applied without impairing therapeutic efficacy.Citation86 Meanwhile, it reduces treatment cost and opens some opportunities for combinations of drugs. Furthermore, by limiting systemic exposure, off-target toxicity is attenuated, especially inflammation and autoimmunity which are often observed after i.v. injection of immunotherapies. Additionally, in the case of ICD-inducing agents, their topical delivery favors enhanced cytotoxicity and potent release of the tumor antigen repertoire. In this scenario, tumor cells stressed/dying upon treatment work as vaccines and induce a polyclonal immune response. This both diminishes the probability to observe resistance and leverages long-term and systemic protection (responsible for the so-called “abscopal effect”).Citation87–90 Moreover, tumors which show a low immune infiltration (e.g. bone and ovary cancers), and do not receive a sufficient dose of drugs after systemic injection, could benefit from a topical administration.Citation91
For the purpose of this review, we focused on clinical trials involving intratumoral immunotherapy that were initiated during the past 3 y (January 2018–June 2021). Of note, complementary review articles have been published on the topic recently.Citation92–94 Out of 153 clinical trials matching our query on clinicaltrials.gov, 64 are investigating virotherapies, 36 PRR agonists, 17 monoclonal antibodies, 7 cytokines, 5 ICD-inducing synthetic agents, 4 adoptive transfer of lymphocytes, 4 therapeutic vaccines, 2 semi-synthetic adjuvants, 1 attenuated bacterial strain, and 14 a combination of different immunotherapeutic strategies (, ). In the following sections, clinical investigations have been reported according to the immunotherapic strategy involved.
Table 2. Clinical trials involving intratumoral immunotherapies started between January 2018 and June 2021
Antibodies
Therapeutic mAbs can either (a) directly bind to surface markers on malignant cells or on their stromal accomplices (e.g. HER2 in some breast cancers), (b) target receptors on leukocytes (e.g. PD1 on T lymphocytes),Citation95 or (c) neutralize some trophic factors which support tumor growth (e.g. vascular endothelial growth factor [VEGF]), in order to precipitate the elimination of the targeted tumor or tumor-supporting cells, to sustain anticancer immunity, and/or to inhibit protumor pathways. Therapeutic mAbs can be sorted into three main categories, namely naked, conjugated and bispecific antibodies.
[i] Naked antibodies. Some naked mAbs act by promoting antibody-dependent cellular phagocytosis (ADCP), antibody-dependent cell-mediated cytotoxicity (ADCC), or complement-dependent cytotoxicity (CDC).Citation96–98 For instance, rituximab which binds to CD20 mediates the killing of B lymphoma cells through such mechanisms.Citation99 Other naked mAbs contribute to cancer cell elimination by exerting either an agonistic or an antagonistic activity on the targeted receptor. For instance, binding of agonistic anti-CD40 to CD40 at the surface of antigen-presenting cells triggers a co-stimulatory signal which promotes maturation of dendritic cells and supports the priming of tumor-specific T cells.Citation100–102 By contrast, binding of antagonistic anti-PD1 to PD1 at the surface of T lymphocytes blocks its interaction with the co-inhibitory immune checkpoint PDL1 at the tumor cell membrane, and thus allows sustained effector T cell activity.Citation17,Citation103 Each ICI aims to interfere with signals associated with cancer immune evasion, and prevent or revert inactivation/exhaustion of T cells responsible for antitumor immunity.Citation17 Yet, in the case of ipilimumab, binding to its immunoinhibitory target CTLA4 at the surface of regulatory CD4+ T lymphocytes (Tregs), which are abundant in the tumor microenvironment (TME), can also trigger Tregs depletion through ADCC, and thus alleviate cancer-associated immunosuppression.Citation33,Citation104,Citation105
Clinically approved therapeutic antibodies, including ICIs, are administered systemically and at a high dose that is expected to saturate their target receptors. However, this goal is hardly achieved in the practice following systemic delivery.Citation106 Moreover, intravenous infusion of ICIs like anti-CTLA4 can trigger dose-dependent (sometimes fatal) immune-related toxic effects. Collectively, these observations are arguing in favor of a local administration. As an illustration, an eight-time reduced dose of anti-CTLA4 injected i.t. demonstrated similar antitumor efficacy as systemic injection in mice, but with a leaked dose in the serum 1000 times lower.Citation107 Along this line, the toxicity of a CD40 agonistic antibody was abolished upon i.t. injection.Citation108 Furthermore, an agonistic antibody binding to glucocorticoid-induced TNF receptor-related protein (GITR, also known as TNFRSF18) showed a superior recruitment and activation of T cells in the TME when injected locally rather than intraperitoneally or i.v. in mice.Citation108,Citation109 Altogether, in addition to efficiently target T cells infiltrating the malignant lesions, i.t. injection of therapeutic mAbs may reduce autoimmune and inflammatory side effects, circumvent the unknowns surrounding tumor tissue penetration, and ease the combination of antibodies. Such combinations have been evaluated with success in preclinical studies involving anti-CTLA4 plus anti-OX40, or anti-PD1 plus anti-CD137.Citation91,Citation110–112 Additionally, some teams are designing a formulation allowing slow i.t. release of mAbs for prolonged and improved efficacy.Citation113
Along with these preclinical incentives, numerous clinical trials have been launched these recent years to evaluate the therapeutic index of mAbs when administered locally (). For instance, the Phase I trial NCT04336098 will assess SRF617, an antibody which neutralizes the ecto-nucleotidase CD39 (a catalyzer of the degradation of ATP into immunosuppressive adenosine).Citation114 SRF617 will be administered locally in patients with advanced solid tumors, either alone or in combination with i.v. infusions of either gemcitabine plus albumin-bound paclitaxel, or pembrolizumab. Other antagonistic antibodies which target PD1, PDL1, or CTLA4 are also being tested following i.t. delivery, either separately or combined. NCT03889912 will characterize the safety and tolerability of weekly i.t. injections of cemiplimab for 12 weeks prior to scheduled surgery in patients with recurrent cutaneous squamous cell carcinoma (CSCC). NCT03316274 will assess i.t. administration of nivolumab in participants with Kaposi sarcoma. NCT04090775 will evaluate the efficacy and adverse events related to cryosurgery combined with i.t immunotherapy with nivolumab + ipilimumab, together with low-dose cyclophosphamide (injectable and oral) in metastatic prostatic adenocarcinoma. In the Phase II/III trial NCT03755739, trans-artery/i.t. infusion of pembrolizumab and/or ipilimumab will be tested for the treatment of advanced solid tumors. Conversely, several antibodies mediating an agonistic effect on co-stimulatory T lymphocyte receptors have shown promising results in preclinical or clinical settings and are currently investigated as i.t. immunotherapy in clinical trials. NCT03792724, a Phase I–II study, will assess i.t. urelumab (an agonistic antibody binding to CD137, also known as TNFRSF9 or 4–1BB) combined with systemic nivolumab in patients with advanced solid neoplasms. The trial will recruit 32 participants to measure the incidence of adverse events, determine the recommended dose, and calculate the response rate. NCT03892525 was designed to test i.t. selicrelumab (anti-CD40) in combination with i.v. atezolizumab (anti-PDL1) in patients with refractory or relapsed B cell lymphoma. However, the study was terminated because drug development stopped. NCT03818542 aimed to evaluate ABBV-927 (anti-CD40) as first-line monotherapy in subjects with locally advanced head and neck squamous cell carcinoma (HNSCC) eligible for surgical resection. Nevertheless, the trial was terminated for strategic considerations. NCT04059588 will evaluate the safety and tolerability of an Fc-engineered anti-CD40 mAb (2141-V11), administered intratumorally for the treatment of patients with metastatic skin lesions. At last, NCT03432741 aimed to determine the feasibility of intralesional microinjections of a broad range of cancer immunotherapies (daratumumab, nivolumab, obinutuzumab, pembrolizumab, rituximab, and trastuzumab) and chemotherapies (belinostat, carfilzomib, gemcitabine, and romidepsin) in patients with lymphoma, or recurrent/resistant stage IV breast cancer. Unfortunately, this pilot Phase I trial is currently suspended for funding reasons ().
[ii] Conjugated antibodies. Immunoconjugates are most frequently constituted of a tumor antigen-targeting antibody covalently paired with a cytotoxic compound. The latter can be a radioactive particle (e.g. isotype yttrium 90 for ibritumomab tiuxetan),Citation115,Citation116 a toxin (e.g. Pseudomonas exotoxin for moxetumomab pasdotox),Citation117 or a chemotherapeutic agent such as a topoisomerase inhibitor (e.g. SN-38 for sacituzumab govitecan) or a microtubule inhibitor (e.g. auristatin for brentuxumab vedotin, mertansine for trastuzumab emtansine)Citation118 ().Citation119–122 By binding to cancer cells, conjugated antibodies concentrate the cytotoxicants into the tumor bed.Citation123 In addition to triggering cancer cell death, they promote the release of tumor antigens and danger signals which may elicit an anticancer immune response.Citation124 For instance, LMB-100 is a humanized anti-mesothelin antibody that is fused with a truncated Pseudomonas exotoxin A.Citation125 The Phase I trial NCT04840615 will evaluate the safety and determine the recommended Phase 2 dose (RP2D) of i.t. injections of LMB-100 combined with i.v. ipilimumab in patients with pleural or peritoneal mesothelioma. In the same line, NCT04547777 is a dose-escalation study aiming to determine the maximum tolerated dose (MTD) of a single i.t. infusion of 2141-V11 (anti-CD40) in combination with the immunotoxin D2C7, delivered i.t. at a fixed dose, in patients with recurrent malignant glioma (). D2C7 is a genetically engineered form of the Pseudomonas exotoxin conjugated with a single-chain variable fragment (scFv) harboring a high binding affinity for the epidermal growth factor receptor (EGFR) and its active mutant EGFRvIII; both being overexpressed on glioblastoma cells.
Yet, some immunoconjugates are non-cytotoxic per se but promote antitumor immunity. For instance, the Phase I trial NCT04502888 is recruiting patients with CSCC or HNSCC to test i.t. delivery of a fusion protein called SL-172154. The latter consists of human signal-regulatory protein alpha and CD40 ligand linked by a Fc fragment (SIRPα-Fc-CD40L).Citation126 SL-172154 shows dual functionality as it concomitantly antagonizes CD47 on malignant cells and agonizes CD40 on antigen-presenting cells. This dose-escalation study will inform the safety profile of SL-172154, and define the MTD and RP2D (). Immunocytokines represent another example of non-cytotoxic conjugated antibodies. They will be introduced in the next section of this review.
[iii] Bispecific antibodies. Through double antigen affinity, bispecific mAbs bring immune cells in close proximity to tumor cells. For instance, blinatumomab binds to CD19 on leukemia cells and can engage T cells through binding to their CD3 ().Citation127 To our knowledge, there were no clinical trials initiated these past 3 y involving intratumoral delivery of bispecific antibodies.
Cytokines
Cytokines are secreted messengers which orchestrate the dynamics and functions of leucocytes. Among them, IL12p70, IFN-I and IFNγ are produced by DCs upon activation and participate in the ignition of TH1 responses which are critical in cancer immunosurveillance.Citation128–130 Upon activation, effector TH1 and TC1 cells secrete IL2 and IFNγ. Not only these cytokines contribute to sustain the immune response (positive feedback loop), but IFNs also harbor direct cytostatic and pro-apoptotic effects on cancer entities which participate to the overall antineoplastic activity.Citation131,Citation132 Thus, even though cytokine injection alone is poorly efficient, it enhances adjuvanticity. Aldesleukin,Citation133 an analog of IL2, the first cytokine to reach the market, showed promising results when injected locally.Citation76 It is currently the subject of a Phase III clinical trial (NCT03233828) assessing the benefit of two i.t. injections of IL2 (as compared to saline in the control group) in suspected or in situ melanoma. NCT03982004 evaluated the safety and side effects of intralesional granulocyte-macrophage colony-stimulating factor (GM-CSF)Citation134 in participants with breast cancer with cutaneous metastases. The cytokine was combined with topical imiquimod, i.v. pembrolizumab, and epicutaneous cryoimmunotherapy. However, the trial was terminated by decision of the sponsor. Several analogs of IFNα are also tested for their capacity to activate DCs and NK cells and to slow down the proliferation of cancer cells. They have successfully treated cystic craniopharyngioma in a preliminary clinical study.Citation135 However, cytokines regulate many biological pathways and their i.v. administration provokes important off-target adverse effects.Citation136,Citation137 For this reason, IL12 immunotherapies have not yet entered Phase III clinical trials due to toxicity.Citation138,Citation139 Their local administration as such did not notably increase their adjuvanticity and/or reduce toxicity due to a rapid leakage in the systemic circulation.Citation140 Therefore, to prolong intratumoral retention, the team of Wittrup proposed a formulation in which the cytokine is anchored to a collagen-binding protein, which was successful in mouse models.Citation138,Citation141 To restrain their expression to targeted tissues, genes encoding cytokines can be inserted into locally injected DNA plasmids, mRNA or viruses (see next sections of this review) allowing cytokines to be recombined in situ. This promising strategy is the object of various preclinical and clinical studies (). For instance, i.t. injection of DCs overexpressing IL12 stimulated antitumor immunity and proved efficacy in mouse models.Citation142,Citation143 Similarly, a Phase 1 trial (NCT03946800) is testing a lipid-encapsulated mRNA encoding IL12 (referred to as MED1191). It will be delivered intratumorally in combination with i.v. durvalumab (anti-PDL1) to 87 subjects with advanced solid tumors. The escalation arm will determine the MTD while the expansion arm will measure the overall response rate (ORR). NCT03871348 evaluates i.t. administration of SAR441000, a mixture of four mRNAs encoding IL12 single chain, IFNα2b, GM-CSF, and IL15sushi,Citation144 alone or combined with i.v. cemiplimab in advanced solid tumors in a dose-escalation and dose-expansion study.Citation145 Three Phase II trials study i.t. electroporation of tavokinogene telseplasmid (Tavo-EP), a DNA plasmid encoding the human IL12, together with PD1 blockade. In NCT04526730, tavo-EP will be tested as a neoadjuvant treatment combined with systemic infusion of nivolumab in participants with operable locally advanced melanoma. NCT03567720 will combine i.t. tavo-EP with i.v. pembrolizumab alone or in addition of i.v. nab-paclitaxel in triple negative breast cancer (TNBC) patients with cutaneous/subcutaneous neoplasms. At last, NCT03823131 will determine whether i.t. tavo-EP combined with i.v. pembrolizumab, together or not with epacadostat (inhibitor of indoleamine 2,3-dioxygenase-1 [IDO1]), enhances the best ORR in HNSCC as compared to historical score for pembrolizumab monotherapy.
In order to enhance their bioavailability and improve their tissue targeting, cytokines can be fused with immunoglobulins, thus generating so-called immunocytokines.Citation76,Citation122 A Phase I/II clinical trial (NCT03958383) will evaluate i.t. administration of the immunocytokine hu14.18-IL2, either alone or combined with radiation therapy ± systemic immune checkpoint blockade (nivolumab ± ipilimumab) in patients with advanced or surgically incurable melanoma. On one hand, the hu14.18 moiety binds to the GD2 antigen expressed at the surface of several tumor histotypes (e.g. melanoma and neuroblastoma) while its Fc component mediates ADCC. On the other hand, the IL2 moiety locally stimulates NK and T cell antitumor immune responses.Citation146 Of note, hu14.18-IL2 has been previously tested clinically (NCT00590824) in patients with resectable recurrent advanced melanoma. This study demonstrated prolonged tumor-free survival following surgery plus 3 courses of hu14.18-IL2.Citation147,Citation148 NCT04362722, a Phase II trial, investigates the therapeutic potential of single or multiple i.t. injection(s) of a cocktail of two immunocytokines, L19IL2 and L19TNF, in patients with basal cell carcinoma or CSCC. These agents consist of a human scFv called L19 which is directed against the extra-domain B of fibronectin (EDB-FN) and fused to either IL2 or TNF. EDB-FN being upregulated in tumor area experiencing neoangiogenesis, L19 contributes to concentrate IL2 in the malignant bed.Citation149 L19IL2/L19TNF will be administered once weekly for up to 4 weeks into all injectable lesions. Of note, this combination showed promising results in a Phase II clinical trial in patients with advanced metastatic melanoma who were not candidates to surgery ().Citation148
PRR agonists
DCs sense the presence of pathogens by means of their PRRs, such as TLRs located at the plasma membrane or in endosomes, as well as some cytosolic adapters like STING1,Citation65 melanoma differentiation-associated protein 5 (MDA-5)Citation150 or retinoic acid-inducible gene I (RIG-I).Citation151–155 These receptors are also involved in the recognition of DAMPs released by stressed or dying cells.Citation156,Citation157 Therefore, over the past two decades, their potent immunostimulatory effects have inspired laboratories in the development of PRR agonists as antineoplastic agents in preclinical and clinical settings.Citation64
TLR3 agonists
Upon activation by endogenous (mammalian) as well as exogenous (viral) double-stranded ribonucleic acids (dsRNA) in endosomes, TLR3 triggers the secretion of pro-inflammatory cytokines, such as IFN-I, and contributes to DC cross-priming.Citation79,Citation158 The critical role of IFNs in the initiation of tumor antigen-specific immunity has encouraged the clinical investigation of TLR3 agonists as single agents or in combination with other therapeutics.Citation22 These agonists have reported immunostimulatory function as well as direct anti-proliferative and pro-apoptotic effects in malignant cells positive for TLR3 (e.g. head and neck carcinoma, lung squamous cell carcinoma and adenocarcinoma).Citation159 The latter property could extend their application to cancer patients with immunodeficiencies. The prototypical TLR3 agonist polyinosinic-polycytidylic acid (poly-IC) and its derivatives are being evaluated as intratumor interventions in advanced oncological indications.Citation160 For instance, poly-IC complexed with poly-L-lysine and carboxymethylcellulose (poly-ICLC/Hiltonol) is under investigation in B-cell lymphoma in association with radiotherapy and recombinant human FMS-like tyrosine kinase-3 ligand (Flt3L) (NCT01976585), and in mesothelioma in a neoadjuvant setting (NCT04525859) (). Already, i.t. poly-ICLC has proven well tolerated and safe in advanced cancer patients, as a standalone application as well as in combination therapies, with signs of immunostimulation and some clinical benefits witnessed.Citation161,Citation162 As another example, BO-112 is a formulation of polyI:C complexed with polyethylenimine. It promotes tumor cell apoptosis with ICD features.Citation78 In immunocompetent mice bearing ectopic colorectal (MC38, 4T1) and melanoma (B16-F10 tumors), i.t. delivery of BO-112 significantly controlled disease progression. This therapeutic effect appeared mediated by IFN-I and IFNγ.Citation78 Two Phase I trials involving i.t. BO-112 have been initiated these past 3 y. NCT04420975 is primarily evaluating the frequency and severity of adverse events and determining dose-limiting toxicities (DLT) of BO-112 when combined with i.v. nivolumab plus standard of care radiotherapy before surgical resection in patients with soft tissue sarcoma (). NCT04777708 will assess the clinical response to local delivery of BO-112 together with i.v. pembrolizumab in patients with hepatocellular carcinoma ().
TLR4 agonists
Despite the numerous TLR4 ligands that have been the subject of preclinical and clinical investigations, only two have been approved by the FDA for clinical use as cancer treatment: BCG and monophosphoryl lipid A (MPL).Citation163,Citation164 NCT03742804 aimed to test G100 as single agent in lymphoma but has been withdrawn because the study sponsor sold and the new owner did not support the study.
TLR7 agonists
TLR7 is an intracellular receptor expressed on endosomal membranes which recognizes nucleosides and nucleotides from intracellular pathogens.Citation165 Upon activation, TLR7 can induce an inflammatory response and the production of IFN-I, both beneficial for triggering antitumor immunity.Citation63,Citation166 In this line, imiquimod received FDA approval as topical standalone application (cream) to activate TLR7 in the environment of superficial basal cell carcinoma. The Phase I NCT03301896 consists of four dose-escalation and two dose-expansion parts testing the TLR7 agonist LHC165 given i.t. as monotherapy or in combination with i.v. PDR001 (anti-PD1) for the treatment of solid tumors (). Similarly, NCT04799054 will evaluate the TLR7/8 agonist TransCon as a single agent or combined with i.v. pembrolizumab in dose-escalation and dose-expansion scenarios for patients with advanced or metastatic solid tumors (). At last, NCT03435640 will assess i.t. delivery of the TLR7/8 agonist NKTR-262 in 3-week treatment cycles with systemic administration of bempegaldesleukin, together or not with nivolumab, in patients with locally advanced or metastatic solid tumors (). Of note, bempegaldesleukin consists of a pegylated IL2 which binds to the β subunit of the IL2 receptor, thus preferentially expanding CD8+ T cells over immunosuppressive regulatory CD4+ T cells.Citation167,Citation168
TLR8 agonists
Similarly to TLR7, detection of single-stranded (ss)RNA by TLR8 induces an inflammatory response and IFN-I secretion.Citation169 NCT03906526 studies the safety and tolerability profile of i.t. and s.c. delivery of the TLR8 agonist motolimod (VTX-2337) in combination with i.v. nivolumab in patients with HNSCC. Meanwhile, tumor immune modulation will be evaluated by quantitating CD8+ T cells infiltrating the neoplastic microenvironment before and after treatment ().
TRL9 agonists
TLR9 is predominantly located intracellularly in immune cells, such as antigen-presenting cells (e.g. conventional and plasmacytoid DCs, macrophages, B cells) or T lymphocytes.Citation170 The main ligands of TLR9 are bacterial unmethylated cytidine phosphate guanosine (CpG) oligodeoxynucleotides (ODNs). Stimulation of TLR9 triggers the production of pro-inflammatory cytokines (e.g. IFN-I, IL6, IL12, TNFα) which activates innate immune actors, including DC and NK cells. In turn, antigen cross-presentation by mature DCs primes the adaptive arm of the immune system which culminates in the destruction of microorganisms or cancer cells.Citation171–173 For the purpose of cancer treatment, unmethylated CpG ODNs have been synthesized to mimic the immunostimulatory activity of bacterial DNA on TLR9.Citation174 Intratumoral delivery of the TLR9 agonist cavrotolimod (AST-008) is being evaluated in a Phase Ib/II trial (NCT03684785) as a standalone or in combination with infusions of either cemiplimab or pembrolizumab in skin cancers and solid tumors with liver metastases (). CMP-001 is a CpG ODN packaged in virus-like particles. CMP-001 is being tested following i.t. delivery in combination with infusions of ICIs targeting CTLA4 (ipilimumab), PD1 (cemiplimab, nivolumab, or pembrolizumab) or PDL1 (atezolizumab), together or not with radiotherapy or surgery, in multiple oncological indications (). NCT03983668 will determine the DLT of i.t. CMP-001 in combination with i.v. pembrolizumab in patients with relapsed and refractory lymphomas. The efficacy of this combination treatment will be evaluated in Phase II trials in advanced operable melanoma (NCT04708418) and in HNSCC participants who have not been previously treated with PD1 blockers (NCT04633278). Four Phase II (or II/III) trials will evaluate i.v. nivolumab associated with intratumor CMP-001 in melanoma. NCT04698187 and NCT03618641 will measure the response rate to the latter combinatorial therapy as well as survival of subjects with refractory unresectable/metastatic melanoma or Stage III melanoma with clinically apparent lymph node involvement, respectively. NCT04401995 and NCT04695977 will compare nivolumab monotherapy to nivolumab plus i.t. CMP-001 in a neoadjuvant setting in patients with Stage III melanoma, or as a first line treatment in participants with unresectable or metastatic melanoma, respectively. NCT04916002 will determine the safety, tolerability and efficacy of i.t. CMP-001 in combination with cemiplimab in patients with advanced/metastatic CSCC, Merkel cell carcinoma (MCC), or TNBC. Three clinical trials will investigate CMP-001 in association with radiotherapy. NCT03507699 aims to test a combinatorial regimen of s.c. CMP-001, i.v. nivolumab, i.v. ipilimumab, and 21-gray (Gy) liver radiation therapy in patients with colorectal cancer (CRC) with liver metastases. NCT04807192 will assess preoperative stereotactic body radiation therapy (SBRT) alone or combined with s.c. followed by i.t. administrations of CMP-001 in patients with early stage TNBC. The completed Phase I study NCT03438318 has evaluated i) the safety and efficacy of i.t. CMP-001 together with atezolizumab, and ii) the benefit of radiation therapy to this combination. The synthetic TLR9 agonist (also known as IMO-2125) in evaluated in Phase I–III trials in co-treatment with infused immune checkpoint antagonists and agonists. More precisely, the Phase I trial NCT04196283 assesses i.t. tilsotolimod plus i.v. ABBV-368 (anti-OX40) in combination with i.v. nab-paclitaxel alone or combined with i.v. ABBV-181 (anti-PD1) in patients with recurrent/metastatic HNSCC. The Phase II study NCT03865082 intends to test the efficacy of i.t. tilsotolimod with ipilimumab and nivolumab in different solid tumors. The Phase III trial NCT03445533 will study the benefits of i.t. tilsotolimod and i.v. ipilimumab in advanced melanoma. Of note, a previous Phase I/II trial assessing i.t. tilsotolimod with systemic ipilimumab in patients with anti-PD1-resistant advanced melanoma showed a rapid induction of local IFNα gene signature, DC maturation and antigen presentation, as well as an expansion of specific T cells; all of which correlated with clinical response.Citation175 The synthetic CpG ODN SD-101 is being investigated together with radiotherapy in various oncological indications. NCT03007732 (Phase II) evaluates i.t. SD-101 with oral prednisone (corticosteroids) and abiraterone acetate (androgen synthesis inhibitor), i.m. leuprolide acetate (gonadotropin-releasing hormone receptor [GnRHR] agonist), SBRT, and i.v. pembrolizumab in patients with newly diagnosed hormone-naive oligometastatic prostate cancer. NCT03322384 (Phase I/II) assesses the efficacy of five intralesional injections of SD101, radiotherapy and oral epacadostat for advanced/refractory solid tumors and lymphoma. NCT04050085 studies the side effects of i.t. SD-101 when delivered together with i.v. nivolumab and radiation therapy in patients with chemotherapy-refractory and metastatic pancreatic cancer. At last, NCT04612530 will evaluate the safety and efficacy of irreversible electroporation (IRE) + nivolumab + i.t. CpG ODN in pancreatic cancer patients, as compared to IRE + nivolumab, and nivolumab monotherapy. Of note, IRE is a recent technique relying on electrical pulses which allows local ablation of malignant lesions. Its cytoreductive propensity is accompanied with antigen release and stimulation of a T cell immune response, thus encouraging its combination with immunotherapy.Citation176
STING agonists
In the tumoral context, upon detection of accumulated mitochondria- or nucleus-derived dsDNA leaking in the cytosol of cells treated by chemotherapy or radiotherapy, STING induces the production of IFN-I which are critical for the initiation of antitumor immune responses. Therefore, the pharmaceutical industry has generated direct STING activators for oncological indications tested either alone or with various chemotherapeutic and immunotherapeutic combinatorial regimens.Citation67,Citation177,Citation178 In this dynamic, several Phase I–II trials have been recently initiated to investigate i.t. delivery of the STING agonists ADU-S100, BI 1387446, E7766, IMSA101, MK-1454, and SYNB1891 (). The Phase I trials NCT04144140 aims to assess safety, tolerability, and preliminary clinical activity of i.t. E7766 as a stand-alone agent in patients with advanced solid tumors or lymphomas. NCT04167137 will determine single-agent MTD of i.t. SYNB1891 as monotherapy, and the RP2D in combination with atezolizumab, in subjects diagnosed with advanced/metastatic solid tumors and lymphoma. The Phase II NCT03937141 evaluates the ORR to i.t. ADU-S100 combined with i.v. pembrolizumab as first-line treatment of adults with PDL1-positive recurrent or metastatic HNSCC. Similarly, NCT04220866 studies i.t. MK-1454 in combination with i.v. pembrolizumab, compared to pembrolizumab alone, as a first-intention treatment of subjects with metastatic, or unresectable, recurrent HNSCC. NCT04020185 is a dose-escalation (Phase I) and dose-expansion (Phase IIA) study of participants receiving i.t. IMSA101 alone or combined with ICI. The Phase I NCT04147234 will assess i.t. BI 1387446 in adults with advanced cancer that failed previous treatment.Citation179 In addition, some participants will receive i.v. infusions of ezabenlimab (formerly BI 754091), a humanized IgG4 anti-PD1 mAb, every 3 weeks.
RIG-I agonists
RIG-I-like receptors are key sensors of virus infection as well as host-derived RNA. Their detection induces the transcription of immune genes including IFN-I. As a consequence, synthetic RIG-I agonists have been synthesized and evaluated in preclinical and clinical studies as vaccine adjuvants, or potentiators of anticancer immunotherapies.Citation151,Citation180–182 For instance, the completed Phase I NCT03739138 aimed to assess safety, tolerability, pharmacokinetics, and preliminary antitumor activity of i.t. injections of MK-4621 delivered via a nucleic acid delivery system, JetPEI™, as monotherapy, and in combination with pembrolizumab in patients with advanced/metastatic solid tumors with no available results yet ().
Nonpathogenic bacteria
Since the early approval of BCG in bladder cancer, additional weakened strains of bacteria are applying to join the armamentarium of cancer treatments. By stimulating multiple PRRs, such as TLR2 and TLR4, and by providing xenoantigens, bacteria attract and activate immune sentinels, and can stimulate antitumor immune activity with reduced toxicity.Citation183–185 In particular, anaerobic strains, which preferentially replicate within tumors, are the objects of several clinical trials. For instance, Clostridium novyi-NTCitation186–188 is being tested i.t. in combination with i.v. pembrolizumab and oral doxycycline in subjects with advanced solid malignancies (NCT03435952) (). While patient enrollment continues, intermediary results from nine patients demonstrated encouraging signals of antineoplastic activity and a manageable toxicity profile.Citation189
Biopolymers
On top of antibodies, cytokines, and PRR agonists, some biopolymers can be endowed with adjuvant properties. Two of them, copaxone (glatiramer acetate) and IP-001 (N-dihydrogalactochitosan), are evaluated clinically as intratumoral cancer immunotherapy (). As such, copaxone is being administered prior to standard of care surgery in patients with percutaneously accessible tumors (NCT03982212). IP-001 is evaluated in a Phase I/II trial following thermal ablation in advanced solid tumors (NCT03993678).
ICD-inducing synthetic agents
In 2005, it was shown that anthracycline-based chemotherapy triggers an immunogenic, rather than tolerogenic, cancer cell apoptosis. Dubbed as immunogenic cell death or ICD, this phenomenon is characterized by the exposure/release of DAMPs along with the spread of antigens that are captured by immature DCs, thus promoting an adaptive immune response.Citation15,Citation190,Citation191 Since this first report on ICD, multiple cancer treatment modalities have been reported to be endowed with such propensity.Citation192,Citation193 They include additional chemotherapeutics (e.g. cyclophosphamide and oxaliplatin), certain physical cues (e.g. radiotherapy and photodynamic therapy), oncolytic viruses (e.g. T-VEC), and some lytic peptides (e.g. LTX-315).Citation16,Citation69,Citation194–197An updated list of all confirmed ICD-inducing agents can be found in the following review:Citation16 Candidates for ICD induction are also indexed such as the platinum salts cisplatin and carboplatin, gemcitabine, pemetrexed, vinca-alkaloids, or taxanes, whose cell death can be accompanied by the release of ICD-related DAMPs, at least in certain cell lines.Citation16 Of note, with the exception of the oncolytic virus T-VEC, ICD-inducing interventions were not reported in because they were not approved for their immune stimulating potential and are thus not commonly classified as immunotherapeutics.
Interestingly, two trials are evaluating i.t. cisplatin, either as a single agent in non-small-cell lung carcinoma (NSCLC) (NCT04809103), or in a formulation referred to as INT230-6 (in which it is combined with vinblastineCitation198 and the excipient salcaprozate sodiumCitation199) in breast cancer (NCT04781725) (). NCT04701645 is a pilot study that will assess the feasibility of implanting in selected tumor deposits some innovative microdevices which diffuse up to 20 drugs locally. Patients with resectable lesions of ovarian, fallopian tube, and peritoneal cancers will be recruited to this purpose. Clinically relevant drugs delivered (alone or in combination) will include some confirmed or potential ICD inducers: carboplatin, cisplatin, cyclophosphamide, doxorubicin, gemcitabine, paclitaxel, pemetrexed, and/or vinorelbine. Appearance of adverse events and response to treatments will be measured ().
Intralesional administration of ICD-inducing peptides like CyPep-1 and LTX-315 has proven antitumor activity in preclinical studies.Citation194,Citation200–202 These data have motivated their translation into the clinic with two Phase II trials recently engaged. First, NCT04796194 aims to assess the ORR of intratumoral LTX-315, a peptide derived from human lactoferrin, in combination with systemic PD1 blockade in patients with percutaneously accessible advanced solid tumors. Second, NCT04260529 is a dose-escalation study that will assess the safety and tolerability of the synthetic oncolytic peptide CyPep-1 and determine its RP2D in advanced solid malignancies ().
Oncolytic virotherapy
OVs selectively infect and kill tumor cells in an immunogenic fashion. Indeed, viral oncolysis releases in the extracellular space both tumor and viral antigens together with danger signals, thus triggering the recruitment of DCs. Numerous OVs are administered locally and have the advantage to prime a polyclonal immune response without requiring tumor antigen identification and administration as a transgene, or as a tumor antigen provided either naked or loaded in DCs.Citation68,Citation203,Citation204 A myriad of OVs are genetically engineered to express immunomodulatory proteins (e.g. cytokines) in order to boost the activation of the immune system.Citation70,Citation205 For more information on oncolytic viruses, a series of Trial Watch dedicated to the topic has been published.Citation68,Citation206,Citation207
Herpesviruses
Some oncolytic viruses have been armed with a transgene expressing GM-CSF in order to enhance antitumor immune responses.Citation70,Citation208–210 This strategy has been applied to the type 1 herpes simplex virus (HSV) T-VEC approved for intratumoral virotherapy of melanoma ().Citation70,Citation211,Citation212 T-VEC is investigated as a single agent in recent trials such as NCT03458117 in locally advanced non-melanoma skin cancer, NCT03921073 in cutaneous angiosarcoma, and NCT04065152 in Kaposi sarcoma. T-VEC is given as a neoadjuvant, either as a standalone in high-risk early melanoma (NCT04427306), or combined with nivolumab in early metastatic melanoma (NCT04330430), or with i.v. pembrolizumab prior to complete lymph node dissection in resectable Stage 3 cutaneous melanoma (NCT03842943). NCT04068181 is a Phase II trial aiming at testing T-VEC in melanoma in combination with i.v. pembrolizumab following disease progression on prior PD1 blockade or as an adjuvant to PD1 therapy. Other Phase I trials combine T-VEC with i.v. atezolizumab: NCT03802604 in operable early breast cancer with residual disease after neoadjuvant chemotherapy, and NCT03256344 in triple negative breast cancer and CRC with liver metastases. NCT04599062 will test T-VEC combined with external beam radiation therapy in soft tissue sarcoma.
Moreover, other oncolytic HSVs equipped with a GM-CSF transgene are tested i.t. in clinical trials (): RP1Citation213 either as a single agent (NCT04349436) or with the anti-PD1 cemiplimab in advanced CSCC (NCT04050436), OrienX010 in melanoma patients in association with anti-PD1, either toripalimab (NCT04197882) or JSS001 (NCT04206358),Citation214 or else the HSV-2 OH2 in combination with i.v. LP002 (anti-PD1) in cancers of the digestive system (NCT04755543).Citation215
At last, additional strains of HSV-1 are clinically evaluated following i.t. delivery: i) G207 with radiation therapy in recurrent/progressive pediatric high-grade glioma (NCT04482933),Citation216,Citation217 ii) RP3 (which expresses proprietary stimulatory agents)Citation218 combined with anti-PD1 in advanced solid tumors (NCT04735978), iii) ONCR-177 (which encodes CCL4, IL-12, Flt3L, anti-CTLA4, anti-PD1) alone or combined with pembrolizumab in advanced/metastatic solid tumors (NCT04348916),Citation219 or iv) VG161 (which expresses IL12, IL15 with its receptor alpha unit, and a PDL1 blocking peptide)Citation218 as single agent in solid tumors including liver cancer (NCT04758897, NCT04806464).
Adenoviruses
With 15 ongoing studies initiated since 2018, adenovirus is the second most common OV in clinical trials and have been among the earliest OVs to enter clinical examination.Citation220–222 Two recent trials are evaluating adenoviruses as single agent (). The dose-escalating Phase I/II trial NCT04097002 will test ORCA-010 as first-line therapy against localized prostate adenocarcinoma.Citation221,Citation223 NCT04673942 is a dose-escalation study of AdAPT-001, expressing TGFβ, in subjects with refractory solid tumors. Oncolytic adenoviruses are also combined with other treatments in the clinic ().Citation224 NCT04714983 evaluates DNX-2440 (expressing OX40L)Citation225 in patients with resectable multifocal liver metastasis scheduled for curative-intent liver resection surgery. NCT03225989 studies LOAd703 (encoding a trimerized membrane-bound extracellular CD40L and 4–1BBL)Citation226 in colorectal, biliary, ovarian, or pancreatic cancer together with gemcitabine immune-conditioning or standard of care chemotherapy. The Phase II trial NCT04685499 evaluates i.t. telomelysin with i.v. pembrolizumab in either recurrent inoperable or progressive head and neck squamous cell carcinoma (HNSCC).Citation227 NCT04391049 studies the side effects of i.t. telomelysin when given together with i.v. carboplatin, i.v. paclitaxel, and radiation therapy.Citation228 NCT03514836 aimed to evaluate the combination of the oncolytic adenovirus ONCOS-102 (armed with GM-CSF)Citation206 delivered i.t., together with s.c. administration of the DC-based prostate cancer vaccine stapuldencel T (also known as DCVac/PCa) and i.v. cyclophosphamide in patients with castration-resistant advanced metastatic prostate cancer. Unfortunately, this trial was terminated due to insufficient accrual. NCT03740256 is a Phase I trial studying the incidence of DLT in patients receiving i.t. CAdVEC in combination with adoptively transferred HER2-specific CAR-T cells in participants with HER2+ cancer.Citation66
Poxviruses
In 1796, Edward Jenner pioneered the concept of vaccines by inoculating attenuated strains of vaccinia virus to vaccinate humans against variola, the agent causing smallpox.Citation229 In 1840, it became the world’s first vaccine and soon the more extensively used for human immunization as well as the more effective public health intervention in human history.Citation230 Since the disease eradication in the late 1970s, even though national vaccination programs ended, continuous research on vaccinia virus has produced numerous strains with improved safety profiles, some of them with oncolytic activity.Citation231 Recently initiated trials are evaluating five oncolytic strains of vaccinia virus either as single agents or in combination with ICIs (). The Phase I NCT03954067 aims to evaluate the safety and tolerability of ASP9801 (which expresses IL7 and IL12) as a single agent and determine the RP2D in (sub)cutaneous and visceral tumors. The dose-escalation trial NCT04725331 evaluates repeated i.t. injections of BT-001, alone or combined with i.v. infusions of pembrolizumab, in skin cancers, NSCLC, sarcoma, or TNBC. Of note, BT-001 is equipped with transgenes encoding a Treg-depleting anti-CTLA4 and GM-CSF.Citation232 Similarly, NCT04787003 aims to define the MTD of i.t. OVV-01, and evaluate its efficacy with or without pembrolizumab or atezolizumab in patients with advanced malignancies. Likewise, NCT04301011 determines the RP2D of i.t Tbio-6517 (which encodes anti-CTLA-4 mAb, Flt3L and IL12), alone and combined to i.v. infusions of pembrolizumab in patients with solid tumors. Pexastimogene devacirepvec (Pexa-Vec, previously known as JX-594)Citation233 is based on the Wyeth strain of vaccinia virus. Pexa-Vec has been genetically inactivated for the gene encoding the viral thymidine kinase, and engineered to express the human GM-CSF and β-galactosidase. NCT03294083 will determine the safety and efficacy of i.t. versus i.v. Pexa-Vec combined with i.v. cemiplimab in patients with advanced renal cell carcinoma. Preliminary results supported that participants may benefit from i.v. Pexa-Vec + cemiplimab with an acceptable safety profile while further investigation is ongoing regarding i.t. Pexa-Vec + cemiplimab.Citation234 Finally, NCT04849260 will first determine the RP2D of Pexa-Vec combined with the anti-PDL1 antibody ZKAB001 in patients with local progression of failed first-line treatment or metastatic melanoma. In the Phase II of the trial, ORR and progression-free survival (PFS) will be estimated.
Paramyxoviruses
Attenuated measle viruses (MV) are studied in two clinical trials (). MV-s-NAP,Citation235 which encodes a secretory form of H. pylori neutrophil-activating protein (s-NAP), is evaluated as a single agent in invasive metastatic breast cancer (NCT04521764). Additionally, NCT04195373 aimed to test the safety and tolerability of TMV-018, an oncolytic measle virus encoding the prodrug converting enzyme “super cytosine deaminase”, in patients with gastrointestinal tumors. The trial was designed to evaluate TMV-018 either alone and in combination with either 5-fluorocytosine or an anti-PD1, or both. However, no subjects could be recruited leading to the assay withdrawal.
Rhabdoviruses
The Phase I trial NCT03865212 studies an oncolytic vesicular stomatitis virus engineered to express human IFNβ and the melanocyte lineage-specific antigen tyrosinase-related protein 1 (VSV-IFNbetaTYRP1) (). The latter transgene intends to induce an immune response specific to melanoma cells while the former contributes to further stimulate immune actors.Citation236–240 The MTD of VSV-IFNbetaTYRP1 will be determined following i.t. plus i.v. delivery in patients with advanced melanoma. By contrast, Voyager V1 (VV1) is a VSV expressing human IFNβ and the human sodium iodide symporter (NIS) for virus tracking by tomography.Citation241 The Phase II trial NCT04291105 will measure anti-tumor activity of VV1 given both i.t. and i.v. in combination with i.v. cemiplimab in melanoma (). In the Phase I NCT03618953, an oncolytic MG1 Maraba virus expressing the HPV E6 and E7 antigens (referred to as MG1-E6E7)Citation242 will be administered i.v. (Arm 1) as well as i.t. and i.v. (Arm 2) following i.m. injection of an adenovirus vaccine expressing the same antigens (Ad-E6E7) (). This heterologous prime-boost oncolytic vaccination will be followed by a systemic infusion of atezolizumab. Similarly, NCT03773744 was a dose-escalation trial of MG1-MAGEA3 given in a heterologous prime-boost vaccination with Ad-MAGEA3Citation243 in patients with previously treated metastatic melanoma or CSCC. Following Ad-MAGEA3 vaccine priming and pembrolizumab infusion, MG1-MAGEA3 vaccine booster should have been administered first i.v. then repeatedly by i.t. injections. Unfortunately, NCT03773744 had to be withdrawn due to insufficient drug supply ().
Picornaviruses
Two recent clinical trials aim to assess safety, tolerability, and efficacy of intratumoral viroimmunotherapy with the coxsackievirus A21, referred to as V937 (formerly CAVATAK®) (). It will be combined with systemic infusion of pembrolizumab in ICI-naïve participants with advanced/metastatic melanoma (NCT04152863) or in cohorts of patients with advanced TNBC, SCC, HCC, gastric cancer, or solid tumors with liver metastases (NCT04521621). Four clinical trials are evaluating PVSRIPO, a modified live attenuated non-neurovirulent poliovirus which ignites an antitumoral immunity following tumor cell oncolysis (). Its natural oncotropism relies on the poliovirus receptor PVR/CD155, an oncofetal antigen overexpressed on tumor cells and used for cell entry.Citation244,Citation245 Preliminary results from the Phase I trial NCT03712358 showed that i.t. administration of PVSRIPO was well tolerated and demonstrated promising antitumor activity in patients with advanced melanoma.Citation246 PVSRIPO will also be tested in combination with i.v. nivolumab in the same oncological indication in patients that failed responding to prior PD-1 blockade (NCT04125719, withdrawn with a resubmission planned). The Phase 2 single-arm trial NCT04479241 will evaluate the safety, tolerability, and initial efficacy of i.t. PVSRIPO followed by i.v pembrolizumab in patients with recurrent brain tumors.Citation247 At last, the early Phase I trial NCT03564782 is recruiting participants to study PVSRIPO bioactivity in the tumor bed after i.t. injection in women with invasive breast cancer scheduled for surgery.
Other virus-based immunotherapies
Non-oncolytic viruses are also applied for cancer immunotherapy as they are sufficient to render tumors more immunogenic by providing both DAMPs and foreign antigens. Moreover, they can be armed with transgenes which further stimulate antitumor pathways (e.g. cytokines and tumor antigens), or inactivate protumor signaling (e.g. tumor suppressors).Citation248,Citation249
Adenoviruses
Three studies evaluate the safety and tolerability of Ad-RTS-hIL12 in glioblastoma patients (). This adenoviral vector allows conditional expression of human IL12 inducible by administration of the small-molecule veledimex. Ad-RTS-hIL12 is administered once i.t. upon standard of care craniotomy and tumor resection. Patients are given oral veledimex prior to surgery and for the following 14 d (NCT03679754, Phase I). Of note, a previous Phase I trial (NCT02026271) showed that this veledimex-regulated transcriptional switch safely controls the dose of IL12 delivered and was well tolerated in glioma patients.Citation139 Ad-RTS-hIL12 plus veledimex is also tested in combination with infusions of anti-PD1: nivolumab (NCT03636477, Phase I) or cemiplimab-rwlc (NCT04006119, Phase II). Other replication-incompetent adenoviruses are considered for cancer immunotherapy together with ICIs (). NCT04013334 will test i.t. injections of the virus MTG201 in combination with 4 weekly i.v. infusions of nivolumab in patients with relapsed malignant pleural mesothelioma. MTG201 is equipped with a transgene encoding the tumor suppressor DKK3/REIC (Dickkopf-3/Reduced expression in immortalized cells) whose expression in cancer cells leads to apoptosis, while overexpression in stromal cells of the TME (e.g. fibroblasts) stimulates antitumor immunity.Citation250 In preclinical studies, a p53-armed adenoviral vector (Ad-p53), alone or combined with PD1 blockade, showed an enhanced control of local treated and distant untreated tumors compared with anti-PD1 alone, suggesting that Ad-p53 immunotherapy mediates abscopal effects and can reverse resistance to ICI.Citation249,Citation251,Citation252 These results encouraged the clinical evaluation of Ad-p53 in combination with PD1 blockade. Along this line, the Phase I–II investigation NCT02842125 aimed at evaluating the safety and efficacy of i.t. Ad-p53 in combination with i.v. nivolumab in patients with recurrent HNSCC. However, this trial was terminated as the cohort has been rolled into a parallel study. In the Phase II trial NCT03544723, i.t. Ad-p53 will be experimented in participants with recurrent or metastatic solid tumors together with i.v. infusions of physician’s choice of approved anti-PD1/anti-PDL1.
Poxvirus
A total of 22 patients with localized prostate cancer were scheduled to be enrolled in NCT04020094 to receive pre-operative i.t. injections of MVA-BN-Brachyury. This latter is a modified vaccinia Ankara-Bavarian Nordic expressing a MHC-I-restricted epitope of the transcription factor Brachyury and three co-stimulatory molecules: B7.1, ICAM-1 and LFA-3. MVA-BN-Brachyury was accompanied with both neoadjuvant and adjuvant combinations of atezolizumab (infusion) plus PROSTVAC (s.c.); a vaccinia virus-based vaccine encoding prostate-specific antigen (PSA).Citation253 The study aimed to assess the safety of this combination immunotherapy and quantify tumor-infiltrating CTLs.Citation254 However, it was stopped due to funding withdrawal ().
Arenaviruses
For treating human papilloma virus (HPV)-related cancers, HB-201, an attenuated lymphocytic choriomeningitis virus (LCMV) encoding an inactivated fusion protein constituted of the HPV-16 oncoproteins E6 and E7, is being tested i.t. in HNSCC and cervical cancers.Citation248 HB-201 will be injected once either as a standalone treatment or combined with chemoradiation in the Phase I trial NCT04630353. By contrast, HB-201 will be integrated in a prime-boost immunization setting, in which vaccine recalls are perpetuated with systemic infusions of either HB-201 or the Pichinde virus-based E6/E7 vaccine (HB-202) in the Phase I/II study NCT04180215 ().
Flavivirus
The Phase I NCT03990493 evaluates i.t. injection of the attenuated strain #45AZ5 of dengue virus-1 (referred to as PV-001-DV), combined with i.v. infusions of autologous monocyte-derived DCs pulsed with tumor lysate (PV-001-DC), in advanced melanoma ().Citation255
Orthomyxoviruses
Influenza vaccines will be investigated as local therapy of oncological indications in two recently registered trials ().Citation256 The explorative Phase II trial NCT04591379 will determine the safety and efficacy of i.t. influenza vaccine as an immune response-enhancing treatment before intended curative surgery in participants with CRC. NCT04697576 investigates influenza vaccine delivered first i.m. then i.t., either prior to surgery in patients with resectable melanoma, or concurrent with standard of care ipilimumab, nivolumab, or pembrolizumab in metastatic melanoma.
Therapeutic vaccines
Therapeutic cancer vaccines are designed to prime an adaptive immunity against neoplastic cells.Citation257,Citation258 They rely on providing an abundant source of tumor-specific antigens (TSAs) or tumor-associated antigens (TAAs) in order to favor their capture and (cross-) presentation to T cells by endogenous DCs. This strategy has the advantage to stimulate a cellular immune response that is highly specific to malignant entities. Cancer vaccines exist in different forms: i) cell lysates, ii) purified antigens, iii) recombinant DNA, RNA, or viruses encoding antigenic determinants, or iv) DCs presenting antigens.Citation259–260 In this dynamic, some efforts are being made for the design of bioinformatics algorithms predicting the binding of antigen epitopes to major histocompatibility complex molecules.Citation261 A proteogenomic approach combining mass spectrometry and RNA sequencing has also been developed for the identification of tumor antigens, including aberrantly expressed TSAs, which remained undetected with previous methods.Citation262,Citation263
Intralesional delivery of cancer vaccines is currently the object of several preclinicalCitation264,Citation265 as well as clinical investigations. In this line, two studies are evaluating the safety of intratumor Ifx-Hu2.0 applied for the care of skin cancers. Ifx-Hu2.0 is a DNA plasmid encoding the highly immunogenic Emm55 streptococcal antigen. It will be administered repeatedly in up to three lesions of cutaneous, subcutaneous or nodal melanoma (NCT03655756) or of MCC or SCC (NCT04160065) ().Citation266,Citation267 Recombinant viruses are also tested in patients for the local production of large amounts of tumor antigens, such as HB-201 and MG1-E6E7 in HPV-related cancers, or MVA-BN-Brachyury in prostate cancer (see previous sections of this review) (). Since the approval of sipuleucel-T,Citation268,Citation269 cancer vaccine candidates relying on DCs loaded with tumor antigens are expanding (e.g. the aforementioned DCVac/Pca and PV-001-DC). To present antigens and/or express immune cell-activating factors, autologous DCs are extracted from patients, then either genetically manipulated or co-cultured with a tumor lysate or antigens.Citation269,Citation270 Some DC-based vaccines are being evaluated clinically following i.t. delivery. First, the Phase I trial NCT03638765 will take advantage of an intraventricular catheter system (Ommaya)Citation271 to deliver activated unloaded autologous DCs (DCVax-Direct) to brain metastases of breast or lung primary tumors (). The main challenge with i.t. injection of DCs is to overcome the highly immunosuppressive intratumoral microenvironment which may impair their function. For this reason, some groups have genetically manipulated DCs to overproduce inflammatory cytokines or co-administered them with adjuvants. For instance, Ad-CCL21-DC is a cancer vaccine comprised of autologous DCs transduced ex vivo with an adenovirus containing the CCL21 gene, a chemokine which drives DC migration and harbors antineoplastic activities.Citation272 This latter approach has been reported to induce efficient anticancer immunity in mouse models of leukemia.Citation273 Ad-CCL21-DC is currently evaluated following i.t. delivery in combination with i.v. pembrolizumab for the treatment of NSCLC (NCT03546361) ().Citation274
Adoptive transfer of lymphocytes
Adoptive cell transfer consists in taking the patient’s own immune cells (e.g. T cells, NK cells, and DCs), expanding and eventually modifying them ex vivo, before reinjecting them to the patient where they will promote or elicit cytotoxic action on cancer cells.Citation95,Citation275 The low count of cells reaching the lesions after systemic injection may result in suboptimal efficacy. To circumvent this limitation, i.t. injection enables to reach higher concentration of effector cells in situ, and to raise the chance of overcoming immunosuppression. On top of the adoptive transfer of DCs previously evoked, other strategies consist in expanding ex vivo autologous NK cells or tumor-infiltrating T lymphocytes in order to deliver a high dose of cytotoxic cells into the lesion.Citation276,Citation277 Two clinical trials are using ex vivo expanded autologous NK cells as single agent in patients with high grade (NCT04254419) or recurrent glioma (NCT04489420) using an intraventricular catheter system ().Citation271 However, not all patients develop tumor-specific T cells. Chimeric antigen receptor T cell (CAR-T) therapy has been thought to overcome this burden. It consists of autologous T cells which are extracted from patients and genetically modified to harbor a chimeric receptor consisting of an scFv targeting a surface tumor antigen. Four CD19 CAR-T cell therapies, namely axicabtagene ciloleucel (YescartaTM), brexucabtagene autoleucel (TecartusTM), lisocabtagene maraleucel (BreyanziTM), and tisagenlecleucel (KymriahTM) are currently approved as a systemic intervention in leukemia/lymphoma-bearing patients.Citation278,Citation279 A fifth CAR-T cell targeting B cell maturation antigen (BCMA) also received FDA approval in multiple myeloma in March 2021 ().Citation280–283 The Phase I trial NCT03389230 is recruiting patients with recurrent or refractory Grade II–IV glioma to investigate i.t. delivery of memory-enriched T cells lentivirally transduced to express a human epidermal growth factor receptor 2 (HER2)-specific, hinge-optimized, 41BB-costimulatory chimeric receptor and a truncated CD19 (HER2(EQ)BBζ/CD19t+). The study will measure the incidence of severe adverse events and determine RP2D, describe persistence and expansion of the CAR-T cells, and estimate median OS. NCT03932565 evaluates a fourth generation CAR-T cells targeting Nectin4 and the fibroblast activation protein (FAP) which additionally express the pro-inflammatory cytokines IL7 and CCL19, or IL12 to overcome the immunosuppressive environment associated with the tumor ().Citation284 Of note, Nectin 4 is a protein which is highly expressed on the surface of various carcinomas and which plays a key role in cancer occurrence and metastasis. Similarly, FAP is highly expressed in tumor stroma.
Combinations of immunotherapeutic strategies
Since cancer immune response involves a coordinate action of diverse immune cells and pathways (), it seems highly relevant to combine different strategies acting at various levels of the cancer-immunity cycle to reach superior therapeutic efficacy.Citation11,Citation285–288 In this line, some clinical investigations are evaluating i.t. delivery of various immunomodulatory agents aiming at reshaping the tumor microenvironment in a way that support the promotion/reinstatement of cancer immunosurveillance ().
Autologous immune cells are infused locally together with immunostimulatory drugs in three recent trials. NCT04952272 will assess in advanced solid cancers the incidence of side effects and primary efficacy of local injection of CpG ODNs, together or not with CAR-T cells releasing anti-OX40. They will be administered consecutively to either microwave ablation or intratumor injection of beads eluting chemotherapeutic compounds that aim to induce the release of tumor-specific antigens. The Phase I trial NCT03707808 will combine i.t. injection of autologous CD1c+ myeloid DCs with i.t. ipilimumab plus avelumab along with systemic nivolumab for the treatment of solid tumors and metastases to soft tissue. This completed trial demonstrated that i.t. injection of autologous CD1c+ myeloid DCs with i.t. co-injection of ipilimumab and avelumab is safe and induced early signs of antitumoral activity in pre-treated patients.Citation289 Similarly, the Phase II clinical trial NCT04571632 will use SBRT along with i.v. pembrolizumab, with or without i.t. avelumab plus ipilimumab plus CD1c+/CD141+ myeloid DCsCitation290 in NSCLC.
NCT03739931 is a dose-escalation study of i.t. mRNA-2752 in patients with relapsed/refractory solid malignancies or lymphoma. mRNA-2752 consists of mRNAs encapsulated in lipid nanoparticles and which encode the human immune checkpoint OX40L, and the cytokines IL23 and IL36γ. This cocktail will be administered alone and in combination with durvalumab.Citation291 At all doses studied, the combinatorial treatment was tolerated and associated with tumor shrinkage. Analyses of tumor and plasma biomarkers suggested a sustained immunomodulatory effect that included an elevation of IFNγ, TNFα, and PDL1 levels. These data comfort the application of mRNA-2752 in association with anti-PDL1.Citation291 Another mixture of three mRNAs, referred to as TriMix, is studied i.t. in a Phase I trial prior to surgery or neoadjuvant chemotherapy in early-stage breast cancer patients (NCT03788083). TriMix encodes the immune checkpoints CD70 and CD40L, and a constitutively active TLR4 which promotes DC activation and support T cell priming.Citation93,Citation292
Other trials are testing i.t. administration of PRR agonists along with other types of immunotherapies. In NCT03928275, response to intralesional delivery of the BCG strain TICECitation293 and recombinant IL2 aldesleukin will be measured in participants with cutaneous metastatic melanoma. As another example, in order to expand the efficacy of the TLR4 agonist MPL, a stable emulsion of glucopyranosyl lipid A (GLA-SE) was developed. The Phase I NCT03982121 aimed to determine the MTD, RP2D, and toxicity profile of i.t. injection of GLA-SE and ipilimumab in combination with i.v. administration of nivolumab and the FOLFOX chemotherapy regimen (i.e. folinic acid, fluorouracil, and oxaliplatin) for the treatment of colorectal liver metastases. Unfortunately, NCT03982121 never began because of withdrawal of the industrial partner. Similarly, in the Phase I trial NCT04270864, ipilimumab and the TLR9 agonist tilsotolimod will be administered i.t. in combination with i.v. nivolumab in patients with advanced cancers. The Phase Ib/II trial NCT04387071 studies the side effects and best dose of the VLP-encapsulated CMP-001 administered i.t. along with the agonistic anti-OX40 mAb INCAGN01949 in participants with advanced pancreatic cancer and other cancers except melanoma. NCT03831295 evaluates i.t. plus i.v. BMS-986178 (anti-OX40) along with i.t. SD-101 in treating patients with solid malignancies that have metastasized. The Phase I trial NCT03410901 will assess DLT, ORR, and PFS of the same regimen of i.t. immunotherapies in combination with radiotherapy in patients with low-grade B-cell non-Hodgkin lymphomas.
Synergistic combinations may also allow to reduce the dose of drugs injected, which both reduce costs and potential adverse events. In this line, CIVO (i.e. Comparative In Vivo Oncology) is an arrayed microinjection technology implanted in a patient’s tumor that simultaneously and locally assess tumor responsiveness to microdoses of multiple drugs.Citation294,Citation295 Three early Phase I clinical trials are currently using this device. First, NCT04272333 studies tumor microenvironment changes following i.t. microdose injection of the TLR8 agonist motolimod as a single agent or concomitant with i.t. nivolumab in patients with HNSCC. Second, NCT04065555 aims to study the tumor microenvironment modulations induced by TAK-981, a SUMOylation inhibitor shown to activate IFN signaling,Citation296 as single agent or combined with i.t. cetuximab or avelumab in HNSCC. Finally, in the early Phase I NCT04541108, cancer patients will receive intratumoral microdoses of BMS-986299 (NLRP3 agonist), relatlimab (anti-LAG3) or ipilimumab, either as single agents or combined with i.t. nivolumab (experimental arm 1). CIVO will also be applied to deliver intralesional microdoses of the STING agonist TAK-676, carboplatin, 5-fluorouracil, or paclitaxel as single agents or in combination (experimental arm 2). In the third experimental arm of NCT04541108, local delivery of microdoses of pembrolizumab will be studied either alone or in association with either MK-0482 or MK-4830, which are antagonists of the inhibitory immune receptors immunoglobulin-like transcript (ILT)-3 and ILT-4, respectively. These treatments will be administered prior to scheduled surgical biopsy or tumor resection surgery. Outcome measures of NCT04541108 will consist in detecting cell death and immune cell biomarkers and determining the relationship between the drugs injected and the incidence of adverse events.
Concluding remarks
Intratumoral immunotherapy has an immediate impact on cancer cells. It locally reshapes the tumor microenvironment in a way that supports cancer immunosurveillance, allowing to reinvigorate tumor-specific immunity. Additionally, this delivery approach offers the advantage to reduce systemic leakage of the therapeutic agent and thus to prevent off-target toxicity. Furthermore, this administration route requires a lower dose to impede tumor progression which opens the gate for drug combinations. Additionally, several therapeutic associations have demonstrated superior efficacy such as ICIs combined to either PRR agonists or ICD-inducing chemotherapy or radiotherapy.Citation195,Citation297,Citation298 Such regimens seem particularly promising since the anticancer immune response involves the coordinated recruitment and actions of multiple immune effectors, which each incarnates a druggable target for accessing optimized efficacy.
However, intratumoral injection suffers from technical challenges. The targeted tumor must have a sufficient size (>1 cm) to ensure injectability with a needle, especially when the lesions are poorly accessible.Citation91 It is therefore mainly used for subcutaneous and mucosal lesions or superficial lymph nodes, as highlighted by the numerous clinical trials which are testing i.t. immunotherapy in melanoma. Conversely, it is technically more challenging to locally deliver immunotherapies to deeper tumors, as it requires imaging or endoscopic guidance, with associated burden such as repeated x-ray exposure and long procedure. Imaging with ultrasound and/or computed tomography enables to guide needle positioning, but also to monitor tumor size evolution and the effects of the treatment on the tumor microenvironment. For most intraperitoneal lesions and tumors of the central nervous system, imaging is not even sufficient. In this setting, i.t. injection requires surgery, which inherently limits the repeatability of injections along the time.Citation91
Different questions also remain to be addressed before implementing the technique on a wider scale. Is there a different response if the agent is injected in a metastasis rather than in the primary tumor? Should all metastases be treated to target a high diversity of tumor antigens and elicit broader polyclonal antitumor response? The dose of the agents to administer also remains to be determined; a simple conversion from the systemic dose cannot be applied due to high heterogeneity among the treatments regarding absorption, distribution, and drug interactions. Moreover, the repartition of the drug into the tumors is not uniform and some very dense tumors are difficult to inject. Depending on the pharmacokinetics of the agent, it may leak out in the blood stream and cause unexpected systemic adverse effects. Importantly, the local trauma induced by the needle may have a deleterious impact on the treatment. Finally, local anesthetic administration is required prior to intratumoral injection, and their effects on the tumor microenvironment as well as on the stability and efficacy of the immunotherapeutics is not well known to date.Citation91 Ongoing and future clinical investigations will have to address these points in order to define the recommendations for intratumoral immunotherapy over systemic treatment, and for optimizing the efficacy of such local monotherapies or combinatorial regimens.
Abbreviations
BCG, Bacillus Calmette–Guérin; CAR-T, chimeric antigen receptor T cell; CIVO, Comparative In Vivo Oncology; CpG, cytidine phosphate guanosine; CSCC, cutaneous squamous cell carcinoma; CTL, cytotoxic T lymphocyte; DAMP, damage-associated molecular pattern; DC, dendritic cell; FDA, food and drug administration; HNSCC, head and neck squamous cell carcinoma; ICD, immunogenic cell death; ICI, immune checkpoint inhibitor; i.t., intratumoral; i.v., intravenous; mAb, monoclonal antibody; NK, natural killer; ODN, oligodeoxynucleotide; OV, oncolytic virus; Pexa-Vec, pexastimogene devacirepvec; PRR, pathogen recognition receptor; RP2D, recommended Phase 2 dose; SBRT, stereotactic body radiation therapy; TH1, type 1 T helper cell lineage; TLR, Toll-like receptor; TSA, tumor-specific antigen; T-VEC, talimogene laherparepvec.
Acknowledgments
JP is supported by the Multi-Organism Institute (ITMO) Aviesan Cancer (National Alliance for Life Sciences and Health), SIRIC Cancer Research and Personalized Medicine (CARPEM), and Institut National du Cancer (INCa). The L.G. lab is supported by a Breakthrough Level 2 grant from the US DoD BRCP (#BC180476P1), by the 2019 Laura Ziskin Prize in Translational Research (#ZP-6177, PI: Formenti) from the Stand Up to Cancer (SU2C), by a Mantle Cell Lymphoma Research Initiative (MCL-RI, PI: Chen-Kiang) grant from the Leukemia and Lymphoma Society (LLS), by a startup grant from the Dept. of Radiation Oncology at Weill Cornell Medicine (New York, US), by a Rapid Response Grant from the Functional Genomics Initiative (New York, US), by industrial collaborations with Lytix Biopharma (Oslo, Norway) and Phosplatin (New York, US), and by donations from Phosplatin (New York, US), the Luke Heller TECPR2 Foundation (Boston, US), Sotio a.s. (Prague, Czech Republic), Noxopharm (Gordon, NWS, Australia) and Onxeo (Paris, France). GK is supported by the Ligue contre le Cancer (équipe labellisée); Agence National de la Recherche (ANR) – Projets blancs; ANR under the frame of E-Rare-2, the ERA-Net for Research on Rare Diseases; AMMICa US23/CNRS UMS3655; Association pour la recherche sur le cancer (ARC); Association “Le Cancer du Sein, Parlons-en!”; Cancéropôle Ile-de-France; Chancelerie des universités de Paris (Legs Poix), Fondation pour la Recherche Médicale (FRM); a donation by Elior; European Research Area Network on Cardiovascular Diseases (ERA-CVD, MINOTAUR); Gustave Roussy Odyssea, the European Union Horizon 2020 Project Oncobiome; Fondation Carrefour; High-end Foreign Expert Program in China (GDW20171100085), Institut National du Cancer (INCa); Inserm (HTE); Institut Universitaire de France; LeDucq Foundation; the LabEx Immuno-Oncology (ANR-18- IDEX-0001); the RHU Torino Lumière; the Seerave Foundation; the SIRIC Stratified Oncology Cell DNA Repair and Tumor Immune Elimination (SOCRATE); and the SIRIC Cancer Research and Personalized Medicine (CARPEM).
Disclosure statement
JGP is named as inventor on patents for cancer vaccination involving an oncolytic rhabdovirus. These patents have been licensed to Turnstone Biologics of which JGP is shareholder. GK is a cofounder of Samsara Therapeutics, everImmune and Therafast Bio. GK has been holding research contracts with Daiichi Sankyo, Eleor, Kaleido, Lytix Pharma, PharmaMar, Samsara, Sanofi, Sotio, Vascage and Vasculox/Tioma. GK is on the Board of Directors of the Bristol Myers Squibb Foundation France. GK is a scientific co-founder of everImmune, Samsara Therapeutics and Therafast Bio.L.G. has received research funding from Lytix Biopharma and Phosplatin, as well as consulting/advisory honoraria from Boehringer Ingelheim, AstraZeneca, OmniSEQ, Onxeo, The Longevity Labs, Inzen, and the Luke Heller TECPR2 Foundation. The other author(s) declare no conflicts of interest. As per standard operations at Oncoimmunology, LG has been excluded from the editorial evaluation of the present article.
Correction Statement
This article has been republished with minor changes. These changes do not impact the academic content of the article.
Additional information
Funding
References
- Coley WB. The treatment of inoperable sarcoma by bacterial toxins (the mixed toxins of the streptococcus erysipelas and the bacillus prodigiosus). Proc R Soc Med. 1910;3:1–31. doi:10.1177/003591571000301601.
- Kamat AM, Flaig TW, Grossman HB, Konety B, Lamm D, O’Donnell MA, Uchio E, Efstathiou JA, Taylor JA. Expert consensus document: consensus statement on best practice management regarding the use of intravesical immunotherapy with BCG for bladder cancer. Nat Rev Urol. 2015;12:225–235. doi:10.1038/nrurol.2015.58.
- Matzinger P. The danger model: a renewed sense of self. Science. 2002;296:301–305. doi:10.1126/science.1071059.
- Land WG, Messmer K. The danger theory in view of the injury hypothesis: 20 years later. Front Immunol. 2012;3:349. doi:10.3389/fimmu.2012.00349.
- Matzinger P. Tolerance, danger, and the extended family. Annu Rev Immunol. 1994;12:991–1045. doi:10.1146/annurev.iy.12.040194.005015.
- Balkwill F, Mantovani A. Inflammation and cancer: back to Virchow? Lancet. 2001;357:539–545. doi:10.1016/S0140-6736(00)04046-0.
- Karin M, Cao Y, Greten FR, Li ZW. NF-kappaB in cancer: from innocent bystander to major culprit. Nat Rev Cancer. 2002;2:301–310. doi:10.1038/nrc780.
- Shankaran V, Ikeda H, Bruce AT, White JM, Swanson PE, Old LJ, Schreiber RD. IFNgamma and lymphocytes prevent primary tumour development and shape tumour immunogenicity. Nature. 2001;410:1107–1111. doi:10.1038/35074122.
- Sprooten J, Garg AD. Type I interferons and endoplasmic reticulum stress in health and disease. Int Rev Cell Mol Biol. 2020;350:63–118.
- Sprooten J, Agostinis P, Garg AD. Type I interferons and dendritic cells in cancer immunotherapy. Int Rev Cell Mol Biol. 2019;348:217–262.
- Vitale I, Shema E, Loi S, Galluzzi L. Intratumoral heterogeneity in cancer progression and response to immunotherapy. Nat Med. 2021;27(2):212–224. doi:10.1038/s41591-021-01233-9.
- Fucikova J, Kline JP, Galluzzi L, Spisek R. Calreticulin arms NK cells against leukemia. Oncoimmunology. 2020;9:1671763. doi:10.1080/2162402X.2019.1671763.
- Rodriguez-Ruiz ME, Vitale I, Harrington KJ, Melero I, Galluzzi L. Immunological impact of cell death signaling driven by radiation on the tumor microenvironment. Nat Immunol. 2020;21:120–134. doi:10.1038/s41590-019-0561-4.
- Buque A, Rodriguez-Ruiz ME, Fucikova J, Galluzzi L. Apoptotic caspases cut down the immunogenicity of radiation. Oncoimmunology. 2019;8:e1655364. doi:10.1080/2162402X.2019.1655364.
- Casares N, Pequignot MO, Tesniere A, Ghiringhelli F, Roux S, Chaput N, Schmitt E, Hamai A, Hervas-Stubbs S, Obeid M, et al. Caspase-dependent immunogenicity of doxorubicin-induced tumor cell death. J Exp Med. 2005;202(12):1691–1701. doi:10.1084/jem.20050915.
- Galluzzi L, Humeau J, Buque A, Zitvogel L, Kroemer G. Immunostimulation with chemotherapy in the era of immune checkpoint inhibitors. Nat Rev Clin Oncol. 2020;17:725–741. doi:10.1038/s41571-020-0413-z.
- Waldman AD, Fritz JM, Lenardo MJ. A guide to cancer immunotherapy: from T cell basic science to clinical practice. Nat Rev Immunol. 2020;20:651–668.
- Lopez-Otin C, Kroemer G. Hallmarks of health. Cell. 2021;184:33–63. doi:10.1016/j.cell.2020.11.034.
- Coventry BJ. Therapeutic vaccination immunomodulation: forming the basis of all cancer immunotherapy. Ther Adv Vaccines Immunother. 2019;7:2515135519862234.
- Dermime S, Armstrong A, Hawkins RE, Stern PL. Cancer vaccines and immunotherapy. Br Med Bull. 2002;62:149–162. doi:10.1093/bmb/62.1.149.
- Hu HG, Li YM. Emerging adjuvants for cancer immunotherapy. Front Chem. 2020;8:601. doi:10.3389/fchem.2020.00601.
- Le Naour J, Galluzzi L, Zitvogel L, Kroemer G, Vacchelli E. Trial watch: TLR3 agonists in cancer therapy. Oncoimmunology. 2020;9(1):1771143. doi:10.1080/2162402X.2020.1771143.
- Weiner LM, Dhodapkar MV, Ferrone S. Monoclonal antibodies for cancer immunotherapy. Lancet. 2009;373:1033–1040. doi:10.1016/S0140-6736(09)60251-8.
- Han X, Vesely MD. Stimulating T cells against cancer with agonist immunostimulatory monoclonal antibodies. Int Rev Cell Mol Biol. 2019;342:1–25.
- Roy DG, Geoffroy K, Marguerie M, Khan ST, Martin NT, Kmiecik J, Bobbala D, Aitken AS, de Souza Ct, Stephenson KB, et al. Adjuvant oncolytic virotherapy for personalized anti-cancer vaccination. Nat Commun. 2021;12:2626. doi:10.1038/s41467-021-22929-z.
- Briukhovetska D, Dorr J, Endres S, Libby P, Dinarello CA, Kobold S. Interleukins in cancer: from biology to therapy. Nat Rev Cancer. 2021;21:481-499.
- Zhang Y, Zhang Z. The history and advances in cancer immunotherapy: understanding the characteristics of tumor-infiltrating immune cells and their therapeutic implications. Cell Mol Immunol. 2020;17:807–821.
- Petroni G, Buque A, Zitvogel L, Kroemer G, Galluzzi L. Immunomodulation by targeted anticancer agents. Cancer Cell. 2021;39:310–345.
- Robert C. A decade of immune-checkpoint inhibitors in cancer therapy. Nat Commun. 2020;11(1):3801. doi:10.1038/s41467-020-17670-y.
- Rakova J, Truxova I, Holicek P, Salek C, Hensler M, Kasikova L, Pasulka J, Holubova M, Kovar M, Lysak D, et al. TIM-3 levels correlate with enhanced NK cell cytotoxicity and improved clinical outcome in AML patients. Oncoimmunology. 2021;10(1):1889822. doi:10.1080/2162402X.2021.1889822.
- Lesokhin AM, Callahan MK, Postow MA, Wolchok JD. On being less tolerant: enhanced cancer immunosurveillance enabled by targeting checkpoints and agonists of T cell activation. Sci Transl Med. 2015;7:280sr1. doi:10.1126/scitranslmed.3010274.
- Vaddepally RK, Kharel P, Pandey R, Garje R, Chandra AB. Review of indications of FDA-approved immune checkpoint inhibitors per NCCN guidelines with the level of evidence. Cancers. 2020;12(3):738. doi:10.3390/cancers12030738.
- Pol J, Kroemer G. Anti-CTLA-4 immunotherapy: uncoupling toxicity and efficacy. Cell Res. 2018;28:501–502. doi:10.1038/s41422-018-0031-9.
- Mueller MM, Fusenig NE. Friends or foes - bipolar effects of the tumour stroma in cancer. Nat Rev Cancer. 2004;4:839–849. doi:10.1038/nrc1477.
- Berraondo P, Sanmamed MF, Ochoa MC, Etxeberria I, Aznar MA, Perez-Gracia JL, Rodríguez-Ruiz ME, Ponz-Sarvise M, Castañón E, Melero I, et al. Cytokines in clinical cancer immunotherapy. Br J Cancer. 2019;120:6–15. doi:10.1038/s41416-018-0328-y.
- Landskron G, De la Fuente M, Thuwajit P, Thuwajit C, Hermoso MA. Chronic inflammation and cytokines in the tumor microenvironment. J Immunol Res. 2014;2014:149185. doi:10.1155/2014/149185.
- Smyth MJ, Godfrey DI, Trapani JA. A fresh look at tumor immunosurveillance and immunotherapy. Nat Immunol. 2001;2:293–299. doi:10.1038/86297.
- Martinek J, Wu TC, Cadena D, Banchereau J, Palucka K. Interplay between dendritic cells and cancer cells. Int Rev Cell Mol Biol. 2019;348:179–215.
- Guerriero JL. Macrophages: their untold story in T cell activation and function. Int Rev Cell Mol Biol. 2019;342:73–93.
- Yamamoto T, Kimura T, Ueta E, Tatemoto Y, Osaki T. Characteristic cytokine generation patterns in cancer cells and infiltrating lymphocytes in oral squamous cell carcinomas and the influence of chemoradiation combined with immunotherapy on these patterns. Oncology. 2003;64:407–415. doi:10.1159/000070300.
- Gajewski TF, Schreiber H, Fu YX. Innate and adaptive immune cells in the tumor microenvironment. Nat Immunol. 2013;14:1014–1022. doi:10.1038/ni.2703.
- Kotsias F, Cebrian I, Alloatti A. Antigen processing and presentation. Int Rev Cell Mol Biol. 2019;348:69–121.
- Buque A, Bloy N, Petroni G, Kroemer G, Galluzzi L. NK cells beat T cells at early breast cancer control. Oncoimmunology. 2020;9:1806010. doi:10.1080/2162402X.2020.1806010.
- Buque A, Bloy N, Perez-Lanzon M, Iribarren K, Humeau J, Pol JG, Levesque S, Mondragon L, Yamazaki T, Sato A, et al. Immunoprophylactic and immunotherapeutic control of hormone receptor-positive breast cancer. Nat Commun. 2020;11:3819. doi:10.1038/s41467-020-17644-0.
- Wu Chuang A, Kepp O, Kroemer G, Bezu L. Endoplasmic reticulum stress in the cellular release of damage-associated molecular patterns. Int Rev Cell Mol Biol. 2020;350:1–28.
- Khodarev NN. Intracellular RNA sensing in mammalian cells: role in stress response and cancer therapies. Int Rev Cell Mol Biol. 2019;344:31–89.
- Audsley KM, McDonnell AM, Waithman J. Cross-presenting XCR1(+) dendritic cells as targets for cancer immunotherapy. Cells. 2020;9:565. doi:10.3390/cells9030565.
- Gardner A, Ruffell B. Dendritic cells and cancer immunity. Trends Immunol. 2016;37:855–865. doi:10.1016/j.it.2016.09.006.
- Schraml BU, Reis e Sousa C. Defining dendritic cells. Curr Opin Immunol. 2015;32:13–20. doi:10.1016/j.coi.2014.11.001.
- Balan S, Saxena M, Bhardwaj N. Dendritic cell subsets and locations. Int Rev Cell Mol Biol. 2019;348:1–68.
- Lee YS, Radford KJ. The role of dendritic cells in cancer. Int Rev Cell Mol Biol. 2019;348:123–178.
- Lhuillier C, Galluzzi L. Preface: dendritic cells: master regulators of innate and adaptive immunity. Int Rev Cell Mol Biol. 2019;349:xi–xvi.
- Tanchot C, Terme M, Pere H, Tran T, Benhamouda N, Strioga M, Banissi C, Galluzzi L, Kroemer G, Tartour E, et al. Tumor-infiltrating regulatory T cells: phenotype, role, mechanism of expansion in situ and clinical significance. Cancer Microenviron. 2013;6:147–157. doi:10.1007/s12307-012-0122-y.
- Rao S, Gharib K, Han A. Cancer immunosurveillance by T cells. Int Rev Cell Mol Biol. 2019;342:149–173.
- Mori S, Jewett A, Murakami-Mori K, Cavalcanti M, Bonavida B. The participation of the Fas-mediated cytotoxic pathway by natural killer cells is tumor-cell-dependent. Cancer Immunology, Immunotherapy: CII. 1997;44:282–290. doi:10.1007/s002620050384.
- Takeda K, Smyth MJ, Cretney E, Hayakawa Y, Yamaguchi N, Yagita H, Okumura K. Involvement of tumor necrosis factor-related apoptosis-inducing ligand in NK cell-mediated and IFN-gamma-dependent suppression of subcutaneous tumor growth. Cell Immunol. 2001;214:194–200. doi:10.1006/cimm.2001.1896.
- Falschlehner C, Schaefer U, Walczak H. Following TRAIL’s path in the immune system. Immunology. 2009;127:145–154. doi:10.1111/j.1365-2567.2009.03058.x.
- Spetz J, Presser AG, Sarosiek KA. T cells and regulated cell death: kill or be killed. Int Rev Cell Mol Biol. 2019;342:27–71.
- Schreiber RD, Old LJ, Smyth MJ. Cancer immunoediting: integrating immunity’s roles in cancer suppression and promotion. Science. 2011;331:1565–1570. doi:10.1126/science.1203486.
- Pages F, Mlecnik B, Marliot F, Bindea G, Ou F-S, Bifulco C, et al. International validation of the consensus Immunoscore for the classification of colon cancer: a prognostic and accuracy study. Lancet. 2018;391(10135):539–545. doi:10.1016/S0140-6736(18)30789-X.
- Fridman WH, Zitvogel L, Sautes-Fridman C, Kroemer G. The immune contexture in cancer prognosis and treatment. Nat Rev Clin Oncol. 2017;14:717–734. doi:10.1038/nrclinonc.2017.101.
- Hensler M, Kasikova L, Fiser K, Rakova J, Skapa P, Laco J, Lanickova T, Pecen L, Truxova I, Vosahlikova S, et al. M2-like macrophages dictate clinically relevant immunosuppression in metastatic ovarian cancer. Journal for Immunotherapy of Cancer. 2020;8. doi:10.1136/jitc-2020-000979
- Frega G, Wu Q, Le Naour J, Vacchelli E, Galluzzi L, Kroemer G, Kepp O. Trial watch: experimental TLR7/TLR8 agonists for oncological indications. Oncoimmunology. 2020;9:1796002. doi:10.1080/2162402X.2020.1796002.
- Smith M, Garcia-Martinez E, Pitter MR, Fucikova J, Spisek R, Zitvogel L, Kroemer G, Galluzzi L. Trial watch: toll-like receptor agonists in cancer immunotherapy. Oncoimmunology. 2018;7:e1526250. doi:10.1080/2162402X.2018.1526250.
- Barber GN. STING: infection, inflammation and cancer. Nat Rev Immunol. 2015;15:760–770. doi:10.1038/nri3921.
- McGrath K, Dotti G. Combining oncolytic viruses with chimeric antigen receptor T cell therapy. Hum Gene Ther. 2021;32(3–4):150–157. doi:10.1089/hum.2020.278.
- Le Naour J, Zitvogel L, Galluzzi L, Vacchelli E, Kroemer G. Trial watch: STING agonists in cancer therapy. Oncoimmunology. 2020;9:1777624. doi:10.1080/2162402X.2020.1777624.
- Pol JG, Levesque S, Workenhe ST, Gujar S, Le Boeuf F, Clements DR, Fahrner J-E, Fend L, Bell JC, Mossman KL, et al. Trial watch: oncolytic viro-immunotherapy of hematologic and solid tumors. Oncoimmunology. 2018;7:e1503032. doi:10.1080/2162402X.2018.1503032.
- Vanmeerbeek I, Sprooten J, De Ruysscher D, Tejpar S, Vandenberghe P, Fucikova J, Spisek R, Zitvogel L, Kroemer G, Galluzzi L, et al. Trial watch: chemotherapy-induced immunogenic cell death in immuno-oncology. Oncoimmunology. 2020;9(1):1703449. doi:10.1080/2162402X.2019.1703449.
- Pol JG, Workenhe ST, Konda P, Gujar S, Kroemer G. Cytokines in oncolytic virotherapy. Cytokine Growth Factor Rev. 2020;56:4–27.
- Buque A, Bloy N, Aranda F, Castoldi F, Eggermont A, Cremer I, Fridman WH, Fucikova J, Galon J, Marabelle A, et al. Trial watch: immunomodulatory monoclonal antibodies for oncological indications. Oncoimmunology. 2015;4:e1008814. doi:10.1080/2162402X.2015.1008814.
- Vanpouille-Box C, Lhuillier C, Bezu L, Aranda F, Yamazaki T, Kepp O, Fucikova J, Spisek R, Demaria S, Formenti SC, et al. Trial watch: immune checkpoint blockers for cancer therapy. Oncoimmunology. 2017;6:e1373237. doi:10.1080/2162402X.2017.1373237.
- Garg AD, Vara Perez M, Schaaf M, Agostinis P, Zitvogel L, Kroemer G, Galluzzi L. Trial watch: dendritic cell-based anticancer immunotherapy. Oncoimmunology. 2017;6:e1328341. doi:10.1080/2162402X.2017.1328341.
- Aranda F, Buque A, Bloy N, Castoldi F, Eggermont A, Cremer I, Fridman WH, Fucikova J, Galon J, Spisek R, et al. Trial watch: adoptive cell transfer for oncological indications. Oncoimmunology. 2015;4:e1046673. doi:10.1080/2162402X.2015.1046673.
- Vacchelli E, Aranda F, Bloy N, Buque A, Cremer I, Eggermont A, Fridman WH, Fucikova J, Galon J, Spisek R, et al. Trial watch-immunostimulation with cytokines in cancer therapy. Oncoimmunology. 2016;5:e1115942. doi:10.1080/2162402X.2015.1115942.
- Pol JG, Caudana P, Paillet J, Piaggio E, Kroemer G. Effects of interleukin-2 in immunostimulation and immunosuppression. J Exp Med. 2020;217. doi:10.1084/jem.20191247.
- Nixon NA, Blais N, Ernst S, Kollmannsberger C, Bebb G, Butler M, Smylie M, Verma S. Current landscape of immunotherapy in the treatment of solid tumours, with future opportunities and challenges. Curr Oncol. 2018;25:e373–e84. doi:10.3747/co.25.3840.
- Aznar MA, Planelles L, Perez-Olivares M, Molina C, Garasa S, Etxeberria I, Perez G, Rodriguez I, Bolaños E, Lopez-Casas P, et al. Immunotherapeutic effects of intratumoral nanoplexed poly I:C. Journal for Immunotherapy of Cancer. 2019;7(1):116. doi:10.1186/s40425-019-0568-2.
- Jelinek I, Leonard JN, Price GE, Brown KN, Meyer-Manlapat A, Goldsmith PK, Wang Y, Venzon D, Epstein SL, Segal DM, et al. TLR3-specific double-stranded RNA oligonucleotide adjuvants induce dendritic cell cross-presentation, CTL responses, and antiviral protection. Journal of Immunology. 2011;186:2422–2429. doi:10.4049/jimmunol.1002845.
- Takeda Y, Kataoka K, Yamagishi J, Ogawa S, Seya T, Matsumoto M. A TLR3-specific adjuvant relieves innate resistance to PD-L1 blockade without cytokine toxicity in tumor vaccine immunotherapy. Cell Rep. 2017;19:1874–1887. doi:10.1016/j.celrep.2017.05.015.
- Gujar S, Pol JG, Kroemer G. Heating it up: oncolytic viruses make tumors ‘hot’ and suitable for checkpoint blockade immunotherapies. Oncoimmunology. 2018;7:e1442169. doi:10.1080/2162402X.2018.1442169.
- Melero I, Castanon E, Alvarez M, Champiat S, Marabelle A. Intratumoural administration and tumour tissue targeting of cancer immunotherapies. Nat Rev Clin Oncol. 2021;18:558–576. doi:10.1038/s41571-021-00507-y.
- De Lombaerde E, De Wever O, De Geest BG. Delivery routes matter: safety and efficacy of intratumoral immunotherapy. Biochim Biophys Acta Rev Cancer. 2021;1875:188526. doi:10.1016/j.bbcan.2021.188526.
- Harrington KJ, Puzanov I, Hecht JR, Hodi FS, Szabo Z, Murugappan S, Kaufman HL. Clinical development of talimogene laherparepvec (T-VEC): a modified herpes simplex virus type-1-derived oncolytic immunotherapy. Expert Rev Anticancer Ther. 2015;15:1389–1403. doi:10.1586/14737140.2015.1115725.
- Vacchelli E, Eggermont A, Sautes-Fridman C, Galon J, Zitvogel L, Kroemer G, Galluzzi L. Trial watch: oncolytic viruses for cancer therapy. Oncoimmunology. 2013;2:e24612. doi:10.4161/onci.24612.
- Renner A, Burotto M, Rojas C. Immune checkpoint inhibitor dosing: can we go lower without compromising clinical efficacy? J Glob Oncol. 2019;5:1–5.
- Abuodeh Y, Venkat P, Kim S. Systematic review of case reports on the abscopal effect. Curr Probl Cancer. 2016;40:25–37. doi:10.1016/j.currproblcancer.2015.10.001.
- Yamazaki T, Kirchmair A, Sato A, Buque A, Rybstein M, Petroni G, Bloy N, Finotello F, Stafford L, Navarro Manzano E, et al. Mitochondrial DNA drives abscopal responses to radiation that are inhibited by autophagy. Nat Immunol. 2020;21(10):1160–1171. doi:10.1038/s41590-020-0751-0.
- Rodriguez-Ruiz ME, Buque A, Hensler M, Chen J, Bloy N, Petroni G, et al. Apoptotic caspases inhibit abscopal responses to radiation and identify a new prognostic biomarker for breast cancer patients. Oncoimmunology. 2019;8:e1655964. doi:10.1080/2162402X.2019.1655964.
- Yamazaki T, Galluzzi L. Mitochondrial control of innate immune signaling by irradiated cancer cells. Oncoimmunology. 2020;9(1):1797292. doi:10.1080/2162402X.2020.1797292.
- Marabelle A, Andtbacka R, Harrington K, Melero I, Leidner R, De Baere T, Robert C, Ascierto PA, Baurain JF, Imperiale M, et al. Starting the fight in the tumor: expert recommendations for the development of human intratumoral immunotherapy (HIT-IT). Annals of Oncology: Official Journal of the European Society for Medical Oncology. 2018;29:2163–2174. doi:10.1093/annonc/mdy423.
- Aznar MA, Tinari N, Rullan AJ, Sanchez-Paulete AR, Rodriguez-Ruiz ME, Melero I. Intratumoral delivery of immunotherapy-act locally, think globally. Journal of Immunology. 2017;198:31–39. doi:10.4049/jimmunol.1601145.
- Hong WX, Haebe S, Lee AS, Westphalen CB, Norton JA, Jiang W, Levy R. Intratumoral immunotherapy for early-stage solid tumors. Clinical Cancer Research: An Official Journal of the American Association for Cancer Research. 2020;26(13):3091–3099. doi:10.1158/1078-0432.CCR-19-3642.
- Champiat S, Tselikas L, Farhane S, Raoult T, Texier M, Lanoy E, et al. Intratumoral immunotherapy: from trial design to clinical practice. Clinical Cancer Research: An Official Journal of the American Association for Cancer Research. 2021;27:665–679. doi:10.1158/1078-0432.CCR-20-0473.
- Houot R, Schultz LM, Marabelle A, Kohrt H. T-cell–based immunotherapy: adoptive cell transfer and checkpoint inhibition. Cancer Immunol Res. 2015;3(10):1115–1122. doi:10.1158/2326-6066.CIR-15-0190.
- Hubert P, Amigorena S. Antibody-dependent cell cytotoxicity in monoclonal antibody-mediated tumor immunotherapy. Oncoimmunology. 2012;1:103–105. doi:10.4161/onci.1.1.17963.
- Mielke D, Bandawe G, Pollara J, Abrahams M-R, Nyanhete T, Moore PL, Thebus R, Yates NL, Kappes JC, Ochsenbauer C, et al. Antibody-Dependent Cellular Cytotoxicity (ADCC)-mediating antibodies constrain neutralizing antibody escape pathway. Front Immunol. 2019;10:2875. doi:10.3389/fimmu.2019.02875.
- Wang B, Yang C, Jin X, Du Q, Wu H, Dall’Acqua W, Mazor Y. Regulation of antibody-mediated complement-dependent cytotoxicity by modulating the intrinsic affinity and binding valency of IgG for target antigen. MAbs. 2020;12:1690959. doi:10.1080/19420862.2019.1690959.
- Weiner GJ. Rituximab: mechanism of action. Semin Hematol. 2010; 47:115–123. doi:10.1053/j.seminhematol.2010.01.011.
- Haase C, Michelsen BK, Jorgensen TN. CD40 is necessary for activation of naive T cells by a dendritic cell line in vivo but not in vitro. Scand J Immunol. 2004;59:237–245. doi:10.1111/j.0300-9475.2004.01390.x.
- Hernandez MG, Shen L, Rock KL. CD40-CD40 ligand interaction between dendritic cells and CD8+ T cells is needed to stimulate maximal T cell responses in the absence of CD4+ T cell help. Journal of Immunology. 2007;178:2844–2852. doi:10.4049/jimmunol.178.5.2844.
- Morrison AH, Diamond MS, Hay CA, Byrne KT, Vonderheide RH. Sufficiency of CD40 activation and immune checkpoint blockade for T cell priming and tumor immunity. Proc Natl Acad Sci U S A. 2020; 117:8022–8031. doi:10.1073/pnas.1918971117.
- Simon S, Labarriere N. PD-1 expression on tumor-specific T cells: friend or foe for immunotherapy? Oncoimmunology. 2017;7:e1364828. doi:10.1080/2162402X.2017.1364828.
- Laurent S, Queirolo P, Boero S, Salvi S, Piccioli P, Boccardo S, et al. The engagement of CTLA-4 on primary melanoma cell lines induces antibody-dependent cellular cytotoxicity and TNF-alpha production. J Transl Med. 2013;11:108. doi:10.1186/1479-5876-11-108.
- Romano E, Kusio-Kobialka M, Foukas PG, Baumgaertner P, Meyer C, Ballabeni P, Michielin O, Weide B, Romero P, Speiser DE, et al. Ipilimumab-dependent cell-mediated cytotoxicity of regulatory T cells ex vivo by nonclassical monocytes in melanoma patients. Proc Natl Acad Sci U S A. 2015;112:6140–6145. doi:10.1073/pnas.1417320112.
- Das R, Verma R, Sznol M, Boddupalli CS, Gettinger SN, Kluger H, Callahan M, Wolchok JD, Halaban R, Dhodapkar MV, et al. Combination therapy with anti-CTLA-4 and anti-PD-1 leads to distinct immunologic changes in vivo. Journal of Immunology. 2015;194:950–959. doi:10.4049/jimmunol.1401686.
- Fransen MF, van der Sluis TC, Ossendorp F, Arens R, Melief CJ. Controlled local delivery of CTLA-4 blocking antibody induces CD8+ T-cell-dependent tumor eradication and decreases risk of toxic side effects. Clinical Cancer Research: An Official Journal of the American Association for Cancer Research. 2013;19:5381–5389. doi:10.1158/1078-0432.CCR-12-0781.
- Knorr DA, Dahan R, Ravetch JV. Toxicity of an Fc-engineered anti-CD40 antibody is abrogated by intratumoral injection and results in durable antitumor immunity. Proc Natl Acad Sci U S A. 2018; 115:11048–11053. doi:10.1073/pnas.1810566115.
- Narumi K, Miyakawa R, Shibasaki C, Henmi M, Mizoguchi Y, Ueda R, et al. Local administration of GITR agonistic antibody induces a stronger antitumor immunity than systemic delivery. Sci Rep. 2019;9:5562. doi:10.1038/s41598-019-41724-x.
- Houot R, Levy R. T-cell modulation combined with intratumoral CpG cures lymphoma in a mouse model without the need for chemotherapy. Blood. 2009;113:3546–3552. doi:10.1182/blood-2008-07-170274.
- Marabelle A, Tselikas L, De Baere T, Houot R. Intratumoral immunotherapy: using the tumor as the remedy. Annals of Oncology: Official Journal of the European Society for Medical Oncology. 2017;28:xii33–xii43. doi:10.1093/annonc/mdx683.
- Palazon A, Martinez-Forero I, Teijeira A, Morales-Kastresana A, Alfaro C, Sanmamed MF, Perez-Gracia JL, Peñuelas I, Hervás-Stubbs S, Rouzaut A, et al. The HIF-1α hypoxia response in tumor-infiltrating T lymphocytes induces functional CD137 (4-1BB) for immunotherapy. Cancer Discov. 2012;2(7):608–623. doi:10.1158/2159-8290.CD-11-0314.
- Tselikas L, De Baere T, Isoardo T, Susini S, Ser-Le Roux K, Polrot M, Adam J, Rouanne M, Zitvogel L, Moine L, et al. Pickering emulsions with ethiodized oil and nanoparticles for slow release of intratumoral anti-CTLA4 immune checkpoint antibodies. Journal for Immunotherapy of Cancer. 2020;8:e000579. doi:10.1136/jitc-2020-000579.
- Perrot I, Michaud HA, Giraudon-Paoli M, Augier S, Docquier A, Gros L, Courtois R, Déjou C, Jecko D, Becquart O, et al. Blocking antibodies targeting the CD39/CD73 immunosuppressive pathway unleash immune responses in combination cancer therapies. Cell Rep. 2019;27:2411–25 e9. doi:10.1016/j.celrep.2019.04.091.
- Rizzieri D. Zevalin((R)) (ibritumomab tiuxetan): after more than a decade of treatment experience, what have we learned? Crit Rev Oncol Hematol. 2016;105:5–17. doi:10.1016/j.critrevonc.2016.07.008.
- Mondello P, Cuzzocrea S, Navarra M, Mian M. 90 Y-ibritumomab tiuxetan: a nearly forgotten opportunityr. Oncotarget. 2016;7:7597–7609. doi:10.18632/oncotarget.6531.
- Dhillon S. Moxetumomab Pasudotox: first global approval. Drugs. 2018;78:1763–1767. doi:10.1007/s40265-018-1000-9.
- Peddi PF, Hurvitz SA. Trastuzumab emtansine: the first targeted chemotherapy for treatment of breast cancer. Future Oncol. 2013;9:319–326. doi:10.2217/fon.13.7.
- Goldenberg DM, Sharkey RM. Antibody-drug conjugates targeting TROP-2 and incorporating SN-38: a case study of anti-TROP-2 sacituzumab govitecan. MAbs. 2019;11:987–995. doi:10.1080/19420862.2019.1632115.
- Okamoto H, Oitate M, Hagihara K, Shiozawa H, Furuta Y, Ogitani Y, et al. Pharmacokinetics of trastuzumab deruxtecan (T-DXd), a novel anti-HER2 antibody-drug conjugate, in HER2-positive tumour-bearing mice. Xenobiotica. 2020;50:1242–1250. doi:10.1080/00498254.2020.1755909.
- Thomas A, Pommier Y. Targeting Topoisomerase I in the era of precision medicine. Clinical Cancer Research: An Official Journal of the American Association for Cancer Research. 2019;25:6581–6589. doi:10.1158/1078-0432.CCR-19-1089.
- Bruins WSC, Zweegman S, Mutis T, van de Donk N. Targeted therapy with immunoconjugates for multiple myeloma. Front Immunol. 2020;11:1155. doi:10.3389/fimmu.2020.01155.
- Ponziani S, Di Vittorio G, Pitari G, Cimini AM, Ardini M, Gentile R, Iacobelli S, Sala G, Capone E, Flavell DJ, et al. Antibody-drug conjugates: the new frontier of chemotherapy. Int J Mol Sci. 2020;21. doi:10.3390/ijms21155510
- Hamilton GS. Antibody-drug conjugates for cancer therapy: the technological and regulatory challenges of developing drug-biologic hybrids. Biologicals. 2015;43:318–332. doi:10.1016/j.biologicals.2015.05.006.
- Hassan R, Alewine C, Mian I, Spreafico A, Siu LL, Gomez-Roca C, Delord J-P, Italiano A, Lassen U, Soria J-C, et al. Phase 1 study of the immunotoxin LMB-100 in patients with mesothelioma and other solid tumors expressing mesothelin. Cancer. 2020;126:4936–4947. doi:10.1002/cncr.33145.
- Yang Y, Yang Z, Yang Y. Potential role of CD47-directed bispecific antibodies in cancer immunotherapy. Front Immunol. 2021;12:686031. doi:10.3389/fimmu.2021.686031.
- Salvaris R, Ong J, Gregory GP. Bispecific antibodies: a review of development, clinical efficacy and toxicity in B-cell lymphomas. J Pers Med. 2021;11:355. doi:10.3390/jpm11050355.
- Teng MW, Bowman EP, McElwee JJ, Smyth MJ, Casanova JL, Cooper AM, Cua DJ. IL-12 and IL-23 cytokines: from discovery to targeted therapies for immune-mediated inflammatory diseases. Nat Med. 2015;21:719–729. doi:10.1038/nm.3895.
- Dunn GP, Koebel CM, Schreiber RD. Interferons, immunity and cancer immunoediting. Nat Rev Immunol. 2006;6:836–848. doi:10.1038/nri1961.
- von Locquenghien M, Rozalen C, Celia-Terrassa T. Interferons in cancer immunoediting: sculpting metastasis and immunotherapy response. J Clin Invest. 2021;131(1). doi:10.1172/JCI143296.
- Medrano RFV, Hunger A, Mendonca SA, Barbuto JAM, Strauss BE. Immunomodulatory and antitumor effects of type I interferons and their application in cancer therapy. Oncotarget. 2017;8:71249–71284. doi:10.18632/oncotarget.19531.
- Castro F, Cardoso AP, Goncalves RM, Serre K, Oliveira MJ. Interferon-gamma at the crossroads of tumor immune surveillance or evasion. Front Immunol. 2018;9:847.
- Aldesleukin. LiverTox: clinical and research information on drug-induced liver injury. Bethesda (MD); 2012.
- Yan WL, Shen KY, Tien CY, Chen YA, Liu SJ. Recent progress in GM-CSF-based cancer immunotherapy. Immunotherapy. 2017;9:347–360. doi:10.2217/imt-2016-0141.
- Cavalheiro S, Di Rocco C, Valenzuela S, Dastoli PA, Tamburrini G, Massimi L, Nicacio JM, Faquini IV, Ierardi DF, Silva NS, et al. Craniopharyngiomas: intratumoral chemotherapy with interferon-alpha: a multicenter preliminary study with 60 cases. Neurosurg Focus. 2010;28:E12. doi:10.3171/2010.1.FOCUS09310.
- Baldo BA. Side effects of cytokines approved for therapy. Drug Saf. 2014;37:921–943.
- Vial T, Descotes J. Immune-mediated side-effects of cytokines in humans. Toxicology. 1995;105:31–57. doi:10.1016/0300-483X(95)03124-X.
- Momin N, Mehta NK, Bennett NR, Ma L, Palmeri JR, Chinn MM, Lutz EA, Kang B, Irvine DJ, Spranger S, et al. Anchoring of intratumorally administered cytokines to collagen safely potentiates systemic cancer immunotherapy. Sci Transl Med. 2019;11:eaaw2614. doi:10.1126/scitranslmed.aaw2614.
- Chiocca EA, Yu JS, Lukas RV, Solomon IH, Ligon KL, Nakashima H, Triggs DA, Reardon DA, Wen P, Stopa BM, et al. Regulatable interleukin-12 gene therapy in patients with recurrent high-grade glioma: results of a phase 1 trial. Sci Transl Med. 2019;11:eaaw5680. doi:10.1126/scitranslmed.aaw5680.
- van Herpen CM, Looman M, Zonneveld M, Scharenborg N, De Wilde PC, van de Locht L, Merkx MAW, Adema GJ, De Mulder PH. Intratumoral administration of recombinant human interleukin 12 in head and neck squamous cell carcinoma patients elicits a T-helper 1 profile in the locoregional lymph nodes. Clinical Cancer Research: An Official Journal of the American Association for Cancer Research. 2004;10:2626–2635. doi:10.1158/1078-0432.CCR-03-0304.
- Li Y, Su Z, Zhao W, Zhang X, Momin N, Zhang C, Wittrup KD, Dong Y, Irvine DJ, Weiss R. Multifunctional oncolytic nanoparticles deliver self-replicating IL-12 RNA to eliminate established tumors and prime systemic immunity. Nature Cancer. 2020;1:882–893. doi:10.1038/s43018-020-0095-6.
- Yao W, Li Y, Zeng L, Zhang X, Zhou Z, Zheng M, et al. Intratumoral injection of dendritic cells overexpressing interleukin12 inhibits melanoma growth. Oncol Rep. 2019;42:370–376.
- Akiyama Y, Watanabe M, Maruyama K, Ruscetti FW, Wiltrout RH, Yamaguchi K. Enhancement of antitumor immunity against B16 melanoma tumor using genetically modified dendritic cells to produce cytokines. Gene Ther. 2000;7:2113–2121. doi:10.1038/sj.gt.3301353.
- Wei X, Orchardson M, Gracie JA, Leung BP, Gao B, Guan H, et al. The Sushi domain of soluble IL-15 receptor alpha is essential for binding IL-15 and inhibiting inflammatory and allogenic responses in vitro and in vivo. Journal of Immunology. 2001;167:277–282. doi:10.4049/jimmunol.167.1.277.
- Bechter O, Utikal J, Baurain J-F, Massard C, Sahin U, Derhovanessian E, Ozoux M-L, Marpadga R, Imedio E-R, Acquavella N, et al. A first-in-human study of intratumoral SAR441000, an mRNA mixture encoding IL-12sc, interferon alpha2b, GM-CSF and IL-15sushi as monotherapy and in combination with cemiplimab in advanced solid tumors. Journal for ImmunoTherapy of Cancer. 2020;8:A416–A416. doi:10.1136/jitc-2020-SITC2020.0391.
- Yamane BH, Hank JA, Albertini MR, Sondel PM. The development of antibody-IL-2 based immunotherapy with hu14.18-IL2 (EMD-273063) in melanoma and neuroblastoma. Expert Opin Investig Drugs. 2009;18:991–1000. doi:10.1517/13543780903048911.
- Albertini MR, Ranheim E, Hank JA, Zuleger C, McFarland T, Collins J, Clements E, Weber SM, Weigel T, Neuman HB, et al. A pilot trial of hu14.18-IL2 in patients with completely resectable recurrent stage III or stage IV melanoma. Journal of Clinical Oncology. 2014;32:9044. doi:10.1200/jco.2014.32.15_suppl.9044.
- Danielli R, Patuzzo R, Di Giacomo AM, Gallino G, Maurichi A, Di Florio A, Cutaia O, Lazzeri A, Fazio C, Miracco C, et al. Intralesional administration of L19-IL2/L19-TNF in stage III or stage IVM1a melanoma patients: results of a phase II study. Cancer Immunology, Immunotherapy: CII. 2015;64:999–1009. doi:10.1007/s00262-015-1704-6.
- Corbellari R, Nadal L, Villa A, Neri D, De Luca R. The immunocytokine L19-TNF eradicates sarcomas in combination with chemotherapy agents or with immune check-point inhibitors. Anticancer Drugs. 2020;31:799–805. doi:10.1097/CAD.0000000000000938.
- Dias Junior AG, Sampaio NG, Rehwinkel J, Balancing Act: A. MDA5 in antiviral immunity and autoinflammation. Trends Microbiol. 2019;27:75–85. doi:10.1016/j.tim.2018.08.007.
- Rehwinkel J, Gack MU. RIG-I-like receptors: their regulation and roles in RNA sensing. Nat Rev Immunol. 2020;20:537–551. doi:10.1038/s41577-020-0288-3.
- Cerboni S, Gentili M, Manel N. Diversity of pathogen sensors in dendritic cells. Adv Immunol. 2013;120:211–237.
- Cui J, Chen Y, Wang HY, Wang RF. Mechanisms and pathways of innate immune activation and regulation in health and cancer. Hum Vaccin Immunother. 2014;10:3270–3285. doi:10.4161/21645515.2014.979640.
- Reis e Sousa C. Dendritic cells as sensors of infection. Immunity. 2001;14:495–498. doi:10.1016/S1074-7613(01)00136-4.
- Szabo A, Rajnavolgyi E. Collaboration of toll-like and RIG-I-like receptors in human dendritic cells: tRIGgering antiviral innate immune responses. Am J Clin Exp Immunol. 2013;2:195–207.
- Kepp O, Tesniere A, Schlemmer F, Michaud M, Senovilla L, Zitvogel L, Kroemer G. Immunogenic cell death modalities and their impact on cancer treatment. Apoptosis. 2009;14:364–375. doi:10.1007/s10495-008-0303-9.
- Tesniere A, Apetoh L, Ghiringhelli F, Joza N, Panaretakis T, Kepp O, Schlemmer F, Zitvogel L, Kroemer G. Immunogenic cancer cell death: a key-lock paradigm. Curr Opin Immunol. 2008;20:504–511. doi:10.1016/j.coi.2008.05.007.
- Vanpouille-Box C, Galluzzi L. Nucleic acid sensing at the interface between innate and adaptive immunity. Int Rev Cell Mol Biol. 2019;344:xi–xv.
- Bianchi F, Pretto S, Tagliabue E, Balsari A, Sfondrini L. Exploiting poly(I:C) to induce cancer cell apoptosis. Cancer Biol Ther. 2017;18:747–756. doi:10.1080/15384047.2017.1373220.
- Sultan H, Salazar AM, Celis E. Poly-ICLC, a multi-functional immune modulator for treating cancer. Semin Immunol. 2020;49:101414. doi:10.1016/j.smim.2020.101414.
- Kyi C, Roudko V, Sabado R, Saenger Y, Loging W, Mandeli J, Thin TH, Lehrer D, Donovan M, Posner M, et al. Therapeutic immune modulation against solid cancers with intratumoral poly-ICLC: a pilot trial. Clinical Cancer Research: An Official Journal of the American Association for Cancer Research. 2018;24:4937–4948. doi:10.1158/1078-0432.CCR-17-1866.
- Rodriguez-Ruiz ME, Perez-Gracia JL, Rodriguez I, Alfaro C, Onate C, Perez G, Gil-Bazo I, Benito A, Inogés S, López-Diaz de Cerio A, et al. Combined immunotherapy encompassing intratumoral poly-ICLC, dendritic-cell vaccination and radiotherapy in advanced cancer patients. Annals of Oncology: Official Journal of the European Society for Medical Oncology. 2018;29:1312–1319. doi:10.1093/annonc/mdy089.
- Alderson MR, McGowan P, Baldridge JR, Probst P. TLR4 agonists as immunomodulatory agents. J Endotoxin Res. 2006;12:313–319. doi:10.1177/09680519060120050701.
- Shetab Boushehri MA, Lamprecht A. TLR4-based immunotherapeutics in cancer: a review of the achievements and shortcomings. Mol Pharm. 2018;15(11):4777–4800. doi:10.1021/acs.molpharmaceut.8b00691.
- Petes C, Odoardi N, Gee K. The toll for trafficking: toll-like receptor 7 delivery to the endosome. Front Immunol. 2017;8:1075. doi:10.3389/fimmu.2017.01075.
- Chi H, Li C, Zhao FS, Zhang L, Ng TB, Jin G, Sha O. Anti-tumor activity of toll-like receptor 7 agonists. Front Pharmacol. 2017;8:304. doi:10.3389/fphar.2017.00304.
- Diab A, Tannir NM, Bentebibel SE, Hwu P, Papadimitrakopoulou V, Haymaker C, Kluger HM, Gettinger SN, Sznol M, Tykodi SS, et al. Bempegaldesleukin (NKTR-214) plus nivolumab in patients with advanced solid tumors: phase I dose-escalation study of safety, efficacy, and immune activation (PIVOT-02). Cancer Discov. 2020;10:1158–1173. doi:10.1158/2159-8290.CD-19-1510.
- Veatch JR, Singhi N, Jesernig B, Paulson KG, Zalevsky J, Iaccucci E, Tykodi SS, Riddell SR. Mobilization of pre-existing polyclonal T cells specific to neoantigens but not self-antigens during treatment of a patient with melanoma with bempegaldesleukin and nivolumab. Journal for Immunotherapy of Cancer. 2020;8:e001591. doi:10.1136/jitc-2020-001591.
- Cervantes JL, Weinerman B, Basole C, Salazar JC. TLR8: the forgotten relative revindicated. Cell Mol Immunol. 2012;9:434–438. doi:10.1038/cmi.2012.38.
- Chuang YC, Tseng JC, Huang LR, Huang CM, Huang CF, Chuang TH. Adjuvant effect of toll-like receptor 9 activation on cancer immunotherapy using checkpoint blockade. Front Immunol. 2020;11:1075. doi:10.3389/fimmu.2020.01075.
- Karapetyan L, Luke JJ, Davar D. Toll-like receptor 9 agonists in cancer. Onco Targets Ther. 2020;13:10039–10060. doi:10.2147/OTT.S247050.
- Krieg AM. Development of TLR9 agonists for cancer therapy. J Clin Invest. 2007;117:1184–1194. doi:10.1172/JCI31414.
- Krieg AM. Toll-like receptor 9 (TLR9) agonists in the treatment of cancer. Oncogene. 2008;27:161–167. doi:10.1038/sj.onc.1210911.
- Melisi D, Frizziero M, Tamburrino A, Zanotto M, Carbone C, Piro G, Tortora G. Toll-like receptor 9 agonists for cancer therapy. Biomedicines. 2014;2:211–228. doi:10.3390/biomedicines2030211.
- Haymaker C, Johnson DH, Murthy R, Bentebibel SE, Uemura MI, Hudgens CW, Safa H, James M, Andtbacka RHI, Johnson DB, et al. Tilsotolimod with ipilimumab drives tumor responses in anti-PD-1 refractory melanoma. Cancer Discov. 2021. doi:10.1158/2159-8290.CD-20-1546.
- Timmer FEF, Geboers B, Ruarus AH, Schouten EAC, Nieuwenhuizen S, Puijk RS, De Vries JJJ, Meijerink MR, Scheffer HJ. Irreversible electroporation for locally advanced pancreatic cancer. Tech Vasc Interv Radiol. 2020;23:100675. doi:10.1016/j.tvir.2020.100675.
- Crunkhorn S. Strengthening the sting of immunotherapy. Nat Rev Immunol. 2020;20:589. doi:10.1038/s41577-020-00447-1.
- Su T, Zhang Y, Valerie K, Wang X-Y, Lin S, Zhu G. STING activation in cancer immunotherapy. Theranostics. 2019;9(25):7759–7771. doi:10.7150/thno.37574.
- Harrington K, Parkes E, Weiss J, Ingham M, Cervantes A, Calvo E, Riese M, Klinkhardt U, Sikken P, Schmohl M, et al. Phase I, first-in-human trial evaluating BI 1387446 (stimulator of interferon genes [STING] agonist) alone and combined with BI 754091 (anti-programmed cell death [PD]-1) in solid tumors. J Immuno Therapy Cancer. 2020;8:A433–A433. doi:10.1136/jitc-2020-SITC2020.0408.
- Kasumba DM, Grandvaux N. Therapeutic targeting of RIG-I and MDA5 might not lead to the same rome. Trends Pharmacol Sci. 2019;40:116–127. doi:10.1016/j.tips.2018.12.003.
- Elion DL, Cook RS. Harnessing RIG-I and intrinsic immunity in the tumor microenvironment for therapeutic cancer treatment. Oncotarget. 2018;9(48):29007–29017. doi:10.18632/oncotarget.25626.
- Iurescia S, Fioretti D, Rinaldi M. The innate immune signalling pathways: turning RIG-I sensor activation against cancer. Cancers. 2020;12:3158. doi:10.3390/cancers12113158.
- Al-Ramadi BK, Fernandez-Cabezudo MJ, El-Hasasna H, Al-Salam S, Attoub S, Xu D, Chouaib S. Attenuated bacteria as effectors in cancer immunotherapy. Ann N Y Acad Sci. 2008;1138:351–357. doi:10.1196/annals.1414.036.
- Kaimala S, Al-Sbiei A, Cabral-Marques O, Fernandez-Cabezudo MJ, Al-Ramadi BK. Attenuated bacteria as immunotherapeutic tools for cancer treatment. Front Oncol. 2018;8:136. doi:10.3389/fonc.2018.00136.
- Kumar S, Sunagar R, Gosselin E. Bacterial protein toll-like-receptor agonists: a novel perspective on vaccine adjuvants. Front Immunol. 2019;10:1144. doi:10.3389/fimmu.2019.01144.
- Staedtke V, Roberts NJ, Bai RY, Zhou S. Clostridium novyi-NT in cancer therapy. Genes Dis. 2016;3:144–152. doi:10.1016/j.gendis.2016.01.003.
- Roberts NJ, Zhang L, Janku F, Collins A, Bai RY, Staedtke V, et al. Intratumoral injection of Clostridium novyi-NT spores induces antitumor responses. Sci Transl Med. 2014;6:249ra111. doi:10.1126/scitranslmed.3008982.
- Wang L, Wang Q, Tian X, Shi X. Learning from Clostridium novyi-NT: how to defeat cancer. J Cancer Res Ther. 2018;14:S1–S6. doi:10.4103/0973-1482.204841.
- Janku F, Fu S, Murthy R, Karp D, Hong D, Tsimberidou A, Gillison M, Adat A, Raina A, Call G, et al. First-in-man clinical trial of intratumoral injection of clostridium Novyi-NT spores in combination with pembrolizumab in patients with treatment-refractory advanced solid tumors. Journal for Immunotherapy of Cancer. 2020;8:A408–A408. doi:10.1136/jitc-2020-SITC2020.0383.
- Gebremeskel S, Johnston B. Concepts and mechanisms underlying chemotherapy induced immunogenic cell death: impact on clinical studies and considerations for combined therapies. Oncotarget. 2015;6:41600–41619. doi:10.18632/oncotarget.6113.
- Fucikova J, Kralikova P, Fialova A, Brtnicky T, Rob L, Bartunkova J, Špíšek R. Human tumor cells killed by anthracyclines induce a tumor-specific immune response. Cancer Res. 2011;71:4821–4833. doi:10.1158/0008-5472.CAN-11-0950.
- Kepp O, Bezu L, Yamazaki T, Di Virgilio F, Smyth MJ, Kroemer G, et al. ATP and cancer immunosurveillance. EMBO J. 2021;40:e108130.
- Galluzzi L, Vitale I, Warren S, Adjemian S, Agostinis P, Martinez AB, Chan TA, Coukos G, Demaria S, Deutsch E, et al. Consensus guidelines for the definition, detection and interpretation of immunogenic cell death. Journal for Immunotherapy of Cancer. 2020;8:e000337. doi:10.1136/jitc-2019-000337.
- Zhou H, Forveille S, Sauvat A, Yamazaki T, Senovilla L, Ma Y, Liu P, Yang H, Bezu L, Müller K, et al. The oncolytic peptide LTX-315 triggers immunogenic cell death. Cell Death Dis. 2016;7:e2134. doi:10.1038/cddis.2016.47.
- Humeau J, Sauvat A, Cerrato G, Xie W, Loos F, Iannantuoni F, Bezu L, Lévesque S, Paillet J, Pol J, et al. Inhibition of transcription by dactinomycin reveals a new characteristic of immunogenic cell stress. EMBO Mol Med. 2020;12:e11622. doi:10.15252/emmm.201911622.
- Spisek R, Charalambous A, Mazumder A, Vesole DH, Jagannath S, Dhodapkar MV. Bortezomib enhances dendritic cell (DC)-mediated induction of immunity to human myeloma via exposure of cell surface heat shock protein 90 on dying tumor cells: therapeutic implications. Blood. 2007;109:4839–4845. doi:10.1182/blood-2006-10-054221.
- Garg AD, More S, Rufo N, Mece O, Sassano ML, Agostinis P, et al. Trial watch: immunogenic cell death induction by anticancer chemotherapeutics. Oncoimmunology. 2017;6:e1386829. doi:10.1080/2162402X.2017.1386829.
- Christensen SB. Natural products that changed society. Biomedicines. 2021;9:472. doi:10.3390/biomedicines9050472.
- Twarog C, Fattah S, Heade J, Maher S, Fattal E, Brayden DJ. Intestinal permeation enhancers for oral delivery of macromolecules: a comparison between salcaprozate sodium (SNAC) and sodium caprate (C10). Pharmaceutics. 2019;11:78. doi:10.3390/pharmaceutics11020078.
- Zhou H, Mondragon L, Xie W, Mauseth B, Leduc M, Sauvat A, Gomes-da-Silva LC, Forveille S, Iribarren K, Souquere S, et al. Oncolysis with DTT-205 and DTT-304 generates immunological memory in cured animals. Cell Death Dis. 2018;9:1086. doi:10.1038/s41419-018-1127-3.
- Szczepanski C, Tenstad O, Baumann A, Martinez A, Myklebust R, Bjerkvig R, Prestegarden L. Identification of a novel lytic peptide for the treatment of solid tumours. Genes Cancer. 2014;5:186–200. doi:10.18632/genesandcancer.18.
- Sveinbjornsson B, Camilio KA, Haug BE, Rekdal O. LTX-315: a first-in-class oncolytic peptide that reprograms the tumor microenvironment. Future Med Chem. 2017;9:1339–1344. doi:10.4155/fmc-2017-0088.
- Li L, Liu S, Han D, Tang B, Ma J. Delivery and biosafety of oncolytic virotherapy. Front Oncol. 2020;10:475. doi:10.3389/fonc.2020.00475.
- Kepp O, Marabelle A, Zitvogel L, Kroemer G. Oncolysis without viruses - inducing systemic anticancer immune responses with local therapies. Nat Rev Clin Oncol. 2020;17:49–64. doi:10.1038/s41571-019-0272-7.
- Huang H, Liu Y, Liao W, Cao Y, Liu Q, Guo Y, Lu Y, Xie Z. Oncolytic adenovirus programmed by synthetic gene circuit for cancer immunotherapy. Nat Commun. 2019;10:4801. doi:10.1038/s41467-019-12794-2.
- Pol J, Buque A, Aranda F, Bloy N, Cremer I, Eggermont A, Erbs P, Fucikova J, Galon J, Limacher J-M, et al. Trial watch-oncolytic viruses and cancer therapy. Oncoimmunology. 2016;5:e1117740. doi:10.1080/2162402X.2015.1117740.
- Pol J, Bloy N, Obrist F, Eggermont A, Galon J, Cremer I, Erbs P, Limacher J-M, Preville X, Zitvogel L, et al. Trial watch: oncolytic viruses for cancer therapy. Oncoimmunology. 2014;3:e28694. doi:10.4161/onci.28694.
- Shi Y, Liu CH, Roberts AI, Das J, Xu G, Ren G, Zhang Y, Zhang L, Yuan ZR, Tan HSW, et al. Granulocyte-macrophage colony-stimulating factor (GM-CSF) and T-cell responses: what we do and don’t know. Cell Res. 2006;16:126–133. doi:10.1038/sj.cr.7310017.
- Fukuhara H, Ino Y, Todo T. Oncolytic virus therapy: a new era of cancer treatment at Dawn. Cancer Sci. 2016;107:1373–1379. doi:10.1111/cas.13027.
- Ma W, He H, Wang H. Oncolytic herpes simplex virus and immunotherapy. BMC Immunol. 2018;19:40. doi:10.1186/s12865-018-0281-9.
- Rehman H, Silk AW, Kane MP, Kaufman HL. Into the clinic: talimogene laherparepvec (T-VEC), a first-in-class intratumoral oncolytic viral therapy. Journal for Immunotherapy of Cancer. 2016;4:53. doi:10.1186/s40425-016-0158-5.
- Pol J, Kroemer G, Galluzzi L. First oncolytic virus approved for melanoma immunotherapy. Oncoimmunology. 2016;5:e1115641. doi:10.1080/2162402X.2015.1115641.
- Koch MS, Lawler SE, Chiocca EA. HSV-1 oncolytic viruses from bench to bedside: an overview of current clinical trials. Cancers. 2020;12:3514. doi:10.3390/cancers12123514.
- Wang X, Cui C, Si L, Li C, Dai J, Mao L, Bai X, Chi Z, Sheng X, Kong Y, et al. A phase Ib clinical trial of neoadjuvant OrienX010, an oncolytic virus, in combination with toripalimab in patients with resectable stage IIIb to stage IVM1a acral melanoma. Journal of Clinical Oncology. 2021;39:9570. doi:10.1200/JCO.2021.39.15_suppl.9570.
- Zhang B, Huang J, Tang J, Hu S, Luo S, Luo Z, Zhou F, Tan S, Ying J, Chang Q, et al. Intratumoral OH2, an oncolytic herpes simplex virus 2, in patients with advanced solid tumors: a multicenter, phase I/II clinical trial. Journal for Immunotherapy of Cancer. 2021;9:e002224. doi:10.1136/jitc-2020-002224.
- Friedman GK, Johnston JM, Bag AK, Bernstock JD, Li R, Aban I, Kachurak K, Nan L, Kang K-D, Totsch S, et al. Oncolytic HSV-1 G207 immunovirotherapy for pediatric high-grade Gliomas. N Engl J Med. 2021;384:1613–1622. doi:10.1056/NEJMoa2024947.
- Markert JM, Razdan SN, Kuo HC, Cantor A, Knoll A, Karrasch M, Nabors LB, Markiewicz M, Agee BS, Coleman JM, et al. A phase 1 trial of oncolytic HSV-1, G207, given in combination with radiation for recurrent GBM demonstrates safety and radiographic responses. Mol Ther. 2014;22:1048–1055. doi:10.1038/mt.2014.22.
- Peters C, Grandi P, Nigim F. Updates on oncolytic virus immunotherapy for cancers. Mol Ther Oncolytics. 2019;12:259–262. doi:10.1016/j.omto.2019.01.008.
- Haines BB, Denslow A, Grzesik P, Lee JS, Farkaly T, Hewett J, et al. ONCR-177, an oncolytic HSV-1 designed to potently activate systemic antitumor immunity. Cancer Immunol Res. 2021;9(3):291–308. doi:10.1158/2326-6066.CIR-20-0609.
- Macedo N, Miller DM, Haq R, Kaufman HL. Clinical landscape of oncolytic virus research in 2020. Journal for Immunotherapy of Cancer. 2020;8:e001486. doi:10.1136/jitc-2020-001486.
- Peter M, Kuhnel F. Oncolytic adenovirus in cancer immunotherapy. Cancers. 2020;12:3354. doi:10.3390/cancers12113354.
- Rosewell Shaw A, Suzuki M. Recent advances in oncolytic adenovirus therapies for cancer. Curr Opin Virol. 2016;21:9–15. doi:10.1016/j.coviro.2016.06.009.
- Dong W, Van Ginkel JW, Au KY, Alemany R, Meulenberg JJ, Van Beusechem VW. ORCA-010, a novel potency-enhanced oncolytic adenovirus, exerts strong antitumor activity in preclinical models. Hum Gene Ther. 2014;25:897–904. doi:10.1089/hum.2013.229.
- Goradel NH, Mohajel N, Malekshahi ZV, Jahangiri S, Najafi M, Farhood B, Mortezaee K, Negahdari B, Arashkia A. Oncolytic adenovirus: a tool for cancer therapy in combination with other therapeutic approaches. J Cell Physiol. 2019;234:8636–8646. doi:10.1002/jcp.27850.
- Springgay LK, Strauwald AM, Ewald B, Robbins JM, Chan WM. Abstract 6713: oncolytic adenoviruses expressing immune modulators enhance tumor cell killing in human cancer 3D microtumor models. Cancer Res. 2020;80:6713.
- Eriksson E, Milenova I, Wenthe J, Stahle M, Leja-Jarblad J, Ullenhag G, Dimberg A, Moreno R, Alemany R, Loskog A, et al. Shaping the tumor stroma and sparking immune activation by CD40 and 4-1BB signaling induced by an armed oncolytic virus. Clinical Cancer Research: An Official Journal of the American Association for Cancer Research. 2017;23:5846–5857. doi:10.1158/1078-0432.CCR-17-0285.
- Kumar V, Giacomantonio MA, Gujar S. Role of myeloid cells in oncolytic reovirus-based cancer therapy. Viruses. 2021;13:654. doi:10.3390/v13040654.
- Ku GY, Winter KA, Williams TM, Adusumilli PS, Nishimura M, Yock A, Ilson DH, Hong TS. NRG Oncology NRG-GI007 trial-in-progress: phase I study of OBP-301 (Telomelysin) and definitive chemoradiation (CRT) for patients with locally advanced esophageal and gastroesophageal adenocarcinoma who are not candidates for surgery. Journal of Clinical Oncology. 2021;39: TPS262. doi:10.1200/JCO.2021.39.3_suppl.TPS262.
- Jacobs BL, Langland JO, Kibler KV, Denzler KL, White SD, Holechek SA, Wong S, Huynh T, Baskin CR. Vaccinia virus vaccines: past, present and future. Antiviral Res. 2009;84:1–13. doi:10.1016/j.antiviral.2009.06.006.
- Belongia EA, Naleway AL. Smallpox vaccine: the good, the bad, and the ugly. Clin Med Res. 2003;1(2):87–92. doi:10.3121/cmr.1.2.87.
- Umer BA, Noyce RS, Franczak BC, Shenouda MM, Kelly RG, Favis NA, Desaulniers M, Baldwin TA, Hitt MM, Evans DH, et al. Deciphering the immunomodulatory capacity of oncolytic vaccinia virus to enhance the immune response to breast cancer. Cancer Immunol Res. 2020;8:618–631. doi:10.1158/2326-6066.CIR-19-0703.
- Marchand J-B, Semmrich M, Fend L, Rehn M, Silvestre N, Teige I, et al. Abstract 5602: BT-001, an oncolytic vaccinia virus armed with a Treg-depletion-optimized recombinant human anti-CTLA4 antibody and GM-CSF to target the tumor microenvironment. Cancer Res. 2020;80:5602.
- Kim JH, Oh JY, Park BH, Lee DE, Kim JS, Park HE, Roh MS, Je JE, Yoon JH, Thorne SH, et al. Systemic armed oncolytic and immunologic therapy for cancer with JX-594, a targeted poxvirus expressing GM-CSF. Mol Ther. 2006;14:361–370. doi:10.1016/j.ymthe.2006.05.008.
- Rha SY, Merchan J, Oh SY, Kim C, Bae WK, Lee HW, Dillon M, Deering R, Kroog G, Ha KS. A phase Ib study of recombinant vaccinia virus in combination with immune checkpoint inhibition (ICI) in advanced renal cell carcinoma (RCC). Cancer Res. 2020;80(16_suppl).
- Iankov ID, Haralambieva IH, Galanis E. Immunogenicity of attenuated measles virus engineered to express Helicobacter pylori neutrophil-activating protein. Vaccine. 2011;29:1710–1720. doi:10.1016/j.vaccine.2010.12.020.
- Holbrook MC, Goad DW, Grdzelishvili VZ. Expanding the spectrum of pancreatic cancers responsive to vesicular stomatitis virus-based oncolytic virotherapy: challenges and solutions. Cancers. 2021;13:1171. doi:10.3390/cancers13051171.
- Jenner AL, Cassidy T, Belaid K, Bourgeois-Daigneault M-C, Craig M. In silico trials predict that combination strategies for enhancing vesicular stomatitis oncolytic virus are determined by tumor aggressivity. Journal for Immunotherapy of Cancer. 2021;9:e001387. doi:10.1136/jitc-2020-001387.
- Galibert M-D, Gilot D, Migault M, Bachelot L, Journé F, Rogiers A, et al. Abstract 3048: a noncoding function of TYRP1 mRNA promotes melanoma growth. Cancer Res. 2017;77:3048.
- Ghanem G, Fabrice J. Tyrosinase related protein 1 (TYRP1/gp75) in human cutaneous melanoma. Mol Oncol. 2011;5(2):150–155. doi:10.1016/j.molonc.2011.01.006.
- Journe F, Id Boufker H, Van Kempen L, Galibert MD, Wiedig M, Sales F, Theunis A, Nonclercq D, Frau A, Laurent G, et al. TYRP1 mRNA expression in melanoma metastases correlates with clinical outcome. Br J Cancer. 2011;105:1726–1732. doi:10.1038/bjc.2011.451.
- Sznol M, Lutzky J, Adjei AA, Powell SF, He AR, Patel M, O’Day S, Pandya NB, Kaesshaefer S, Reckner M, et al. Phase II trial of Voyager-V1 (vesicular stomatitis virus expressing human IFNβ and NIS, VV1), in combination with cemiplimab (C) in patients with NSCLC, melanoma, HCC or endometrial carcinoma. Journal of Clinical Oncology. 2020;38: TPS3161. doi:10.1200/JCO.2020.38.15_suppl.TPS3161.
- Atherton MJ, Stephenson KB, Nikota JK, Hu QN, Nguyen A, Wan Y, Lichty BD. Preclinical development of peptide vaccination combined with oncolytic MG1-E6E7 for HPV-associated cancer. Vaccine. 2018;36:2181–2192. doi:10.1016/j.vaccine.2018.02.070.
- Pol JG, Acuna SA, Yadollahi B, Tang N, Stephenson KB, Atherton MJ, et al. Preclinical evaluation of a MAGE-A3 vaccination utilizing the oncolytic Maraba virus currently in first-in-human trials. Oncoimmunology. 2019;8:e1512329. doi:10.1080/2162402X.2018.1512329.
- Holl EK, Brown MC, Boczkowski D, McNamara MA, George DJ, Bigner DD, Gromeier M, Nair SK. Recombinant oncolytic poliovirus, PVSRIPO, has potent cytotoxic and innate inflammatory effects, mediating therapy in human breast and prostate cancer xenograft models. Oncotarget. 2016;7(48):79828–79841. doi:10.18632/oncotarget.12975.
- Walton RW, Brown MC, Sacco MT, Gromeier M, Pfeiffer JK. Engineered oncolytic poliovirus PVSRIPO subverts MDA5-dependent innate immune responses in cancer cells. J Virol. 2018;92. doi:10.1128/JVI.00879-18.
- Beasley GM, Nair SK, Farrow NE, Landa K, Selim MA, Wiggs CA, Jung S-H, Bigner DD, True Kelly A, Gromeier M, et al. Phase I trial of intratumoral PVSRIPO in patients with unresectable, treatment-refractory melanoma. Journal for Immunotherapy of Cancer. 2021;9:e002203. doi:10.1136/jitc-2020-002203.
- Sloan AE, Buerki RA, Murphy C, Kelly AT, Ambady P, Brown M, Butowski NA, Cavaliere R, Curry WT, Desjardins A, et al. LUMINOS-101: phase 2 study of PVSRIPO with pembrolizumab in recurrent glioblastoma. 2021;39(15_suppl).
- Burman B, Niu J, Leidner RS, Wang D, Richardson DL, Iacobucci C, Hwang A, Qing X, Matushansky I, Zamarin D, et al. A phase I/II study of HB-201, an arenavirus-based cancer immunotherapy, alone, or in combination with anti-PD-1 in patients with HPV16+ cancers. Journal of Clinical Oncology. 2020;38(15).
- Shaw AR, Suzuki M. Immunology of adenoviral vectors in cancer therapy. Mol Ther Methods Clin Dev. 2019;15:418–429. doi:10.1016/j.omtm.2019.11.001.
- Suzawa K, Shien K, Peng H, Sakaguchi M, Watanabe M, Hashida S, MAKI Y, YAMAMOTO H, TOMIDA S, SOH J, et al. Distant bystander effect of REIC/DKK3 gene therapy through immune system stimulation in thoracic malignancies. Anticancer Res. 2017;37:301–307. doi:10.21873/anticanres.11321.
- Sobol RE, Chada S, Wiederhold DB, Yun C-O, Ahn H, Oh E, Murthy R, Subbiah V, Menander KB. Effect of adenoviral p53 (Ad-p53) tumor suppressor immune gene therapy on checkpoint inhibitor resistance and abscopal therapeutic efficacy. Journal of Clinical Oncology. 2017;35:e14610–e. doi:10.1200/JCO.2017.35.15_suppl.e14610.
- Sobol RE, Menander KB, Chada S, Wiederhold D, Sellman B, Talbott M, Nemunaitis JJ. Analysis of adenoviral p53 gene therapy clinical trials in recurrent head and neck squamous cell carcinoma. Front Oncol. 2021;11:645745. doi:10.3389/fonc.2021.645745.
- Madan RA, Arlen PM, Mohebtash M, Hodge JW, Gulley JL. Prostvac-VF: a vector-based vaccine targeting PSA in prostate cancer. Expert Opin Investig Drugs. 2009;18:1001–1011. doi:10.1517/13543780902997928.
- Maughan BL, Sanchez A, O’Neil BB, Lowrance WT, Dechet CB, Albertson DJ, Sirohi D, Gupta S, Swami U, Agarwal N. A phase Ib/II trial of perioperative intratumoral MVA-BN-brachyury (MVA) plus systemic PROSTVAC and atezolizumab (Atezo) for intermediate-risk and high-risk localized prostate cancer (AtezoVax). Journal of Clinical Oncology. 2020;36(6_suppl).
- Li C-X, Qi Y-D, Feng J, Zhang X-Z. Cell-based bio-hybrid delivery system for disease treatments. Advanced Nanobiomed Research. 2021;2000052. doi:10.1002/anbr.202000052.
- Kabiljo J, Laengle J, Bergmann M. From threat to cure: understanding of virus-induced cell death leads to highly immunogenic oncolytic influenza viruses. Cell Death Discov. 2020;6:48. doi:10.1038/s41420-020-0284-1.
- Jou J, Harrington KJ, Zocca MB, Ehrnrooth E, Cohen EEW. The changing landscape of therapeutic cancer vaccines-novel platforms and neoantigen identification. Clinical Cancer Research: An Official Journal of the American Association for Cancer Research. 2021;27:689–703. doi:10.1158/1078-0432.CCR-20-0245.
- Saxena M, Van Der Burg SH, Melief CJM, Bhardwaj N. Therapeutic cancer vaccines. Nat Rev Cancer. 2021;21:360–378.
- Guo C, Manjili MH, Subjeck JR, Sarkar D, Fisher PB, Wang XY. Therapeutic cancer vaccines: past, present, and future. Adv Cancer Res. 2013;119:421–475.
- Liu W, Tang H, Li L, Wang X, Yu Z, Li J. Peptide-based therapeutic cancer vaccine: current trends in clinical application. Cell Prolif. 2021;54:e13025. doi:10.1111/cpr.13025.
- Peng M, Mo Y, Wang Y, Wu P, Zhang Y, Xiong F, Guo C, Wu X, Li Y, Li X, et al. Neoantigen vaccine: an emerging tumor immunotherapy. Mol Cancer. 2019;18:128. doi:10.1186/s12943-019-1055-6.
- Laumont CM, Vincent K, Hesnard L, Audemard E, Bonneil E, Laverdure JP, Gendron P, Courcelles M, Hardy M-P, Côté C, et al. Noncoding regions are the main source of targetable tumor-specific antigens. Sci Transl Med. 2018;10:eaau5516. doi:10.1126/scitranslmed.aau5516.
- Ehx G, Larouche JD, Durette C, Laverdure JP, Hesnard L, Vincent K, et al. Atypical acute myeloid leukemia-specific transcripts generate shared and immunogenic MHC class-I-associated epitopes. Immunity. 2021;54:737–52 e10. doi:10.1016/j.immuni.2021.03.001.
- Pol J, Bloy N, Buque A, Eggermont A, Cremer I, Sautes-Fridman C, Galon J, Tartour E, Zitvogel L, Kroemer G, et al. Trial watch: peptide-based anticancer vaccines. Oncoimmunology. 2015;4:e974411. doi:10.4161/2162402X.2014.974411.
- Bezu L, Kepp O, Cerrato G, Pol J, Fucikova J, Spisek R, Zitvogel L, Kroemer G, Galluzzi L. Trial watch: peptide-based vaccines in anticancer therapy. Oncoimmunology. 2018;7:e1511506. doi:10.1080/2162402X.2018.1511506.
- Markowitz J, Brohl A, Sarnaik AA, Eroglu Z, De Aquino DB, Khushalani N, et al. Abstract CT241: trial in progress: iFx-Hu2.0 (plasmid DNA coding for Emm55 streptococcal antigen in a cationic polymer) phase I first in human study for unresectable stage III or stage IV cutaneous melanoma. Cancer Res. 2020;80:CT241–CT.
- Markowitz J, Kodumudi KN, Aquino DBD, Sondak VK, Pilon-Thomas S. Abstract CT119: trial in progress: first in human Phase I study using a plasmid DNA coding for Emm55 streptococcal antigen (IFx-Hu2.0) in patients with unresectable stage III or stage IV cutaneous melanoma. Cancer Res. 2019;79:CT119–CT.
- Madan RA, Antonarakis ES, Drake CG, Fong L, Yu EY, McNeel DG, Lin DW, Chang NN, Sheikh NA, Gulley JL, et al. Putting the pieces together: completing the mechanism of action Jigsaw for Sipuleucel-T. J Natl Cancer Inst. 2020;112:562–573. doi:10.1093/jnci/djaa021.
- Anassi E, Ndefo UA. Sipuleucel-T (provenge) injection: the first immunotherapy agent (vaccine) for hormone-refractory prostate cancer. P T. 2011;36:197–202.
- Handy CE, Antonarakis ES. Sipuleucel-T for the treatment of prostate cancer: novel insights and future directions. Future Oncol. 2018;14:907–917. doi:10.2217/fon-2017-0531.
- Magill ST, Choy W, Nguyen MP, McDermott MW. Ommaya reservoir insertion: a technical note. Cureus. 2020;12:e7731.
- Kar UK, Srivastava MK, Andersson A, Baratelli F, Huang M, Kickhoefer VA, Dubinett SM, Rome LH, Sharma S, et al. Novel CCL21-vault nanocapsule intratumoral delivery inhibits lung cancer growth. PloS One. 2011;6:e18758. doi:10.1371/journal.pone.0018758.
- Stefanski HE, Jonart L, Goren E, Mule JJ, Blazar BR, Labrecque N. A novel approach to improve immune effector responses post transplant by restoration of CCL21 expression. PloS One. 2018;13:e0193461. doi:10.1371/journal.pone.0193461.
- Lee JM, Garon EB, Baratelli F, Schaue D, Pak PS, Wallace WD, Suh R, Abtin F, Zeng G, Dubinett SM. Phase I trial of CCL21 gene modified dendritic cells in non-small cell lung cancer. Journal of Clinical Oncology. 2010;28(15_suppl).
- Rosenberg SA, Restifo NP, Yang JC, Morgan RA, Dudley ME. Adoptive cell transfer: a clinical path to effective cancer immunotherapy. Nat Rev Cancer. 2008;8:299–308. doi:10.1038/nrc2355.
- Xie G, Dong H, Liang Y, Ham JD, Rizwan R, Chen J. CAR-NK cells: a promising cellular immunotherapy for cancer. EBioMedicine. 2020;59:102975. doi:10.1016/j.ebiom.2020.102975.
- Kundu S, Gurney M, O’Dwyer M. Generating natural killer cells for adoptive transfer: expanding horizons. Cytotherapy. 2021;23:559–566. doi:10.1016/j.jcyt.2020.12.002.
- Beyar-Katz O, Gill S. Advances in chimeric antigen receptor T cells. Curr Opin Hematol. 2020;27:368–377. doi:10.1097/MOH.0000000000000614.
- Han D, Xu Z, Zhuang Y, Ye Z, Qian Q. Current progress in CAR-T cell therapy for hematological malignancies. J Cancer. 2021;12:326–334. doi:10.7150/jca.48976.
- Approvals expand multiple myeloma treatment options. Cancer Discov. 2021; 11: OF5. doi:10.1158/2159-8290.CD-NB2021-0338.
- Powell K, Russler-Germain D, Prasad V. Idecabtagene vicleucel: questions regarding the appropriate role and cost. Br J Haematol. 2021. doi:10.1111/bjh.17784.
- Rodriguez-Lobato LG, Ganzetti M, Fernandez de Larrea C, Hudecek M, Einsele H, Danhof S. CAR T-cells in multiple myeloma: state of the art and future directions. Front Oncol. 2020;10:1243.
- Sadeqi Nezhad M, Yazdanifar M, Abdollahpour-Alitappeh M, Sattari A, Seifalian A, Bagheri N. Strengthening the CAR-T cell therapeutic application using CRISPR/Cas9 technology. Biotechnol Bioeng. 2021;118:3691–3705. doi:10.1002/bit.27882.
- De Jaeghere EA, Denys HG, De Wever O. Fibroblasts fuel immune escape in the tumor microenvironment. Trends Cancer. 2019;5:704–723. doi:10.1016/j.trecan.2019.09.009.
- Petroni G, Buque A, Yamazaki T, Bloy N, Liberto MD, Chen-Kiang S, Formenti SC, Galluzzi L. Radiotherapy delivered before CDK4/6 inhibitors mediates superior therapeutic effects in ER breast cancer. Clinical Cancer Research: An Official Journal of the American Association for Cancer Research. 2021;27:1855–1863. doi:10.1158/1078-0432.CCR-20-3871.
- Pilones KA, Hensler M, Daviaud C, Kraynak J, Fucikova J, Galluzzi L, et al. Converging focal radiation and immunotherapy in a preclinical model of triple negative breast cancer: contribution of Vista blockade. Oncoimmunology. 2020;9:1830524. doi:10.1080/2162402X.2020.1830524.
- Yamazaki T, Buque A, Ames TD, Galluzzi L. PT-112 induces immunogenic cell death and synergizes with immune checkpoint blockers in mouse tumor models. Oncoimmunology. 2020;9(1):1721810. doi:10.1080/2162402X.2020.1721810.
- Chen DS, Mellman I. Oncology meets immunology: the cancer-immunity cycle. Immunity. 2013;39:1–10. doi:10.1016/j.immuni.2013.07.012.
- Schwarze JK, Awada G, Cras L, Tijtgat J, Forsyth R, Dufait I, et al. Intratumoral combinatorial administration of CD1c (BDCA-1)(+) myeloid dendritic cells plus ipilimumab and avelumab in combination with intravenous low-dose nivolumab in patients with advanced solid tumors: a phase IB clinical trial. Vaccines (Basel). 2020 Nov 10;8(4):670.
- Jongbloed SL, Kassianos AJ, McDonald KJ, Clark GJ, Ju X, Angel CE, Chen CJJ, Dunbar PR, Wadley RB, Jeet V, et al. Human CD141+ (BDCA-3)+ dendritic cells (DCs) represent a unique myeloid DC subset that cross-presents necrotic cell antigens. J Exp Med. 2010;207:1247–1260. doi:10.1084/jem.20092140.
- Patel MR, Bauer TM, Jimeno A, Wang D, LoRusso P, Do KT, Stemmer SM, Maurice-Dror C, Geva R, Zacharek S, et al. A phase I study of mRNA-2752, a lipid nanoparticle encapsulating mRNAs encoding human OX40L, IL-23, and IL-36γ, for intratumoral (iTu) injection alone and in combination with durvalumab. Journal of Clinical Oncology. 2020;38:3092.
- Beck JD, Reidenbach D, Salomon N, Sahin U, Tureci O, Vormehr M, Kranz LM. mRNA therapeutics in cancer immunotherapy. Mol Cancer. 2021;20:69. doi:10.1186/s12943-021-01348-0.
- Lardone RD, Chan AA, Lee AF, Foshag LJ, Faries MB, Sieling PA, Lee DJ. Mycobacterium bovis bacillus calmette-guerin alters melanoma microenvironment favoring antitumor T cell responses and improving M2 macrophage function. Front Immunol. 2017;8:965. doi:10.3389/fimmu.2017.00965.
- Klinghoffer RA, Bahrami SB, Hatton BA, Frazier JP, Moreno-Gonzalez A, Strand AD, Kerwin WS, Casalini JR, Thirstrup DJ, You S, et al. A technology platform to assess multiple cancer agents simultaneously within a patient’s tumor. Sci Transl Med. 2015;7:284ra58. doi:10.1126/scitranslmed.aaa7489.
- Klinghoffer R, Thompson MJ, Davidson DJ, Bertout JA, Sottero KHW, Grenley MO, et al. Multidrug analyses in cancer patients via intratumoral microdosing with CIVO: a microinjection technology for phase 0 drug investigation. Journal of Clinical Oncology. 2018;36:e23569–e. doi:10.1200/JCO.2018.36.15_suppl.e23569.
- Nakamura A, Song K, Grossman S, Xega K, Zhang Y, Berger A, et al. 552 SUMOylation inhibitor TAK-981 activates NK cells and macrophages via type I interferon signaling and shows synergistic activity in combination with rituximab and daratumumab in preclinical models. Journal for Immunotherapy of Cancer. 2020;8(Suppl 3):A334–A5. doi:10.1136/jitc-2020-SITC2020.0552.
- Pfirschke C, Engblom C, Rickelt S, Cortez-Retamozo V, Garris C, Pucci F, Yamazaki T, Poirier-Colame V, Newton A, Redouane Y, et al. Immunogenic chemotherapy sensitizes tumors to checkpoint blockade therapy. Immunity. 2016;44:343–354. doi:10.1016/j.immuni.2015.11.024.
- Twyman-Saint Victor C, Rech AJ, Maity A, Rengan R, Pauken KE, Stelekati E, Benci JL, Xu B, Dada H, Odorizzi PM, et al. Radiation and dual checkpoint blockade activate non-redundant immune mechanisms in cancer. Nature. 2015;520:373–377. doi:10.1038/nature14292.