ABSTRACT
Ligands for Stimulator of Interferon Genes (STING) receptor are under investigation as adjuvants in cancer therapy. Multiple effects have been described, including induction of immunogenic cell death and enhancement of CD8 T-cell mediated anti-tumor immunity. However, the potential effects of STING ligands on activation and effector functions of tumor-reactive human γδ T cells have not yet been investigated. We observed that cyclic dinucleotide as well as novel non-dinucleotide STING ligands diABZI and MSA-2 co-stimulated cytokine induction in Vδ2 T cells within peripheral blood mononuclear cells but simultaneously inhibited their proliferative expansion in response to the aminobisphosphonate Zoledronate and to γδ T-cell specific phosphoantigen. In purified γδ T cells, STING ligands co-stimulated cytokine induction but required the presence of monocytes. STING ligands strongly stimulated IL-1β and TNF-α secretion in monocytes and co-stimulated cytokine induction in short-term expanded Vδ2 γδ T-cell lines. Simultaneously, massive cell death was triggered in both cell populations. Activation of STING as revealed by TBK1/IRF3 phosphorylation and IP-10 secretion varied among STING-expressing tumor cells. STING ligands modulated tumor cell killing by Vδ2 T cells as analyzed in Real-Time Cell Analyzer to variable degree, depending on the tumor target and time course kinetics. Our study reveals complex regulatory effects of STING ligands on human γδ T cells in vitro. These results help to define conditions where STING ligands might boost the efficacy of γδ T cell immunotherapy in vivo.
Introduction
γδ T cells have recently attracted substantial attention as potential effector cells in T cell-based cancer immunotherapy. This is mainly based on two prominent features of γδ T cells, namely (i) the HLA-independent mode of activation and tumor cell recognition, and (ii) the strong capacity to kill a broad range of leukemic and solid tumor cells.Citation1 The two major subsets of human γδ T cells differ in their pathway of tumor cell recognition. Vδ2 (usually paired with Vγ9) γδ T cells are the dominant γδ T-cell subset in the peripheral blood. The T-cell receptor (TCR) mediated activation of Vγ9Vδ2 T cells (termed Vδ2 in the following) critically depends on the expression of butyrophilin (BTN) family members on accessory cells and tumor cells, specifically BTN3A1 and BTN2A1.Citation2–4 Pyrophosphates such as (E)-4-Hydroxy-3-methyl-but-2-enyl pyrophosphate (HMBPP) secreted by microbes or isopentenyl pyrophosphate (IPP) overproduced by transformed cells bind to the intracellular butyrophilin B30.2 domain and induce a conformational change of extracellular BTN3A1/BTN2A1 domains. This leads to the unmasking of ligands which bind to TCR Vγ9 germline-encoded regions, thereby triggering TCR activation.Citation5 Importantly, the conformational change of BTN3A can be mimicked by agonistic antibodies such as clone 20.1 which then induce selective activation and expansion of Vδ2 T cells in the presence of IL-2.Citation2,Citation6 The recognition of tumor cells by Vδ2 T cells depends on the endogenous overproduction of IPP in transformed cells, which also activates the Vγ9Vδ2 TCR in a BTN3A1-dependent manner. IPP is an intermediate of the eukaryotic mevalonate pathway of cholesterol synthesis. IPP levels in normal cells are below the threshold to activate γδ T cells, but stressed and transformed cells have a dysregulated mevalonate pathway, leading to accumulation of IPP and subsequent Vδ2 T-cell activation.Citation7 The mevalonate pathway can be manipulated pharmacologically. Nitrogen-containing aminobisphosphonates like Zoledronate (ZOL) block farnesyl pyrophosphate synthase, an enzyme downstream of IPP synthesis. ZOL treatment increases the susceptibility of tumor cells to Vδ2 T-cell mediated lysis, but also induces selective activation and expansion of Vδ2 T cells when added to peripheral blood mononuclear cells (PBMC) in the presence of IL-2.Citation8,Citation9 In addition to the TCR, Vδ2 T cells usually express NKG2D, an activating receptor for HLA class I-related stress-induced ligands like MICA/B and UL-16 binding proteins (ULBP) 1–6.Citation1,Citation10 Taken together, Vδ2 T cells can be selectively activated by pyrophosphates (“phosphoantigens”, pAg), agonistic anti-BTN3A1 antibodies, and aminobisphosphonates, and tumor cell killing can be triggered by both the TCR and NKG2D.Citation11 The second most frequent human γδ T-cell subset expresses Vδ1, which can associate with various Vγ elements. While TCR ligands for Vδ1 (and other non-Vδ2) γδ T cells are much less defined, these cells also exert potent anti-tumor activity, which is mainly executed by activating NK receptors like NKp30 and NKp44.Citation1 Protocols for the preferential expansion of such Delta One T (“DOT”) cells have been reported,Citation12 and both Vδ2 and Vδ1/DOT T cells are in development for clinical application.Citation11,Citation13 Currently, different strategies are pursued along this line, including in vivo activation of γδ T cells with bispecific γδ T-cell engagers or agonistic anti-BTN3A1 antibodies, and the adoptive transfer of in vitro expanded Vδ2 T cells, Vδ1/DOT cells, or chimeric antigen receptor (CAR)-modified γδ T cells.Citation11,Citation13
A challenge for any kind of cell-based cancer immunotherapy is the immunosuppressive tumor microenvironment. There is a multitude of mechanisms exerted by tumor cells, stroma cells, tumor-associated macrophages, and other cells to negatively impact on tumor-reactive T cells including γδ T cells.Citation14 One strategy to potentially counteract the immunosuppressive micromilieu is to stimulate innate immune mechanisms through activation of receptors like Toll-like receptors (TLR) or stimulator of interferon genes (STING).Citation15 The cyclic GMP-AMP synthase (cGAS)/STING pathway is a sensor of cytosolic DNA, which activates type I interferon responses via phosphorylation of TBK1 and IRF3, but also initiates pro-inflammatory cytokines through activation of NF-κB.Citation16 The cGAS/STING pathway can promote anti-tumor immunity by various mechanisms including induction of apoptosis in some tumor cells and modulation of innate and adaptive immunity.Citation17–29 As a consequence, application of agonistic STING ligands might synergize with chemo-/radiotherapy, tumor vaccines, checkpoint inhibitors, or adoptive immune cell therapy including CAR T cells.Citation15,Citation30 The prototypic agonists of STING are cyclic dinucleotides (CDN) like 2ʹ3ʹ-cGAMP that are generated upon binding of dsDNA to cGAS.Citation31 Uptake of CDN is promoted by specific transporter molecules.Citation32,Citation33 Recently, two classes of potent non-CDN STING ligands have been described, both of which can enter cells without a specific transporter.Citation34,Citation35 The adjuvant activity of such non-CDN STING ligands for enhancing anti-tumor immunity is currently under investigation in multiple settings.Citation19,Citation34–36
In the present study, we have investigated the effects of both CDN and novel non-CDN STING ligands on the activation and effector functions of human Vδ2 T cells. Our results reveal a critical role of monocytes in the modulation of γδ T-cell activation by STING ligands and a tumor cell type specific modulation of tumor cell killing. We discuss the relevance of our observations for the optimization of γδ T-cell-based cancer immunotherapies.
Materials and methods
Isolation of immune cell populations
Ficoll-Hypaque density gradient centrifugation was used to isolate peripheral blood mononuclear cells (PBMC) from leukocyte concentrates of healthy adult blood donors obtained from the Institute of Transfusion Medicine, University Hospital Schleswig-Holstein. The use of leukocyte concentrates and peripheral blood from healthy donors was approved by the Ethics committee of Kiel University Medical Faculty (code D546/16). Written informed consent was obtained from all donors, and the research was conducted in accordance with the Declaration of Helsinki. γδ T cells were purified from PBMC by positive magnetic selection using the anti-TCRγ/δ Microbead Kit from Miltenyi Biotec (Bergisch Gladbach, Germany) following the instructions of the company. To increase the purity of γδ T cells, two consecutive LS columns were applied. The purity of isolated γδ T cells was > 97%. Monocytes were isolated from PBMC by negative isolation using the Pan Monocyte Isolation Kit following the instructions of the company (Miltenyi Biotech). Negatively isolated monocytes contained >92% CD14+ monocytes and <0.5% contaminating CD3+ T cells.
Cell cultures. PBMC and purified γδ T cells with or without added purified monocytes were cultured in 96-well round bottom microtiter plates as detailed in the Results section. Complete culture medium was RPMI 1640 (Thermo Fisher Scientific) supplemented with antibiotics (100 U/mL penicillin, 100 µg/mL streptomycin) and 10% of heat-inactivated low endotoxin fetal bovine serum (Bio&Sell, Feucht, Germany). All cell cultures were incubated at 37° C in a humidified atmosphere of 5% CO2. Stock solutions of STING agonists and inhibitors were prepared as recommended by manufacturers and were stored at −20°C until use. STING ligands 2ʹ3ʹ-cGAMP (cGAMP), 2ʹ3ʹ-cGAMP control, c-di-AMP, cAIMP, 2ʹ3ʹ-c-di-AM(PS)2 (Rp,Rp), c-di-GMP, c-di-IMP, the inactive control c-di-UMP, and TBK1 inhibitor BX795 were obtained from Invivogen (Toulouse, France), non-dinucleotide STING ligands diABZI and MSA-2 were from MedChemExpress (Biozol, Eching, Germany). Zoledronate (ZOL) and recombinant human IL-2 (Proleukin) were kindly provided by Novartis (Basel, Switzerland), (E)-4-Hydroxy-3-methyl-but-2-enyl pyrophosphate (HMBPP) was purchased from Echelon Biosciences (Salt Lake City, UT, USA). Final concentrations of ZOL and HMBPP were 2.5 μM and 1 nM or 10 nM, respectively. In some experiments, purified γδ T cells were cultured in microtiter plates coated with 0.5 µg anti-CD3 mAb OKT3 (Biolegend, San Diego, CA, USA) per well. Where indicated, cell cultures were supplemented with IL-2 (50 IU/mL). Short-term Vδ2 T-cell lines were established from PBMC as previously reported.Citation37 Briefly, PBMC were stimulated with 2.5 μM ZOL and 50 IU/mL IL-2. IL-2 was added every other day, and cell cultures were split every 2–3 days starting at day 6. Following this protocol, the purity of γδ T-cell lines after 14 d was routinely > 92%. For intracellular analysis of cytokine expression, 400.000 PBMC or 100.000 purified γδ T cells with or without additional 100.000 monocytes per well were cultured for 24 h in round-bottom microtiter plates in the presence or absence of STING ligands and HMBPP (without exogenous IL-2). 3 μM monensin was added during the last 4 h to prevent cytokine secretion.
Tumor cell lines
The glioblastoma line U251MG (ECACC 89081403) was obtained from the European Collection of Authenticated Cell Cultures (ECACC, Salisbury, UK). Pancreatic ductal adenocarcinoma (PDAC) cell lines Panc89, PancTu-1 and BxPC3Citation38 were provided by Dr. Christian Röder, Institute for Experimental Cancer Research (UKSH Kiel, Germany). Tumor cell lines were maintained in a complete culture medium, and 0.05% trypsin/0.02% EDTA was used to detach adherent cell lines from flasks. The identity of cell lines had been verified by short tandem repeats analysis before freezing multiple aliquots, which were used for 8 weeks. All cell lines were regularly screened for mycoplasma contamination by RT-PCR (Venor® GeM classic, Minerva Biolabs GmbH, Germany) and were found to be negative. THP1-Dual KI-hSTING-A162 cells were obtained from Invivogen.
Immunoblot analysis of STING activation
Tumor cell lines and short-term expanded Vδ2 T-cell lines were activated for 4 h with STING ligands diABZI (100 nM) or MSA-2 (25 µM). Cell lysates were prepared and subjected to Western blot analysis. Membranes were sequentially probed with monoclonal rabbit antibodies against phospho-TBK1 (#5481), phospho-IRF-3 (#37829), TBK1 (#3504), and IRF-3 (#11904) obtained from Cell Signaling Technology Europe (Frankfurt, Germany), monoclonal mouse anti-STING antibody (#675902) from Biolegend, or polyclonal goat anti-β-actin antibody (#sc-1615) from Santa Cruz Biotechnology (Dallas, TX, USA). ECL reagents (GE Healthcare) were used for chemiluminescence detection using Hyper Film (GE Healthcare). Samples for the detection of phospho-TBK1/TBK1, phospho-IRF3/IRF3, and STING/β-actin were run on separate gels.
Cytotoxicity assay by Real-Time Cell Analyzer
Cytotoxic effector activity of short-term expanded γδ T-cell lines against U251MG and BxPC3 target cells was determined by Real-Time Cell Analyzer (RTCA, X-Celligence, ACEA, San Diego, CA, USA) which measures the decrease of impedance of adherent tumor cells over extended time periods as a correlate of cell lysis. RTCA was performed as previously described.Citation39 Briefly, 104 tumor cells in complete medium were added to each well of the micro-E plates. After overnight incubation, γδ effector T cells were added at an effector/target ratio of 10:1 or 2:1 with or without STING ligands and/or HMBPP as a positive control to enhance TCR-dependent lysis. The impedance of the cells was recorded via electronic sensors on the bottom of the 96-well micro-E-plate every 3 min for up to 48 h. Results were analyzed with RTCA software (version 2.0.0.1301, ACEA Biosciences Inc.) and normalized as described.Citation39 Results of several experiments with different γδ T-cell lines are summarized as percentage of cell death induced by γδ T-cells at various time points in relation to the corresponding tumor cell index in medium and in Triton-X100 (maximal lysis). Time point zero was defined as first measurement after addition of γδ T cells.
Flow cytometry
The following mAb were obtained from BD Biosciences (Heidelberg, Germany): anti-CD3-PE/APC/BV605 (clone SK7), anti-CD14-FITC/APC (clone MoP9), anti-IFN-γ-PE or -PE/Cy7 (clone 4S.B3), anti-TNF-α-PE (clone MAb 11). Anti-STING-AF488 (clone 723505) was purchased from R&D Systems (Wiesbaden, Germany), anti-Vδ2-FITC (clone IMMU389) was obtained from Beckman Coulter (Krefeld, Germany), rabbit monoclonal pTBK1-PE (#13498S) from Cell Signaling Technology. For cell surface staining, 3 × 105 cells were washed, stained in V-bottom microtiter plates for 20 min on ice with mAb, washed twice, and resuspended in 1% paraformaldehyde. For intracellular staining, cells were washed with staining buffer and permeabilized using Cytofix/Cytoperm kit (BD Biosciences) before staining with fluorochrome-conjugated mAb. Staining for intracellular detection of pTBK1 in response to STING ligand stimulation was performed using the BD transcription factor buffer set. All analyses were measured on a FACS-Canto or LSR-Fortessa cytometer (BD Biosciences), using DIVA (Data-Interpolating Variational Analysis) for acquisition and FlowJo™ v10.6.1 for data analysis.
Determination of γδ T-cell expansion
Absolute numbers of viable Vδ2 T cells per microculture well were measured after 7 d by a flow cytometry-based method termed standard cell dilution assay (SCDA) as described previously.Citation40 Briefly, cultured cells from 96-well round-bottom plates were washed and stained with anti-Vδ2-FITC mAb. After one washing step, cells were resuspended in sample buffer containing a defined number of fixed standard cells and 0.2 μg/ml propidium iodide (PI). Standard cells were purified CD4 T cells that had been stained with APC-labeled antibodies, and thereafter had been fixed in 1% paraformaldehyde. Based on the known number of standard cells (FITC−PI+APC+), the absolute number of viable Vδ2 T cells (FITC+PI−) in a given microculture well was determined as described previously.Citation40 The gating strategy used in SCDA is illustrated in Suppl. Fig. S1a. The expansion rate was calculated by dividing the number of Vδ2 T cells determined by SCDA after 7 d of culture by the number of Vδ2 T cells calculated to be present within the starting PBMC responder cell population (Suppl. Fig. S1b).
Cell death analysis
Cell death of monocytes was measured using the LIVE/DEAD™ Fixable Red Dead Cell Stain Kit (Thermo Fisher Scientific). Cell death of γδ T-cell lines was measured by flow cytometry following combined annexin V-FITC (Mabtag, Friesoythe, Germany) and PI staining. In some experiments, neutralizing anti-IFN-β IgG (Invivogen) was added at 5 µg/ml during the 4 h incubation of γδ T-cell lines.
Measurement of cytokines in cell culture supernatants
IFN-γ, granzyme B (GrB), IFN-β, IL-1β, and CXCL-10/IP-10 were quantified in cell culture supernatants by ELISA with the respective DuoSet ELISA Kits from R&D Systems following the manufacturer’s instructions. In each experimental setting, two replicates were included.
Statistical analysis
Statistical comparisons were made between groups using one- or two-way ANOVA analysis and Dunnett’s multiple-comparison test against respective internal controls. All analyses were done with the Graphpad Prism version 8 software. Levels of significance were set as * p < .05, ** p < .01, *** p < .001 and **** p < .0001.
Results
STING ligands inhibit expansion but co-stimulate cytokine induction in γδ T cells within PBMC
In an initial set of experiments, we investigated how STING ligands modulate the activation of Vδ2 γδ T cells within PBMC at the level of cellular expansion and cytokine induction. We used predetermined optimal concentrations of ZOL and HMBPP for selective activation of Vδ2 T cells.Citation6 ZOL requires the presence of monocytes, which then generate the pAg IPP,Citation9 while HMBPP acts through direct binding to BTN3A1/2A1 molecules and does not absolutely depend on presence of monocytes.Citation5,Citation6 First, we analyzed cellular expansion in the absence or the presence of CDN and non-CDN STING ligands by measuring the absolute number of viable Vδ2 T cells after seven days by flow cytometry. We first performed dose-titration experiments of non-CDN ligands MSA-2 and diABZI (Suppl. Fig. S2a). The selected concentrations used in further experiments (25 µM MSA-2 and 100 nM diABZI) are in the range of reported EC50 concentrations.Citation34,Citation35 As expected, both HMBPP and ZOL induced strong expansion of CD3+Vδ2+ T cells as shown in the representative dot plot in . STING ligands including both CDN ligand cGAMP and non-CDN ligands MSA-2 and diABZI strongly inhibited proliferation of Vδ2 T cells in response to ZOL, while the cGAMP control ligand was inactive (). Vδ2 T-cell proliferation stimulated by pAg HMBPP was also significantly inhibited, even though to somewhat lower levels compared to ZOL (). The strong inhibition of ZOL-stimulated γδ T-cell expansion was also observed with additional potent CDN STING ligands including cAIMP, Rp,Rp, and c-di-AMP, but not with the much less active non-adenosine CDN STING ligands (c-di-GMP, c-di-IMP) and the inactive control ligand c-di-UMP (Suppl. Fig. S2b). Next, we analyzed IFN-γ and TNF-α induction in Vδ2 T cells within PBMC activated for 24 h with STING ligands in the absence or presence of HMBPP. Again, we first performed dose titration experiments to define optimal concentrations of non-CDN STING ligands (Suppl. Fig. S3a) which led us to use 25 µM MSA-2 and 100 nM diABZI in all subsequent experiments. The gating strategy for analysis of cytokine expression in CD3+Vδ2+ T cells among activated PBMC is displayed in Suppl. Fig. S3b. Representative histograms are displayed for IFN-γ in and for TNF-α in , and a summary of four experiments is shown for IFN-γ in and for TNF-α in . Interestingly, the non-CDN ligands MSA-2 and diABZI induced significant IFN-γ and TNF-α expression in Vδ2 T cells in the absence of HMBPP (/d, left-bar diagrams), whereas cGAMP was largely ineffective on its own. While all three STING ligands significantly co-stimulated IFN-γ induction in the presence of the TCR stimulus HMBPP (, right bar graph), TNF-α expression was again co-stimulated by MSA-2 and diABZI but not by cGAMP (, right bar graph).
Figure 1. Inhibition of Vδ2 T-cell expansion by active STING ligands. 1.5 × 105 PBMC per well obtained from healthy donors were stimulated with 10 nM HMBPP or 2.5 µM Zoledronate in the presence of 50 IU/mL IL-2 and absence or presence of 25 µM MSA-2, 100 nM diABZI, or 10 µg/mL cGAMP/cGAMP control. All cultures were set up in duplicates. After 7 d, absolute numbers of viable Vδ2 T cells per well were quantified by standard cell dilution assay (SCDA). (a) Representative dot plots of CD3+Vδ2+ T cells after 7 d of activation of PBMC with HMBPP (middle), Zoledronate (right), or medium only (left). Absolute numbers of viable Vδ2 T cells following activation of PBMC with HMBPP (b) or Zoledronate (c) in the absence or presence of STING ligands. Mean ± SD of eight to 12 independent experiments are shown. Statistical significance: *** p < .001; ns, not significant.
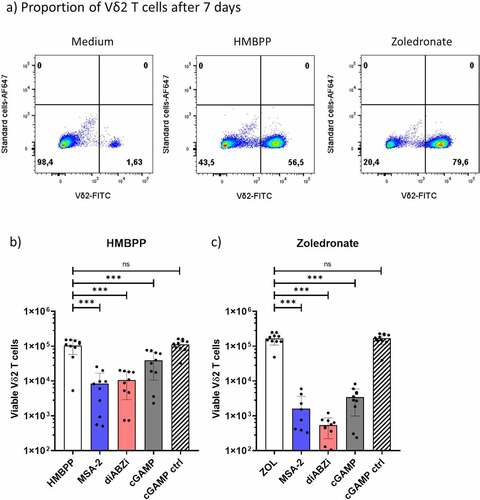
Figure 2. Co-stimulation of cytokine induction in Vδ2 T-cells within PBMC. 4 × 105 PBMC obtained from healthy donors were cultured in the presence of 25 µM MSA-2, 100 nM diABZI or 10 µg/mL cGAMP/cGAMP control in medium or in the additional presence of 10 nM HMBPP. After 24 h, monensin was added for 3 h before surface staining for CD3 and Vδ2 and intracellular staining for IFN-γ (a,b) or TNF-α (c,d). For analysis, a gate was set on CD3+Vδ2+ cells, and 2.000 events were acquired. (a) and (c) representative histograms of one experiment. Black histogram: isotype control; colored histograms in the absence (blue) or presence (red) of HMBPP: IFN-γ (a), TNF-α (c). (b) and (d) Mean ± SD of four experiments for IFN-γ (b) and TNF-α (d). Statistical significance: * p < .05, ** p < .01, *** p < .001; ns, not significant.
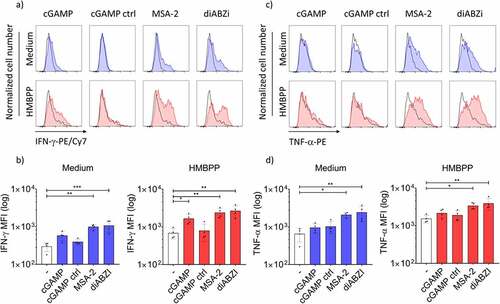
Role of monocytes
In mixed cell populations like PBMC, effects of STING ligands on γδ T-cell activation might depend on accessory cells such as monocytes which play a crucial role in γδ T-cell activation.Citation6,Citation9 Therefore, we analyzed co-stimulatory effects of STING ligands on purified γδ T cells in the absence or presence of monocytes. We observed that purified γδ T cells expressed STING at low levels but comparable to monocytes, as shown by flow cytometric analysis (Suppl. Fig. S4a). Next, we asked if STING can be directly activated in γδ T cells by analyzing TBK1 phosphorylation in response to STING ligands and TCR activation with HMBPP or anti-CD3 mAb. To this end, purified γδ T cells were stimulated for 4 h with STING ligands MSA-2 or diABZI and/or HMBPP or anti-CD3 mAb. Thereafter, pTBK1 was analyzed by flow cytometry. Under these conditions, we observed little if any TBK1 phosphorylation upon HMBPP stimulation but slightly more upon anti-CD3 mAb activation (Suppl. Fig. S5a/b/c) while there was no TBK1 phosphorylation in response to MSA-2 or diABZI alone (Suppl. Fig. S5c, left bar graph). TBK1 phosphorylation in response to HMBPP or anti-CD3 mAb activation was strongly inhibited in the presence of the specific TBK1 inhibitor BX795 (Suppl. Fig. S5c). We went on to analyze IFN-γ induction in purified γδ T cells stimulated with HMBPP in the absence or presence of monocytes and STING ligands MSA-2 or diABZI. IFN-γ in 24 h cell culture supernatants was quantified by ELISA. As presented in , HMBPP in the absence of monocytes stimulated some IFN-γ secretion. In line with the lack of STING activation in freshly isolated γδ T cells as revealed by pTBK1 analysis (Suppl. Fig. S5), the IFN-γ secretion was not modulated, neither by MSA-2 nor by diABZI. As expected, HMBPP stimulated much higher levels of IFN-γ in the presence of added monocytes (, right bar graph). Under these conditions, both STING ligands significantly co-stimulated IFN-γ secretion (mean values: diABZI 2.360 pg/mL, MSA-2 1.733 pg/mL, medium 1.027 pg/mL), in agreement with the results obtained with PBMC ().
Figure 3. Role of monocytes in γδ T-cell co-stimulation. a) 105 purified γδ T cells per microculture well were activated with 10 nM HMBPP in the absence or presence of 105 purified monocytes and additional absence or presence of MSA-2 or diABZI as indicated. All cultures were set up in duplicates. After 24 h, cell culture supernatants were collected, and IFN-γ was quantified by ELISA. Mean ± SD of four individual experiments. Note that values on the Y-axis are displayed on a logarithmic scale. b,c,d) 105 purified monocytes were stimulated for 24 h with LPS, diABZI, MSA-2, or cGAMP/cGAMP control. All cultures were set up in duplicates. IL-1β (b), IP-10 (c) and IFN-γ (d) in cell culture supernatants were quantified by ELISA. Mean ± SD of five (IP-10), six (IL-1β) or three (IFN-γ) experiments are shown. e) Cell death induction in purified monocytes by STING ligands. 105 purified monocytes were stimulated for indicated time periods with STING ligands. Cell death was determined by LIVE/DEAD™ Fixable Red Dead Cell Stain Kit. Mean ± SD of three independent experiments. Ligand concentrations in all experiments were 25 µM MSA-2, 100 nM diABZI, 10 µg/mL cGAMP/cGAMP control, and 100 ng/mL LPS. Statistical significance: * p < .05, ** p < .01, *** p < .001 and **** p < .0001. ns, not significant.
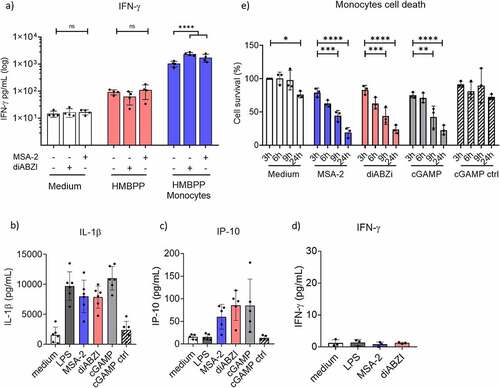
We previously reported that IL-1β plays a role in monocyte-dependent IFN-γ induction in Vδ2 T cells triggered by TLR8 ligands.Citation41 As shown in , active (but not inactive cGAMP control) STING ligands also potently stimulated IL-1β secretion in monocytes. Evidence for STING activation in monocytes is demonstrated by secretion of IFN type-I pathway-dependent IP-10 in response to STING ligands but not LPS (). On the other hand, purified monocytes did not secrete any IFN-γ under our experimental conditions (), thus excluding a potential contribution of monocytes to the secretion of IFN-γ in the γδ T-cell/monocyte co-cultures shown in . Secretion of mature IL-1β requires processing of pro-IL-1β by caspase-1 associated with cell death induction by pyroptosis.Citation42 Accordingly, the active STING ligands MSA-2, diABZI and cGAMP, but not cGAMP control, induced massive cell death in purified monocytes, starting as early as 3 h after stimulation and culminating after 24 h (). Taken together, STING ligands induce IL-1β secretion and rapid cell death in monocytes which might contribute to early cytokine co-stimulation but also to growth inhibition in Vδ2 T cells among PBMC.
STING ligands co-stimulate cytokine induction and induce cell death in activated γδ T cells
We observed strong STING expression in Vδ2 T-cell lines expanded for 14 d with ZOL and IL-2 (Suppl. Fig. S4b and ). In contrast to resting γδ T cells, MSA-2 and diABZI directly activated STING in ZOL-expanded γδ T-cell lines, as demonstrated by Western blot analysis for TBK1 and IRF3 phosphorylation (), and for TBK1 phosphorylation also by flow cytometry (). The decreased levels of STING expression at 37 kDa and corresponding increased levels of higher MW (around 75 kDa) expression upon ligand activation reflects STING dimerization.Citation43,Citation44 In line with direct activation of STING in expanded γδ T cells, we detected small amounts of IFN-β in supernatants of 4 h activated γδ T cells but not after 24 h (). In contrast, we did not detect IFN-γ, GrB, IP-10, or IL-1β in the supernatants of expanded γδ T-cell lines stimulated for 24 h with STING ligands MSA-2 or diABZI only (not shown). However, contrary to purified resting γδ T cells, both STING ligands did co-stimulate IFN-γ and GrB secretion () in such Vδ2 T-cell lines in the presence of HMBPP. Interestingly, the anti-CD3 mAb-stimulated IFN-γ and GrB secretion was even reduced in the presence of STING ligands when compared to the medium controls set as 100% (). However, it should be noted that in the absence of STING ligands, anti-CD3 mAb stimulated much higher amounts of IFN-γ and GrB secretion compared to HMBPP (mean values HMBPP: IFN-γ 363 pg/mL, GrB 1.695 pg/mL; mean values anti-CD3: IFN-γ 1.741 pg/mL, GrB 8.650 pg/mL). While MSA-2 and diABZI co-stimulated cytokine secretion as detected in cell culture supernatants, both STING ligands induced massive cell death in the activated γδ T-cell lines as revealed by flow cytometric analysis of Annexin V+/PI+ cells (/g).
Figure 4. STING activation, co-stimulation and cell death induction in short-term expanded Vδ2 T-cell lines. Short-term γδ T cell lines expanded for 14 d contained ≥ 92% CD3+Vδ2+ γδ T-cells. a) Western blot analysis of STING expression and TBK1/IRF3 phosphorylation in response to STING ligands. Vδ2 T-cell lines were activated for 4 h with STING ligands and subsequently processed for Western blot analysis. Results with three different Vδ2 T-cell lines are shown. b) pTBK1 analysis by flow cytometry, same conditions as in (a). Left: representative histogram: MSA-2 (blue), diABZI (red); dotted histogram: medium control. Right graph: mean ± SD of four experiments. c) IFN-β secretion by STING ligand-activated Vδ2 T cells. Vδ2 T-cell lines were activated with MSA-2 or diABZI for 4 h (left) and 24 h (right). IFN-β in cell culture supernatants was measured by ELISA. Mean ± SD of three experiments. d) Vδ2 T-cell lines were cultured in medium or presence of 10 nM HMBPP or immobilized anti-CD3 mAb, and additional presence of absence of MSA-2 or diABZI. Supernatants were collected after 24 h and analyzed by ELISA for IFN-γ and GrB. Mean ± SD of six experiments. e,f) Vδ2 T-cell lines were cultured for 24 h in medium or with MSA-2 or diABZI as indicated. Cell death was analyzed by Annexin V/PI staining and FACS analysis. e) Representative dot plot of Annexin V-FITC and PI staining. f) Mean ± SD of five experiments. Ligand concentrations in all experiments were 25 µM MSA-2 and 100 nM diABZI. Statistical significance: * p < .05, ** p < .01, *** p < .001 and **** p < .0001.
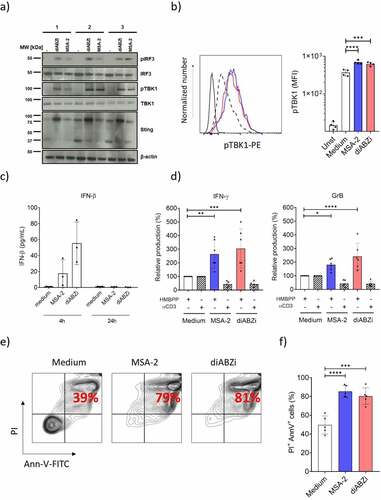
Modulation of the tumor cell/γδ T-cell interaction by STING ligands
In the next step, we investigated the effects of STING ligands on three different PDAC cells (Panc89, BxPC3, PancTU-1) and one glioblastoma (U251MG) line. In contrast to the three other tumor cell lines, PancTU-1 expressed very low levels of STING as revealed by Western blot analysis (). We investigated the direct responsiveness to STING ligands by analyzing phosphorylation of key signaling molecules (IRF3, TBK1) and secretion of IP-10. Panc89 and BxPC3 responded well to MSA-2 and diABZI as revealed by phosphorylation of IRF3 (Western blot, ) and TBK1 (Western blot, ; flow cytometry, ), and secretion of IP-10 (). STING activation in U251MG was less pronounced in all three read-out systems, while PancTU-1 were almost unresponsive, in line with their faint STING expression. We did not detect IFN-α and/or IFN-β secretion in any of the tumor cell lines (not shown). The STING ligands differentially modulated the proliferation as analyzed in the RTCA system. Tumor cells were treated for 3 h with ligands, washed, and then plated on RTCA plates. After 24 h, Triton-X was added to some wells to induce maximum killing, and proliferation was monitored over a total of 48 h. As shown in , MSA-2 and diABZI slightly delayed the growth of U251MG and BxPC3 cells but not of Panc89 and PancTU-1. Next, we investigated how STING ligands modulate the killing of tumor cells when added together with γδ T effector cells. The result of a representative RTCA graph with BxPC3 target cells is shown in . It illustrates that there was increased tumor cell death (i.e., reduced impedance) at later time points in the presence of STING ligands. A summary of six experiments with different short-term expanded Vδ2 T-cell lines at E/T ratio of 10:1 and cell death analyzed by RTCA at 6 and 24 h time points is presented in . At the early time point of 6 h, we noticed only moderate effects of the added STING ligands. However, when analyzed after 24 h, the presence of all three STING ligands significantly increased the killing of BxPC3. In the presence of pAg HMBPP, killing was strongly increased, both at 6 and 24 h. The increased lysis in the presence of HMBPP was not modulated by STING ligands. Tumor cell lines differ intrinsically in their susceptibility to γδ T-cell killing. While PDAC including BxPC3 are moderately sensitive, some glioblastoma lines like U251MG are much more sensitive. Using the same high E/T ratio of 10:1, U251MG target cells were in fact more efficiently killed than BxPC3 already after 6 h, which was further enhanced to complete lysis (as expected) in the presence of HMBPP (). Killing of U251MG cells further increased at 24 h and 48 h, but there were only moderate effects of MSA-2 and diABZI at the 10:1 E/T ratio. To better dissect potential effects of STING ligands, we also performed RTCA assays with U251MG target cells at low E/T ratio of 2:1. Lysis of U251MG target cells by Vδ2 T-cell lines in the absence of STING ligands or HMBPP gradually increased over time, reaching up to 50% after 48 h (). The presence of diABZI further increased lysis at 24 and 48 h. Interestingly, at the early time points of 6 and 24 h, both MSA-2 and diABZI STING ligands significantly reduced the enhancing effect of pAg HMBPP ().
Figure 5. STING activation in tumor cell lines. PDAC tumor cell lines Panc89, BxPC3, PancTU-1, and glioblastoma line U251MG were activated with STING ligands (25 µM MSA-2, 100 nM diABZI, 10 µg/mL cGAMP/cGAMP ctrl). a) Western blot analysis of STING expression and IRF3/TBK1 phosphorylation after 4 h of activation. b) Flow cytometry analysis of TBK1 phosphorylation (pTBK1) was measured after 4 h stimulation with MSA-2 (blue histograms), diABZI (red histograms) or medium (black histograms). Results of one out of three similar experiments are shown. c) IP-10 production by tumor cells in response to STING ligands. 104 tumor cells were cultured in duplicates in flat bottom microtiter plates in the absence or presence of STING ligands as indicated. After 24 h, cell culture supernatants were collected and analyzed for IP-10 by ELISA. Mean values of two independent experiments are shown. d) Tumor cell lines were pre-incubated for 3 h with the indicated ligands, washed, and plated on RTCA plates (104 cells per well). The impedance was recorded over 48 h. After 24 h, Triton X-100 was added as indicated.
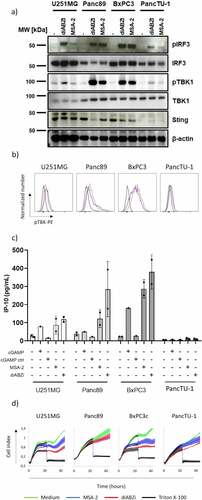
Figure 6. Enhancement of γδ T-cell killing of BxPC3 tumor cells by STING ligands. 104 BxPC3 tumor cells per well were plated in duplicates in RTCA plates and incubated overnight. Thereafter, 105 short-term expanded Vδ2 T cells were added per well in the absence or presence of 25 µM MSA-2, 100 nM diABZI or 10 µg/ml cGAMP, and the additional absence or presence of 1 nM HMBPP (E/T ratio 10:1). The impedance was recorded every 3 min for additional 24 h. Triton X-100 was added to induce maximal lysis. a) Representative RTCA graphs to illustrate effects of MSA-2, diABZI and cGAMP. b) The percentage of specific lysis at 6 and 24 h after addition of γδ T effector cells was calculated from the RTCA graph. Mean values of six independent experiments with different γδ T cells are shown. Statistical significance: * p < .05, ** p < .01, *** p < .001 and **** p < .0001. ns, not significant.
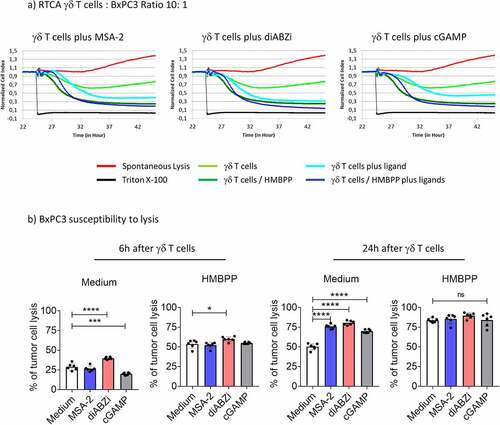
Figure 7. Modulation of γδ T-cell killing of U251MG tumor cells by STING ligands. 104 U251MG tumor cells per well were plated in duplicates in RTCA plates and incubated overnight. Thereafter, 105 (E/T ratio of 10:1) or 2 × 104 (E/T ratio of 2:1) short-term expanded Vδ2 T cells were added per well in the absence or presence of 25 µM MSA-2 or 100 nM diABZI, and the additional absence or presence of 1 nM HMBPP. The impedance was recorded every 3 min for additional 48 h. Triton X-100 was added to induce maximal lysis. The percentage of specific lysis at 6 h, 24 h and 48 h after addition of γδ T effector cells was calculated from the RTCA graph. a) E/T ratio of 10:1; b) E/T ratio of 2:1. Mean values of six independent experiments with different γδ T cells are shown. Statistical significance: * p < .05, ** p < .01, *** p < .001 and **** p < .0001. ns, not significant.
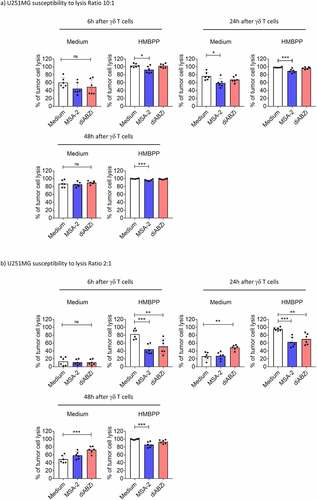
Discussion
γδ T cells are promising candidates for cellular cancer immunotherapy. Adoptive transfer of allogeneic γδ T cells expanded from healthy donors is safe and offers the perspective for off-the-shelf cellular products.Citation45 Other recent developments to harness γδ T cells for cancer immunotherapy include the design of bi-specific γδ T-cell engagers, the application of agonistic anti-BTN3A1 antibodies for in vivo activation of Vδ2 T cells, or the CAR modification of γδ T cells.Citation11,Citation13,Citation46 However, based on experience with other immunotherapies, it is expected that γδ T-cell immunotherapy will require additional strategies to enhance the efficacy and to counteract the immunosuppressive tumor microenvironment.Citation11,Citation14 Along this line, STING ligands have attracted much attention as they have been shown to promote anti-tumor activity at multiple levels, including direct induction of cell death in some tumors,Citation17,Citation23 modulation of tumor cell antigenicity,Citation24 synergistic action with cytokines, chemotherapeutic drugs and checkpoint inhibitors,Citation19,Citation21,Citation25,Citation26 and modulation of the tumor microenvironment to enhance CD8 T-cell immunity.Citation18,Citation22,Citation27,Citation47 The recent discovery of potent non-CDN ligands which circumvent the poor bio-availability of therapeutically administered CDN STING ligands has even further spurred the interest.Citation35,Citation36
In our studies, CDN as well as the potent non-CDN STING ligands MSA-2 and diABZI strongly inhibited the proliferative expansion of Vδ2 T cells when PBMC were activated by ZOL or HMBPP in the presence of exogenous IL-2. Simultaneously, the STING ligands co-stimulated IFN-γ and TNF-α production in Vδ2 T cells within PBMC when analyzed at 24 h after activation. Growth arrest of pAg-activated human γδ T cells despite CD69 up-regulation triggered by CDN STING ligands has been previously reported by Tosolini et al.Citation48 In these studies, only adenosine-containing CDN exerted this inhibitory effect that was related to monocyte death induced by the adenosine receptor A2a expressed on the surface of monocytes.Citation48,Citation49 We now demonstrate that the growth-inhibitory effect of STING ligands on human γδ T-cell expansion is not limited to CDN but is at least as strong with diABZI and MSA-2, the two newly developed non-CDN STING ligands. Similar to CDN, diABZI and MSA-2 potently stimulate IP-10 and inflammasome activation (i.e., strong IL-1β production) in monocytes but simultaneously induce massive cell death which, however, is unrelated to A2a receptor expression on monocytes. When we analyzed freshly isolated γδ T cells, MSA-2 and diABZI co-stimulated IFN-γ secretion in response to HMBPP only in the presence of monocytes. In such co-cultures, it was important to exclude a potential contribution of monocytes to the measured IFN-γ levels, since monocytes have been reported to produce IFN-γ under certain conditions.Citation50,Citation51 However, we failed to detect any IFN-γ in supernatants of STING ligand-activated monocytes which contained expected levels of IL-1β and IP-10 (). In contrast to freshly isolated γδ T cells, co-stimulation of IFN-γ and GrB secretion in short-term expanded Vδ2 T-cell lines was observed also in the absence of added monocytes. Therefore, it appears that the responsiveness to STING ligands differs between resting and activated γδ T cells even though both express the STING receptor.
While STING is commonly considered a critical innate sensor, it is also active in T cells beyond in vitro expanded γδ T cells as shown here. However, the overall effect of STING ligands on T-cell activation depends on parameters like the quality and signal strength of the TCR stimulus.Citation52 So far, such studies have been mainly performed with the CDN cGAMP and the non-CDN DMXAA, which only works in mice. Activation of STING inhibits T-cell proliferation in response to anti-CD3/anti-CD28 activation in an mTORC1-dependent manner.Citation29,Citation43,Citation53 Under appropriate conditions, STING ligands including tumor-intrinsic STING ligands also induce apoptosis in murine and human T cells.Citation54–56 In line with these reports, we also noticed massive cell death of short-term expanded Vδ2 T-cell lines in the presence of MSA-2 or diABZI. Importantly, we could demonstrate direct activation of STING in expanded γδ T cells as revealed by TBK1 and IRF3 phosphorylation. Furthermore, we also detected IFN-β in supernatants of STING-activated Vδ2 T-cell lines at early (4 h) but not late (24 h) time points. This is the first demonstration that human γδ T cells can actually secrete type I IFN. We assume that the IFN-β secreted by STING-activated γδ T cells is used in an autocrine fashion, resulting in lack of IFN-β in 24 h supernatants. Since type I IFN has been implicated in T-cell apoptosis,Citation57,Citation58 we also analyzed cell death induced in Vδ2 T-cell lines by diABZI in the presence of neutralizing anti-IFN-β antibody, which, however, did not prevent cell death (not shown). Cell death was also not significantly prevented in the presence of the pan-caspase inhibitor Z-VAD-FMK (not shown). Therefore, the molecular characterization of cell death pathways triggered in human γδ T cells by STING ligands will require further investigation. Overall, the results of our in vitro studies with human γδ T cells and novel non-CDN STING ligands are largely in line with the available evidence that STING mediates mainly inhibitory signals in T cells leading to growth arrest or cell death while simultaneously (co-)stimulating cytokine production.
In view of the potential use of STING ligands to boost γδ T-cell immunotherapy, we put a focus in our study on the modulation of tumor cell killing by γδ T cells. Epithelial cancer cell lines (glioblastoma U251MG, PDAC cells Panc89, BxPC3, PancTU-1) expressed variable levels of STING and responded differentially to STING activation as revealed by TBK1 and IRF3 phosphorylation, and IP-10 secretion. Notably, none of the tumor cell lines secreted type I IFN in response to STING ligands, which is in line with a previous analysis of various epithelial cancer cell lines.Citation59 We observed that MSA-2 and diABZI had a direct effect on U251MG and BxPC3 cells by slightly retarding their growth kinetics as revealed in RTCA, without inducing cell death (not shown). However, there was no correlation between growth retardation, TBK1/IRF3 phosphorylation, and IP-10 secretion. Thus, Panc89 cells showed stronger TBK1 phosphorylation and IP-10 secretion but little if any effect on growth patterns in response to diABZI in comparison to U251MG, whereas the strong STING activation correlated with growth retardation in BxPC3 cells. On the other hand, PancTU-1 expressed low levels of STING and showed hardly any functional response to the STING ligands. These results with only a limited number of different cancer cell lines underscore the variability of transformed cells with respect to the activation of the tumor-intrinsic STING pathway, spanning from no response to growth arrest, modulation of antigenicity, and cell death induction.Citation17,Citation19,Citation23,Citation24,Citation60 This heterogeneity of consequences of tumor-intrinsic STING activation is certainly one of the parameters to be considered in promoting STING agonists as cancer therapeutics.Citation54,Citation61
The modulation of γδ T-cell mediated tumor cell killing by STING ligands was investigated with U251MG and BxPC3 target cells that responded to STING activation as discussed above. The RTCA system allowed us to monitor tumor cell lysis over extended time periods () but also to calculate the percentage of specific lysis at selected time points. At the E/T ratio of 10:1, the two tumor targets differed substantially in their susceptibility. Strong killing of U251MG was recorded already after 6 h, and was further enhanced after 24 h and 48 h. The additional TCR activation by HMBPP further increased killing to almost complete lysis already after 6 h, whereas the addition of STING ligands only moderately modified but tended to slightly reduce tumor cell killing at 6 h and 24 h. To dissect the STING effects further, we also used lower E/T ratio of 2:1 with U251MG target cells. Under these conditions, the STING ligands enhanced killing in the absence of HMBPP, but actually decreased lysis at the 6 h and 24 h time points in the presence of HMBPP. The advantage of the RTCA system is that the dynamics of the tumor target and effector cell interaction can be monitored over extended time periods as also shown here. While these results reveal a complex modulation of the killing of a γδ T-cell sensitive target cell by STING ligands, the underlying mechanisms need to be investigated in more detail in future studies.
In contrast to U251MG, STING ligands significantly enhanced the lysis of BxPC3 target cells at the E/T ratio of 10:1 after 24 h. Like with other PDAC cells, the susceptibility of BxPC3 to γδ T-cell killing per se is moderate but can be strongly enhanced by additional TCR activation with pAg or tumor-targeting bispecific antibodies.Citation62,Citation63 In line, HMBPP enhanced the lysis of BxPC3, but this was not further modulated by STING ligands. While only representative tumor target cells were analyzed here, our research nevertheless reveals the possible range of effects of novel and therapeutically applicable STING ligands on the modulation of tumor cell killing by Vδ2 γδ T cells. Our results indicate that STING ligands perhaps have limited capacity to enhance killing of cancer cells, which are intrinsically susceptible to γδ T cells, but might significantly enhance the effector activity of γδ T cells against less susceptible tumor cells in the absence of γδ T-cell selective (phospho)antigens.
We investigated effects of STING ligands on γδ T-cell activation and on tumor cell lysis, but did not address additional cellular components of the tumor microenvironment. From a therapeutical perspective, the intratumoral application of STING ligands is the most effective. Local injection of STING ligands can repolarize macrophagesCitation47 and is effective in murine tumor models also in various combination protocols.Citation64,Citation65 Given that such studies are on-going in patients with various tumors,Citation66,Citation67 the potential activation of intratumoral γδ T cells by local application of STING ligands is of great interest and needs to be addressed in future studies.
Our current study focused on human γδ T cells because of the ongoing efforts to apply γδ T cells to cancer immunotherapy.Citation11 We are aware of the limitations of such in vitro experiments. The role of γδ T cells in immune surveillance and control of tumor development has been convincingly demonstrated in mouse models, notably with γδ T-cell deficient TCRδ−/− mice.Citation68 Mice are amenable to genetic manipulation, and it will be informative to investigate the potential interplay of STING ligands with tumor development and γ∂ T-cell activation in suitable mouse tumor models. To this end, TCRδ−/− mice but also mice with selective inactivation of STING or IFNαR1 in γδ T cells will help to gain more mechanistic insights. This notwithstanding, it must be emphasized that no mouse model can adequately reflect the anti-tumor activity of human Vγ9Vδ2 γδ T cells because mice lack the equivalent of a phosphoantigen-recognizing γδ TCR.Citation1
Conclusions
Our results reveal the complexity of STING ligand effects on human γδ T cells, which (co)stimulate cytokine induction and enhance cytotoxicity in a tumor cell-selective way but inhibit γδ T-cell expansion and induce cell death in expanded γδ T cells. Taking this in consideration will help to define conditions where novel STING ligands will help to improve γδ T-cell immunotherapy.
Abbreviations
BTN | = | butyrophylin |
CDN | = | cyclic dinucleotide |
GrB | = | granzyme B |
HMBPP | = | (E)-4-Hydroxy-3-methyl-but-2-enyl pyrophosphate |
IFN | = | interferon |
IPP | = | isopentenyl pyrophosphate |
pAg | = | phosphoantigen |
PBMC | = | peripheral blood mononuclear cells |
PDAC | = | pancreatic ductal adenocarcinoma |
RTCA | = | Real Time Cell Analyzer |
SCDA | = | standard cell dilution assay |
STING | = | Stimulator of Interferon Genes |
TCR | = | T-cell receptor |
ZOL | = | Zoledronate |
Authors’ contributions
RS and DK designed and conceived the study; RS performed most of the experiments; MZ supported cell death analysis by flow cytometry; ML performed Western blot experiments; CP contributed to flow cytometry and RTCA analysis; DW supported RTCA experiments; DK and DW supervised the work; DK wrote the first draft, all authors contributed to the discussion of the manuscript.
Consent for publication
All authors have seen and approved the final manuscript.
Availability of data and material
Original data are available upon reasonable request. Only commercially available reagents were used throughout the study.
Supplemental Material
Download PDF (1.9 MB)Acknowledgments
We gratefully acknowledge the expert technical assistance of Monika Kunz.
Disclosure statement
No potential conflict of interest was reported by the author(s).
Supplementary material
Supplemental data for this article can be accessed on the publisher’s website
Additional information
Funding
References
- Silva-Santos B, Mensurado S, Coffelt SB. γδ T cells: pleiotropic immune effectors with therapeutic potential in cancer. Nat Rev Cancer. 2019 Jul;19(7):392–15. doi:10.1038/s41568-019-0153-5.
- Harly C, Guillaume Y, Nedellec S, Peigné CM, Mönkkönen H, Mönkkönen J, Li J, Kuball J, Adams EJ, Netzer S, et al. Key implication of CD277/butyrophilin-3 (BTN3A) in cellular stress sensing by a major human γδ T-cell subset. Blood. 2012 Sep 13;120(11):2269–2279. doi:10.1182/blood-2012-05-430470.
- Rigau M, Ostrouska S, Fulford TS, Johnson DN, Woods K, Ruan Z, McWilliam HEG, Hudson C, Tutuka C, Wheatley AK, et al. Butyrophilin 2A1 is essential for phosphoantigen reactivity by γδ T cells. Science. 2020 Feb 7;367(6478):eaay5516. doi:10.1126/science.aay5516.
- Karunakaran MM, Willcox CR, Salim M, Paletta D, Fichtner AS, Noll A, Starick L, Nöhren A, Begley CR, Berwick KA, et al. Butyrophilin-2A1 directly binds germline-encoded regions of the Vγ9Vδ2 TCR and is essential for phosphoantigen sensing. Immunity. 2020 Mar 17;52(3):487–498.e6. doi:10.1016/j.immuni.2020.02.014.
- Herrmann T, Fichtner AS, Karunakaran MM. An update on the molecular basis of phosphoantigen recognition by Vγ9Vδ2 T cells. Cells. 2020 Jun 9;9(6):1433. doi:10.3390/cells9061433.
- Nerdal PT, Peters C, Oberg HH, Zlatev H, Lettau M, Quabius ES, Sousa S, Gonnermann D, Auriola S, Olive D, et al. Butyrophilin 3A/CD277-dependent activation of human γδ T Cells: accessory cell capacity of distinct leukocyte populations. J Immunol. 2016 Oct 15;197(8):3059–3068. doi:10.4049/jimmunol.1600913.
- Gruenbacher G, Thurnher M. Mevalonate metabolism in immuno-oncology. Front Immunol. 2017 Dec 1;8:1714. doi:10.3389/fimmu.2017.01714.
- Gober HJ, Kistowska M, Angman L, Jenö P, Mori L, De Libero G. Human T cell receptor gammadelta cells recognize endogenous mevalonate metabolites in tumor cells. J Exp Med. 2003 Jan 20;197(2):163–168. doi:10.1084/jem.20021500.
- Roelofs AJ, Jauhiainen M, Mönkkönen H, Rogers MJ, Mönkkönen J, Thompson K. Peripheral blood monocytes are responsible for gammadelta T cell activation induced by zoledronic acid through accumulation of IPP/DMAPP. Br J Haematol. 2009 Jan;144(2):245–250. doi:10.1111/j.1365-2141.2008.07435.x.
- Chitadze G, Lettau M, Luecke S, Wang T, Janssen O, Fürst D, Mytilineos J, Wesch D, Oberg HH, Held-Feindt J, et al. NKG2D- and T-cell receptor-dependent lysis of malignant glioma cell lines by human γδ T cells: modulation by temozolomide and A disintegrin and metalloproteases 10 and 17 inhibitors. Oncoimmunology. 2015 Dec 10;5(4):e1093276. doi:10.1080/2162402X.2015.
- Kabelitz D, Serrano R, Kouakanou L, Peters C, Kalyan S. Cancer immunotherapy with γδ T cells: many paths ahead of us. Cell Mol Immunol. 2020 Sep;17(9):925–939. doi:10.1038/s41423-020-0504-x. Epub 2020 Jul 22. Erratum in: Cell Mol Immunol. 2020 Sep 1.
- Di Lorenzo B, Simões AE, Caiado F, Tieppo P, Correia DV, Carvalho T, Da Silva MG, Déchanet-Merville J, Schumacher TN, Prinz I, et al. Broad cytotoxic targeting of acute myeloid leukemia by polyclonal delta one T cells. Cancer Immunol Res. 2019 Apr;7(4):552–558. doi:10.1158/2326-6066.CIR-18-0647.
- Sebestyen Z, Prinz I, Déchanet-Merville J, Silva-Santos B, Kuball J. Translating gammadelta (γδ) T cells and their receptors into cancer cell therapies. Nat Rev Drug Discov. 2020 Mar;19(3):169–184. doi:10.1038/s41573-019-0038-z.
- Wesch D, Kabelitz D, Oberg HH. Tumor resistance mechanisms and their consequences on γδ T cell activation. Immunol Rev. 2020 Nov;298(1):84–98. doi:10.1111/imr.12925.
- Du H, Xu T, Cui M. cGAS-STING signaling in cancer immunity and immunotherapy. Biomed Pharmacother. 2021 Jan;133:110972. doi:10.1016/j.biopha.2020.110972.
- Hopfner KP, Hornung V. Molecular mechanisms and cellular functions of cGAS-STING signalling. Nat Rev Mol Cell Biol. 2020 Sep;21(9):501–521. doi:10.1038/s41580-020-0244-x.
- Wang-Bishop L, Wehbe M, Shae D, James J, Hacker BC, Garland K, Chistov PP, Rafat M, Balko JM, Wilson JT. Potent STING activation stimulates immunogenic cell death to enhance antitumor immunity in neuroblastoma. J Immunother Cancer. 2020 Mar;8(1):e000282. doi:10.1136/jitc-2019-000282.
- Zaidi AH, Kelly RJ, Gorbunova A, Omstead AN, Salvitti MS, Zheng P, Kosovec JE, Lee S, Ayazi S, Babar L, et al. Intratumoral immunotherapy with STING agonist, ADU-S100, induces CD8+ T-cell mediated anti-tumor immunity in an esophageal adenocarcinoma model. Oncotarget. 2021 Feb 16;12(4):292–303. doi:10.18632/oncotarget.27886.
- Chipurupalli S, Ganesan R, Dhanabal SP, Kumar MS, Robinson N. Pharmacological STING activation is a potential alternative to overcome drug-resistance in melanoma. Front Oncol. 2020 May 14;10:758. doi:10.3389/fonc.2020.00758.
- Miao L, Qi J, Zhao Q, Wu QN, Wei DL, Wei XL, Liu J, Chen J, Zeng ZL, Ju HQ, et al. Targeting the STING pathway in tumor-associated macrophages regulates innate immune sensing of gastric cancer cells. Theranostics. 2020 Jan 1;10(2):498–515. doi:10.7150/thno.37745.
- Esteves AM, Papaevangelou E, Dasgupta P, Galustian C. Combination of Interleukin-15 with a STING agonist, ADU-S100 analog: a potential immunotherapy for prostate cancer. Front Oncol. 2021 Mar 10;11:621550. doi:10.3389/fonc.2021.621550.
- Corrales L, Glickman LH, McWhirter SM, Kanne DB, Sivick KE, Katibah GE, Woo SR, Lemmens E, Banda T, Leong JJ, et al. Direct activation of STING in the tumor microenvironment leads to potent and systemic tumor regression and immunity. Cell Rep. 2015 May 19;11(7):1018–1030. doi:10.1016/j.celrep.2015.04.031.
- Tang CH, Zundell JA, Ranatunga S, Lin C, Nefedova Y, Del Valle JR, Hu CC. Agonist-mediated activation of STING induces apoptosis in malignant B cells. Cancer Res. 2016 Apr 15;76(8):2137–2152. doi:10.1158/0008-5472.CAN-15-1885.
- Falahat R, Perez-Villarroel P, AW M, Zhu G, Pilon-Thomas S, GN B, JJ M. STING signaling in melanoma cells shapes antigenicity and can promote antitumor T-cell activity. Cancer Immunol Res. 2019 Nov;7(11):1837–1848. doi:10.1158/2326-6066.CIR-19-0229.
- Ghaffari A, Peterson N, Khalaj K, Vitkin N, Robinson A, Francis JA, Koti M. STING agonist therapy in combination with PD-1 immune checkpoint blockade enhances response to carboplatin chemotherapy in high-grade serous ovarian cancer. Br J Cancer. 2018 Aug;119(4):440–449. doi:10.1038/s41416-018-0188-5.
- Ager CR, Reilley MJ, Nicholas C, Bartkowiak T, Jaiswal AR, Curran MA. Intratumoral STING activation with T-cell checkpoint modulation generates systemic antitumor immunity. Cancer Immunol Res. 2017 Aug;5(8):676–684. doi:10.1158/2326-6066.CIR-17-0049.
- Sivick KE, Desbien AL, Glickman LH, Reiner GL, Corrales L, Surh NH, Hudson TE, Vu UT, Francica BJ, Banda T, et al. Magnitude of therapeutic STING activation determines CD8+ T cell-mediated anti-tumor immunity. Cell Rep. 2018 Dec 11;25(11):3074–3085.e5. doi:10.1016/j.celrep.2018.11.047. Erratum in: Cell Rep. 2019 Oct 15;29(3):785-789.
- Li W, Lu L, Lu J, Wang X, Yang C, Jin J, Wu L, Hong X, Li F, Cao D, et al. cGAS-STING–mediated DNA sensing maintains CD8+T cell stemness and promotes antitumor T cell therapy. Sci Transl Med. 2020 Jun 24;12(549):549. doi:10.1126/scitranslmed.aay9013.
- Gutjahr A, Papagno L, Nicoli F, Kanuma T, Kuse N, Cabral-Piccin MP, Rochereau N, Gostick E, Lioux T, Perouzel E, et al. The STING ligand cGAMP potentiates the efficacy of vaccine-induced CD8+ T cells. JCI Insight. 2019 4;4;Apr(7):e125107. doi:10.1172/jci.insight.125107.
- Pu F, Chen F, Liu J, Zhang Z, Shao Z. Immune regulation of the cGAS-STING signaling pathway in the tumor microenvironment and its clinical application. Onco Targets Ther. 2021 Mar 1;14:1501–1516. doi:10.2147/OTT.S298958.
- Gao P, Ascano M, Wu Y, Barchet W, Gaffney BL, Zillinger T, Serganov AA, Liu Y, Jones RA, Hartmann G, et al. Cyclic [G(2’,5’)pA(3’,5’)p] is the metazoan second messenger produced by DNA-activated cyclic GMP-AMP synthase. Cell. 2013 May 23;153(5):1094–1107. doi:10.1016/j.cell.2013.04.046.
- Ritchie C, Cordova AF, Hess GT, Bassik MC, Li L. SLC19A1 Is an Importer of the Immunotransmitter cGAMP. Mol Cell. 2019 Jul 25;75(2):372–381.e5. doi:10.1016/j.molcel.2019.05.006.
- Luteijn RD, Zaver SA, Gowen BG, Wyman SK, Garelis NE, Onia L, McWhirter SM, Katibah GE, Corn JE, Woodward JJ, et al. SLC19A1 transports immunoreactive cyclic dinucleotides. Nature. 2019 Sep;573(7774):434–438. doi:10.1038/s41586-019-1553-0. Epub 2019 Sep 11. Erratum in: Nature. 2020 Mar;579(7800):E12.
- Pan BS, Perera SA, Piesvaux JA, Presland JP, Schroeder GK, Cumming JN, Trotter BW, Altman MD, Buevich AV, Cash B, et al. An orally available non-nucleotide STING agonist with antitumor activity. Science. 2020 Aug 21;369(6506). PMID: 32820094. doi:10.1126/science.aba6098.
- Ramanjulu JM, Pesiridis GS, Yang J, Concha N, Singhaus R, Zhang SY, Tran JL, Moore P, Lehmann S, Eberl HC, et al. Design of amidobenzimidazole STING receptor agonists with systemic activity. Nature. 2018 Dec;564(7736):439–443. doi:10.1038/s41586-018-0705-y. Erratum in: Nature. 2019 Jun;570(7761):E53.
- Chin EN, Yu C, Vartabedian VF, Jia Y, Kumar M, Gamo AM, Vernier W, Ali SH, Kissai M, Lazar DC, et al. Antitumor activity of a systemic STING-activating non-nucleotide cGAMP mimetic. Science. 2020 Aug 21;369(6506):993–999. doi:10.1126/science.abb4255.
- Peters C, Kouakanou L, Oberg HH, Wesch D, Kabelitz D. In vitro expansion of Vγ9Vδ2 T cells for immunotherapy. Methods Enzymol. 2020;631:223–237. doi:10.1016/bs.mie.2019.07.019.
- Sipos B, Möser S, Kalthoff H, Török V, Löhr M, Klöppel G. A comprehensive characterization of pancreatic ductal carcinoma cell lines: towards the establishment of an in vitro research platform. Virchows Arch. 2003 May;442(5):444–452. doi:10.1007/s00428-003-0784-4.
- Oberg HH, Peters C, Kabelitz D, Wesch D. Real-time cell analysis (RTCA) to measure killer cell activity against adherent tumor cells in vitro. Methods Enzymol. 2020;631:429–441. doi:10.1016/bs.mie.2019.07.020.
- Pechhold K, Pohl T, Kabelitz D. Rapid quantification of lymphocyte subsets in heterogeneous cell populations by flow cytometry. Cytometry. 1994 Jun 1;16(2):152–159. doi:10.1002/cyto.990160209.
- Serrano R, Coch C, Peters C, Hartmann G, Wesch D, Kabelitz D. Monocyte-dependent co-stimulation of cytokine induction in human γδ T cells by TLR8 RNA ligands. Sci Rep. 2021 Jul 27;11(1):15231. doi:10.1038/s41598-021-94428-6.
- Chan AH, Schroder K. Inflammasome signaling and regulation of interleukin-1 family cytokines. J Exp Med. 2020 6;217(1):Jan. doi:10.1084/jem.20190314.
- Imanishi T, Unno M, Kobayashi W, Yoneda N, Matsuda S, Ikeda K, Hoshii T, Hirao A, Miyake K, Barber GN, et al. Reciprocal regulation of STING and TCR signaling by mTORC1 for T-cell activation and function. Life Sci Alliance. 2019 25;2(1):Jan. doi:10.26508/lsa.201800282.
- Humphries F, Shmuel-Galia L, Jiang Z, Wilson R, Landis P, Ng SL, Parsi KM, Maehr R, Cruz J, Morales-Ramos A, et al. A diamidobenzimidazole STING agonist protects against SARS-CoV-2 infection. Sci Immunol. 2021 May 18;6(59):59. doi:10.1126/sciimmunol.abi9002.
- Xu Y, Xiang Z, Alnaggar M, Kouakanou L, Li J, He J, Yang J, Hu Y, Chen Y, Lin L, et al. Allogeneic Vγ9Vδ2 T-cell immunotherapy exhibits promising clinical safety and prolongs the survival of patients with late-stage lung or liver cancer. Cell Mol Immunol. 2021 Feb;18(2):427–439. doi:10.1038/s41423-020-0515-7.
- De Gassart A, Le KS, Brune P, Agaugué S, Sims J, Goubard A, Castellano R, Joalland N, Scotet E, Collette Y, et al. Development of ICT01, a first-in-class, anti-BTN3A antibody for activating Vγ9Vδ2 T cell-mediated antitumor immune response. Sci Transl Med. 2021 Oct 20;13(616):616. doi:10.1126/scitranslmed.abj0835.
- Ager CR, Boda A, Rajapakshe K, Lea ST, Di Francesco ME, Jayaprakash P, Slay RB, Morrow B, Prasad R, Dean MA, et al. High potency STING agonists engage unique myeloid pathways to reverse pancreatic cancer immune privilege. J Immunother Cancer. 2021;9(8):Aug. doi:10.1136/jitc-2021-003246.
- Tosolini M, Pont F, Verhoeyen E, Fournié JJ. Cyclic dinucleotides modulate human T-cell response through monocyte cell death. Eur J Immunol. 2015 Dec;45(12):3313–3323. doi:10.1002/eji.201545697.
- Tosolini M, Pont F, Bétous D, Ravet E, Ligat L, Lopez F, Poupot M, Poirot M, Pérouzel É, Tiraby G, et al. Human monocyte recognition of adenosine-based cyclic dinucleotides unveils the A2a Gαs protein-coupled receptor tonic inhibition of mitochondrially induced cell death. Mol Cell Biol. 2015 Jan;35(2):479–495. doi:10.1128/MCB.01204-14.
- Darwich L, Coma G, Peña R, Bellido R, Blanco EJ, Este JA, Borras FE, Clotet B, Ruiz L, Rosell A, et al. Secretion of interferon-gamma by human macrophages demonstrated at the single-cell level after costimulation with interleukin (IL)-12 plus IL-18. Immunology. 2009 Mar;126(3):386–393. doi:10.1111/j.1365-2567.2008.02905.x.
- Kraaij MD, Vereyken EJ, Leenen PJ, van Den Bosch TP, Rezaee F, Betjes MG, Baan CC, Rowshani AT. Human monocytes produce interferon-gamma upon stimulation with LPS. Cytokine. 2014 May;67(1):7–12. doi:10.1016/j.cyto.2014.02.001.
- Imanishi T, Saito T, Cell Co-stimulation T. Functional modulation by innate signals. Trends Immunol. 2020 Mar;41(3):200–212. doi:10.1016/j.it.2020.01.003.
- Cerboni S, Jeremiah N, Gentili M, Gehrmann U, Conrad C, Stolzenberg MC, Picard C, Neven B, Fischer A, Amigorena S, et al. Intrinsic antiproliferative activity of the innate sensor STING in T lymphocytes. J Exp Med. 2017 Jun 5;214(6):1769–1785. doi:10.1084/jem.20161674.
- Larkin B, Ilyukha V, Sorokin M, Buzdin A, Vannier E, Poltorak A. Cutting edge: activation of STING in T cells induces Type I IFN responses and cell death. J Immunol. 2017 Jul 15;199(2):397–402. doi:10.4049/jimmunol.1601999.
- Gulen MF, Koch U, Haag SM, Schuler F, Apetoh L, Villunger A, Radtke F, Ablasser A. Signalling strength determines proapoptotic functions of STING. Nat Commun. 2017 Sep 5;8(1):427. doi:10.1038/s41467-017-00573-w.
- Wu J, Dobbs N, Yang K, Yan N. Interferon-independent activities of mammalian STING mediate antiviral response and tumor immune evasion. Immunity. 2020 Jul 14;53(1):115–126.e5. doi:10.1016/j.immuni.2020.06.009.
- Kaser A, Nagata S, Tilg H. Interferon alpha augments activation-induced T cell death by upregulation of Fas (CD95/APO-1) and Fas ligand expression. Cytokine. 1999 Oct;11(10):736–743. doi:10.1006/cyto.1998.0484.
- Gamero AM, Potla R, Sakamoto S, Baker DP, Abraham R, Larner AC. Type I interferons activate apoptosis in a Jurkat cell variant by caspase-dependent and independent mechanisms. Cell Signal. 2006 Aug;18(8):1299–1308. doi:10.1016/j.cellsig.2005.10.008.
- Qiao Y, Zhu S, Deng S, Zou SS, Gao B, Zang G, Wu J, Jiang Y, Liu YJ, Chen J. Human cancer cells sense cytosolic nucleic acids through the RIG-I-MAVS pathway and cGAS-STING pathway. Front Cell Dev Biol. 2021 Jan 8;8:606001. doi:10.3389/fcell.2020.606001.
- Kwon J, Bakhoum SF. The Cytosolic DNA-Sensing cGAS-STING pathway in cancer. Cancer Discov. 2020 Jan;10(1):26–39. doi:10.1158/2159-8290.CD-19-0761.
- Amouzegar A, Chelvanambi M, JN F, WJ S, JJ L. STING Agonists as Cancer Therapeutics. Cancers (Basel). 2021 May 30;13(11):2695. doi:10.3390/cancers13112695.
- Oberg HH, Peipp M, Kellner C, Sebens S, Krause S, Petrick D, Adam-Klages S, Röcken C, Becker T, Vogel I, et al. Novel bispecific antibodies increase γδ T-cell cytotoxicity against pancreatic cancer cells. Cancer Res. 2014 Mar 1;74(5):1349–1360. doi:10.1158/0008-5472.CAN-13-0675.
- Jonescheit H, Oberg HH, Gonnermann D, Hermes M, Sulaj V, Peters C, Kabelitz D, Wesch D. Influence of Indoleamine-2,3-Dioxygenase and its Metabolite Kynurenine on γδ T cell cytotoxicity against ductal pancreatic adenocarcinoma cells. Cells. 2020 May 6;9(5):1140. doi:10.3390/cells9051140.
- Perera SA, Kopinja JE, Ma Y, Muise ES, Laskey J, Chakravarthy K, Chen Y, Cui L, Presland J, Sathe M, et al. STimulator of INterferon genes agonism accelerates anti-tumor activity in poorly immunogenic tumors. Mol Cancer Ther. 2021 Nov 23. molcanther.MCT-21-0136-A.2021. doi:10.1158/1535-7163.MCT-21-0136. molcanther.MCT-21-0136-A.2021.
- Shi J, Liu C, Luo S, Cao T, Lin B, Zhou M, Zhang X, Wang S, Zheng T, Li X. STING agonist and IDO inhibitor combination therapy inhibits tumor progression in murine models of colorectal cancer. Cell Immunol. 2021 Aug;366:104384. doi:10.1016/j.cellimm.2021.104384.
- Meric-Bernstam F, Sweis RF, Hodi FS, Messersmith WA, Andtbacka RHI, Ingham M, Lewis N, Chen X, Pelletier M, Chen X, et al. Phase I dose-escalation trial of MIW815 (ADU-S100), an intratumoral STING agonist, in patients with advanced/metastatic solid tumors or lymphomas. Clin Cancer Res. 2021 Oct 29. clincanres.CCR-21-1963-E.2021. doi:10.1158/1078-0432.CCR-21-1963.
- Le Naour J, Zitvogel L, Galluzzi L, Vacchelli E, Kroemer G. Trial watch: STING agonists in cancer therapy. Oncoimmunology. 2020 Jun 16;9(1):1777624. doi:10.1080/2162402X.2020.1777624.
- Girardi M, Oppenheim DE, Steele CR, Lewis JM, Glusac E, Filler R, Hobby P, Sutton B, Tigelaar RE, Hayday AC. Regulation of cutaneous malignancy by gammadelta T cells. Science. 2001 Oct 19;294(5542):605–609. doi:10.1126/science.1063916.