ABSTRACT
We evaluated the immunological responses of lymph-node involved (stage III) melanoma patients to adjuvant dendritic cell vaccination with subsets of naturally occurring dendritic cells (nDCs). Fifteen patients with completely resected stage III melanoma were randomized to receive adjuvant dendritic cell vaccination with CD1c+ myeloid dendritic cells (cDC2s), plasmacytoid dendritic cells (pDCs) or the combination. Immunological response was the primary endpoint and secondary endpoints included safety and survival. In 80% of the patients, antigen-specific CD8+ T cells were detected in skin test-derived T cells and in 55% of patients, antigen-specific CD8+ T cells were detectable in peripheral blood. Functional interferon-γ-producing T cells were found in the skin test of 64% of the patients. Production of nDC vaccines meeting release criteria was feasible for all patients. Vaccination only induced grade 1–2 adverse events, mainly consisting of fatigue. In conclusion, adjuvant dendritic cell vaccination with cDC2s and/or pDCs is feasible, safe and induced immunological responses in the majority of stage III melanoma patients.
KEYWORDS:
Introduction
As dendritic cells (DCs) are the most potent antigen-presenting cells, presenting antigens to naive T cells, they play a pivotal role in the induction of adaptive immune responses against tumors.Citation1 For DC vaccination of cancer patients, autologous DCs are matured and loaded with the relevant tumor antigens ex vivo and are subsequently administered to the patient to induce tumor-specific T-cell responses in vivo.Citation2 Because T cell activation is highly antigen-specific, the toxicity profile of DC vaccination is mild.Citation3
Recent major breakthroughs in immunotherapy in cancer patients mainly consist of clinical benefit from immune checkpoint inhibitors (ICI). Unfortunately, and in contrast to DC vaccination, these drugs can give rise to serious immune-related toxicity due to the enhancement of non-tumor-specific immune responses against healthy cells.Citation4 To date, survival benefit with DC vaccination has not been established. However, DC vaccination induced functional tumor-specific T-cell responses and long-lasting clinical responses.Citation3 Together with the current knowledge that immunotherapy is able to induce long-term survival benefit and the favorable toxicity profile of DC vaccination, optimization of DC vaccination is an important focus of cancer research.
Until recently, most studies with DC-based immunotherapy were performed with autologous DCs ex vivo differentiated from monocytes or CD34+ progenitors. However, the potency of these so-called monocyte-derived DCs (moDCs) may be hampered by their extensive culture period of 5–9 days with cytokines such as granulocyte-macrophage colony-stimulating factor (GM-CSF) and interleukin (IL)-4 that is needed to differentiate the cells into DCs. Especially, IL-4 potentially reduces the migration capacity of DCs.Citation5–7 A few years ago, direct isolation of the scarce naturally circulating DCs (nDCs) from blood became possible, thereby omitting this intensive culture period used for the production of moDCs.Citation8 After direct isolation, nDCs are prepared for vaccination by maturation and antigen loading within two to three days. The two major subsets of nDCs are myeloid DCs (also called ‘conventional’ or ‘classical’ DCs) and plasmacytoid DCs (pDCs). The major subset of myeloid DCs, cDC2, is characterized by CD11c and CD1c (BDCA-1). A minor population, cDC1, is CD11c and CD141 (BDCA-3) positive. PDCs express both CD303 (BDCA-2) and CD304 (BDCA-4).Citation9 At the start of this trial clinical-grade isolation of cDC2s and pDCs was feasible, isolation of cDC1s only recently became feasible. Vaccination with CD1c+ cDC2s or pDCs has been tested in clinical trials in metastatic (stage IV) melanoma and metastastic prostate cancer patients. At time of writing, results of three trials were published and showed that in stage IV melanoma patients and metastatic prostate cancer patients, vaccination with pDCs or CD1c+ cDC2s led to immunological responses and, in some patients, to long-term survival.Citation10–12
Multiple potential advances in the production of the nDC vaccines are incorporated in our trial. First, the mode of maturation for both DC subsets is optimized. DC maturation is crucial for proper T cell activation.Citation13–15 Especially, exposure of DCs to pathogen-associated molecular patterns, such as Toll-like receptor ligands, yields DCs that induce potent T helper 1 and cytotoxic T cell responses.Citation16–19 Conventional DC2s and pDCs express Toll-like receptors 7 and 8, respectively, which can be triggered by single-stranded RNA (ssRNA). We have shown that complexes of ssRNA stabilized with protamine (pR) can activate both cDC2s and pDCs into functional mature DCs, secreting IL-12 and interferon (IFN)α, respectively.Citation20 Second, we showed that a DC subpopulation expressing both CD1c and the monocytic marker CD14, attenuates the induction of T cell responses.Citation21 Therefore, in our trial, we depleted CD14+ cells prior to the positive selection of cDC2s. Third, we expanded the pool of antigens used to load the DCs. We added the cancer-testis antigens (CTA) MAGE-C2, MAGE-A3 and NY-ESO-1 to the previously used tumor-associated antigens (TAA) gp100 and tyrosinase. Both these CTA and TAA are known to be frequently expressed in melanoma tissue.Citation22,Citation23 Besides, antigens binding HLA types other than HLA-A2.1 were added, to enable induction of immunological responses in HLA-A2.1 negative patients. In addition to a broader panel of HLA-restricted peptides, we added peptide pools with overlapping peptides that cover the complete antigen sequence and bind multiple HLA types, both major histocompatibility complex (MHC) class I and II.
Besides the aforementioned changes that potentially improve cDC2 and pDC vaccinations compared to previous trials, we assessed the administration of cDC2s and pDCs simultaneously. When combined, cDC2s and pDCs might have a synergistic effect, as they possess a distinct phenotype, capacity in pathogen detection, and produce different cytokines.Citation24,Citation25 In a murine model, vaccination with combined cDC2s and pDCs was superior in reducing tumor size and increased survival compared to either one of the subsets alone.Citation26 Therefore, combining both subsets might further enhance the induction of an immunological and clinical response.
We investigated vaccination with these improved and combined cDC2s and pDCs in lymph-node involved (stage III) melanoma patients. Cutaneous melanoma is an aggressive form of skin cancer due to its metastatic potential. Compared to patients with stage IV melanoma, patients with completely resected stage III melanoma harbor less tumor burden, hence less tumor-induced immune suppressionCitation27 which might hamper response to DC vaccination. As such, DC vaccination might be more successful in stage III melanoma patients. This is endorsed by superior induction of antigen-specific T cells by DC vaccination in stage III compared to stage IV melanoma patients.Citation28,Citation29 In addition, a retrospective analysis of stage III melanoma patients receiving adjuvant moDC vaccination showed improved overall survival (OS) compared to their matched controls.Citation30 Stage III melanoma is treated with surgical resection with curative intent. Unfortunately, despite complete surgical resection, patients have a high risk of recurrence resulting in 5-year OS rates between 40 and 78%.Citation31 Therefore, effective adjuvant therapy for this group of patients is warranted. At time of trial enrollment, no adjuvant therapy significantly impacting survival was registered and for this reason, we included patients with completely resected stage III melanoma to receive adjuvant nDC therapy. In the meantime, after completion of the enrollment phase, several drugs were approved for use as an adjuvant therapy.Citation32–35
Here we present the results on immunological response, feasibility and safety in our randomized phase II trial including stage III melanoma patients receiving adjuvant DC vaccination with cDC2s, pDCs or the combination of both (combiDCs). This is the first clinical trial with combiDCs in melanoma patients.
Patients and methods
Clinical protocol
Fifteen patients with stage III cutaneous melanoma, according to the 7th edition of the American Joint Committee on Cancer (AJCC) staging system,Citation31 were included. Patients were randomly assigned on a 1:1:1 basis to receive cDC2s, pDCs, or combiDCs. The primary endpoint was immunological response to single and combined cDC2 and pDC vaccination. Safety, recurrence-free survival (RFS), OS and health-related quality of life assessment (HRQoL) were secondary objectives. This trial (NCT02574377) has been approved by the Central Committee on Research Involving Human Subjects and is in concordance with the Declaration of Helsinki as defined by the International Conference on Harmonization.
Eligible patients were 18–75 years of age with histologically documented stage III melanoma, completely removed including a radical lymph node dissection (RLND) within 12 weeks prior to the start of study (apheresis). Other key inclusion criteria were WHO performance score 0 or 1 and a normal serum lactate dehydrogenase. Exclusion criteria included autoimmune diseases, immunosuppressive conditions and any concurrent adjuvant therapy including radiotherapy. Due to the use of keyhole limpet hemocyanin (KLH), patients with a known allergy to shell fish were excluded. Informed consent was obtained from all participants.
Four weeks after an apheresis, the first vaccination of a cycle consisting of three biweekly vaccinations was administered (Supplementary ). This cycle of three vaccinations was repeated twice, with an interval of six months between cycles. Between 1 and 2 weeks after the third vaccination of each cycle, patients were assessed for their immunological response by skin tests. If patients did not reach the first immunological assessment they were replaced, since this was the primary endpoint of our trial. Patients were followed for up to 5 years with follow-up visits every 3 months during the first 2 years and every 6 months for the last 3 years. Assessment for recurrent disease was performed by medical history, physical examination, and when indicated, by imaging.
Figure 1. cDC2 and pDC isolation and vaccine preparation
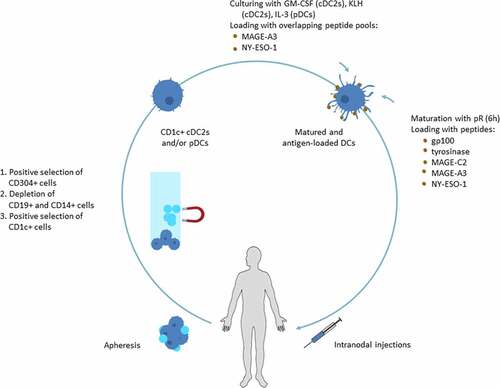
Adverse events were scored according to the Common Terminology Criteria for Adverse Events (CTCAE) version 4.0. Adverse events considered as possibly, probably or definitely related to the study drug according to the investigator were considered treatment-related.
Dendritic cell isolation and vaccine preparation
Patients were vaccinated with autologous cDC2s and/or pDCs loaded with tumor peptides and overlapping peptide pools (). Autologous mononuclear cells were harvested by apheresis. PDCs and cDC2s were isolated with the fully automated and enclosed immunomagnetic CliniMACS Prodigy® isolation system (Miltenyi Biotec, Bergisch Gladbach, Germany) with GMP-grade magnetic bead-coupled antibodies (Miltenyi Biotec) following the manufacturer’s guidelines. When patients were randomized for vaccination with both pDCs and cDC2s, pDCs were selected with anti-CD304 (BDCA-4) coupled beads first, followed by depletion of CD19+ and CD14+ cells and positive selection of CD1c+ cells. For the single cDC2 group, CD1c+ (BDCA-1+) cells were positively selected after depletion of CD19+ and CD14+ cells, and for the single pDC group only the pDC selection step was completed. cDC2s were cultured overnight at a concentration of 1.5 × 106 cells/ml with 800IU/ml recombinant human GM-CSF in TexMACS GMP medium (both Miltenyi Biotec) supplemented with 2% human serum (HS) (Sanquin, Amsterdam, the Netherlands) and KLH (Immucothel, Biosyn Arzneimittel GmbH) for immunomonitoring. PDCs were cultured overnight at a concentration of 1.5 × 106 cells/ml with 10 ng/ml recombinant human IL-3 in TexMACS GMP medium (both Miltenyi Biotec) supplemented with 2% pooled HS (Sanquin). Cells used for the delayed-type hypersensitivity (DTH) skin test were cultured without KLH. During overnight culturing MACS® GMP-grade PepTivators®, overlapping peptide pools of the CTA MAGE-A3 and NY-ESO-1 (Miltenyi Biotec) covering the sequence of the entire antigen, were added. After overnight culture, the DCs were matured with 10 µl/ml premixed protamine HCL (Meda Pharma, Amstelveen, the Netherlands; 10 µg)/mRNA (Universitätsklinikum Erlangen, Erlangen, Germany; 5 µg) (protamine/mRNA (pR)) for 6 hours. After 3 hours of maturation, viability and phenotyping was assessed and a mix of fourteen peptides of TAA gp100 and tyrosinase and CTA MAGE-C2, MAGE-A3 and NY-ESO-1 (all Leiden University Medical Center, Leiden, the Netherlands) (Supplementary Table 1) was added for the last 3 hours of maturation. DCs used for DTH skin injections were either loaded with peptides or overlapping peptide pools. The procedure had to give rise to mature cDC2s and pDCs meeting the following release criteria after 6 hours of maturation: >50% viability and >50% CD83 (cDC2) and/or >50% CD80 (pDC) expression. In addition, purity, assessed as in process control directly after CliniMACS Prodigy isolation, had to be >50% CD1c+CD20− (cDC2) and/or >50% BDCA2+ CD123+(pDC). One vaccine consisted of 2–5 × 106 cDC2s and/or 1–3 × 106 pDCs. Cells were cryopreserved in TexMACS medium containing 10% dimethyl sulfoxide (DMSO; WAK chemie Medical GmbH, Steinbach, Germany) and 40% Albuman (Sanquin) and were thawed on the day of administration. For combined pDC and cDC2 vaccines, both subsets were pooled in one syringe after thawing. Vaccines were checked for sterility prior to clinical application (Eurofins Bactimm, Nijmegen, the Netherlands). An experienced radiologist administered the vaccines intranodally in a clinically tumor-free lymph node under ultrasound guidance. If necessary, apheresis was repeated prior to a subsequent cycle to produce a sufficient number of vaccines.
Tumor antigen-specific and functional T cells
DTH skin tests were performed between 1 and 2 weeks after the third vaccination of each cycle, as described previously.Citation36 Depending on randomization, activated cDC2s and/or pDCs (0.1–0.5 × 106 cells) were injected intradermally at the back of the patient. Two days after injection, skin punch biopsies (6 mm) were obtained from all injection sites to assess the T cell responses. The biopsy specimens were cut in half; one half was cryopreserved and the other half was cut and cultured for 2–5 weeks in IL-2 (100 IU/ml; Novartis, Basel, Switzerland). After culturing, the skin-test infiltrating lymphocytes (SKILs) were tested for the presence of CD8+ T cells specific for the different tumor antigens used. To analyze occurrence of antigen-specific T cells, SKILs of HLA-A2.1 positive patients were stained with CD8 and tetrameric MHC-peptide complexes (Sanquin) or dextrameric MHC-peptide complexes (Immudex, Copenhagen, Denmark) containing peptides of the relevant HLA type as described previously.Citation36 CD8+ T cells recognizing these MHC-peptide complexes are further referred to as antigen-specific CD8+ T cells. To control for background staining, samples were stained with tetrameric or dextrameric MHC complexes containing an irrelevant peptide. To test T cell functionality SKILs were cocultured with autologous peripheral blood mononuclear cells (PBMCs) pulsed with the different relevant peptides, overlapping peptide pools, carcinoembryonic antigen (CEA) (negative control) (Leiden University Medical Center), or no peptide (negative control). Production of IFNγ was measured in the supernatants by cytometric bead array according to the manufacturer’s instruction (eBioscience, Vienna, Austria or BD Biosciences, San Jose, CA) after 24 hours of coculture. In addition, PBMCs of HLA-A2.1, HLA-A1 or HLA-B35 positive patients were tested for the presence of antigen-specific CD8+ T cells by staining with tetrameric or dextrameric MHC-peptide complexes as described above.
Flow cytometry
Purity and phenotype of cDC2s and pDCs after immunomagnetic isolation were determined by flow cytometry with a FACSVerse® (BD biosciences) or MACS Quant® (Miltenyi Biotec). Purity was analyzed directly after CliniMACS Prodigy isolation. For this purpose, the following primary monoclonal antibodies (mAbs) and appropriate fluorescence minus one controls were used: anti-CD1c-Viobright FITC, anti-BDCA2-PE, anti-CD123-APC, anti-CD20-PE-Vio770, anti-CD45-APC-Vio770, anti-CD14-Viogreen, anti-FcεRI-BioBlue, anti-CD14-FITC, anti-CD15-PE, anti-CD56-APC and anti-CD3-BioBlue. The phenotype of cDC2s and pDCs after 3 hours of pR stimulation was analyzed using the following mAbs and appropriate isotype controls: anti-HLA-ABC-APC, anti-HLA-DR/DP/DQ-APC, anti-CCR7-APC, anti-CD80-APC, anti-CD83-APC and anti-CD86-APC (all Miltenyi Biotec). After 6 hours of pR stimulation cytokine production of cDC2s and pDCs was measured in the supernatant by cytometric bead array according to the manufacturer’s instruction (Miltenyi Biotec).
Proliferative response to KLH
Cellular responses against KLH were measured in a proliferation assay. PBMCs were isolated from blood samples after each vaccination. 1 × 105 PBMCs were plated per well of a 96-well tissue culture microplate either in the presence or absence of KLH. After 4 days of culture, 1 μCi/well of tritiated thymidine was added and incorporation of tritiated thymidine was measured in a beta-counter. A proliferation index (proliferation with KLH/proliferation without KLH) of >2 was considered positive.
Immunohistochemistry staining of antigen expression
Available tumor tissue of primary melanoma, lymph node metastasis and recurrent disease of the 15 patients was analyzed. To determine antigen expression, we stained for gp100, tyrosinase, NY-ESO-1, MAGE-C2 and MAGE-A. The different MAGE-A antigens are highly homologousCitation37 leading to cross-reactivity, that is, T cell receptors recognize multiple members of the MAGE-A antigen superfamily.Citation38 In addition, the epitopes of the peptides we used to load the DCs are found in different MAGE-A antigens and T cells induced by DC vaccination could therefore potentially also respond to multiple MAGE-A antigens. 4 µm slices were cut from formalin-fixed paraffin-embedded (FFPE) tumor tissue and placed on glass slides. Tissue was deparaffinized in xylene, rehydrated in ethanol and washed in distilled water. Antigen retrieval was performed by boiling in EnVision™ FLEX target retrieval solution (pH 9, K8004, Dako, Santa Clara CA) for 10 minutes for gp100 and tyrosinase samples or in citrate buffer (pH 6, CBB999, ScyTek Laboratories, Logan, UT) for 15 minutes for MAGE-C2, MAGE-A and NY-ESO-1 samples. After cooling down, slides were washed with distilled water and EnVision™ FLEX Wash Buffer (DM831, Dako). Slides were blocked with antibody diluent and afterward incubated with primary antibodies for the detection of gp100 (M063401, clone HMB45, Dako, dilution: 1/600), tyrosinase (MONX10591, clone T311; Monosan, Uden, the Netherlands; dilution: 1/200), MAGE-A (sc-20034, clone 6C1; Santa Cruz Biotechnology, Dallas, TX; dilution: 1/50), MAGE-C2 (HPA062230, rabbit polyclonal; Merck, Kenilworth, NJ; dilution: 1/200) and NY-ESO-1 (MABC1151, clone D8.38, Merck, dilution: 1/200) for 1 hour. Next, slides were incubated with Opal Polymer HRP (NEL801001KT; PerkinElmer, Waltham, MA) for 30 min. Fluorescent visualization was performed with the Opal 650 (NEL801001KT; PerkinElmer) for 10 minutes. All incubation steps were performed at room temperature. Slides were washed with EnVision™ FLEX Wash Buffer between different incubation steps. Mounting was performed with DAPI Fluoromount-G (0100–20; SouthernBiotech, Birmingham, AL). Slides were prescanned using the PerkinElmer Vectra, version 3.0.4 (PerkinElmer) at 10x magnification. Positivity was scored using whole scanned overviews in Phenochart, version 1.0.9 (PerkinElmer) and 20x regions for representation were selected. For the lymph node tissue, the last resected lymph node (harvested during sentinel node biopsy or RLND) containing metastatic disease prior to the start of study was used for staining. Because of the high expression of both gp100 and tyrosinase in melanoma,Citation39 we only assessed the expression of these proteins in patients with recurrent disease. Of these patients, we stained the tissue of the recurrence and the last available material prior to the start of study. Tumor tissue was scored positive for the expression of an antigen when expression was present in at least 1% of tumor cells.
Statistical analysis
The estimates of RFS and OS were calculated using Kaplan–Meier probability. RFS was defined as time from apheresis to first date of recurrence and date of death from any cause was used to calculate OS. When events had not occurred, survival was censored at the date of last follow-up. Follow-up duration was determined from date of apheresis to date of last follow-up and censored for death. To prevent a guarantee-time bias interfering with immunological and clinical responses, we primarily analyzed the data of the immunological response after the first cycle. P values of less than 0.05 were considered significant. All statistical analyses were conducted using IBM SPSS Statistics for Windows, version 25.0 (IBM Corp., Armonk, NY). Figures were created with GraphPad Prism, version 5.03 (GraphPad Software, Inc., La Jolla, CA).
Questionnaire
Before and during the study we measured the health-related quality of life (HRQoL) of the participants with the EORTC QLQ-C30 (version 3.0) questionnaire. Results of the HRQoL analysis have been reported separately.Citation40
Results
Patients
Between October 2015 and August 2016, 17 patients were included of whom 15 were evaluable. Two patients (pDC-5 and pDC-6) were replaced because of not having reached the first immunological assessment around week 9 due to rapid recurrence. The 15 patients had a median age of 50 (range 19–72) years (for baseline characteristics see ). Substages were equally divided over treatment groups, all but three patients had stage IIIB or IIIC melanoma. At data cutoff on April 21th 2021, all alive patients completed their study follow-up of 5 years.
Table 1. Baseline characteristics
Vaccine characteristics
A new apheresis was necessary for two patients in the cDC2 group, due to low purity or viability of the initial cDC2 product. One patient initially randomized in the combiDC group switched to the cDC2 group due to low pDC retrieval. Production of DC products meeting release criteria was feasible for all patients (Supplementary Figure 2A-F, 3, 4, 5). Median yield, purity and viability were 67 × 106, 64% and 87% for cDC2 products and 48 × 106, 62% and 93% for pDC products, respectively. Cytokine production of cDC2s and pDCs separately shows that mature cDC2s produced IL-12 and mature pDCs produced IFNα, as expected (Supplementary Figure 2G-H).
All patients received the required 2–5 × 106 cDC2s and/or 1–3 × 106 pDCs in each vaccine. In the thawed vaccines, median viability of DCs was 92% (range 72–99%) for cDC2s and 90% (range 59–98%) for pDCs.
Adverse events
All 15 patients experienced at least one grade 1–2 adverse event (), mostly consisting of fatigue. Three patients experienced an injection site reaction, and two patients had flu-like symptoms. During apheresis, 1 grade 3 and 1 grade 4 adverse event were observed, consisting of hypokalemia and hypocalcemia, respectively. These electrolyte disturbances occurred simultaneously in the same patient and are a known possible complication of the apheresis due to interaction with the citrate anticoagulant used during apheresis. Both disturbances were corrected completely with suppletion of electrolytes. The two replaced patients did not experience grade 3 or 4 or any other striking adverse events.
Table 2. Treatment-related adverse events (n = 15)
KLH-specific T cell responses
All cDC2s were loaded with the control antigen KLH. pDCs were not cultured in the presence of KLH, since pDCs are unable to take up KLH protein.Citation41 None of the patients had a KLH-specific proliferation index >2 at baseline. After vaccination, PBMCs of all patients vaccinated with cDCs (cDC2 group and combiDC group) showed increased proliferation, indicating that the cDC2s induced de novo T cell responses in these patients (Supplementary Figure 6).
Induction of antigen-specific T cell responses and antigen expression in melanoma tissue
After each cycle of three vaccinations, DTH skin tests were performed to analyze the presence of antigen-specific T cells in SKILs. Tetramer- or dextramer-staining of SKILs of HLA-A2.1 positive patients showed antigen-specific CD8+ T cells in 4 out of 5 (80%) patients tested after the first vaccination cycle (, Supplementary Table 2, Supplementary Figure 7). In PBMCs, antigen-specific CD8+ T cells were found in 7 out of 11 (64%) HLA-A2.1, HLA-A1, or HLA-B35 positive patients tested after the first vaccination cycle. A lower detection rate of antigen-specific CD8+ T cells in PBMCs compared to SKILs is in line with previous findings and probably due to the accumulation of antigen-specific T cells at the DTH injection site in contrast to a low frequency of those cells circulating in the peripheral blood.Citation11,Citation36,Citation42,Citation43 The presence of tumor-specific T cells in PBMCs before treatment was analyzed for six patients. In none of the tested patients, preexisting responses were detected.
Table 3. Detailed immunological response after each vaccination cycle
Functionality of T cells was tested by IFNγ production of SKILs cocultured with autologous PBMCs loaded with the relevant antigens. After the first vaccination cycle, IFNγ-producing T cells were found in 9 out of 14 (64%) patients tested. Functional T cells were detected more often in patients vaccinated with pDCs or the combiDCs than in patients treated with cDC2s alone, as T cells of 5 out of 5, 4 out of 5 and 0 out of 4 patients per group produced IFNγ, respectively. For patients with functional IFNγ-producing T cells after the first vaccination cycle, median RFS was not reached compared to 17.1 months for patients without functional T cells (p = .23) (Supplementary Figure 8).
Of the 14 tested patients, 4 patients showed functional T cells against the NY-ESO-1 overlapping peptide pool of whom one (25%) patient showed response against the NY-ESO-1 peptide mix. For MAGE-A3, 2 (33%) of the 6 patients with a functional T cell response against the overlapping peptide pool showed a functional response against the peptide mix as well. The more frequent responses to the overlapping peptide pools substantiate the potential benefit of their addition to the mixes of peptides.
In this study, patients were not selected based on their HLA-type and all patients received DCs loaded with the complete peptide mix. Patients can only potentially respond immunologically to vaccination when the HLA-type of the patient matches the HLA-type of the HLA-binding peptides loaded on the DCs. In addition to HLA-binding peptides, for both MAGE-A3 and NY-ESO-1 overlapping peptide pools were used, which enables potential responses independent of HLA-type. Therefore, every patient could respond to the MAGE-A3 and NY-ESO-1 overlapping peptide pools. In addition, based on their HLA type, all patients could at least respond to one of the HLA-specific peptides used for antigen loading. When all cycles are taken into account, none of the patients showed a T cell response against an antigen they could not potentially respond to. Eight out of 15 (53%) patients could potentially respond to the TAA gp100 and tyrosinase and responses were discovered in 13% and 38% of those patients, respectively (). Fifty percent of patients that could potentially respond to the CTA MAGE-C2 did show a T cell response. All patients could respond to the MAGE-A3 peptides or peptide pool and in 11 (73%) of them a T cell response was detected. In 8 (73%) of the 11 patients that could respond to NY-ESO-1 peptides, specific T cells were found. All patients could respond to the NY-ESO-1 peptide pool and 53% of them responded.
Figure 2. Antigen-specific T cell responses
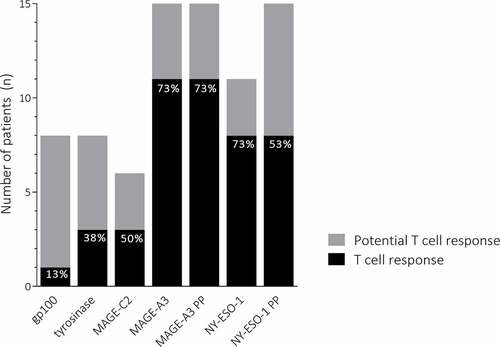
We studied the expression of the antigens used for antigen loading of the DC product in the primary melanoma, lymph node metastasis and lesion of recurrent disease of vaccinated patients. Representative images are shown in Supplementary Figure 9. Gp100 and tyrosinase were expressed in all lesions analyzed (). Expression of MAGE-C2, MAGE-A and NY-ESO-1 was expressed in a lower number of patients: MAGE-C2 in 36%, MAGE-A in 36% and NY-ESO-1 in 7% of the primary lesions. MAGE-C2 was expressed in 58% of lymph node metastases analyzed, for MAGE-A and NY-ESO-1 this was 42% and 25%, respectively. In 7 (50%) patients from whom tissue was analyzed, the antigen against which a T cell response was detected and the antigen expression on the last resected tumor tissue prior to the start of the experimental adjuvant therapy, matched (; patients highlighted in green).
Figure 3. Immunological response and antigen expression
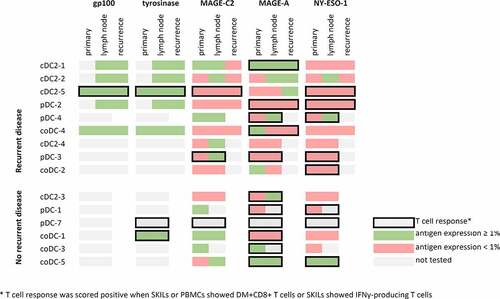
Clinical responses
Thirteen of 15 patients completed 2 or 3 cycles of 3 vaccinations, of whom 6 patients are still free of recurrence. The majority of patients with recurrent disease had stage IIIC melanoma at inclusion. Patient coDC-3 withdrew after two cycles of vaccinations due to personal circumstances but has no recurrent disease. Median RFS for all patients was 19.4 months (Supplementary figure 10A). For patients that received cDC2 or pDC vaccination median RFS was 17.1 and 47.7 months, respectively. In the combiDC group median RFS was not reached. Median OS has not been reached at time of data cutoff (Supplementary figure 10B). Four patients died from a melanoma-related event, two of them received combined cDC2s and pDCs and two cDC2s alone. Subgroups are too small and heterogeneous to draw conclusions about differences between subgroups, however the combination does not seem to be inferior to vaccination with either subset alone.
Discussion
This is the first clinical trial in which melanoma patients were vaccinated with the combination of CD1c+ cDC2s and pDCs. Vaccination with cDC2s and pDCs alone or combined induced immunological responses. Production of the combined vaccine proved to be feasible. In addition, treatment was well-tolerated, and the mild toxicity profile is in line with earlier trials investigating DC vaccination.Citation3,Citation44 Treatment groups were too small to draw conclusions about differences in immunological or clinical response between the three groups. However, combination therapy does not seem to be inferior to vaccination with cDC2s or pDCs alone.
In this trial, cDC2s were predepleted for CD14+ cells and both cDC2s and pDCs were matured by stimulation with pR, a TLR8/9 stimulus. Production of nDC vaccines meeting predefined release criteria for purity, maturation and viability was achieved for all patients in all groups. PR-matured pDCs produced high amounts of IFNα, which is a common hallmark of activated pDCs and important for their function in enhancing the cellular response and stimulation of cells of the innate immune system important for an anti-tumor response.Citation45,Citation46 PR-matured cDC2s produced IL-12, important to turn naive CD4+ and CD8+ T cells into T-helper 1 and cytotoxic T cells, respectively.Citation47 In addition, costimulatory molecules were highly expressed on both subsets after pR maturation, which is in line with our previous findings.Citation20
In this trial, cDC2s and pDCs were cultured separately and pooled before injection. Coculturing might further improve the nDC vaccine as this enables cross-activation between cDC2s and pDCs during maturation, possibly leading to a superior maturation state of DCs, as previously shown.Citation48 It has been shown that when cDC2s and pDCs are matured in a coculture, cytokine production and expression of costimulatory molecules is higher and the activation of other immune cells that could play an important role in the anti-tumor response, such as natural killer cells and plasma cells, is stronger.Citation25,Citation46,Citation48,Citation49 Furthermore, nDC vaccination could be further improved by the addition of the even less frequent CD141+ (BDCA-3) subset of cDCs (cDC1s). These cells play an important role in cross presentation of necrotic cell-derived antigens and activation of CD8+ T cells.Citation50–52 Recently, it became possible to select these scarce CD141+ cDC1s from the blood by magnetic separation.Citation53 All three subsets of nDCs have distinct phenotypes and mechanisms of influencing the immune response, probably making their function complementary. Hence, and considering the excellent capacity of CD141+ cDC1s to cross-present tumor cell-derived antigens, DC vaccination with a combination of all three subsets could induce even more potent anti-tumor responses as compared to either of the single DC subsets.Citation54
We expanded the pool of antigens of the two TAA gp100 and tyrosinase used in previous trials, with the three CTA MAGE-C2, MAGE-A3, and NY-ESO-1 which are present frequently in melanoma.Citation22 Immunological responses against each of the antigens used were observed. The expression of CTA in melanoma tissue was lower compared to TAA. As such, analysis of the antigen expression prior to the start of study could further improve the selection of antigens used. As was done in this trial, it is important to take the frequency of HLA types into account when peptides binding certain HLA-types are used for antigen loading. This can be circumvented by the use of overlapping peptide pools as was done in this trial, which increased the immunological responses against these antigens. Overlapping peptide pools are therefore of interest for future trials in addition to the use of individual peptides.
Immunological responses were detected in the majority of patients. After the first vaccination cycle antigen-specific CD8+ T cells in SKILs were found in 80% and functional T cells in 64% of tested patients treated with adjuvant nDC vaccination. These numbers are at least comparable to the amount of antigen-specific CD8+ and functional T cells after adjuvant moDC vaccination of stage III melanoma patients we reported earlier and higher than was reported for cDC2 or pDC vaccination in stage IV melanoma.Citation10,Citation11 Drawing conclusions about a possible difference between nDC and moDC vaccination is difficult because clinical characteristics of stage III patients are not homogeneous and more antigens were used in the here described trial. The number of patients with functional T cells after combined cDC2 and pDC vaccination was as high as for pDC vaccination alone, and might be superior compared to cDC2 vaccination alone. Although groups are small, the combination does not seem to be inferior in eliciting immunological responses, compared to the single subsets alone and allows application in a larger patient cohort.
In one patient (cDC2-5) with a T cell response against both gp100 and tyrosinase, one patient (coDC-1) with a T cell response against tyrosinase and one patient (cDC2-1) with a T cell response against MAGE-A3, the respective antigens were present in the tumor tissue prior to the start of study and in cDC2-5 and cDC2-1 remained present in the recurrent lesion. The continuation of antigen expression despite an antigen-specific T cell response indicates incomplete killing of the antigen-expressing tumor cells. This might be due to immune suppressive factors, suppressing the cytotoxicity of T cells. Escape mechanisms that might be present are, for example, immunosuppressive immune cells such as myeloid-derived suppressor cells and regulatory T cells or the upregulation of inhibitory immune checkpoints, such as CTLA-4 and PD-L1.Citation55 The addition of therapy interfering with these immunosuppressive mechanisms inhibiting the anti-tumor effect of DC vaccination is therefore of interest for future research. Combination of DC vaccination with mAbs against the checkpoint molecule CTLA-4, which inhibits activation of T cells, already showed promising clinical responses when combined with DC vaccination.Citation56,Citation57 MAbs interfering with the PD-1/PD-L1 pathway inhibit T cell exhaustion in the tumor microenvironment and could therefore also enhance the anti-tumor effect of T cells induced by DC vaccination. Due to their superior survival benefit and toxicity profile compared to anti-CTLA mAbs it is a logical next step to combine anti-PD-1 mAbs and DC vaccination in future research investigating combination therapy.Citation58,Citation59
We included stage III melanoma patients, a heterogeneous group, of which most were high-risk patients (stage IIIB or IIIC). Because of the high-risk of relapse, multiple phase III trials have been conducted investigating immune checkpoint inhibition and targeted therapy. Results of these trials led to the approval of the immune checkpoint inhibition by nivolumab and pembrolizumab (both anti-PD-1 mAbs) based on improved RFS and targeted therapy with combined dabrafenib and trametinib (BRAF and MEK inhibitor) for BRAF V600-mutated melanoma based on OS benefit by both the European Medicines Agency (EMA) and Food and Drug Administration (FDA).Citation33–35 In addition, the FDA approved adjuvant use of the immune checkpoint inhibitor ipilimumab (anti-CTLA-4 mAbs).Citation60 However, toxicity of these adjuvant therapies is high as 14–46% of patients encountered treatment-related grade 3–4 events.Citation33–35,Citation60 The mild toxicity profile of adjuvant DC vaccination is favorable compared to these registered treatment options, as only grade 1–2 adverse events occurred and these mainly consisted of fatigue.
As previously shown, nDC vaccination does not withhold a clinically relevant improvement of the HRQoL, probably due to recovery from RLND, during adjuvant therapy.Citation40 This finding is supported by HRQoL data of DC vaccination in other malignancies.Citation61,Citation62 HRQoL of patients treated with adjuvant nivolumab or dabrafenib/trametinib remained unchanged during the trial.Citation33,Citation63 However, despite the expected recovery from RLND, a non-clinically relevant decline in HRQoL during adjuvant treatment with ipilimumab and pembrolizumab was found.Citation64,Citation65 In the adjuvant setting HRQoL is an important aspect, as half of the stage III patients will not endure a relapse without adjuvant therapy, but are exposed to the potentially severe toxicity. In addition, melanoma patients are relatively young and the majority is of working-age, making the impact on HRQoL even more important. Of course, impact on survival is critical in the choice of therapy. The survival benefit of adjuvant nDC vaccination is currently investigated in our placebo-controlled randomized controlled trial (NCT02993315) in which patients receive combined CD1c+ cDC2s and pDCs. Results should be assessed in the context of our current standard of care in the adjuvant setting.
In conclusion, production of a vaccine with combined cDC2s and pDCs is feasible. Adjuvant treatment of stage III melanoma patients with both nDC subsets simultaneously was well tolerated with only mild grade 1–2 adverse events. DC-based immunotherapy was capable of inducing immunological response and combined cDC2 and pDC vaccination was not inferior to vaccination with each of the single subsets alone. This allowed further exploration of the clinical response to combined DC vaccination in a placebo-controlled clinical trial in which patients were enrolled prior to the approval of the currently registered adjuvant therapies. Results are expected at the beginning of 2022.
Disclosure of interest
The authors report no conflict of interest
Supplemental Material
Download Zip (12.4 MB)Acknowledgments
The authors thank the patients for their participation in the clinical trial. Furthermore, they thank the technicians of the Tumor Immunology Laboratory Annemiek J. de Boer, Kevin J.H. Bos, Tjitske Duiveman-de Boer, Michel A.M. Olde Nordkamp, Tom G.M. van Oorschot, Carlijn J. Popelier, Jeanette M. Pots, Nicole M. Scharenborg, Mandy W.M.M. van de Rakt, Valeska de Ruiter and Kiek N. Verrijp for experimental support. The authors thank Simone M. Hins-de Bree for clinical support.
Disclosure statement
No potential conflict of interest was reported by the author(s).
Supplementary material
Supplemental data for this article can be accessed on the publisher’s website
Additional information
Funding
References
- Banchereau J, Steinman RM. Dendritic cells and the control of immunity. Nature. 1998;392(6673):245–12. doi:10.1038/32588.
- Palucka K, Banchereau J. Cancer immunotherapy via dendritic cells. Nat Rev Cancer. 2012;12(4):265–277. doi:10.1038/nrc3258.
- Anguille S, Smits EL, Lion E, van Tendeloo VF, Berneman ZN. Clinical use of dendritic cells for cancer therapy. Lancet Oncol. 2014;15(7):e257–67. doi:10.1016/S1470-2045(13)70585-0.
- Baraibar I, Melero I, Ponz-Sarvise M, Castanon E. Safety and Tolerability of Immune Checkpoint Inhibitors (PD-1 and PD-L1) in Cancer. Drug Saf. 2019;42(2):281–294. doi:10.1007/s40264-018-0774-8.
- Soruri A, Kiafard Z, Dettmer C, Riggert J, Kohl J, Zwirner J. IL-4 down-regulates anaphylatoxin receptors in monocytes and dendritic cells and impairs anaphylatoxin-induced migration in vivo. J Immunol. 2003;170(6):3306–3314. doi:10.4049/jimmunol.170.6.3306.
- Breckpot K, Corthals J, Bonehill A, Michiels A, Tuyaerts S, Aerts C, Heirman C, Thielemans K. Dendritic cells differentiated in the presence of IFN-β and IL-3 are potent inducers of an antigen-specific CD8+ T cell response. J Leukoc Biol. 2005;78(4):898–908. doi:10.1189/jlb.0105052.
- Thurnher M, Zelle-Rieser C, Ramoner R, Bartsch G, Holtl L. The disabled dendritic cell. FASEB J. 2001;15(6):1054–1061. doi:10.1096/fsb2fj000508hyp.
- Wimmers F, Schreibelt G, Skold AE, Figdor CG, De Vries IJ. Paradigm shift in dendritic cell-based immunotherapy: from in vitro generated monocyte-derived DCs to naturally circulating DC subsets. Front Immunol. 2014;5:165. doi:10.3389/fimmu.2014.00165.
- MacDonald KP, Munster DJ, Clark GJ, Dzionek A, Schmitz J, Hart DN. Characterization of human blood dendritic cell subsets. Blood. 2002;100(13):4512–4520. doi:10.1182/blood-2001-11-0097.
- Schreibelt G, Bol KF, Westdorp H, Wimmers F, Aarntzen EHJG, Duiveman-de Boer T, van de Rakt MWMM, Scharenborg NM, de Boer AJ, Pots JM, et al. Effective clinical responses in metastatic melanoma patients after vaccination with primary myeloid dendritic cells. Clin Cancer Res. 2016;22(9):2155–2166. doi:10.1158/1078-0432.CCR-15-2205.
- Tel J, Aarntzen EHJG, Baba T, Schreibelt G, Schulte BM, Benitez-Ribas D, Boerman OC, Croockewit S, Oyen WJG, van Rossum M, et al. Natural human plasmacytoid dendritic cells induce antigen-specific T-cell responses in melanoma patients. Cancer Res. 2013;73(3):1063–1075. doi:10.1158/0008-5472.CAN-12-2583.
- Westdorp H, Creemers JHA, van Oort IM, Schreibelt G, Gorris MAJ, Mehra N, Simons M, de Goede AL, van Rossum MM, Croockewit AJ, et al. Blood-derived dendritic cell vaccinations induce immune responses that correlate with clinical outcome in patients with chemo-naive castration-resistant prostate cancer. J Immunother Cancer. 2019;7(1):302. doi:10.1186/s40425-019-0787-6.
- Jonuleit H, Giesecke-Tuettenberg A, Tuting T, Thurner-Schuler B, Stuge TB, Paragnik L, Kandemir A, Lee PP, Schuler G, Knop J, et al. A comparison of two types of dendritic cell as adjuvants for the induction of melanoma-specific T-cell responses in humans following intranodal injection. Int J Cancer. 2001;93(2):243–251. doi:10.1002/ijc.1323.
- De Vries IJ, Krooshoop DJ, Scharenborg NM, Lesterhuis WJ, Diepstra JH, Van Muijen GN, Strijk SP, Ruers TJ, Boerman OC, Oyen WJG, et al. Effective migration of antigen-pulsed dendritic cells to lymph nodes in melanoma patients is determined by their maturation state. Cancer Res. 2003;63(1):12–17.
- de Vries IJ, Lesterhuis WJ, Scharenborg NM, Engelen LP, Ruiter DJ, Gerritsen MJ, Croockewit S, Britten CM, Torensma R, Adema GJ, et al. Maturation of dendritic cells is a prerequisite for inducing immune responses in advanced melanoma patients. Clin Cancer Res. 2003;9(14):5091–5100.
- Boullart AC, Aarntzen EH, Verdijk P, Jacobs JF, Schuurhuis DH, Benitez-Ribas D, Schreibelt G, van de Rakt MWMM, Scharenborg NM, de Boer A, et al. Maturation of monocyte-derived dendritic cells with Toll-like receptor 3 and 7/8 ligands combined with prostaglandin E2 results in high interleukin-12 production and cell migration. Cancer Immunol Immunother. 2008;57(11):1589–1597. doi:10.1007/s00262-008-0489-2.
- Grauer O, Poschl P, Lohmeier A, Adema GJ, Bogdahn U. Toll-like receptor triggered dendritic cell maturation and IL-12 secretion are necessary to overcome T-cell inhibition by glioma-associated TGF-beta2. J Neurooncol. 2007;82(2):151–161. doi:10.1007/s11060-006-9274-2.
- Mailliard RB, Wankowicz-Kalinska A, Cai Q, Wesa A, Hilkens CM, Kapsenberg ML, Kirkwood JM, Storkus WJ, Kalinski P. alpha-type-1 polarized dendritic cells: a novel immunization tool with optimized CTL-inducing activity. Cancer Res. 2004;64(17):5934–5937. doi:10.1158/0008-5472.CAN-04-1261.
- Sporri R, Reis E Sousa C. Inflammatory mediators are insufficient for full dendritic cell activation and promote expansion of CD4+ T cell populations lacking helper function. Nat Immunol. 2005;6(2):163–170. doi:10.1038/ni1162.
- Skold AE, van Beek JJ, Sittig SP, Bakdash G, Tel J, Schreibelt G, de Vries IJM. Protamine-stabilized RNA as an ex vivo stimulant of primary human dendritic cell subsets. Cancer Immunol Immunother. 2015;64(11):1461–1473. doi:10.1007/s00262-015-1746-9.
- Bakdash G, Buschow SI, Gorris MA, Halilovic A, Hato SV, Skold AE, Schreibelt G, Sittig SP, Torensma R, Duiveman-de Boer T, et al. Expansion of a BDCA1+CD14+myeloid cell population in melanoma patients may attenuate the efficacy of dendritic cell vaccines. Cancer Res. 2016;76(15):4332–4346. doi:10.1158/0008-5472.CAN-15-1695.
- Tio D, Kasiem FR, Willemsen M, van Doorn R, van der Werf N, Hoekzema R, Luiten RM, Bekkenk MW. Expression of cancer/testis antigens in cutaneous melanoma: a systematic review. Melanoma Res. 2019;29(4):349–357. doi:10.1097/CMR.0000000000000569.
- de Vries TJ, Fourkour A, Punt CJ, Ruiter DJ, van Muijen GN. Analysis of melanoma cells in peripheral blood by reverse transcription-polymerase chain reaction for tyrosinase and MART-1 after mononuclear cell collection with cell preparation tubes: a comparison with the whole blood guanidinium isothiocyanate RNA isolation method. Melanoma Res. 2000;10:119–126.
- Schreibelt G, Tel J, Sliepen KH, Benitez-Ribas D, Figdor CG, Adema GJ, de Vries IJM. Toll-like receptor expression and function in human dendritic cell subsets: implications for dendritic cell-based anti-cancer immunotherapy. Cancer Immunol Immunother. 2010;59(10):1573–1582. doi:10.1007/s00262-010-0833-1.
- Bakdash G, Schreurs I, Schreibelt G, Tel J. Crosstalk between dendritic cell subsets and implications for dendritic cell-based anticancer immunotherapy. Expert Rev Clin Immunol. 2014;10(7):915–926. doi:10.1586/1744666X.2014.912561.
- Lou Y, Liu C, Kim GJ, Liu YJ, Hwu P, Wang G. Plasmacytoid dendritic cells synergize with myeloid dendritic cells in the induction of antigen-specific antitumor immune responses. J Immunol. 2007;178(3):1534–1541. doi:10.4049/jimmunol.178.3.1534.
- Gajewski TF, Meng Y, Blank C, Brown I, Kacha A, Kline J, Harlin H. Immune resistance orchestrated by the tumor microenvironment. Immunol Rev. 2006;213(1):131–145. doi:10.1111/j.1600-065X.2006.00442.x.
- Boudewijns S, Bol KF, Schreibelt G, Westdorp H, Textor JC, van Rossum MM, Scharenborg NM, de Boer AJ, van de Rakt MWMM, Pots JM, et al. Adjuvant dendritic cell vaccination induces tumor-specific immune responses in the majority of stage III melanoma patients. Oncoimmunology. 2016;5(7):e1191732. doi:10.1080/2162402X.2016.1191732.
- Aarntzen EHJG, Bol K, Schreibelt G, Jacobs JFM, Lesterhuis WJ, Van Rossum MM, Adema GJ, Figdor CG, Punt CJA, De Vries IJM, et al. Skin-test infiltrating lymphocytes early predict clinical outcome of dendritic cell-based vaccination in metastatic melanoma. Cancer Res. 2012;72(23):6102–6110. doi:10.1158/0008-5472.CAN-12-2479.
- Bol K, Aarntzen EHJG, Hout FEMI, Schreibelt G, Creemers JHA, Lesterhuis WJ, Gerritsen WR, Grunhagen DJ, Verhoef C, Punt CJA. Favorable overall survival in stage III melanoma patients after adjuvant dendritic cell vaccination. OncoImmunology. 2016;5(1):1. doi:10.1080/2162402X.2015.1057673.
- Balch CM, Gershenwald JE, Soong S-J, Thompson JF, Atkins MB, Byrd DR, Buzaid AC, Cochran AJ, Coit DG, Ding S, et al. Final version of 2009 AJCC melanoma staging and classification. J clin oncol. 2009;27(36):6199–6206. doi:10.1200/JCO.2009.23.4799.
- Eggermont AM, Chiarion-Sileni V, Grob JJ, Dummer R, Wolchok JD, Schmidt H, Hamid O, Robert C, Ascierto PA, Richards JM, et al. Prolonged survival in stage III melanoma with ipilimumab adjuvant therapy. N Engl J Med. 2016;375(19):1845–1855. doi:10.1056/NEJMoa1611299.
- Weber J, Mandala M, Del Vecchio M, Gogas HJ, Arance AM, Cowey CL, Dalle S, Schenker M, Chiarion-Sileni V, Marquez-Rodas I, et al. Adjuvant nivolumab versus ipilimumab in resected stage III or IV melanoma. N Engl J Med. 2017;377(19):1824–1835. doi:10.1056/NEJMoa1709030.
- Long GV, Hauschild A, Santinami M, Atkinson V, Mandala M, Chiarion-Sileni V, Larkin J, Nyakas M, Dutriaux C, Haydon A, et al. Adjuvant dabrafenib plus trametinib in stage III BRAF-mutated melanoma. N Engl J Med. 2017;377(19):1813–1823. doi:10.1056/NEJMoa1708539.
- Eggermont AMM, Blank CU, Mandala M, Long GV, Atkinson V, Dalle S, Haydon A, Lichinitser M, Khattak A, Carlino MS, et al. Adjuvant pembrolizumab versus placebo in resected stage III melanoma. N Engl J Med. 2018;378(19):1789–1801. doi:10.1056/NEJMoa1802357.
- de Vries IJ, Bernsen MR, Lesterhuis WJ, Scharenborg NM, Strijk SP, Gerritsen MJ, Ruiter DJ, Figdor CG, Punt CJA, Adema GJ, et al. Immunomonitoring tumor-specific T cells in delayed-type hypersensitivity skin biopsies after dendritic cell vaccination correlates with clinical outcome. J Clin Oncol. 2005;23(24):5779–5787. doi:10.1200/JCO.2005.06.478.
- Simpson AJ, Caballero OL, Jungbluth A, Chen YT, Old LJ. Cancer/testis antigens, gametogenesis and cancer. Nat Rev Cancer. 2005;5(8):615–625. doi:10.1038/nrc1669.
- Chinnasamy N, Wargo JA, Yu Z, Rao M, Frankel TL, Riley JP, Hong JJ, Parkhurst MR, Feldman SA, Schrump DS, et al. A TCR targeting the HLA-A*0201-restricted epitope of MAGE-A3 recognizes multiple epitopes of the MAGE-A antigen superfamily in several types of cancer. J Immunol. 2011;186(2):685–696. doi:10.4049/jimmunol.1001775.
- de Vries TJ, Smeets M, de Graaf R, Hou-Jensen K, Brocker EB, Renard N, Eggermont AMM, van Muijen GNP, Ruiter DJ. Expression of gp100, MART-1, tyrosinase, and S100 in paraffin-embedded primary melanomas and locoregional, lymph node, and visceral metastases: implications for diagnosis and immunotherapy. A study conducted by the EORTC Melanoma Cooperative Group. J Pathol. 2001;193(1):13–20. doi:10.1002/1096-9896(2000)9999:9999<::AID-PATH729>3.0.CO;2-D.
- Bloemendal M, Rietveld MJA, van Willigen WW, Gerritsen WR, Figdor CG, Bonenkamp JJ, Westdorp H, Boudewijns S, Koornstra RHT, Adang EMM, et al. Health-related quality of life analysis in stage III melanoma patients treated with adjuvant dendritic cell therapy. Clin Transl Oncol. 2019;21(6):774–780. doi:10.1007/s12094-018-1987-0.
- Benitez-Ribas D, Adema GJ, Winkels G, Klasen IS, Punt CJ, Figdor CG, de Vries IJM. Plasmacytoid dendritic cells of melanoma patients present exogenous proteins to CD4+ T cells after Fc gamma RII-mediated uptake. J Exp Med. 2006;203(7):1629–1635. doi:10.1084/jem.20052364.
- Bol KF, Figdor CG, Aarntzen EH, Welzen ME, van Rossum MM, Blokx WA, van de Rakt MW, Scharenborg NM, de Boer AJ, Pots JM, et al. Intranodal vaccination with mRNA-optimized dendritic cells in metastatic melanoma patients. Oncoimmunology. 2015;4(8):e1019197. doi:10.1080/2162402X.2015.1019197.
- Aarntzen EHJG, Schreibelt G, Bol K, Lesterhuis WJ, Croockewit AJ, de Wilt JHW, van Rossum MM, Blokx WAM, Jacobs JFM, Duiveman-de Boer T, et al. Vaccination with mRNA-electroporated dendritic cells induces robust tumor antigen-specific CD4+ and CD8+T cells responses in stage III and IV melanoma patients. Clin Cancer Res. 2012;18(19):5460–5470. doi:10.1158/1078-0432.CCR-11-3368.
- Draube A, Klein-Gonzalez N, Mattheus S, Brillant C, Hellmich M, Engert A, Von Bergwelt-baildon M. Dendritic cell based tumor vaccination in prostate and renal cell cancer: a systematic review and meta-analysis. PLoS One. 2011;6(4):e18801. doi:10.1371/journal.pone.0018801.
- Ito T, Liu YJ, Kadowaki N. Functional diversity and plasticity of human dendritic cell subsets. Int J Hematol. 2005;81(3):188–196. doi:10.1532/IJH97.05012.
- Skold AE, Mathan TSM, van Beek JJP, Florez-Grau G, van Den Beukel MD, Sittig SP, Boullart ACI, Aarntzen EHJG, Verdijk P, Jacobs JFM, et al. Naturally produced type I IFNs enhance human myeloid dendritic cell maturation and IL-12p70 production and mediate elevated effector functions in innate and adaptive immune cells. Cancer Immunol Immunother. 2018;67(9):1425–1436. doi:10.1007/s00262-018-2204-2.
- Nizzoli G, Krietsch J, Weick A, Steinfelder S, Facciotti F, Gruarin P, Bianco A, Steckel B, Moro M, Crosti M, et al. Human CD1c+ dendritic cells secrete high levels of IL-12 and potently prime cytotoxic T-cell responses. Blood. 2013;122(6):932–942. doi:10.1182/blood-2013-04-495424.
- Piccioli D, Sammicheli C, Tavarini S, Nuti S, Frigimelica E, Manetti AG, Nuccitelli A, Aprea S, Valentini S, Borgogni E, et al. Human plasmacytoid dendritic cells are unresponsive to bacterial stimulation and require a novel type of cooperation with myeloid dendritic cells for maturation. Blood. 2009;113(18):4232–4239. doi:10.1182/blood-2008-10-186890.
- van Beek JJ, Gorris MA, Skold AE, Hatipoglu I, Van Acker HH, Smits EL, de Vries IJM, Bakdash G. Human blood myeloid and plasmacytoid dendritic cells cross activate each other and synergize in inducing NK cell cytotoxicity. Oncoimmunology. 2016;5(10):e1227902. doi:10.1080/2162402X.2016.1227902.
- Collin M, Bigley V. Human dendritic cell subsets: an update. Immunology. 2018;154(1):3–20. doi:10.1111/imm.12888.
- Jongbloed SL, Kassianos AJ, McDonald KJ, Clark GJ, Ju X, Angel CE, Chen CJJ, Dunbar PR, Wadley RB, Jeet V, et al. Human CD141+ (BDCA-3)+ dendritic cells (DCs) represent a unique myeloid DC subset that cross-presents necrotic cell antigens. J Exp Med. 2010;207(6):1247–1260. doi:10.1084/jem.20092140.
- Bachem A, Guttler S, Hartung E, Ebstein F, Schaefer M, Tannert A, Salama A, Movassaghi K, Opitz C, Mages HW, et al. Superior antigen cross-presentation and XCR1 expression define human CD11c+CD141+ cells as homologues of mouse CD8+ dendritic cells. J Exp Med. 2010;207(6):1273–1281. doi:10.1084/jem.20100348.
- Bol KF, Schreibelt G, Rabold K, Wculek SK, Schwarze JK, Dzionek A, Teijeira A, Kandalaft LE, Romero P, Coukos G, et al. The clinical application of cancer immunotherapy based on naturally circulating dendritic cells. J Immunother Cancer. 2019;7(1):109. doi:10.1186/s40425-019-0580-6.
- Worah K, Mathan TSM, Vu Manh TP, Keerthikumar S, Schreibelt G, Tel Jd, Duiveman-de Boer T, Sköld AE, van Spriel AB, de Vries IJM, et al. Proteomics of human dendritic cell subsets reveals subset-specific surface markers and differential inflammasome function. Cell Rep. 2016;16(11):2953–2966. doi:10.1016/j.celrep.2016.08.023.
- Emerson DA, Redmond WL. Overcoming tumor-induced immune suppression: from relieving inhibition to providing costimulation with T cell agonists. BioDrugs. 2018;32(3):221–231. doi:10.1007/s40259-018-0277-2.
- Ribas A, Comin-Anduix B, Chmielowski B, Jalil J, de La Rocha P, McCannel TA, Ochoa MT, Seja E, Villanueva A, Oseguera DK, et al. Dendritic cell vaccination combined with CTLA4 blockade in patients with metastatic melanoma. Clin Cancer Res. 2009;15(19):6267–6276. doi:10.1158/1078-0432.CCR-09-1254.
- Wilgenhof S, Corthals J, Heirman C, van Baren N, Lucas S, Kvistborg P, Thielemans K, Neyns B. Phase II study of autologous monocyte-derived mRNA electroporated dendritic cells (TriMixDC-MEL) plus ipilimumab in patients with pretreated advanced melanoma. J clin oncol. 2016;34(12):1330–1338. doi:10.1200/JCO.2015.63.4121.
- Versteven M, Van Den Bergh JMJ, Marcq E, Smits ELJ, Van Tendeloo VFI, Hobo W, Lion E. Dendritic cells and programmed death-1 blockade: a joint venture to combat cancer. Front Immunol. 2018;9:394. doi:10.3389/fimmu.2018.00394.
- Achkar T, Tarhini AA. The use of immunotherapy in the treatment of melanoma. J Hematol Oncol. 2017;10(1):88. doi:10.1186/s13045-017-0458-3.
- Eggermont AM, Chiarion-Sileni V, Grob JJ, Dummer R, Wolchok JD, Schmidt H, Hamid O, Robert C, Ascierto PA, Richards JM, et al. Adjuvant ipilimumab versus placebo after complete resection of high-risk stage III melanoma (EORTC 18071): a randomised, double-blind, phase 3 trial. Lancet Oncol. 2015;16(5):522–530. doi:10.1016/S1470-2045(15)70122-1.
- Leonhartsberger N, Ramoner R, Falkensammer C, Rahm A, Gander H, Holtl L, Thurnher M. Quality of life during dendritic cell vaccination against metastatic renal cell carcinoma. Cancer Immunol Immunother. 2012;61(9):1407–1413. doi:10.1007/s00262-012-1207-7.
- Burgdorf SK, Fischer A, Myschetzky PS, Munksgaard SB, Zocca MB, Claesson MH, Rosenberg J. Clinical responses in patients with advanced colorectal cancer to a dendritic cell based vaccine. Oncol Rep. 2008;20:1305–1311.
- Schadendorf D, Hauschild A, Santinami M, Atkinson V, Mandala M, Chiarion-Sileni V, Larkin JMG, Nyakas M, Dutriaux C, Haydon A. Effect on health-related quality of life (HRQOL) of adjuvant treatment (tx) with dabrafenib plus trametinib (D + T) in patients (pts) with resected stage III BRAF-mutant melanoma. J Clin Oncol. 2018;36(15_suppl):15. doi:10.1200/JCO.2018.36.15_suppl.9590.
- Coens C, Suciu S, Chiarion-Sileni V, Grob JJ, Dummer R, Wolchok JD, Schmidt H, Hamid O, Robert C, Ascierto PA, et al. Health-related quality of life with adjuvant ipilimumab versus placebo after complete resection of high-risk stage III melanoma (EORTC 18071): secondary outcomes of a multinational, randomised, double-blind, phase 3 trial. Lancet Oncol. 2017;18(3):393–403. doi:10.1016/S1470-2045(17)30015-3.
- Coens C, Bottomley A, Blank CU, Mandala M, Long GV, Atkinson VG, Dalle S, Haydon A, Lichinitser M, Khattak A, et al. Health-related quality-of-life results for pembrolizumab versus placebo after complete resection of high-risk stage III melanoma from the EORTC 1325-MG/Keynote 054 trial: an international randomized double-blind phase III trial. Ann Oncol. 2018;29:456. doi:10.1093/annonc/mdy289.034.