ABSTRACT
Metformin, a drug prescribed to treat type 2 diabetes, has been reported to possess antitumor activity via immunity activation. However, the influence of metformin on natural killer (NK) cells is not fully understood. Here, we investigated whether metformin exerts a potent anticancer effect by activating NK cells. The results showed that sustained exposure to metformin enhances the cytolytic activity of NK-92 cells. Moreover, this enhancement of cytotoxicity by metformin was also observed in NK cells from healthy peripheral blood and cancer patient ascites. Mechanistically, metformin induced activation of the JAK1/2/3/STAT5 and AKT/mTOR pathways in a p38 MAPK-dependent manner rather than an AMPK-dependent manner. In vivo experiments, metformin also improved cancer surveillance of NK cells in mouse models of lymphoma clearance and metastatic melanoma. Additionally, combination treatment with metformin and anti-PD-1 antibodies increased the therapy response rates of B16F10 melanoma. Moreover, metformin treatment increased NK cell and T cell infiltration in tumors. Therefore, these results provide a deeper understanding of metformin on the effector function of NK cells and will contribute to the development and applications of metformin in cancer treatment strategies.
1. Introduction
Natural killer (NK) cells are cytotoxic lymphocytes in the innate immune system. NK cells play critical roles in early control of the body’s antitumor immune response and intracellular pathogen infections.1–Citation3 Unlike T and B cells, NK cells exhibit broad tumor cytotoxicity by recognizing nonself cells without the requirement for prior stimulation or major histocompatibility complex (MHC) restrictions.Citation4 NK cells also initiate the adaptive immune response to cancer.Citation5 Numerous human studies have shown that NK cell functional deficiency is a key risk factor for developing various types of cancer and is a typical feature of diverse patients with cancer.Citation6–10 In support of this notion, high incidences of tumors and metastasis were reported in experimental mice with defects in NK cell number, function, or both.Citation11,Citation12 Moreover, the extent of NK cell dysfunction is correlated with the cancer prognosis.Citation13,Citation14 Due to the crucial roles of NK cells in immune responses, various strategies have been developed to restore NK cell function, including adoptive cell transfer, cytokine therapies, and monoclonal antibodies targeting activating and inhibitory receptors and the tumor microenvironment.Citation15–17 However, these methods have several limitations. For example, cytokines used on NK cells during in vivo expansion generate various side effects. High-dose interleukin-2 (IL-2) results in severe capillary leak syndrome, and low-dose IL-2 has the disadvantage of stimulating CD25hi regulatory T cells (Tregs).Citation18 Although IL-15 improves NK cell homeostasis, expansion, and cytolytic capacity without expanding Tregs, it has cytokine-associated toxicities similar to those observed with IL-2, including capillary leak syndrome, hypotension, fever, and chills.Citation19 Therefore, there is an urgent need to develop novel and safe strategies to enhance NK cell activity for targeted cancer therapy.
Metformin is a widely used oral medication to treat type 2 diabetes (T2D) and has been recognized as a safe and well-tolerated drug based on several decades of clinical experience.Citation20 Interestingly, several case-control studies for patients with T2D have described unexpected anticancer effects.Citation21,Citation22 Mounting evidence suggests that metformin can reduce the tumor burden in various cancer types, including lung, prostate, colon and breast cancer.Citation23–26 Many in vivo studies have reported that metformin can modulate the antitumor immune responses. Metformin maintains high cytotoxic T lymphocyte (CTL) activity in tumor tissues,Citation27 and enhances the antitumor activity of CD8+ T lymphocytes.Citation28,Citation29 Moreover, a polarization shift from M2 to M1 of macrophages is inhibited by metformin.Citation30 Metformin also reduces of CD39 and CD73 to block myeloid-derived suppressor cell activity in patients with ovarian cancer.Citation31 In addition, metformin down-regulates the level of chemokines and pro-inflammatory cytokines, and reduces intratumoral hypoxic area. It has been considered that gut microbiota may be an important mediator of metformin which positively affects anti-tumor immunity in vivo.Citation32 Although metformin on the many immune cell populations has been studied, the influence of metformin on NK cells is still not clear.
In this study, wedemonstrated the direct activation effects of metformin on NK cells, which eventually results in the inhibition of tumor metastasis and growth, and improves the therapeutic effects of PD-1 antibody. Mechanistically, Metformin significantly enhanced NK cell cytotoxicity via JAK1/2/3/STAT5 and AKT/mTOR pathway activation in a p38 MAPK-dependent manner rather than an AMPK-dependent manner. The upregulation of NK function and will be significant for development of metformin in cancer therapy, and may pave a new avenue to understand potent anticancer immunity effect of metformin .
2. Materials and methods
2.1 Mice and human samples
All animal work was performed in accordance with the Institutional Animal Care and was approved by the Ethics for Animal Experiments committee of the Ocean University of China (OUC-SMP-2019-06-02). All mice were housed in specific pathogen-free conditions. Six- to eight-week-old mice with similar weights and mixed genders were randomized prior to experimentation. C57BL/6 mice were purchased from the Jinan Pengyue Experimental Animal Center. Human blood samples from healthy adult donors and ascites samples from lung adenocarcinoma patients were obtained from the Qilu Hospital of Shandong University (Qingdao) under protocols (KYLL-2016038) approved by the ethics committee.
2.2 Cell lines and cell culture
Human NIH-H1975, A549, K562, MCF-7, and A2058 cells and mouse YAC-1, RMA (murine T-cell lymphoma), and B16F10 cells were purchased from the Cell Bank of the Chinese Academy of Sciences (Shanghai, China). RMA-S (an RMA variant with decreased cell surface expression of major histocompatibility complex class I molecules) was constructed and conserved by our laboratory. K562 cells were cultured in Iscove’s modified Dulbecco’s medium (IMDM) (HyClone Laboratories, Logan, UT), and NIH-H1975, A549, YAC-1, RMA, and RMA-S cells were maintained in tissue culture flasks with RPMI 1640 (HyClone Laboratories, Logan, UT). A2058 and B16F10 cells were cultured in Dulbecco’s modified Eagle’s medium (DMEM) (HyClone Laboratories, Logan, UT), both containing 10% fetal bovine serum (FBS, HyClone) and penicillin (100 U/mL)/streptomycin (100 μg/mL) (Gibco BRL, Grand Island, NY). NK-92 cells were cultured in Alpha Minimum Essential Medium (HyClone) supplemented with 12.5% horse serum (Gibco BRL), 12.5% FBS (HyClone), IL-2 (100 U/mL) (PeproTech, Rocky Hill, NJ, USA) and penicillin (100 U/mL)/streptomycin (100 μg/mL). All incubations were performed at 37°C in a 5% CO2-containing atmosphere.
2.3 NK cells and T cells activation
PBMCs were cultured for at least 1 week in RPMI-1640 complete medium with 10 ng/ml IL-2 (PeproTech) and 20 ng/ml IL-15 (PeproTech) for NK cells activation and expansion before use. Mice splenocytes were cultured in RPMI-1640 complete medium containing 20 ng/ml IL-15/IL-15 R (eBioscience) for at least 3 days for NK cells activation before use as described previously.Citation33 To acquire activated T cells, PBMCs were cultured in RPMI-1640 with Human CD3/CD28 T cell activator (10970; STEMCELL Technologies) and IL-2 (10 ng/mL; PeproTech) for one week as described previously.Citation29
2.4 Cell viability assay
The cells were treated with metformin at a series of concentrations (0, 1, 10, 100, and 1000 μM), and the cell viability was measured using Cell Counting Kit-8 (Beyotime Institute of Biotechnology, Shanghai, China) following the manufacturer’s general protocol.
2.5 Cytotoxicity assay
NK cell-mediated cytotoxicity was determined with a lactate dehydrogenase (LDH) assay (Beyotime Institute of Biotechnology, Shanghai, China). Cytotoxicity against cancer cells was measured at effector/target (E/T) ratios of 5:1 at 4 h.
For flow cytometric analysis, K562 cells were prelabeled with carboxyfluorescein diacetate succinimidyl ester (CFSE) and cocultured with effector cells. Subsequently, the cells were stained with PI. CFSE (+) PI (+) double-positive cells were defined as damaged K562 cells.
2.6 Enzyme-linked immunosorbent assays
ELISA kits for the detection of human IFN-γ, granzyme B and perforin (Dakewe, Beijing, China) were used. Briefly, NK-92 cells treated with various concentrations of metformin or not were cultured in 24-well plates (3 × 105/ml) for 48 h. Target cells (5 × 103) were plated in 96-cell plates, effector cells were added to the plates at effector/target (E/T) ratios of 5:1 and incubated for 12 h at 37°C, and then the supernatants were collected for ELISA according to the manufacturer’s instructions.
2.7 Cell transfection
The short interfering RNAs that targeted AMPK (si-AMPK), p38 MAPK (si- p38 MAPK) and corresponding siRNA negative controls (si NC) were purchased from Shanghai GenePharma Co., Ltd. Transfections were performed using the Lipofectamine 3000 kit (Invitrogen; Thermo Fisher Scientific, Inc.), according to the manufacturer’s protocol. The sequences were as follows:
si-AMPK 5ʹ-GAGCGACUAUCAAAGACAUTT-3ʹ;
si- p38 MAPK 5ʹ-GGGGCAGTTAGTACTTAAGTA-3ʹ
2.8 Western blot analysis
Harvested cells were homogenized in RIPA supplemented with protease inhibitor cocktail at 4°C for 30 min. Equal amounts of protein per sample were fractionated by SDS-PAGE. The protein bands were transferred onto nitrocellulose filter membranes, blocked with 5% nonfat milk for 1 h at room temperature. The antibodies used are summarized in Table S1. The relative densities of the protein bands were analyzed with NIH ImageJ software.
2.9 In vivo pulmonary metastasis assay
B16F10 melanoma cells were collected from cell cultures by trypsinization and then injected into the tail veins of C57BL/6 mice (2.5 × 105 cells in 100 μl of PBS). To deplete the NK cells, i.p. injections of rabbit anti-asialo-GM1 (50 µl) or control rabbit serum (Sigma-Aldrich) were performed for 1 day before an injection of B16F10 cells. After 14 days of tumor implantation, the mice were euthanized, and their lungs were fixed in Fekete’s solution. The number of nodules of metastatic tumor cells in the lungs was determined by using a dissection microscope.
2.10 In vivo lymphoma clearance assay
Lymphoma cells expressing MHC class-I (RMA) were labeled with a low CFSE concentration (0.3 µM), whereas those with defective expression of MHC class-I (RMA-s) were labeled with a high CFSE concentration (6 µM). The cells were mixed in a 1:1 ratio (1 × 106 cells per cell type) and injected i.p. into C57BL/6 mice for 12 h. To deplete the NK cells, i.p. injections of rabbit anti-asialo-GM1 (50 µl) or control rabbit serum were performed for 1 day before an injection of CFSE-stained RMA and RMA-s cells at a ratio of 1:1. Rejection of NK cell-sensitive RMA-s relative to NK cell-resistant RMA cells in the peritoneal cavity was measured using flow cytometry and calculated as follows: 1 – ([CFSE low/CFSE high] input/[CFSE low/CFSE high] output) × 100%.
2.11 Metformin plus anti-PD1 therapy
For depletion of the NK cells, the mice were administered i.p. injections of 50 µL rabbit anti-asialo-GM1 at Day 1. Then, B16F10 melanoma cells (5 × 105 cells per mouse) were subcutaneously inoculated into C57BL/6 mice on day 0, and the mice were subsequently randomly separated into groups. From Day 3, mice were started on either 0.2 mg anti-PD-1 (clone RPM1-14, Bio X cell) or hamster IgG isotype control (clone 2A3, Bio X Cell) and injected every 3 days intraperitoneally, and metformin (1,1-dimethylbiguanide hydrochloride, purchased from Solarbio (Beijing, China)) (100 mg/kg) or PBS were injected daily intraperitoneally. Starting at the onset of the antitumor experiment, the body weight of the mice was measured every 3 days, and the tumor sizes were measured every 3 days starting from Day 7. The tumor sizes (cm3) were calculated as 0.5 × A × B,Citation1Citation2 where A was the longest length and B was the shortest length of the tumor. On Day 21, the mice were euthanized using CO2 inhalation. Spleens and tumors were immediately collected.
2.12 Flow-cytometry analyses
Single-cell suspensions of splenocytes were prepared. Antibodies (clones) targeting the following proteins were purchased from BD Biosciences (San Jose, CA). The antibodies used are summarized in Table S2. Subsequently, the cells were analyzed using FACS Canto flow cytometry, and the data were analyzed using FlowJo software. The results of the flow cytometry are presented as percentages of the positive fluorescent cells.
2.13 Immunohistochemistry
Tumor tissues were fixed in 4% paraformaldehyde; the paraffin-embedded tissue sections (4 µm) were deparaffinized, dehydrated and treated with 3% hydrogen peroxide to block endogenous peroxidase. The slides were incubated with the following primary antibodies summarized in Table S2. The images were acquired at 20× magnification using a ZEISS Axioskop2 plus advanced positive microscope.
2.14 Statistical analysis
The data were analyzed using GraphPad Prism 5.0 (GraphPad Inc., San Diego, CA, USA) software. Data are expressed as the mean± SEM. Statistical analyses were conducted by two-tailed Student’s t-test or one-way ANOVA. Two-way ANOVA with multiple comparisons was used to compare tumor growth over time. Dunnett’s test was performed for multiple comparison posttests. P values < .05 were considered statistically significant.
3. Results
3.1 Metformin promotes NK cell-mediated lysis activity directly.
We first determined whether metformin regulates NK cell cytolytic activity directly. NK-92 cells were treated with metformin at different concentrations, then washed and co-cultured with various cancer cells to evaluate the cytolytic activity with an LDH assay. As shown in , the cytolysis activity of the NK-92 cells was remarkably increased in a dose-dependent manner with maximum kill rate more than doubled control. In addition, NK-92 cells were treated with 100 µM metformin for 24, 48, and 72 h and then washed and co-cultured with the target cells K562, and the results revealed that metformin upregulated NK-92 cell-mediated cytotoxicity in a time-dependent manner ()). Moreover, in these experiments, after being exposed to metformin, the cell viabilities of NK-92, A549, H1975, A2058, K562 and MCF-7 cells were not obviously changed, indicating that metformin did not cause cytotoxicity to these cells in the indicated concentrations of metformin (Fig. S1).
Figure 1. Metformin promotes NK cell-mediated lysis activity. NK-92 cell cytotoxicity toward cancer cell lines was measured using an LDH release assay (a). NK92 cells were treated with 100 µM metformin for 24 h, 48 h or 72 h, and then cytotoxicity toward K562 cells was measured using an LDH release assay (b). NK-92 cells were treated with two different concentrations of metformin for 24 h and then incubated with K562 cells for 4 h at 37°C. The expression of CFSE/PI (c, d) and CD56+ CD107a+ were then observed with flow cytometry (e, f). Perforin, granzyme B and IFN-γ expressions in NK-92 cells treated with metformin were determined using ELISA (g-i). NK-92 cells were treated with different concentrations of metformin for 24 h, and the expression of NKG2D, NKp30 and NKp44 was then observed using western blots (j). Data represent the mean ± SEM (n = 6). Data shown represent at least three independent experiments. *p < .05, **p < .01, ***p < .001 by two-tailed Student’s t-test (a, b, g, h, i), one-way ANOVA with the Dunnett’s test (d, f)
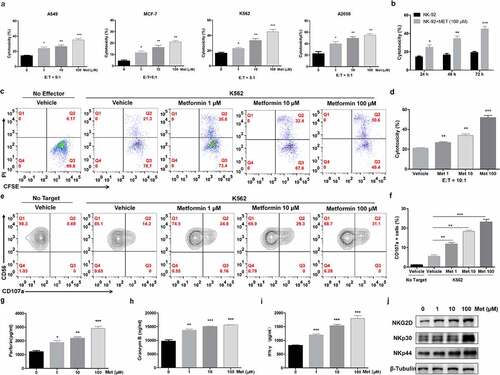
The effect of metformin on the cytotoxicity of NK-92 was further supported by flow cytometry analysis with CFSE/PI staining. Similar to the LDH analysis results, the target cell lysis ability of NK-92 cells was promoted by treatment with metformin in a dose-dependent manner (). We then determined whether the treatment of NK cells with metformin resulted in an increase in CD107a expression, an important membrane marker in the degranulation of NK cells for cytolytic activity. NK-92 cells were treated with metformin for 24 h, and the expression of CD107a was then detected by flow cytometry. As shown in , CD107a expression on NK-92 cells treated with 100 µM metformin increased 2.4-fold in comparison to that of the untreated control group. Next, we assessed the effect of metformin on NK cell cytokine production. As shown in , IFN-γ, granzyme B and perforin production by NK cells were significantly enhanced by treatment with metformin.
In human NK cells, activating receptors such as NKG2D and the natural cytotoxicity receptors NKp30 and NKp44 recognize ligands expressed on abnormal cells enhancing NK cell activity.Citation34 As shown in , the expression of NKG2D, NKp30 and NKp44 in the cells was increased by metformin. Collectively, these results indicate that metformin promotes the activation and natural cytotoxicity of NK cells.
3.2 Metformin enhances the cytolytic activity of primary NK cells.
We also investigated whether metformin increases the cytolytic activity of human peripheral blood NK cells (PBNK). After activation, PBMCs were treated with or without metformin. The effect of metformin on the cytotoxicity of primary NK cells was assessed by flow cytometry analysis with CFSE/PI staining or CD107a assays. As shown in , metformin enhanced the cytolytic activity of PBMCs and increased CD107a expression in the CD3−CD56+CD16+ NK cell population, indicating that metformin promotes cytolytic activity of human primary NK cells. It has been reported that in vitro activated T cell mediated metformin-treated cancer cell killing.Citation29 Conversely, we assessed the T cells changes post direct metformin treatment by flow cytometry. We found metformin did not affect the proportions of CD3+CD4+ and CD3+CD8+ T cells in PBMCs (), and metformin did not affect the expression of CD107a in CD8+ T cells ).
Figure 2. Metformin enhances the cytolytic activity of primary NK cells from healthy donors. PBMCs from healthy donors were activated and pretreated with metformin for 20 h, then mixed with K562 cells. After 4 h of incubation at 37°C, the expression of CFSE/PI (a), CD3−CD56+CD16+/CD107a (b), CD4+ and CD8+ T cells (c) and CD8+/CD107a (d) in PBMCs from healthy donors were then observed with flow cytometry. Data represent the mean ± SEM (n = 6). Data shown represent at least three independent experiments. *p < .05, **p < .01, ***p < .001 by one-way ANOVA with the Dunnett’s test
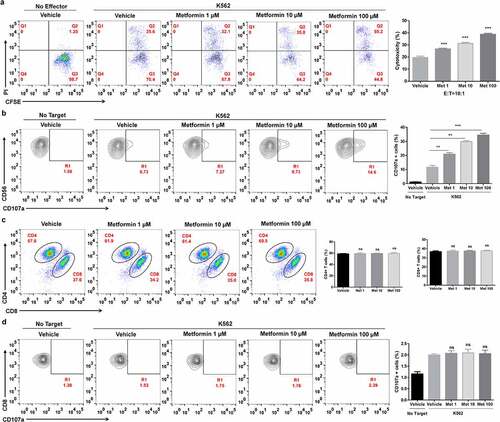
3.3 Metformin enhances the cytolytic activity of cancer patient ascites NK cells.
The ability of metformin to enhance ascites NK cells from the pleural fluid of lung cancer patients (ASC NK) was further evaluated. Cells were obtained from the peritoneal cavity of a cohort of lung cancer patients immediately prior to undergoing cytoreductive surgery. Fresh ASC NKs were incubated overnight with metformin and then placed in culture with the K562 cell line. As shown in , the cytolysis activity of the ASC NKs in the CD3−CD56+CD16+ NK cell population was remarkably upregulated with the increase of metformin. Accordingly, the CD107a expression was obviously induced by metformin ()). Likewise, metformin did not affect the proportions of CD4+ and CD8+ T cells in the CD3+ T cell population and the cytotoxicity of CD8+ T cells in peritoneal cavity of lung cancer patients (). These experiments highlight the capability of metformin to enhance both immune functions of PBNKs and ASC NKs.
Figure 3. Metformin enhances the cytolytic activity of ascites NK cells from cancer patients. Cells from the ascites of lung cancer patients were activated and pretreated with metformin for 20 h, then mixed with K562 cells. After 4 h of incubation at 37°C, the expression of CFSE/PI (a), CD3−CD56+CD16+ /CD107a (b), CD4+ and CD8+ T cells (c) and CD3+CD8+/CD107a (d) in the ascites of lung cancer patients were then observed with flow cytometry. Data represent the mean ± SEM (n = 6). Data shown represent at least three independent experiments. *p < .05, **p < .01, ***p < .001 by one-way ANOVA with the Dunnett’s test
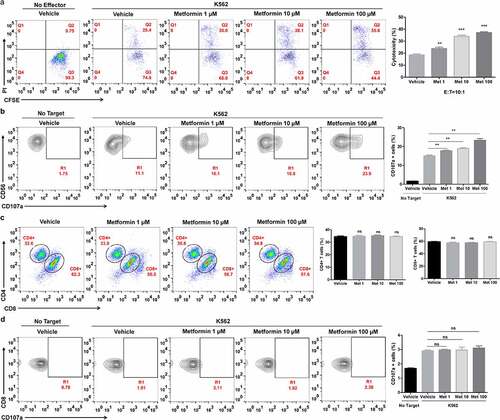
3.4 Metformin promotes NK cell immunity in a p38 MAPK-dependent manner at least partly.
To investigate the molecular mechanism by which metformin synergistically activates NK cells, we evaluated the changes of the JAK/STAT and AKT/mTOR pathways, which are involved in NK cell immune activation.Citation35,Citation36 Cells were harvested at 0, 1, 3, 6, 9 and 12 h after metformin treatment to investigate the JAK-STAT and AKT/mTOR pathway expression patterns over time. Metformin led to increases of the total and phosphorylated levels of JAK1/2/3 (), STAT5 (), mTOR and AKT (), whereas STAT1 and STAT3 were not altered under the same conditions. Metformin has been reported to exert its antitumor effects in an AMPK-dependent or AMPK-independent manner.Citation37 Metformin also has the ability to induce the activation of p38 MAPK, which plays an important role in controlling innate immunity.Citation38 Thus, we assessed whether NK cell activation by metformin could be mediated by AMPK or p38 MAPK. We found that metformin failed to enhance phospho-AMPK, but improved phospho-p38 in NK cells ). Furthermore, the AMPK inhibitor Compound C had no effect on the metformin-activated JAK1/STAT5 pathways (Fig. S2), while the p38 MAPK inhibitor Doramapimod obviously blocked the JAK1/STAT5 and mTOR/AKT signaling pathway ().
Figure 4. Metformin activates NK cells through the p38 MAPK-dependent pathway. The expression levels of the total and phosphorylated JAK1/2/3 (a, b), STAT1/3/5 (c, d) and mTOR/AKT (e, f) in metformin-treated cells were compared with those in untreated metformin-treated cells by western blotting. The amounts of NKG2D, phosphorylated p38 MAPK, and AMPK in metformin-treated cells were compared with those in untreated metformin-treated cells (g, h). NK-92 cells were pretreated with MAPK inhibitor Doramapimod (i, j, k), treated with metformin, and then analyzed by western blot. NK-92 cells were pretreated with si- p38 MAPK, and then treated with metformin. The expression levels of phosphorylated p38 MAPK, JAK1 and STAT5 were analyzed by western blot (l, m, n). Data represent the mean ± SEM (n = 6). Data shown represent at least three independent experiments. *p < .05, **p < .01, ***p < .001. The data were analyzed using the two-tailed Student’s t-test
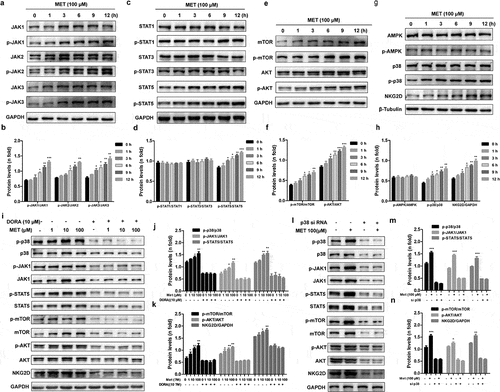
We then treated NK-92 cells with si-p38 MAPK for 48 h to knock down p38 MAPK, and then administered metformin for another 24 h. Metformin-induced p38 MAPK upregulation was significantly decreased. We also found that the JAK1/STAT5 and mTOR/AKT signaling pathway was no longer activated (, m and n). However, when we treated NK-92 cells with si-AMPK to knock down AMPK, we found si AMPK did not affect the metformin-activated JAK1/STAT5 pathways (Fig. S2). Taken together, these results indicate that metformin promotes the cytolytic activity of NK cells in a p38 MAPK-dependent manner at least partly.
3.5 Metformin improves NK cell-mediated lymphoma clearance in vivo.
The ability of NK cells to kill abnormal cells lacking MHC class-I molecules in vivo is well established.Citation39 To determine whether metformin enhances NK cell function in vivo, we used a syngeneic tumor clearance model as described. In this model, NK cells preferentially kill MHC class-I-deficient RMA-s cells rather than their parent RMA.Citation40 To facilitate their identification, RMA and RMA-s cells were labeled with different concentrations of carboxyfluorescein succinimidyl ester (CFSE), and then equal numbers of cells were co-injected intraperitoneally (i.p.) into the mice for 12 h (). To determine the elimination of metformin on the RMA-s cells was mainly mediated by NK cells, we first used an antibody specific to the ganglioside asialo-GM1 to deplete NK cell. The efficacy of anti-asialo-GM1-mediated depletion of NK cells was confirmed in the splenocytes of mice stained to detect CD3−NKp46+ NK cells (, c). We found pretreatment with 100 mg/kg metformin (i.p.) prior to NK cell-sensitive RMA-s cell injection significantly enhanced the peritoneal clearance of RMA-s cells, which was diminished after anti-asialo-GM1-mediated depletion of NK cells (, e). Furthermore, the increase of RMA-s cell clearance with metformin treatment was dose-dependent at 25–100 mg/kg (, g). These results suggest that metformin improves cancer immune surveillance dependent on NK cell in vivo .
Figure 5. Metformin enhances NK cell-mediated lymphoma clearance in vivo. CFSE-stained RMA (low CFSE concentration) and RMA-s cells (high CFSE concentration) were mixed at a ratio of 1:1 and then injected i.p. into C57BL/6 mice, and the rejection of RMAs relative to the peritoneal cavity was measured using flow cytometry (a). C57BL/6 mice were treated with i.p. injection of anti-asialo-GM1 (50 µL) or control serum for three consecutive days before an i.p. injection of cells. After 12 h post challenge of RMA:RMA-s cells, mice were sacrificed and the frequencies of NK cells (CD3−NKp46+) in the spleen of each group are shown as representative flow cytometry profile (b) and summary graph of statistical bar charts (c). C57BL/6 mice received either metformin (100 mg/kg; i.p.) or vehicle with or without anti-asialo-GM1 (50 µL) for 3 consecutive days before cell injection. Representative result (d) and a summary graph of statistical bar charts (e) showing the percentage of rejected RMA-s cells. C57BL/6 mice received increasing doses of metformin (i.p.) for 3 consecutive days before cells injection. Representative result (f) and summary graph of statistical bar charts (g) showing the percentage of rejected RMA-s cells. Data represent the mean ± SEM of 4 mice per group. Data shown represent at least three independent experiments. *p < .05, **p < .01, ***p < .001. The data were analyzed using by two-tailed Student’s t-test (c, e), one-way ANOVA with the Dunnett’s test (g)
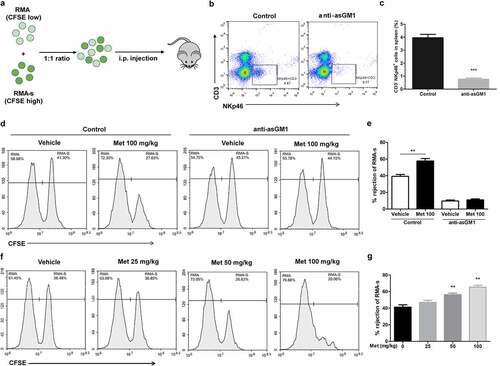
3.6 Metformin raises NK cell-mediated protection against pulmonary metastatic melanoma in vivo.
Given the important role of NK cells in inhibiting the growth of metastatic tumors in a mouse model,Citation41 we explored whether metformin inhibited the development of metastatic tumors in a B16F10 mouse model. To this end, B16F10 melanoma cells were injected into mice intravenously (i.v.) and assessed the effect of metformin on pulmonary metastatic growth (). Metformin effectively prevented the lung colonization of systemically injected B16F10 cells, which was abrogated by anti-asialo-GM1 antibody injected into mice (, c). Flow-cytometry analyses revealed the frequencies of PanNK (CD3−NK1.1+) and NK cells expressing activated receptor (CD3−NKp46+) in spleen () were remarkably enhanced in metformin-treated mice. To assess NK cell cytotoxic function ex vivo in these tumor-bearing mice, spleenic NK cells were isolated from each group of mice and incubated with YAC-1 target cells for 4 h. Compared with the control group, the metformin significantly increased cytolytic activity of spleenic NK cells in a dose-dependent manner (). These results support that metformin mediated cancer immune surveillance against B16F10 cells is associated with enhancement of the cytotoxic potential of activated NK cells.
Figure 6. Metformin enhances NK cell-mediated protection against pulmonary metastatic melanoma in vivo. (a) C57BL/6 mice received an i.v. injection of 2 × 105 B16F10 cells and then received either metformin or vehicle daily. After 14 days of tumor implantation, the mice were euthanized, and the number of metastatic tumor colonies in the lungs was counted after fixation. Representative lung image (b) and a summary graph of statistical bar charts (c) showing pulmonary metastases of B16F10 cells. The frequencies of CD3−NK1.1+ cell (d, f) and the frequencies of CD3−NKp46+ cells (e, g) in the spleen of each group are shown as representative flow cytometry. Thereafter, mouse primary NK cells were incubated with YAC-1 target cells for 4 h (h). Data represent the mean ± SEM of 6 mice per group. Data shown represent at least three independent experiments. *p < .05, **p < .01, ***p < .001 by one-way ANOVA with the Dunnett’s test
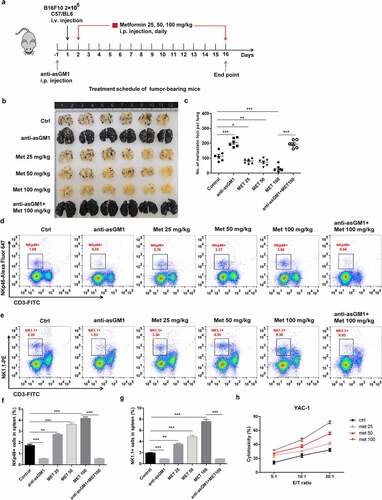
3.7 The combination of metformin with anti-PD-1 strengthens therapeutic efficacy against melanoma growth.
Next, we evaluated whether metformin could further increase checkpoint blockade-mediated antitumor immunity. Metformin treatment alone or in combination with PD-1 antibody αPD-1 (clone RPM1-14, BioXcell, 200 μg) was administered to B16F10 melanoma tumor-bearing mice (). As expected, metformin or αPD-1 alone obviously inhibited tumor growth compared to control, while a combination treatment of metformin and αPD-1 exhibited a significant delay in tumor growth compared to the αPD-1 treatment group (, c). The antitumor effect of combination therapy was dependent on NK cells. In B16-F10 tumor-bearing mice depleted of NK cells using an anti-Asialo-GM1 antibody, the therapeutic efficacy of metformin plus αPD-1 was significantly reduced (, e).
Figure 7. The combination of metformin with anti-PD-1 enhances the therapeutic efficacy against established B16F10 melanoma tumors. The experimental scheme of metformin therapy combined with PD-1 blockade (a). Measurement of tumor volume (b, d) and weight in each group (c, e). The frequencies of CD3−NK1.1+ cells (f), the frequencies of CD3−NKp46+ cells (g) and the frequencies of CD3+CD8+ cells (h) in the spleen of each group are shown as representative flow cytometry. Data represent the mean ± SEM of 6 mice per group. Each symbol represents an individual mouse. Data shown represent at least three independent experiments. *p < .05, **p < .01, ***p < .001. The data were analyzed using the Two-way ANOVA (b and d) or two-tailed Student’s t-test (the rest)
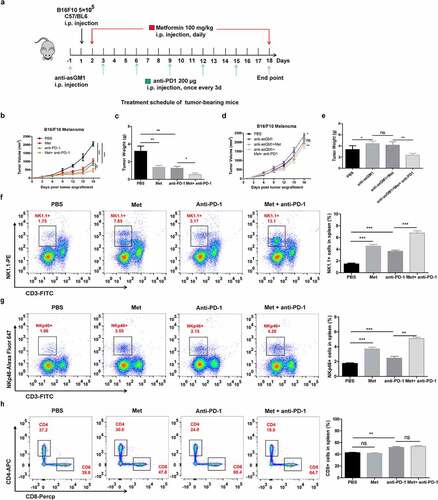
We then investigated the impact of metformin and αPD-1 alone or combination treatment on both NK cells and T cells. In terms of the ratio of NK cells in circulation, mice treated with metformin or αPD-1 alone had significantly higher ratio of CD3− NK1.1+ cells in the spleen compared to the control-treated mice, while the ratio of CD3− NK1.1+ cells in the combination treatment of metformin and αPD-1 was 3.9 folds higher than that in the αPD-1 treatment group (). We observed similar results when we quantified the ratio of CD3− NKp46+ cells in the spleen (). As respected, we found that CD3− CD8+ T cells in the spleen were significantly increased in the αPD-1 treatment group but not in the metformin group. The combination therapy was not more effective than in the αPD-1 treatment group ().
We further detected NK cells and T cells infiltration in tumor tissue by immunohistochemical staining analysis. As shown in , b, the presence of infiltrating NK cells in metformin- or αPD-1-treated groups was 3.9 and 2.6 folds more than the control group, and the presence of infiltrating NK cells in metformin and αPD-1 combination treated group was 6.4 folds compared to αPD-1 treatment. Similar results were obtained when we quantified the frequency of granzyme B in the tumor (, d). Additionally, metformin treatment led to a 1.7 folds increase in the quantity of CD8+ T cells in the tumor tissue compared with the vehicle treatment, and αPD-1 treatment led to a 3.9 folds increase compared with the vehicle treatment. Metformin and αPD-1 combination treatment led to a 6.5 folds increase in the quantity of CD8+ T cells in the tumor tissue compared with the αPD-1 alone (, f). Taken together, metformin in combination with αPD-1 significantly up-regulated the number of activated NK cells as well as the number of CD8+ T cells in the tumor. These results indicate that metformin-induced NK cell activation strengthens the therapy efficacy of PD-1 blockade. It was noted in tumor tissue, metformin distinctly increased the levels of phosphorylated p38 MAPK in control mice than in anti-asialo-GM1 antibody treated mice, supporting (Fig. S3), further supporting metformin promotes NK cell immunity in a p38 MAPK-dependent manner.
Figure 8. The combination of metformin with anti-PD-1 promotes NK cells and T cells recruitment to the tumor microenvironment. The summarized data and representative results of NK1.1 (a) (b), Granzyme B (c) (d) and CD8 (e) (f) staining of tumors by IHC. All images were obtained at ×200 and ×400 magnification. Data represent the mean ± SEM of 6 mice per group. Data shown represent at least three independent experiments. *p < .05, **p < .01, ***p < .001. The data were analyzed using the two-tailed Student’s t-test
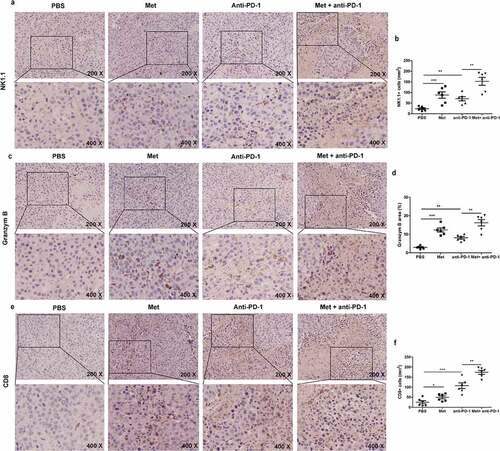
4. Discussion
NK cells are major effectors of cancer immunosurveillance owing to their intrinsic capacity to rapidly kill cells undergoing carcinogenesis.Citation42 The present study demonstrated the anticancer effects of metformin and revealed that metformin enhanced NK cell-mediated cytotoxicity in vitro and in vivo. Previous studies demonstrated that metformin showed antitumor effects by directly acting on tumor cells at higher doses (at least 10 mM).Citation43 Here, in this study, we present evidences to support metformin activates the cytolytic activity of NK cells directly with a lower dose of metformin at 1–100 μM which did not cause significant tumor cell growth inhibition. The improvement of cytotoxicity by metformin was not only observed in NK-92 cell line, but also NK cells from healthy peripheral blood and cancer patient ascites. Moreover, the anticancer potential of metformin was supported by its significant NK cell-dependent inhibition of tumor burden in different in vivo models of syngeneic lymphoma clearance and pulmonary metastatic melanoma. In addition, metformin did not induce any toxic or lethal effects in the in vivo study. This suggests that metformin has potential as a promising candidate for clinical application to enhance antitumor immunity of NK cells.
Several studies have shown that PD-1 expression on NK cells results in decreased cytokine production, degranulation, and cytotoxicity, indicating that these coinhibitory molecules act not only on T cells but also on NK cells.Citation44,Citation45 In our B16F10 tumor-bearing mice, metformin in combination with αPD-1 treatment further increased the effector function of NK cells, leading to the increased regression of established tumors. The number of tumor-infiltrating NK cells and the expression of the major component of cytotoxic granules, granzyme B, were significantly upregulated by metformin and αPD-1, and the combination treatment was more effective than αPD-1 alone. We noted that the ratio of CD8+ T cells in splenic lymphocytes was not increased and activated after metformin treatment, which is consistent to results in CD8+ T cells from PBMCs of normal people and the pleural fluid of lung cancer patients, while the frequency of infiltrating CD8+ T cells in tumors was significantly increased. It has been demonstrated that metformin treatment shifts the tumor immune microenvironment (TIME) in human esophageal cancer from a protumoral state toward a more antitumoral state,Citation46 and metformin has also been shown to directly promote antigen-specific naïve CD8+ T cells migration into tumors and exert antitumor immunity.Citation27 However, to our knowledge, there is no report that metformin directly induces activation and promotes cytotoxic effect of CD8+ T cells. Furthermore, Activated NK cells can promote DC maturation and CD8+ T-cell priming,Citation47,Citation48 thus, we speculate that the directly activation of NK cells at the tumor site induced by metformin may reprogram the TIME and recruit CD8+ T cells from peripheral blood to tumor tissue. This kind of crosstalk between NK cells and T cells may be important for fully understanding the mechanism of metformin in activating antitumor immunity, which is necessary to study in the future.
It appears that metformin, at least in part, has an antitumor effect through activation of the AMPK signaling pathway. Cha et al. have shown that metformin reduces the stability and membrane localization of PD-L1 of cancer cells in an AMPK-dependent manner and thereby contributes to the enhancement of CTL against cancer cells (5 mM).Citation29 In our study, we confirmed that the AMPK pathway could not be activated by low-dose metformin in NK cells. Metformin has the ability to induce the activation of p38 MAPK,Citation38 which is involved in triggering the polarization and secretion of cytolytic granules in NK cells.Citation42 Our investigations indicate that metformin significantly induces activation of p38 MAPK in vitro and in vivo, and the improvement of the JAK1/2/3/STAT5 and AKT/mTOR pathways was dependent on activation of p38 MAPK in NK cells. This pathway provides a new opportunity to promote NK cell function in antitumor immunotherapy.
In summary, we demonstrated that metformin treatment activated NK cells and thereby enhanced antitumor immunity in vitro and in vivo. Additionally, we showed that metformin activated innate immunity in NK cells via JAK1/2/3/STAT5 and AKT/mTOR pathway activation in a p38 MAPK-dependent manner at least partly. Our findings support the notion that metformin enhances host antitumor immunity and is involved in promoting the therapeutic effects of checkpoint cancer immunotherapies. However, further investigation in future clinical studies is required to confirm these findings. Also, our research may pave a new avenue to understand potent anticancer immunity effect of metformin.
Supplemental Material
Download ()Disclosure statement
The authors declare that they have no competing interests.
Supplementary material
Supplemental data for this article can be accessed on the publisher’s website
Additional information
Funding
References
- Hodgins JJ, Khan ST, Park MM, Auer RC, Killers AM. 2.0: NK cell therapies at the forefront of cancer control. J Clin Invest. 2019;129(9):3499–14. doi:10.1172/JCI129338.
- Shimasaki N, Jain A, Campana D. NK cells for cancer immunotherapy. Nat Rev Drug Discov. 2020;19(3):200–218. doi:10.1038/s41573-019-0052-1.
- Souza-Fonseca-Guimaraes F, Cursons J, Huntington ND. The emergence of natural killer cells as a major target in cancer immunotherapy. Trends Immunol. 2019;40(2):142–158. doi:10.1016/j.it.2018.12.003.
- Imai K, Matsuyama S, Miyake S, Suga K, Nakachi K. Natural cytotoxic activity of peripheral-blood lymphocytes and cancer incidence: an 11-year follow-up study of a general population. Lancet. 2000;356(9244):1795–1799. doi:10.1016/S0140-6736(00)03231-1.
- Lee H, Da Silva IP, Palendira U, Scolyer RA, Long GV, Wilmott JS. Targeting NK Cells to Enhance Melanoma Response to Immunotherapies. Cancers (Basel). 2021;13(6):1363. doi:10.3390/cancers13061363.
- Pierson BA, Miller JS. CD56+bright and CD56+dim natural killer cells in patients with chronic myelogenous leukemia progressively decrease in number, respond less to stimuli that recruit clonogenic natural killer cells, and exhibit decreased proliferation on a per cell basis. Blood. 1996;88(6):2279–2287. doi:10.1182/blood.V88.6.2279.bloodjournal8862279.
- Cichocki F, Grzywacz B, Miller JS. Human NK cell development: one road or many? Front Immunol. 2019;10:2078. doi:10.3389/fimmu.2019.02078.
- Myers JA, Miller JS. Exploring the NK cell platform for cancer immunotherapy. Nat Rev Clin Oncol. 2021;18(2):85–100. doi:10.1038/s41571-020-0426-7.
- Carotta S. Targeting NK cells for anticancer immunotherapy: clinical and preclinical approaches. Front Immunol. 2016;7:152. doi:10.3389/fimmu.2016.00152.
- Morvan MG, Lanier LL. NK cells and cancer: you can teach innate cells new tricks. Nat Rev Cancer. 2016;16(1):7–19. doi:10.1038/nrc.2015.5.
- Talmadge JE, Meyers KM, Prieur DJ, Starkey JR. Role of NK cells in tumour growth and metastasis in beige mice. Nature. 1980;284(5757):622–624. doi:10.1038/284622a0.
- Hanna N, Burton RC. Definitive evidence that natural killer (NK) cells inhibit experimental tumor metastases in vivo. J Immunol. 1981;127:1754–1758.
- Coca S, Perez-Piqueras J, Martinez D, Colmenarejo A, Saez MA, Vallejo C, Martos JA, Moreno M. The prognostic significance of intratumoral natural killer cells in patients with colorectal carcinoma. Cancer. 1997;79(12):2320–2328. doi:10.1002/(SICI)1097-0142(19970615)79:12<2320::AID-CNCR5>3.0.CO;2-P.
- Takeuchi H, Maehara Y, Tokunaga E, Koga T, Kakeji Y, Sugimachi K. Prognostic significance of natural killer cell activity in patients with gastric carcinoma: a multivariate analysis. Am J Gastroenterol. 2001;96(2):574–578. doi:10.1111/j.1572-0241.2001.03535.x.
- Suen WC, Lee WY, Leung KT, Pan XH, Li G. Natural killer cell-based cancer immunotherapy: a review on 10 years completed clinical trials. Cancer Invest. 2018;36(8):431–457. doi:10.1080/07357907.2018.1515315.
- Grossenbacher SK, Aguilar EG, Murphy WJ. Leveraging natural killer cells for cancer immunotherapy. Immunotherapy. 2017;9(6):487–497. doi:10.2217/imt-2017-0013.
- Guillerey C, Huntington ND, Smyth MJ. Targeting natural killer cells in cancer immunotherapy. Nat Immunol. 2016;17(9):1025–1036. doi:10.1038/ni.3518.
- Brehm C, Huenecke S, Esser R, Kloess S, Quaiser A, Betz S, Zimmermann O, Soerensen J, Passweg JR, Klingebiel T, et al. Interleukin-2-stimulated natural killer cells are less susceptible to mycophenolate mofetil than non-activated NK cells: possible consequences for immunotherapy. Cancer Immunol Immunother. 2014;63(8):821–833. doi:10.1007/s00262-014-1556-5.
- Conlon KC, Lugli E, Welles HC, Rosenberg SA, Fojo AT, Morris JC, Fleisher TA, Dubois SP, Perera LP, Stewart DM, et al. Redistribution, hyperproliferation, activation of natural killer cells and CD8 T cells, and cytokine production during first-in-human clinical trial of recombinant human interleukin-15 in patients with cancer. J Clin Oncol. 2015;33(1):74–82. doi:10.1200/JCO.2014.57.3329.
- Morales DR, Morris AD. Metformin in cancer treatment and prevention. Annu Rev Med. 2015;66(1):17–29. doi:10.1146/annurev-med-062613-093128.
- Evans JM, Donnelly LA, Emslie-Smith AM, Alessi DR, Morris AD. Metformin and reduced risk of cancer in diabetic patients. BMJ. 2005;330(7503):1304–1305. doi:10.1136/bmj.38415.708634.F7.
- Quinn BJ, Kitagawa H, Memmott RM, Gills JJ, Dennis PA. Repositioning metformin for cancer prevention and treatment. Trends Endocrinol Metab. 2013;24(9):469–480. doi:10.1016/j.tem.2013.05.004.
- Curry JM, Johnson J, Mollaee M, Tassone P, Amin D, Knops A, Whitaker-Menezes D, Mahoney MG, South A, Rodeck U, et al. Metformin Clinical Trial in HPV+ and HPV− Head and Neck Squamous Cell Carcinoma: impact on Cancer Cell Apoptosis and Immune Infiltrate. Front Oncol. 2018;8:436. doi:10.3389/fonc.2018.00436.
- Petrera M, Paleari L, Clavarezza M, Puntoni M, Caviglia S, Briata IM, Oppezzi M, Mislej EM, Stabuc B, Gnant M, et al. The ASAMET trial: a randomized, phase II, double-blind, placebo-controlled, multicenter, 2x2 factorial biomarker study of tertiary prevention with low-dose aspirin and metformin in stage I-III colorectal cancer patients. BMC Cancer. 2018;18(1):1210. doi:10.1186/s12885-018-5126-7.
- Higurashi T, Hosono K, Takahashi H, Komiya Y, Umezawa S, Sakai E, Uchiyama T, Taniguchi L, Hata Y, Uchiyama S, et al. Metformin for chemoprevention of metachronous colorectal adenoma or polyps in post-polypectomy patients without diabetes: a multicentre double-blind, placebo-controlled, randomised phase 3 trial. Lancet Oncol. 2016;17(4):475–483. doi:10.1016/S1470-2045(15)00565-3.
- Martin-Castillo B, Pernas S, Dorca J, Alvarez I, Martinez S, Perez-Garcia JM, Batista-López N, Rodríguez-Sánchez CA, Amillano K, Domínguez S, et al. A phase 2 trial of neoadjuvant metformin in combination with trastuzumab and chemotherapy in women with early HER2-positive breast cancer: the METTEN study. Oncotarget. 2018;9(86):35687–35704. doi:10.18632/oncotarget.26286.
- Eikawa S, Nishida M, Mizukami S, Yamazaki C, Nakayama E, Udono H. Immune-mediated antitumor effect by type 2 diabetes drug, metformin. Proc Natl Acad Sci U S A. 2015;112:1809–1814.
- Zhang Z, Li F, Tian Y, Cao L, Gao Q, Zhang C, Zhang K, Shen C, Ping Y, Maimela NR, et al. Metformin Enhances the Antitumor Activity of CD8+T Lymphocytes via the AMPK–miR-107–Eomes–PD-1 Pathway. J Immunol. 2020;204(9):2575–2588. doi:10.4049/jimmunol.1901213.
- Cha JH, Yang WH, Xia W, Wei Y, Chan LC, Lim SO, Li C-W, Kim T, Chang -S-S, Lee -H-H, et al. Metformin promotes antitumor immunity via endoplasmic-reticulum-associated degradation of PD-L1. Mol Cell. 2018;71(4):606–620. doi:10.1016/j.molcel.2018.07.030.
- Uehara T, Eikawa S, Nishida M, Kunisada Y, Yoshida A, Fujiwara T, Kunisada T, Ozaki T, Udono H. Metformin induces CD11b+-cell-mediated growth inhibition of an osteosarcoma: implications for metabolic reprogramming of myeloid cells and anti-tumor effects. Int Immunol. 2019;31(4):187–198. doi:10.1093/intimm/dxy079.
- Li L, Wang L, Li J, Fan Z, Yang L, Zhang Z, Zhang C, Yue D, Qin G, Zhang T, et al. Metformin-induced reduction of CD39 and CD73 blocks myeloid-derived suppressor cell activity in patients with ovarian cancer. Cancer Res. 2018;78(7):1779–1791. doi:10.1158/0008-5472.CAN-17-2460.
- Kim K, Yang W-H, Jung Y-S, Cha J-H. A new aspect of an old friend: the beneficial effect of metformin on anti-tumor immunity. BMB Rep. 2020;53(10):512–520. doi:10.5483/BMBRep.2020.53.10.149.
- Zhen L, Jiacheng B, Wan X. Artemisinin sensitizes tumor cells to NK cell-mediated cytolysis. Biochem Biophys Res Commun. 2020;524(2):418–423. doi:10.1016/j.bbrc.2020.01.094.
- Smyth MJ, Hayakawa Y, Takeda K, Yagita H. New aspects of natural-killer-cell surveillance and therapy of cancer. Nat Rev Cancer. 2002;2(11):850–861. doi:10.1038/nrc928.
- Gotthardt D, Trifinopoulos J, Sexl V, Putz EM. JAK/STAT Cytokine Signaling at the Crossroad of NK Cell Development and Maturation. Front Immunol. 2019;10:2590. doi:10.3389/fimmu.2019.02590.
- Ali AK, Nandagopal N, Lee SH. IL-15-PI3K-AKT-mTOR: a critical pathway in the life journey of natural killer cells. Front Immunol. 2015;6:355. doi:10.3389/fimmu.2015.00355.
- Ruixia M, Bin Y, Riker AI, Yaguang X. Metformin and cancer immunity. Acta Pharmacol Sin. 2020;41(11):1403–1409. doi:10.1038/s41401-020-00508-0.
- Xiao Y, Liu F, Sanhua L, Jiang N, Changyan Y, Zhu X, Qin Y, Hui J, Liangjie M, Changwei S, et al. Metformin promotes innate immunity through a conserved PMK-1/p38 MAPK pathway. Virulence. 2019. doi:10.1080/21505594.2019.1706305.
- Karre K, Ljunggren HG, Piontek G, Kiessling R. Selective rejection of H-2-deficient lymphoma variants suggests alternative immune defence strategy. Nature. 1986;319(6055):675–678. doi:10.1038/319675a0.
- Gascoyne DM, Long E, Veiga-Fernandes H, De Boer J, Williams O, Seddon B, Coles M, Kioussis D, Brady HJM. The basic leucine zipper transcription factor E4BP4 is essential for natural killer cell development. Nat Immunol. 2009;10(10):1118–1124. doi:10.1038/ni.1787.
- Merzoug LB, Marie S, Satoh-Takayama N, Lesjean S, Albanesi M, Luche H, Fehling HJ, Santo JPD, Vosshenrich CAJ. Conditional ablation of NKp46+cells using a novel Ncr1greenCre mouse strain: NK cells are essential for protection against pulmonary B16 metastases. Eur J Immunol. 2014;44(11):3380–3391. doi:10.1002/eji.201444643.
- Ochayon DE, Ali A, Alarcon PC, Krishnamurthy D, Kottyan LC, Borchers MT. IL-33 promotes type 1 cytokine expression via p38 MAPK in human NK cells. J Leukoc Biol. 2020;107(4):663-671.
- Sciannimanico S, Grimaldi F, Vescini F, De Pergola G, Iacoviello M, Licchelli B, Guastamacchia E, Giagulli VA, Triggiani V. Metformin: up to Date. Endocr Metab Immune Disord Drug Targets. 2020;20(2):172–181. doi:10.2174/1871530319666190507125847.
- Pesce S, Greppi M, Tabellini G, Rampinelli F, Parolini S, Olive D, Moretta L, Morreta A, Marcenaro E. Identification of a subset of human NK cells expressing high levels of PD-1 receptor: a phenotypic and functional characterization. J Allergy Clin Immunol. 2017;139(1):335–346. doi:10.1016/j.jaci.2016.04.025.
- Benson DM, Bakan CE, Mishra A, Hofmeister CC, Efebera Y, Becknell B, Baiocchi RA, Zhang J, Yu J, Smith MK. The PD-1/PD-L1 axis modulates the natural killer cell versus multiple myeloma effect: a therapeutic target for CT-011, a novel monoclonal anti– PD-1 antibody. Blood. 2010;116(13):2286–2294. doi:10.1182/blood-2010-02-271874.
- Wang SH, Lin YS, Xiong X, Wang L, Guo Y, Chen YP. Low-dose metformin reprograms the tumor immune microenvironment in human esophageal cancer: results of a phase II clinical trial. Clin Cancer Res. 2020;26(18):4921–4932. doi:10.1158/1078-0432.CCR-20-0113.
- Srivastava RM, Lee SC, Pedro A, Filho A, Lord CA, Jie H-B, Davidson HC, Gibson SP, Gooding WE, Ferrone S, et al. Cetuximab-activated natural killer and dendritic cells collaborate to trigger tumor antigen-specific T-cell immunity in head and neck cancer patients. Clin Cancer Res. 2013;19(7):1858–1872. doi:10.1158/1078-0432.CCR-12-2426.
- Bald T, Krummel MF, Smyth MJ, Barry KC. The NK cell-cancer cycle: advances and new challenges in NK cell-based immunotherapies. Nat Immunol. 2020;21(8):835–847. doi:10.1038/s41590-020-0728-z.