ABSTRACT
The past decades witnessed the clinical employment of targeted therapies including but not limited to tyrosine kinase inhibitors (TKIs) that restrain a broad variety of pro-tumorigenic signals. TKIs can be categorized into (i) agents that directly target cancer cells, (ii) normalize angiogenesis or (iii) affect cells of the hematologic lineage. However, a clear distinction of TKIs based on this definition is limited by the fact that many TKIs designed to inhibit cancer cells have also effects on immune cells that are being discovered. Additionally, TKIs originally designed to target hematological cancers exhibit bioactivities on healthy cells of the same hematological lineage. TKIs have been described to improve immune recognition and cancer immunosurveillance, providing the scientific basis to combine TKIs with immunotherapy. Indeed, combination of TKIs with immunotherapy showed synergistic effects in preclinical models and clinical trials and some combinations of TKIs normalizing angiogenesis with immune checkpoint blocking antibodies have already been approved by the FDA for cancer therapy. However, the identification of appropriate drug combinations as well as optimal dosing and scheduling needs to be improved in order to obtain tangible progress in cancer care. This Trial Watch summarizes active clinical trials combining TKIs with various immunotherapeutic strategies to treat cancer patients.
Introduction
Despite the caveat of major clinical side effects and mostly modest long-term therapeutic efficacy, chemotherapy with systemically active cytotoxicants (such as DNA-intercalating agents or microtubular poisons), that affect both malignant and healthy cells, is still the most frequently employed treatment for many types of cancer.Citation1–24 The last two decades have witnessed the development of novel antineoplastic therapies including targeted anticancer agents, which held the promise to limit nonspecific toxicity while increasing treatment efficacy.Citation25–27 The most commonly used compounds in precision medicine are signal transduction inhibitors that target oncogenic serine/threonine and tyrosine kinases.Citation28 Here, we focus on bona fide tyrosine kinase inhibitors (TKIs)Citation29–32 that have been approved by the FDA since the turn of the millennium and have meanwhile entered into clinical practice.Citation33,Citation34
TKIs target receptor tyrosine kinases as well as non-receptor tyrosine kinases.Citation35–37 Receptor tyrosine kinase inhibitors include, in chronological order of approval, gefitinibCitation38 targeting epidermal growth factor receptor (EGFR), approved for non-small cell lung cancer (NSCLC) in 2003;Citation39 erlotinibCitation40 targeting EGFR approved for NSCLC and pancreatic cancer (PC) in 2004;Citation41 sorafenib targeting vascular endothelial growth factor receptor (VEGFR), platelet-derived growth factor receptor (PDGFR), KIT and fms like tyrosine kinase (FLT) 3, approved for renal cell carcinoma (RCC) and hepatocellular carcinoma (HCC) in 2005;Citation42 sunitinibCitation43 targeting VEGFR, KIT, PDGFR, rearranged during transfection (RET), colony stimulating factor 1 receptor (CSF1R) and FLT3, approved for RCC and gastrointestinal stromal tumor (GIST) in 2006;Citation44 lapatinibCitation45 targeting EGFR and human epidermal growth factor receptor (HER) 2, approved for breast cancer (BC) in 2007;Citation46 pazopanibCitation47 targeting VEGFR, PDGFR and KIT, approved for RCC in 2009; vandetanib targeting RET, VEGFR, fibroblast growth factor receptor (FGFR) 3 and EGFR approved for thyroid carcinoma (TC) in 2011; crizotinibCitation48 targeting anaplastic lymphoma kinase (ALK) and MET, approved for NSCLC in 2011;Citation49 axitinib targeting VEGFR, PDGFR, KIT, RET, CSF1R and FLT3 approved for RCC in 2012; cabozantinib targeting VEGFR, PDGFR, KIT and FLT3 approved for TC in 2012; regorafenib targeting VEGFR-1, −2, −3 and TIE2, approved for colorectal cancer (CRC), GIST in 2012 and hepatocellular carcinoma in 2017; afatinib targeting EGFR, approved for NSCLC in 2013;Citation50 ceritinib targeting ALK, approved for NSCLC in 2014;Citation51 alectinib targeting ALK, approved for NSCLC in 2015;Citation52 lenvatinib targeting VEGFR, approved for TC, RCC in 2015;Citation53 osimertinib targeting EGFR, approved for NSCLC in 2015;Citation54 neratinib targeting EGFR, approved for BC in 2017;Citation55 brigatinib targeting ALK, EGFR, approved for NSCLC in 2017;Citation56 tivozanib targeting VEGFR, approved for RCC in 2021;Citation57 dacomitinib targeting EGFR, approved for NSCLC in 2018;Citation58 lorlatinib targeting ALK, approved for NSCLC in 2018;Citation59 larotrectinib targeting tropomyosin receptor kinases (TRKs), approved for solid tumors in 2018;Citation60 gilteritinib targeting FLT3 approved for acute myeloid leukemia (AML) in 2018;Citation61 erdafitinib targeting FGFR, approved for transitional cell carcinoma (TCC) in 2019;Citation62 pexidartinib targeting CSF1R, KIT, FLT3, approved for tenosynovial giant cell tumor in 2019;Citation63 entrectinib targeting neurotrophic tyrosine receptor kinase (NTRK) 1/2/3, ROS1, ALK, approved for NSCLC in 2019;Citation64,Citation65 avapritinib targeting KIT, PDGFR, approved for GIST in 2020; tucatinib targeting HER2, approved for BC in 2020; pemigatinib targeting FGFR, approved for cholangiocarcinoma in 2020;Citation66 capmatinib targeting MET, approved for NSCLC in 2020;Citation67 selpercatinib targeting RET, approved for TC and NCSLC in 2020;Citation68 ripretinib targeting KIT, PDGFR, approved for GIST in 2020;Citation69 pralsetinib targeting RET, approved for NSCLC, TC in 2020;Citation70 and finally tepotinib targeting MET, approved for NSCLC in 2021.Citation71
Inhibitors of non-receptor tyrosine kinases encompass imatinib,Citation31 targeting ABL1, KIT and PDGFR, approved for chronic myelogenous leukemia (CML), B cell acute lymphoblastic leukemia (ALL)and GIST in 2001;Citation30,Citation72 dasatinibCitation73 targeting ABL1, PDGFR, KIT, SRC, approved for CML and ALL in 2006; nilotinib targeting ABL1, PDGFR, KIT, approved for CML in 2007; ruxolitinibCitation74 targeting janus kinase (JAK) 2, approved for myelofibrosis in 2011;Citation75 bosutinib targeting ABL1, approved for CML in 2012; ponatinib targeting ABL1, approved for CML in 2012; ibrutinib targeting Bruton’s tyrosine kinase (BTK), approved for mantle cell lymphoma (MCL) in 2013;Citation76 acalabrutinib targeting BTK, approved for MCL and chronic lymphoblastic leukemia (CLL) in 2017; fostamatinib targeting spleen tyrosine kinase (SYK), approved for autoimmune thrombocytopenia in 2018; fedratinib targeting JAK3 and FLT3, approved for myelofibrosis in 2019; and zanubrutinib targeting BTK, approved for MCL in 2019, respectively.
Of note, a strictly target-based distinction of TKIs is hampered by the fact that most TKIs target multiple kinases with varying efficacy.
Many of the above listed TKIs have been successfully introduced into the clinical management of cancer, resulting in a significantly increased overall survival (OS). For instance, imatinib, that among other kinases inhibits BCR-ABL, converted the otherwise rapidly fatal CML into a manageable condition with a five-year progression-free survival (PFS) of 82–90%.Citation77–80 Imatinib also inhibits c-KIT and PDGFR and induced significant clinical responses leading to 69–74% OS at 2 year follow-up as first-line treatment of advanced GIST.Citation81,Citation82 Dasatinib, a second-generation TKI that is more potent than imatinib and active against several forms of imatinib-resistant CML carrying BCR-ABL mutations, achieves similar response rates with a 5 year PFS of 86%.Citation83 The imatinib derivative nilotinib, a second-generation BCR-ABL inhibitor with improved specificity and affinity, was initially approved for imatinib-resistant CML and has more recently become available as first-line treatment for CML.Citation78 In some cases, nilotinib achieved deep and long-lasting molecular remissions, thus allowing for discontinuation of the treatment.Citation84,Citation85 Ponatinib, another potent BCR-ABL inhibitor, outperformed dasatinib and nilotinib and triggered a sustained cytogenetic response in CML or ALL patients who experienced resistance to, or unacceptable side effects from, dasatinib or nilotinib. Moreover, no mutation-conferring resistance arose over a 15-months median follow-up period.Citation86,Citation87
Gefitinib is a selective inhibitor of EGFR, approved as first-line treatment of NSCLCs that bear sensitizing EGFR mutations,Citation88 that exerted a beneficial effect on PFS, notably in patients with EGFR mutation, and an objective response rate of 71.2% versus 47.3% for the chemotherapy treated group. However, no significant difference in OS was detectable.Citation89 Similarly, the EGFR inhibitors erlotinib and afatinib depicted limited efficacy on OS in patients with EGFR mutated NSCLC.Citation90,Citation91 Osimertinib, a third-generation EGFR inhibitor, outperformed gefitinib and erlotinib in previously untreated advanced NSCLC with EGFR mutation. Citation92,Citation93 Treatment with osimertinib significantly prolonged OS when compared to gefitinib and erlotinib (38.6 months versus 31.8 months). Moreover, the safety profiles were similar, although osimertinib was administered for 20.7 months versus 11.5 months of gefitinib and erlotinib exposure.Citation94
Ruxolitinib, an inhibitor of JAK 1 and 2, is efficacious in treating myelofibrosis.Citation95,Citation96 It achieved a significant reduction of spleen volume in 41.9% of patients and 67% of responding patients had long-lasting responses (48 weeks or more).Citation97 Ruxolitinib demonstrated a superior clinical efficacy when compared to the best available therapy. Accordingly, ruxolitinib-treated patients experienced a substantial amelioration of spleen size, disease-related symptoms and quality of life.Citation98
Crizotinib, ceritinib and lorlatinib are first, second and third-generation ALK inhibitors, respectively.Citation99 Ceritinib demonstrated a higher selectivity for ALK and an increased potency of inhibition than crizotinib. In fact, the FDA approved crizotinib to treat ROS-1 and ALK-positive NSCLC, whereas ceritinib was approved to treat only ALK-positive NSCLC. Lorlatinib was designed to better penetrate the blood–brain barrier and treat NSCLC patients with brain metastases. Accordingly, a phase II trial reported a meaningful intracranial response after lorlatinib administration both in treatment naïve patients and in those who progressed after treatment with up to three different ALK inhibitors.Citation100,Citation101
Lapatinib reversibly inhibits HER2 and EGFR and is approved in combination with capecitabine for the treatment of HER2-positive advanced breast cancer, offering an effect on PFS as compared to monochemotherapy.Citation102,Citation103 Neratinib is a pan-HER inhibitor that irreversibly binds to its target. It is approved for second-line combinations with capecitabine in HER2-positive advanced breast cancer that progressed after HER2 directed therapy. Neratinib cotreatment with capecitabine increased OS as compared to lapatinib continuation.Citation104 Tucatinib, yet another HER2 inhibitor, has recently been approved in combination with trastuzumab and capecitabine, for advanced unresectable or metastatic HER2 positive breast cancer. Tucatinib increased the median PFS to 7.8 months as compared to 5.6 months in patients that received only trastuzumab and capecitabine.
Sorafenib targets VEGFR, PDGFR, KIT, FLT3 and was approved for RCC and HCC in 2005. Sorafenib, which inhibits both cancer cell proliferation and angiogenesis, improved progression-free survival of RCC patients resistant to conventional therapies from 2.8 months to 5.5 months. However, adverse events were more common in the sorafenib-treated group than in placebo-treated controls.Citation105 Similar results were achieved in advanced hepatocellular carcinoma patients, who showed a 3-month improvement in median survival and time to radiologic progression compared to the placebo group.Citation106 These results led to its approval for the treatment of advanced RCC and unresectable HCC in 2005 and 2007, respectively.Citation107
Sunitinib is a multikinase inhibitor that targets amongst other kinases c-KIT and PDGFR and is effective against imatinib-resistant GIST with a median PFS of about 6 months.Citation108 Furthermore, due to its ability to inhibit VEGF, sunitinib is employed as first-line treatment of advanced RCC achieving a progression-free survival of 11.1 months.Citation109,Citation110
Axitinib, yet another multikinase inhibitor, targets VEGFR, PDGFR, KIT, RET, CSF1R and FLT3. It was approved for RCC in 2012 for its increased PFS of 6.7 months compared to 4.7 months with a standard sorafenib treatment.Citation111,Citation112
Cabozantinib targeting VEGFR, PDGFR, KIT and FLT3 improved PFS in patients with medullary thyroid cancer from 4 to 11.2 months. While prolonged PFS was independent of the tumor mutational status, cabozantinib extended overall survival only in those patients harboring a specific mutation in the RET gene. Patients who harbored RET M918T mutation and were treated with cabozantinib exhibited a median OS of 44.3 months compared to 18.9 months for the placebo group.Citation113,Citation114 Cabozantinib also extended PFS of radioiodine-refractory differentiated thyroid cancer patients previously treated with VEGFR-targeted therapy, leading to its approval by the FDA in September 2021.Citation115–118 Cabozantinib was approved in intermediate and poor-risk previously untreated advanced kidney cancer patients in December 2017 following a phase 2 trial (NCT01835158). Median progression-free survival for patients taking cabozantinib was 8.6 months compared with 5.3 months for patients taking sunitinib.
Regorafenib dually inhibits VEGFR and TIE2 and is approved for the third-line therapy of advanced GIST. Treatment with regorafenib provides benefit for patients with resistance to both imatinib and sunitinib with a median PFS of 4.8 months.Citation119
Lenvatinib, VEGFR inhibitor, is a therapeutic choice for locally recurrent or metastatic thyroid cancer, advanced renal cell carcinoma (in combination with everolimus) after one antiangiogenic therapy and unresectable hepatocellular carcinoma. OS of unresectable hepatocellular carcinoma patients treated with lenvatinib was 13.6 months as compared to 12.3 months of sorafenib-treated patients.Citation120,Citation121In patients with metastatic clear cell renal cell carcinoma, lenvatinib, alone or in combination with everolimus, significantly prolonged PFS compared to everolimus treatment.Citation122 In thyroid cancer patients, the response rate after lenvatinib treatment was 64.8% vs 1.5% of the placebo group.Citation123,Citation124
Ibrutinib irreversibly inhibits BTK, which plays a key role in B-cell receptor signaling, and is approved for the treatment of mantel cell lymphoma (MCL) as well as CLL depicting a significant increase in PFS as compared with standard chemoimmunotherapy.Citation125 Acalabrutinib, a second-generation BTK-inhibitor, was approved for MCL, and more recently for CLL and SLL with increased efficacy and durability of the response.Citation126 Zanubrutinib, yet another second-generation BTK inhibitor, is approved for MCL and further extended PFS to 21.1 months in patients with relapsed or refractory disease.Citation127,Citation128
Despite the clinical success against some cancers, continuous treatment with TKI often results in acquired resistance, rendering TKI-mediated cytostatic effects mostly transitory.Citation129–131 Second and third generation TKI with higher response rates have been developed to remedy such downfall. However, combination regimens of TKIs and therapies targeting separate pro-tumoral pathways may represent another solution.
During the past decade immunotherapies have been introduced into clinical routine, demonstrating promising results in many cancer types.Citation132–141 Combinatorial strategies employing targeted agents together with immunotherapy may overcome TKI resistance.
This Trial Watch summarizes all active (not yet recruiting, recruiting, active not recruiting) clinical trials (as of October 2021) combining already FDA approved TKIs with various immunotherapeutic strategies. The clinical trials are presented in three tables corresponding to (i) TKIs with cytotoxic or cytostatic effects on cancer cells (); (ii) TKIs normalizing angiogenesis (); and (iii) TKIs initially conceived to treat hematological cancers which target cells of the hematologic lineage (). However, this classification is problematic in thus far that many of the TKIs falling into group 1 have also effects on immune cells, such as dendritic cells, and TKIs normalizing angiogenesis (as exemplified by cabozantinib) also have cytotoxic and cytostatic effects on cancer cells.Citation142
Table 1. Active clinical trials combining TKIs with cytotoxic or cytostatic effects on cancer cells and immunotherapies (source: ClinicalTrial.gov)
Table 2. Active clinical trials combining TKIs normalizing angiogenesis and immunotherapies (source: ClinicalTrial.gov)
Table 3. Active clinical trials combining TKIs originally developed to target hematological cancers and immunotherapies (source: ClinicalTrial.gov)
Many lines of evidence indicate that TKIs mediate non-cell autonomous mechanisms of action including the immune-dependent elimination of tumor cells, laying the foundation for the potential synergy of TKIs and immunotherapy.Citation143 Here, we will discuss preclinical evidence in favor of such combination therapies and then describe the most promising combinations that are currently being evaluated in clinical trials.
Preclinical evidence for immunostimulatory effects of TKIs targeting cancer cells
TKIs listed in halt tumor cell proliferation and survival, thus mediating a cytotoxic or cytostatic effect on cancer cells.Citation144 In addition, they shape the tumor environment and may switch it from immunosuppressive or tumor-permissive to immune-stimulating and tumor-intolerant.Citation7,Citation145–147
For instance, imatinib can act on DCs to inhibit endogenous c-KIT and to stimulate their capacity to activate NK cells with tumoricidal activity,Citation148,Citation149 a finding that has been validated in patients with GIST.Citation150,Citation151 Imatinib reduced the burden of c-KIT-positive GISTs in mice by inhibiting tumor cell proliferation, but also by reducing tryptophan-derived immunosuppressive metabolites through the inhibition of indoleamine-2,3-dioxygenase (IDO) expression. Therefore, imatinib boosted intratumoral CD8+ T cell activation and proliferation, thus promoting tumor cell killing by cytotoxic lymphocytes.Citation152 Combination of imatinib with anti-cytotoxic T lymphocyte antigen 4 (CTLA-4) blocking antibody significantly decreased tumor size compared to single treatments, as a result of the increased interferon (IFN)-γ production by intratumoral CD8+ T cells.Citation153 Of note, Seifert and colleagues showed that intratumoral CD8+ T cells displayed surface programmed cell death protein 1 (PD-1), and GIST cells as well as tumor infiltrating leukocytes expressed programmed cell death protein 1 (PD-L1). Therefore, the authors investigated the therapeutic efficacy of concurrent administration of imatinib and anti-PD-1 or anti-PD-L1. Importantly, the efficacy of imatinib plus anti-PD-1 was visible as early as 1 week after treatment and persisted for 3 months.Citation154–156
Inhibition of EGFR by erlotinib or osimertinib increased secretion of C-X-C motif chemokine ligand (CXCL) 10 (notoriously attracting CD8+ T cells) and reduced C-C motif chemokine ligand (CCL) 22 (which is a chemoattractant for T regulatory cells (Tregs)).Citation157 Accordingly, EGFR inhibitors reprogram the immune environment and increase CD8+ T cell-mediated killing of lung adenocarcinoma cells.Citation157 Erlotinib showed impressive preclinical success when combined with anti-PD-1 blockade therapy. Sugiyama and colleagues treated tumors derived from murine lung adenocarcinoma cell lines engineered to express human mutant EGFR and implanted them subcutaneously or intravenously into immunocompetent mice. Erlotinib plus anti-PD-1 showed superior therapeutic efficacy compared to single treatments.Citation145,Citation157 However, despite the fact that the oncogenic signaling may induce PD-L1 upregulation in NSCLC, the superiority of immune checkpoint inhibitors in advanced EGFR-mutant NSCLC is only moderate in patients. Indeed, multiple mechanisms, including dynamic immune TME, PD-L1 expression levels and low tumor mutational burden, may account for the conflicting results regarding associations between the EGFR mutation status and response rates with PD-L1/PD-1 inhibitors.Citation158
However, despite the fact that the oncogenic signaling may induce PD-L1 upregulation in NSCLC, the superiority of immune checkpoint inhibitors in advanced EGFR-mutant NSCLC is only moderate in patients. Indeed, multiple mechanisms including dynamic immune TME, PD-L1 expression levels and low tumor mutational burden, may account for the conflicting results regarding associations between the EGFR mutation status and response rates with PD-L1/PD-1 inhibitors.Citation158 Dasatinib also sensitized resistant tumors to anti-PD1 effect. Tu and collaborators identified discoidin domain-containing receptor 2 (DDR2) kinase, one of the dasatinib targets, as responsible for anti-PD1 resistance. Thus, its inhibition in combination with PD-1 blockade reduced growth and induced regression of subcutaneous prostate, colon and sarcoma tumors. The increased number of CD8+ T cells among tumor infiltrating lymphocytes (TILs) suggested the recognition of specific tumor antigens occurring only in mice treated with dasatinib and anti-PD1.Citation159
Crizotinib, an inhibitor of ALK, MET and ROS kinases,Citation160,Citation161 stopped tumor cell proliferation and induced immunogenic cell death,Citation12 which alerts the immune system to the presence of the tumor and triggers a specific response.Citation162–164 Thus, immunotherapy with an anti-PD1 antibody administered after crizotinib cured almost 90% of mice bearing orthotropic NSCLCs.Citation145,Citation165,Citation166
Ruxolitinib reduced tumor cell proliferation by inhibiting the signaling cascade involving JAK and signal transducer and activator of transcription (STAT).Citation167 Moreover, it suppressed the production of immunosuppressive cytokines (such as interleukin (IL)-6, IL-10 and granulocyte-macrophage colony-stimulating factor (GM-CSF)) by pancreatic tumor cells through STAT3 inhibition.Citation168 Treatment of orthotopic pancreatic tumors with ruxolitinib switched the tumor environment to immunostimulation and increased the number of infiltrating CD8+ T cells as well as the expression of IL-21 and IL-17A. Combination with anti-PD-1 showed a synergistic activity.Citation169 Moreover, ruxolitinib has been successfully combined with oncolytic viral therapy. This effect may be explained by a ruxolitinib-mediated inhibition of the type-I-IFN antiviral response, thus sensitizing tumor cells to the lytic effect of the virus.Citation170–172
Preclinical evidence for immunostimulatory effects of TKIs targeting angiogenesis
Tumor-induced angiogenesis interferes with immunosurveillance. For instance, newly formed blood vessels within tumors are frequently malformed, dysfunctional and leaky, thus representing a physical barrier for immune cell infiltration.Citation173 In addition, endothelial cells may express immunosuppressive molecules,Citation174 such as PD-L1 or Fas ligand (FasL) and cause T cell inactivation or death before they ever reach tumor cells.Citation175 Moreover, abnormal tumor blood vessels fail to deliver sufficient oxygen to the malignant tissue, which is frequently hypoxic. Hypoxia triggers secretion of chemokines attracting suppressive immune populations, such as myeloid-derived suppressor cells (MDSCs), tumor associated macrophages (TAM) of the M2 subtype or Tregs.Citation176–179 Accordingly, inhibition or normalization of intratumoral angiogenesis reverses the state of immunosuppression of the tumor environment and improves immunosurveillance.Citation180,Citation181 Notably, inhibitors of VEGFR interfere with angiogenesis and serve as immune modulators.
Sunitinib, a TKI targeting multiple pathways, reduced newly formed blood vessels within adenocarcinomas and breast tumors. Addition of sunitinib or sorafenib to a vaccine against a tumor-derived antigen improved CD4+ and CD8+ T cell infiltration into tumors and increased the frequency of antigen-specific T cells.Citation182 As a consequence, vaccination coupled to angiogenesis inhibition significantly reduced tumor burden, achieving complete remissions in 20% of tumor-bearing mice.Citation182 Sorafenib, another TKI targeting multiple kinases, was tested together with vaccination to treat breast tumors. Thus, a dendritic cell (DC)-based, GM-CSF-secreting, HER2 targeted cellular vaccine increased CD4+ and CD8+ T cell infiltration into tumors and reduced tumor burden in a particularly efficient fashion when the vaccine was combined with sorafenib.Citation183,Citation184
Axitinib is a specific VEGFR inhibitor, the therapeutic efficacy of which relies on functional T cells.Citation185 Accordingly, immunotherapeutic strategies boosting T cell function greatly improved axitinib therapeutic success. In particular, addition of anti-PD-1 and antibody-mediated blockade of T cell immunoglobulin and mucin-domain containing (TIM)-3 plus an anti-CD137 agonistic antibody to axitinib induced complete regression of more than 90% of lung and colon carcinomas.Citation185 Similarly, lenvatinib-mediated tumor growth control relied on the presence of functional CD8+ T cells and synergized with PD-1 blockade.Citation185
Preclinical evidence for the use of ibrutinib and next-generation BTK inhibitors
Ibrutinib and BTK next generation inhibitorsCitation186–192 were originally developed to target hematological cancers. However, they also target immune cells of the hematologic lineage.Citation193,Citation194 Ibrutinib influences the phenotype and function of both innate and adaptive immune cells. For instance, ibrutinib improves DC maturation,Citation195 enhancing their capacity to prime T cells. In addition, it suppresses Th2 differentiation in vitro, in vivo and in CLL patients.Citation196 Mechanistically, ibrutinib reduces the expression of immunosuppressive molecules, such as PD-1 or CTLA-4, on the T cell surface.Citation197,Citation198 As a consequence, T cells of ibrutinib-treated patients effectively kill tumor cells. Such direct immunological consequences of ibrutinib might be explained by inhibition of interleukin-2-inducible T-cell kinase (ITK) as an off-target effect.Citation199 Next-generation BTK inhibitors are more specific. However, BTK expression has recently been described in T cells, suggesting that even highly selective BTK inhibitors may act on immune cells to improve their anticancer function.Citation200
BTK inhibitor effects on T cells have been exploited to improve the engraftment of chimeric antigen receptor-T (CAR-T) cells and CAR-T-mediated tumor clearance. As a matter of fact, CD19-directed CAR-T cells generated from ibrutinib-treated patients showed improved engraftment in blood and bone marrow after reinfusion into the patients.Citation201 Accordingly, concurrent treatment of xenograft models of ALL or CLL with human CAR-T cells and ibrutinib reduced tumor burden and greatly increased mouse survival, compared to single treatments. The efficacy of the combinatorial treatment could be attributed to an increased expansion of CAR-T cells after engraftment as well as improved effector functions, due to the downregulation of PD-1.Citation201 A similar synergism was described in a model of MCL. Combination of CD19-directed CAR-T cells and ibrutinib yielded long-term disease control in 80–100% of the mice.Citation202
One preclinical study explored the combination of acalabrutinib and CAR-T cells targeting CD19+ tumor cells. Combination of acalabrutinib and CD19-specific CAR-T cells was tested in a xenogeneic tumor model where luciferase-expressing B-ALL precursor cells were injected into NOD SCID gamma (NGS) mice.Citation203 The combinatorial treatment elicited superior cytotoxic effects compared to single agents, resulting in increased mouse survival and reduced tumor burden. Improved efficacy resulted from simultaneous inhibition of BTK in tumor cells by acalabrutinib, which enhanced their CAR-T cell-mediated lysis. However, acalabrutinib also improved CAR-T cell-mediated killing of CD19+ tumor cells in vitro and increased cytokine release by CAR-T cells. Accordingly, in vivo injection of acalabrutinib increased the frequency of CAR-T cells in the blood and skewed their phenotype toward that of memory T cells.Citation203
TKIs and modulation of gut dysbiosis
TKIs are often causing adverse effects in the digestive tract, including diarrhea.Citation204 In fact, fecal microbiota transplantation (FMT) from healthy donors has been randomized against placebo to treat TKI–induced diarrhea in patients with metastatic renal cell carcinoma (NCT04040712). The primary outcome was the resolution of diarrhea at four weeks. Healthy donor FMT was more effective than placebo in treating TKI-induced diarrhea, when a successful engraftment of allogeneic feces was obtained.Citation205
Moreover, the impact of the baseline taxonomic composition of the stools before TKI-based therapy has been recently studied in randomized trials testing the effects of yogurt products in advanced kidney cancer patients. Among those 20 evaluable for response, 15 patients achieved objective responses that were correlated with the fecal overrepresentation of immunogenic metagenomic species (such as Akkermansia muciniphila and Barnesiella intestinihominis).Citation206,Citation207 Moreover, Derosa et al. confirmed in preclinical studies that TKIs exert a direct effect on the composition of the gut commensals in naïve animals orally given three different types of TKIs daily for 3 weeks.Citation205 All three TKIs markedly induced significant changes in the alpha- and beta-diversity of the microbiota over time, in both BALB/c and C57BL/6 mice, with a common dominant deviation of the microbiota composition. Sunitinib and cabozantinib favored a higher abundance of immunostimulatory Alistipes senegalensis, as observed in humans. In C57BL/6 intestines, there was an over-representation of the immunostimulatory Eubacterium siraeum, among other species shared by all three TKIs (such as Akkermansia muciniphila, especially for cabozantinib). Altogether, we concluded that TKIs induced a significant and prototypic microbiota shift including immunostimulatory commensals that could be harnessed to improve the efficacy of ICIs in RCC patients.
Ongoing clinical trials
Driven by the aforementioned preclinical experimentation, the potential synergism between TKIs and immunotherapies is being evaluated in cancer patients. In March 2022, the website https://www.clinicaltrials.gov listed 408 combinations of TKIs and immunotherapy (). Thirty-one FDA approved TKIs are being combined with immunotherapy for several therapeutic indications. With the only exception of ponatinib, alectinib, gilteritinib and fedratinib all TKIs are being tested in combination with PD-1 or PD-L1 blockade therapies.
Very promising is the combination of ABL TKIs and blinatumomab, a bispecific CD19-directed CD3 T-cell engager indicated for the treatment of Philadelphia chromosome-negative relapsed or refractory B-cell precursor acute lymphoblastic leukemia (ALL), and minimal residual disease (MRD)-positive B-cell precursor ALL. Results of a phase II trial evaluating the efficacy of dasatinib and blinatumomab in newly diagnosed ALL adult patients showed impressive results (NCT02744768). In this trial, 61 patients received dasatinib as induction therapy, followed by consolidation treatment with blinatumomab (58 patients received at least one cycle). At the end of the induction phase, 98% of patients exhibited a complete hematological response (defined as ≤5% bone marrow blasts, absence of blasts in the peripheral blood, no extramedullary involvement and full recovery of the peripheral blood count) and 29% had a molecular response (defined as ratio between BCR-ABL1 to ABL1 equal 0 as detected by qPCR from bone marrow samples). Two cycles of blinatumomab increased the molecular responses to 60%. At 18 months, the OS was 95% and disease-free survival (DFS) amounted to 88%. Interestingly, sequencing of the ABL gene in 15 patients experiencing an increase in minimal residual disease after dasatinib treatment revealed ABL mutations in 7 patients. Even more interestingly, such mutations were undetectable when dasatinib was combined with blinatumomab, arguing in favor of the hypothesis that immunotherapy might clear resistant clones arising in the context of a TKI treatment.Citation208 Such results encouraged a Phase III trial (NCT04722848, not yet recruiting) which will assess the efficacy of ponatinib followed by blinatumomab in patients with BCR-ABL+ ALL treatment naïve. Patients will receive ponatinib for 10 weeks and then will receive blinatumomab (minimum 2 cycles, up to a maximum of 5). The active comparator arm will be chemotherapy plus imatinib. A second Phase III trial (NCT04530565, recruiting) is evaluating the combination of dasatinib or ponatinib with blinatumomab (simultaneous administration) together with chemotherapy and steroids. Treatment scheduling is of major importance. For instance, the trial I–II NCT02574078 tested the combination of crizotinib (250 mg twice daily) and nivolumab (240 mg once every two weeks) administered simultaneously, and 38% of patients developed severe hepatic toxicities. Similarly, mice treated with crizotinib and anti-PD1 at the same time exhibited liver toxicity. However, no signs of liver toxicity were observed when PD-1 blockade was administered one week after crizotinib.Citation166
The number of clinical trials testing angiogenesis inhibitors plus immunotherapies is impressive. The majority of them combine antiangiogenic TKIs with PD-1 blockade.Citation209 Notably, results of the phase Ib/II trial (KEYNOTE-146, NCT02501096) demonstrated robust therapeutic efficacy of lenvatinib plus pembrolizumab in six different cancer types: urothelial cancer, head and neck squamous cell carcinoma, melanoma, non-small-cell lung cancer, renal cell carcinoma and endometrial cancer.Citation210 In the endometrial cancer cohort, enrolling 94 patients with previously treated metastatic endometrial cancer, lenvatinib plus pembrolizumab yielded an objective response rate of 38.3% with 10 complete responses (10.6%). These results spurred accelerated FDA approval of lenvatinib plus pembrolizumab in 2019 for advanced endometrial cancer patients progressing after prior systemic therapy (not candidates for curative surgery or radiation). Moreover, these remarkable results encouraged the development of the LEAP program, which is currently evaluating the efficacy of lenvatinib and pembrolizumab in various clinical indications in nine phase III and three phase II trials.Citation210
Recently, in January 2021, FDA approved the combination of nivolumab and cabozantinib as first-line treatment for patients with advanced renal cell carcinoma. A pivotal phase III trial (CHECKMATE-9ER, NCT03141177) evaluated the efficacy of nivolumab, administered intravenously every two weeks, and daily cabozantinib in treatment-naïve renal cell carcinoma patients. The active comparator was sunitinib. Patients treated with nivolumab and cabozantinib exhibited improved progression-free survival (16.6 months vs 8.3 months) and overall response rates (55.7% vs 27.1%) when compared with sunitinib-treated patients.Citation211
Ibrutinib and BTK next-generation inhibitors are frequently combined with anti-PD1 monoclonal antibodies. However, efforts are being made to evaluate combinations with CAR T cells engineered to recognize CD19. Such efforts stemmed from the observation that treatment with ibrutinib prior to CAR-T cell infusion had some beneficial effects in heavily pretreated CLL patients. The phase I trial NCT00466531 investigated safety and efficacy of CD19 CAR-T cells in 16 patients, 5 of whom had received ibrutinib before leukapheresis or CAR-T cell infusion. T cells isolated from such patients showed greater expansion efficiency and had more frequently a central memory phenotype. Altogether, objective responses were observed in 12 out of 16 patients (4 out of 5 treated with ibrutinib). Moreover, three patients experienced complete responses (2 were on ibrutinib at CAR-T cell infusion).Citation212
As mentioned in the introduction, the classification used throughout this Trial Watch is not flawless. Several TKIs belonging to the first category also inhibit TKs expressed by immune cells, and a few clinical trials exploit this feature. For instance, ruxolitinib suppresses JAKs-STATs pathways in tumor cells as well as in immune cells and blunts the secretion of cytokines including IL-6, IFN-γ and TNF-α. A case report described a beneficial effect of ruxolitinib in reducing cytokine release syndrome after treatment with CD19/CD22 bispecific CAR-T cells without impairing CAR-T cell anti-tumor effects. With the same rationale, the Phase II–III trial NCT03117751 evaluates this JAK inhibitor in combination with CAR-T cells.
Concluding remarks
The combination of TKIs that target tumor-promoting pathways and immunotherapy might constitute a highly promising approach to treat cancer patients owing to the facts that: (i) TKIs reduce tumor size by halting cancer cell proliferation or by starving tumor cells to death; (ii) TKIs simultaneously improve immune-mediated recognition and elimination of tumor cells; and (iii) immunotherapy boosts immunosurveillance against mutant cancer cells that are on the verge of developing resistance to TKIs ()), iv) TKI act on the intestinal barrier and modulate gut dysbiosis. Preclinical studies have demonstrated the efficacy of such combinations in multiple mouse models. This Trial Watch has provided an overview of active clinical trials testing TKIs and immunotherapies as oncological indications (). The number of on-going clinical trials is impressive, reflecting an ever-increasing interest in such a combinatorial strategy. Moreover, the diversity of cancers being treated with combination therapies suggests that such a strategy may successfully target many different types of cancer. The identification of appropriate drug combinations as well as optimal dosing and scheduling will be essential for obtaining tangible improvements in cancer care. For this, cognitive insights still will be essential. Thus, the elucidation of the immunomodulatory (immunostimulatory or immunosuppressive) side effects of each TKI will be mandatory to choose the right immunotherapeutic combination partner. Then, appropriate scheduling (simultaneous or asynchronous, TKI first or immunotherapy first, TKI provided in a continuous or intermittent fashion, etc.) will be important to obtain the best imaginable clinical benefit for cancer patients.Citation213
Figure 1. Functional TKI categories. Tyrosine kinase inhibitors (TKIs) can be functionally categorized into agents with cytotoxic or cytostatic effects on cancer cells leading to the establishment of chemokine gradients and improving the elimination of tumors by CD8+ cytotoxic T lymphocytes (CTLs) (A). TKIs from the second category are endowed with angiogenesis normalizing effects and favor immune cell migration toward tumors (B). The third category of TKIs encompasses inhibitors originally developed to target hematological cancers that have been found to also activate immune cells such as dendritic cells and CTLs (C).
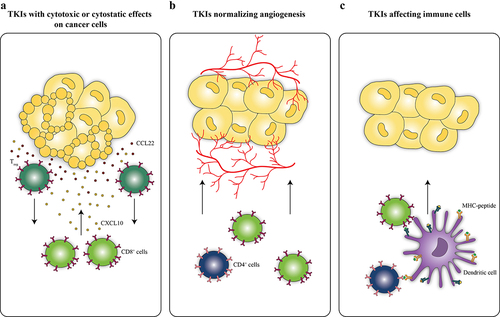
List of abbreviations
ALCL | = | Anaplastic large cell lymphoma |
ALK | = | Anaplastic lymphoma kinase |
ALL | = | Acute lymphoblastic leukemia |
AML | = | Acute myeloid leukemia |
BC | = | Breast cancer |
BCMA | = | B-cell maturation antigen |
BCR-Abl | = | Breakpoint cluster region-Abelson |
BTK | = | Bruton’s tyrosine kinase |
CAR | = | Chimeric antigen receptor |
CCL | = | C-C motif chemokine ligand |
CLL | = | Chronic lymphocytic leukemia |
CML | = | Chronic myelogenous leukemia |
CNS | = | Central nervous system |
CRC | = | Colorectal cancer |
CSF1R | = | Colony stimulating factor 1 receptor |
CTLA-4 | = | Cytotoxic T lymphocyte antigen 4 |
CTX | = | Cyclophosphamide |
CXCL | = | C-X-C motif chemokine ligand |
DC | = | Dendritic cell |
D-CIK | = | Dendritic and cytokine-induced killer cell |
DFMO | = | Difluoromethylornithine |
DLBCL | = | Diffuse large B-cell lymphoma |
EBV | = | Epstein–Barr virus |
EGF | = | Epidermal growth factor |
EGFR | = | Epidermal growth factor receptor |
FGFR | = | Fibroblast growth factor receptor |
FLT3 | = | Fms like tyrosine kinase 3 |
G-CSF | = | Granulocyte colony-stimulating factor |
GM-CSF | = | Granulocyte-macrophage colony-stimulating factor |
GIST | = | Gastrointestinal stromal tumor |
HAIC | = | Hepatic arterial infusion chemotherapy |
HER | = | Human epidermal growth factor receptor |
HCC | = | Hepatocellular carcinoma |
HIV | = | Human immunodeficiency virus |
HL | = | Hodgkin lymphoma |
IDO | = | Indoleamine-pyrrole 2,3-dioxygenase |
IGFR | = | Insulin-like growth factor receptor |
IL | = | Interleukin |
ILT-4 | = | Immunoglobulin-like transcript 4 |
InsR | = | Insulin receptor |
IFN | = | Interferon |
JAK | = | Janus kinase |
LAG-3 | = | Lymphocyte-activation gene 3 |
LLy | = | Lymphoblastic lymphoma |
LTK | = | Leukocyte receptor tyrosine kinase |
MCL | = | Mantle cell lymphoma |
MM | = | Multiple myeloma |
NHL | = | Non-Hodgkin lymphoma |
NIS | = | Sodium iodine symporter |
NOS | = | Not other specified |
NSCLC | = | Non-small-cell lung carcinoma |
OS | = | Overall survival |
PC | = | Pancreatic cancer |
PEG | = | Polyethylene glycol |
PD-1 | = | Programmed cell death protein 1 |
PDGFR | = | Platelet-derived growth factor receptor |
PD-L1 | = | Programmed cell death protein 1 |
PFS | = | Progression free survival |
RCC | = | Renal cell carcinoma |
RET | = | Rearranged during transfection |
SLL | = | Small lymphocytic lymphoma |
STAT | = | Signal transducer and activator of transcription |
TACE | = | Transarterial chemoembolization |
TC | = | Thyroid carcinoma |
TCC | = | Transitional cell carcinoma |
TIGIT | = | T cell immunoreceptor with Ig and ITIM domains |
TIL | = | Tumor infiltrating lymphocyte |
TLR | = | Toll-like receptor |
TNBC | = | Triple-negative breast cancer |
VEGFR | = | Vascular endothelial growth factor receptor |
VSV | = | Vesicular stomatitis virus |
Acknowledgments
OK is supported by the DIM Elicit of the Ile de France and the Institut National du Cancer (INCa). GK is supported by the Ligue contre le Cancer (équipe labellisée); Agence National de la Recherche (ANR) – Projets blancs; AMMICa US23/CNRS UMS3655; Association pour la recherche sur le cancer (ARC); Cancéropôle Ile-de-France; Fondation pour la Recherche Médicale (FRM); a donation by Elior; Equipex Onco-Pheno-Screen; European Joint Programme on Rare Diseases (EJPRD); Gustave Roussy Odyssea, the European Union Horizon 2020 Projects Oncobiome and Crimson; Fondation Carrefour; Institut National du Cancer (INCa); Institut Universitaire de France; LabEx Immuno-Oncology (ANR-18-IDEX-0001); a Cancer Research ASPIRE Award from the Mark Foundation; the RHU Immunolife; Seerave Foundation; SIRIC Stratified Oncology Cell DNA Repair and Tumor Immune Elimination (SOCRATE); and SIRIC Cancer Research and Personalized Medicine (CARPEM). This study contributes to the IdEx Université de Paris ANR-18-IDEX-0001. MCM is supported by CARPEM. AP has been supported by European Union Horizon 2020 Marie Skłodowska-Curie Innovative Training Network (ITN-ETN) Grant, Award No.: 675712 and the Fondation “ARC pour la recherche sur le cancer”.
Disclosure statement
OK is a scientific co-founder of Samsara. GK has been holding research contracts with Daiichi Sankyo, Eleor, Kaleido, Lytix Pharma, PharmaMar, Samsara, Sanofi, Sotio, Tollys, Vascage and Vasculox/Tioma. GK has been consulting for Reithera. GK is on the Board of Directors of the Bristol Myers Squibb Foundation France. GK is a scientific co-founder of everImmune, Osasuna Therapeutics, Samsara Therapeutics and Therafast Bio. GK is the inventor of patents covering therapeutic targeting of aging, cancer, cystic fibrosis and metabolic disorders. MCM is named as an inventor on a patent describing a method for the diagnosis and prognosis of breast cancer.
Data availability statement
The data that support the findings of this study are openly available in clinicaltrials.gov at https://clinicaltrials.gov under the reference numbers cited in this article.
Additional information
Funding
References
- Braña MF, Cacho M, Gradillas A, de Pascual-Teresa B, Ramos A. Intercalators as anticancer drugs. Curr Pharm Des. 2001;7(17):1745–27. doi:10.2174/1381612013397113.
- Einhorn J. Nitrogen mustard: the origin of chemotherapy for cancer. Int J Radiat Oncol Biol Phys. 1985;11(7):1375–1378. doi:10.1016/0360-3016(85)90254-8.
- Martínez R, Chacón-García L. The search of DNA-intercalators as antitumoral drugs: what it worked and what did not work. Curr Med Chem. 2005;12(2):127–151. doi:10.2174/0929867053363414.
- Wintrobe MM, Huguley CMJ. Nitrogen-mustard therapy for Hodgkin’s disease, lymphosarcoma, the leukemias, and other disorders. Cancer. 1948;1(3):357–382. doi:10.1002/1097-0142(194809)1:3<357::aid-cncr2820010303>3.0.co;2-v.
- Yamazaki T, Wennerberg E, Hensler M, Buque A, Kraynak J, Fucikova J, Zhou XK, Sveinbjornsson B, Rekdal O, Demaria S, et al. LTX-315-enabled, radiotherapy-boosted immunotherapeutic control of breast cancer by NK cells. Oncoimmunology. 2021;10(1):1962592. doi:10.1080/2162402X.2021.1962592.
- Yamazaki T, Kirchmair A, Sato A, Buque A, Rybstein M, Petroni G, Bloy N, Finotello F, Stafford L, Manzano EN, et al. Mitochondrial DNA drives abscopal responses to radiation that are inhibited by autophagy. Nat Immunol. 2020;21(10):1160–1171. doi:10.1038/s41590-020-0751-0.
- Vitale I, Shema E, Loi S, Galluzzi L. Intratumoral heterogeneity in cancer progression and response to immunotherapy. Nat Med. 2021;27(2):212–224. doi:10.1038/s41591-021-01233-9.
- Tanchot C, Terme M, Pere H, Tran T, Benhamouda N, Strioga M, Banissi C, Galluzzi L, Kroemer G, Tartour E. Tumor-infiltrating regulatory T cells: phenotype, role, mechanism of expansion in situ and clinical significance. Cancer Microenviron. 2013;6(2):147–157. doi:10.1007/s12307-012-0122-y.
- Vanpouille-Box C, Demaria S, Formenti SC, Galluzzi L. Cytosolic DNA sensing in organismal tumor control. Cancer Cell. 2018;34(3):361–378. doi:10.1016/j.ccell.2018.05.013.
- Petroni G, Buque A, Yamazaki T, Bloy N, Liberto MD, Chen-Kiang S, Formenti SC, Galluzzi L. Radiotherapy delivered before CDK4/6 inhibitors mediates superior therapeutic effects in ER(+) breast cancer. Clin Cancer Res. 2021;27(7):1855–1863. doi:10.1158/1078-0432.CCR-20-3871.
- Petroni G, Buque A, Coussens LM, Galluzzi L. Targeting oncogene and non-oncogene addiction to inflame the tumour microenvironment. Nat Rev Drug Discov. 2022. doi:10.1038/s41573-022-00415-5.
- Kroemer G, Galassi C, Zitvogel L, Galluzzi L. Immunogenic cell stress and death. Nat Immunol. 2022;23(4):487–500. doi:10.1038/s41590-022-01132-2.
- Kepp O, Menger L, Vacchelli E, Locher C, Adjemian S, Yamazaki T, Martins I, Sukkurwala AQ, Michaud M, Senovilla L, et al. Crosstalk between ER stress and immunogenic cell death. Cytokine Growth Factor Rev. 2013;24(4):311–318. doi:10.1016/j.cytogfr.2013.05.001.
- Galluzzi L, Zitvogel L, Kroemer G. Immunological mechanisms underneath the efficacy of cancer therapy. Cancer Immunol Res. 2016;4(11):895–902. doi:10.1158/2326-6066.CIR-16-0197.
- Galluzzi L, Humeau J, Buque A, Zitvogel L, Kroemer G. Immunostimulation with chemotherapy in the era of immune checkpoint inhibitors. Nat Rev Clin Oncol. 2020;17(12):725–741. doi:10.1038/s41571-020-0413-z.
- Buque A, Bloy N, Perez-Lanzon M, Iribarren K, Humeau J, Pol JG, Levesque S, Mondragon L, Yamazaki T, Sato A, et al. Immunoprophylactic and immunotherapeutic control of hormone receptor-positive breast cancer. Nat Commun. 2020;11(1):3819. doi:10.1038/s41467-020-17644-0.
- Fucikova J, Palova-Jelinkova L, Klapp V, Holicek P, Lanickova T, Kasikova L, Drozenova J, Cibula D, Alvarez-Abril B, Garcia-Martinez E, et al. Immunological control of ovarian carcinoma by chemotherapy and targeted anticancer agents. Trends Cancer. 2022;8(5):426–444. doi:10.1016/j.trecan.2022.01.010.
- Aranda F, Vacchelli E, Eggermont A, Galon J, Fridman WH, Zitvogel L, Kroemer G, Galluzzi L. Trial watch: immunostimulatory monoclonal antibodies in cancer therapy. Oncoimmunology. 2014;3(1):e27297. doi:10.4161/onci.27297.
- Aranda F, Vacchelli E, Eggermont A, Galon J, Sautes-Fridman C, Tartour E, Zitvogel L, Kroemer G, Galluzzi L. Trial watch: peptide vaccines in cancer therapy. Oncoimmunology. 2013;2(12):e26621. doi:10.4161/onci.26621.
- Pimm ML, Hotaling J, Henty-Ridilla JL. Profilin choreographs actin and microtubules in cells and cancer. Int Rev Cell Mol Biol. 2020;355:155–204. doi:10.1016/bs.ircmb.2020.05.005.
- Pervushin N, Senichkin V, Zhivotovsky B, Kopeina GS. Mcl-1 as a “barrier” in cancer treatment: can we target it now? Int Rev Cell Mol Biol. 2020;351:23–55. doi:10.1016/bs.ircmb.2020.01.002.
- Glab JA, Cao Z, Puthalakath H. Bcl-2 family proteins, beyond the veil. Int Rev Cell Mol Biol. 2020;351:1–22. doi:10.1016/bs.ircmb.2019.12.001.
- Gillyard T, Davis J. DNA double-strand break repair in cancer: a path to achieving precision medicine. Int Rev Cell Mol Biol. 2021;364:111–137. doi:10.1016/bs.ircmb.2021.06.003.
- Rasmussen ML, Gama V. A connection in life and death: the BCL-2 family coordinates mitochondrial network dynamics and stem cell fate. Int Rev Cell Mol Biol. 2020;353:255–284. doi:10.1016/bs.ircmb.2019.12.005.
- Zhong L, Li Y, Xiong L, Wang W, Wu M, Yuan T, Yang W, Tian C, Miao Z, Wang T, et al. Small molecules in targeted cancer therapy: advances, challenges, and future perspectives. Signal Transduct Target Ther. 2021;6(1):201. doi:10.1038/s41392-021-00572-w.
- Baudino TA. Targeted cancer therapy: the next generation of cancer treatment. Curr Drug Discov Technol. 2015;12(1):3–20. doi:10.2174/1570163812666150602144310.
- Maso K, Grigoletto A, Vicent MJ, Pasut G. Molecular platforms for targeted drug delivery. Int Rev Cell Mol Biol. 2019;346:1–50. doi:10.1016/bs.ircmb.2019.03.001.
- Ferguson FM, Gray NS. Kinase inhibitors: the road ahead. Nat Rev Drug Discov. 2018;17(5):353–377. doi:10.1038/nrd.2018.21.
- Pottier C, Fresnais M, Gilon M, Jérusalem G, Longuespée R, Sounni NE. Tyrosine kinase inhibitors in cancer: breakthrough and challenges of targeted therapy. Cancers. 2020;12(3):731. doi:10.3390/cancers12030731.
- Cohen MH, Williams G, Johnson JR, Duan J, Gobburu J, Rahman A, Benson K, Leighton J, Kim SK, Wood R, et al. Approval summary for imatinib mesylate capsules in the treatment of chronic myelogenous leukemia. Clin Cancer Res. 2002;8(5):935–942.
- Capdeville R, Buchdunger E, Zimmermann J, Matter A. Glivec (STI571, imatinib), a rationally developed, targeted anticancer drug. Nat Rev Drug Discov. 2002;1(7):493–502. doi:10.1038/nrd839.
- Cohen P, Cross D, Jänne PA. Kinase drug discovery 20 years after imatinib: progress and future directions. Nat Rev Drug Discov. 2021;20(7):551–569. doi:10.1038/s41573-021-00195-4.
- Roskoski RJ. Properties of FDA-approved small molecule protein kinase inhibitors: a 2021 update. Pharmacol Res. 2021;165:105463. doi:10.1016/j.phrs.2021.105463.
- Tan AC, Tan DSW. Targeted therapies for lung cancer patients with oncogenic driver molecular alterations. J Clin Oncol. 2022;40(6):611–625. doi:10.1200/JCO.21.01626.
- Robinson DR, Wu YM, Lin SF. The protein tyrosine kinase family of the human genome. Oncogene. 2000;19(49):5548–5557. doi:10.1038/sj.onc.1203957.
- Lemmon MA, Schlessinger J. Cell signaling by receptor tyrosine kinases. Cell. 2010;141(7):1117–1134. doi:10.1016/j.cell.2010.06.011.
- Gocek E, Moulas AN, Studzinski GP. Non-receptor protein tyrosine kinases signaling pathways in normal and cancer cells. Crit Rev Clin Lab Sci. 2014;51(3):125–137. doi:10.3109/10408363.2013.874403.
- Muhsin M, Graham J, Kirkpatrick P. Gefitinib. Nat Rev Drug Discov. 2003;2(7):515–516. doi:10.1038/nrd1136.
- Cohen MH, Williams GA, Sridhara R, Chen G, McGuinn WDJ, Morse D, Abraham S, Rahman A, Liang C, Lostritto R, et al. United States food and drug administration drug approval summary: gefitinib (ZD1839; Iressa) tablets. Clin Cancer Res. 2004;10(4):1212–1218. doi:10.1158/1078-0432.ccr-03-0564.
- Minna JD, Dowell J. Erlotinib hydrochloride. Nat Rev Drug Discov. 2005;Suppl:S14–5. doi:10.1038/nrd1612.
- Johnson JR, Cohen M, Sridhara R, Chen Y-F, Williams GM, Duan J, Gobburu J, Booth B, Benson K, Leighton J, et al. Approval summary for erlotinib for treatment of patients with locally advanced or metastatic non-small cell lung cancer after failure of at least one prior chemotherapy regimen. Clin Cancer Res. 2005;11(18):6414–6421. doi:10.1158/1078-0432.CCR-05-0790.
- Kane RC, Farrell AT, Saber H, Tang S, Williams G, Jee JM, Liang C, Booth B, Chidambaram N, Morse D, et al. Sorafenib for the treatment of advanced renal cell carcinoma. Clin Cancer Res. 2006;12(24):7271–7278. doi:10.1158/1078-0432.CCR-06-1249.
- Atkins M, Jones CA, Kirkpatrick P. Sunitinib maleate. Nat Rev Drug Discov. 2006;5(4):279–280. doi:10.1038/nrd2012.
- Goodman VL, Rock EP, Dagher R, Ramchandani RP, Abraham S, Gobburu JVS, Booth BP, Verbois SL, Morse DE, Liang CY, et al. Approval summary: sunitinib for the treatment of imatinib refractory or intolerant gastrointestinal stromal tumors and advanced renal cell carcinoma. Clin Cancer Res. 2007;13(5):1367–1373. doi:10.1158/1078-0432.CCR-06-2328.
- Moy B, Kirkpatrick P, Kar S, Goss P. Lapatinib. Nat Rev Drug Discov. 2007;6(6):431–432. doi:10.1038/nrd2332.
- Ryan Q, Ibrahim A, Cohen MH, Johnson J, Ko C, Sridhara R, Justice R, Pazdur R. FDA drug approval summary: lapatinib in combination with capecitabine for previously treated metastatic breast cancer that overexpresses HER-2. Oncologist. 2008;13(10):1114–1119. doi:10.1634/theoncologist.2008-0816.
- Bukowski RM, Yasothan U, Kirkpatrick P. Pazopanib. Nat Rev Drug Discov. 2010;9(1):17–18. doi:10.1038/nrd3073.
- Shaw AT, Yasothan U, Kirkpatrick P. Crizotinib. Nat Rev Drug Discov. 2011;10(12):897–898. doi:10.1038/nrd3600.
- Kazandjian D, Blumenthal GM, Chen H-Y, He K, Patel M, Justice R, Keegan P, Pazdur R. FDA approval summary: crizotinib for the treatment of metastatic non-small cell lung cancer with anaplastic lymphoma kinase rearrangements. Oncologist. 2014;19(10):e5–11. doi:10.1634/theoncologist.2014-0241.
- Dungo RT, Keating GM. Afatinib: first global approval. Drugs. 2013;73(13):1503–1515. doi:10.1007/s40265-013-0111-6.
- Khozin S, Blumenthal GM, Zhang L, Tang S, Brower M, Fox E, Helms W, Leong R, Song P, Pan Y, et al. FDA approval: ceritinib for the treatment of metastatic anaplastic lymphoma kinase-positive non-small cell lung cancer. Clin Cancer Res. 2015;21(11):2436–2439. doi:10.1158/1078-0432.CCR-14-3157.
- Larkins E, Blumenthal GM, Chen H, He K, Agarwal R, Gieser G, Stephens O, Zahalka E, Ringgold K, Helms W, et al. FDA approval: alectinib for the treatment of metastatic, ALK-positive non-small cell lung cancer following crizotinib. Clin Cancer Res. 2016;22(21):5171–5176. doi:10.1158/1078-0432.CCR-16-1293.
- Nair A, Lemery SJ, Yang J, Marathe A, Zhao L, Zhao H, Jiang X, He K, Ladouceur G, Mitra AK, et al. FDA approval summary: lenvatinib for progressive, radio-iodine-refractory differentiated thyroid cancer. Clin Cancer Res. 2015;21(23):5205–5208. doi:10.1158/1078-0432.CCR-15-1377.
- Greig SL. Osimertinib: first global approval. Drugs. 2016;76(2):263–273. doi:10.1007/s40265-015-0533-4.
- Singh H, Walker AJ, Amiri-Kordestani L, Cheng J, Tang S, Balcazar P, Barnett-Ringgold K, Palmby TR, Cao X, Zheng N, et al. U.S. food and drug administration approval: neratinib for the extended adjuvant treatment of early-stage HER2-positive breast cancer. Clin Cancer Res. 2018;24(15):3486–3491. doi:10.1158/1078-0432.CCR-17-3628.
- Markham A. Brigatinib: first global approval. Drugs. 2017;77(10):1131–1135. doi:10.1007/s40265-017-0776-3.
- Chang E, Weinstock C, Zhang L, Fiero MH, Zhao M, Zahalka E, Ricks TK, Fourie Zirkelbach J, Qiu J, Yu J, et al. FDA approval summary: tivozanib for relapsed or refractory renal cell carcinoma. Clin Cancer Res. Aug 2021. doi:10.1158/1078-0432.CCR-21-2334.
- Shirley M. Dacomitinib: first global approval. Drugs. 2018;78(18):1947–1953. doi:10.1007/s40265-018-1028-x.
- Syed YY. Lorlatinib: first global approval. Drugs. 2019;79(1):93–98. doi:10.1007/s40265-018-1041-0.
- Scott LJ. Larotrectinib: first global approval. Drugs. 2019;79(2):201–206. doi:10.1007/s40265-018-1044-x.
- Pulte ED, Norsworthy KJ, Wang Y, Xu Q, Qosa H, Gudi R, Przepiorka D, Fu W, Okusanya OO, Goldberg KB, et al. FDA approval summary: gilteritinib for relapsed or refractory acute myeloid leukemia with a FLT3 mutation. Clin Cancer Res. 2021;27(13):3515–3521. doi:10.1158/1078-0432.CCR-20-4271.
- Markham A. Erdafitinib: first global approval. Drugs. 2019;79(9):1017–1021. doi:10.1007/s40265-019-01142-9.
- Lamb YN. Pexidartinib: first approval. Drugs. 2019;79(16):1805–1812. doi:10.1007/s40265-019-01210-0.
- Marcus L, Donoghue M, Aungst S, Myers CE, Helms WS, Shen G, Zhao H, Stephens O, Keegan P, Pazdur R. FDA approval summary: entrectinib for the treatment of NTRK gene fusion solid tumors. Clin Cancer Res. 2021;27(4):928–932. doi:10.1158/1078-0432.CCR-20-2771.
- Cocco E, Scaltriti M, Drilon A. NTRK fusion-positive cancers and TRK inhibitor therapy. Nat Rev Clin Oncol. 2018;15(12):731–747. doi:10.1038/s41571-018-0113-0.
- Hoy SM. Pemigatinib: first approval. Drugs. 2020;80(9):923–929. doi:10.1007/s40265-020-01330-y.
- Mathieu LN, Larkins E, Akinboro O, Roy P, Amatya AK, Fiero MH, Mishra-Kalyani PS, Helms WS, Myers CE, Skinner AM, et al. FDA approval summary: capmatinib and tepotinib for the treatment of metastatic NSCLC harboring MET Exon 14 skipping mutations or alterations. Clin Cancer Res. 2022;28(2):249–254. doi:10.1158/1078-0432.CCR-21-1566.
- Bradford D, Larkins E, Mushti SL, Rodriguez L, Skinner AM, Helms WS, Price LSL, Zirkelbach JF, Li Y, Liu J, et al. FDA approval summary: selpercatinib for the treatment of lung and thyroid cancers with RET gene mutations or fusions. Clin Cancer Res. 2021;27(8):2130–2135. doi:10.1158/1078-0432.CCR-20-3558.
- Dhillon S. Ripretinib: first approval. Drugs. 2020;80(11):1133–1138. doi:10.1007/s40265-020-01348-2.
- Kim J, Bradford D, Larkins E, Pai-Scherf LH, Chatterjee S, Mishra-Kalyani PS, Wearne E, Helms WS, Ayyoub A, Bi Y, et al. FDA approval summary: pralsetinib for the treatment of lung and thyroid cancers with RET gene mutations or fusions. Clin Cancer Res. 2021;27(20):5452–5456. doi:10.1158/1078-0432.CCR-21-0967.
- Markham A. Tepotinib: first approval. Drugs. 2020;80(8):829–833. doi:10.1007/s40265-020-01317-9.
- Dagher R, Cohen M, Williams G, Rothmann M, Gobburu J, Robbie G, Rahman A, Chen G, Staten A, Griebel D, et al. Approval summary: imatinib mesylate in the treatment of metastatic and/or unresectable malignant gastrointestinal stromal tumors. Clin Cancer Res. 2002;8(10):3034–3038.
- Kantarjian H, Jabbour E, Grimley J, Kirkpatrick P. Dasatinib. Nat Rev Drug Discov. 2006;5(9):717–718. doi:10.1038/nrd2135.
- Mesa RA, Yasothan U, Kirkpatrick P. Ruxolitinib. Nat Rev Drug Discov. 2012;11(2):103–104. doi:10.1038/nrd3652.
- Deisseroth A, Kaminskas E, Grillo J, Chen W, Saber H, Lu HL, Rothmann MD, Brar S, Wang J, Garnett C, et al. U.S. food and drug administration approval: ruxolitinib for the treatment of patients with intermediate and high-risk myelofibrosis. Clin Cancer Res. 2012;18(12):3212–3217. doi:10.1158/1078-0432.CCR-12-0653.
- de Claro Ra, McGinn KM, Verdun N, Lee S-L, Chiu H-J, Saber H, Brower ME, Chang CJG, Pfuma E, Habtemariam B, et al. FDA approval: ibrutinib for patients with previously treated mantle cell lymphoma and previously treated chronic lymphocytic leukemia. Clin Cancer Res. 2015;21(16):3586–3590. doi:10.1158/1078-0432.CCR-14-2225.
- Hasford J, Baccarani M, Hoffmann V, Guilhot J, Saussele S, Rosti G, Guilhot F, Porkka K, Ossenkoppele G, Lindoerfer D, et al. Predicting complete cytogenetic response and subsequent progression-free survival in 2060 patients with CML on imatinib treatment: the EUTOS score. Blood. 2011;118(3):686–692. doi:10.1182/blood-2010-12-319038.
- Hochhaus A, Baccarani M, Silver RT, Schiffer C, Apperley JF, Cervantes F, Clark RE, Cortes JE, Deininger MW, Guilhot F, et al. European leukemiaNet 2020 recommendations for treating chronic myeloid leukemia. Leukemia. 2020;34(4):966–984. doi:10.1038/s41375-020-0776-2.
- Jabbour E, Kantarjian H. Chronic myeloid leukemia: 2020 update on diagnosis, therapy and monitoring. Am J Hematol. 2020;95(6):691–709. doi:10.1002/ajh.25792.
- Kreutzman A, Yadav B, Brummendorf TH, Gjertsen BT, Hee Lee M, Janssen J, Kasanen T, Koskenvesa P, Lotfi K, Markevärn B, et al. Immunological monitoring of newly diagnosed CML patients treated with bosutinib or imatinib first-line. Oncoimmunology. 2019;8(9):e1638210. doi:10.1080/2162402X.2019.1638210.
- Blay J-Y, Kang Y-K, Nishida T, von Mehren M. Gastrointestinal stromal tumours. Nat Rev Dis Primers. 2021;7(1):22. doi:10.1038/s41572-021-00254-5.
- Verweij J, Casali PG, Zalcberg J, LeCesne A, Reichardt P, Blay J-Y, Issels R, van Oosterom A, Hogendoorn PCW, Van Glabbeke M, et al. Progression-free survival in gastrointestinal stromal tumours with high-dose imatinib: randomised trial. Lancet. 2004;364(9440):1127–1134. doi:10.1016/S0140-6736(04)17098-0.
- Hjorth-Hansen H, Stenke L, Söderlund S, Dreimane A, Ehrencrona H, Gedde-Dahl T, Gjertsen BT, Höglund M, Koskenvesa P, Lotfi K, et al. Dasatinib induces fast and deep responses in newly diagnosed chronic myeloid leukaemia patients in chronic phase: clinical results from a randomised phase-2 study (NordCML006). Eur J Haematol. 2015;94(3):243–250. doi:10.1111/ejh.12423.
- Gresse M, Kim TD, le Coutre P. Nilotinib. recent results in cancer research. Fortschritte der Krebsforschung. 2018;212:69–85. doi:10.1007/978-3-319-91439-8_3.
- Jain P, Kantarjian H, Alattar ML, Jabbour E, Sasaki K, Nogueras Gonzalez G, Dellasala S, Pierce S, Verstovsek S, Wierda W, et al. Long-term molecular and cytogenetic response and survival outcomes with imatinib 400 mg, imatinib 800 mg, dasatinib, and nilotinib in patients with chronic-phase chronic myeloid leukaemia: retrospective analysis of patient data from five clinical trials. Lancet Haematol. 2015;2(3):e118–28. doi:10.1016/S2352-3026(15)00021-6.
- Cortes JE, Kim D-W, Pinilla-Ibarz J, le Coutre P, Paquette R, Chuah C, Nicolini FE, Apperley JF, Khoury HJ, Talpaz M, et al. A phase 2 trial of ponatinib in Philadelphia chromosome-positive leukemias. N Engl J Med. 2013;369(19):1783–1796. doi:10.1056/NEJMoa1306494.
- Vener C, Banzi R, Ambrogi F, Ferrero A, Saglio G, Pravettoni G, Sant M. First-line imatinib vs second- and third-generation TKIs for chronic-phase CML: a systematic review and meta-analysis. Blood Adv. 2020;4(12):2723–2735. doi:10.1182/bloodadvances.2019001329.
- Skoulidis F, Heymach JV. Co-occurring genomic alterations in non-small-cell lung cancer biology and therapy. Nat Rev Cancer. 2019;19(9):495–509. doi:10.1038/s41568-019-0179-8.
- Fukuoka M, Y-L W, Thongprasert S, Sunpaweravong P, Leong -S-S, Sriuranpong V, Chao T-Y, Nakagawa K, Chu D-T, Saijo N, et al. Biomarker analyses and final overall survival results from a phase III, randomized, open-label, first-line study of gefitinib versus carboplatin/paclitaxel in clinically selected patients with advanced non-small-cell lung cancer in Asia (IPASS). J Clin Oncol. 2011;29(21):2866–2874. doi:10.1200/JCO.2010.33.4235.
- Rosell R, Carcereny E, Gervais R, Vergnenegre A, Massuti B, Felip E, Palmero R, Garcia-Gomez R, Pallares C, Sanchez JM, et al. Erlotinib versus standard chemotherapy as first-line treatment for European patients with advanced EGFR mutation-positive non-small-cell lung cancer (EURTAC): a multicentre, open-label, randomised phase 3 trial. Lancet Oncol. 2012;13(3):239–246. doi:10.1016/S1470-2045(11)70393-X.
- Krawczyk P, Kowalski DM, Ramlau R, Kalinka-Warzocha E, Winiarczyk K, Stencel K, Powrózek T, Reszka K, Wojas-Krawczyk K, Bryl M, et al. Comparison of the effectiveness of erlotinib, gefitinib, and Afatinib for treatment of non-small cell lung cancer in patients with common and rare EGFR gene mutations. Oncol Lett. 2017;13(6):4433–4444. doi:10.3892/ol.2017.5980.
- Remon J, Steuer CE, Ramalingam SS, Felip E. Osimertinib and other third-generation EGFR TKI in EGFR-mutant NSCLC patients. Ann Oncol. 2018;29(suppl_1):i20–i27. doi:10.1093/annonc/mdx704.
- Soria JC, Ramalingam SS. Osimertinib in EGFR mutation-positive advanced NSCLC. N Engl J Med. 2018;378(13):1262–1263. doi:10.1056/NEJMc1801669.
- Ramalingam SS, Vansteenkiste J, Planchard D, Cho BC, Gray JE, Ohe Y, Zhou C, Reungwetwattana T, Cheng Y, Chewaskulyong B, et al. Overall survival with osimertinib in untreated, EGFR-mutated advanced NSCLC. N Engl J Med. 2020;382(1):41–50. doi:10.1056/NEJMoa1913662.
- Tefferi A. Primary myelofibrosis: 2021 update on diagnosis, risk-stratification and management. Am J Hematol. 2021;96(1):145–162. doi:10.1002/ajh.26050.
- Cervantes F, Pereira A. Does ruxolitinib prolong the survival of patients with myelofibrosis? Blood. 2017;129(7):832–837. doi:10.1182/blood-2016-11-731604.
- Verstovsek S, Mesa RA, Gotlib J, Levy RS, Gupta V, DiPersio JF, Catalano JV, Deininger M, Miller C, Silver RT, et al. A double-blind, placebo-controlled trial of ruxolitinib for myelofibrosis. N Engl J Med. 2012;366(9):799–807. doi:10.1056/NEJMoa1110557.
- Harrison C, Kiladjian -J-J, Al-Ali HK, Gisslinger H, Waltzman R, Stalbovskaya V, McQuitty M, Hunter DS, Levy R, Knoops L, et al. JAK inhibition with ruxolitinib versus best available therapy for myelofibrosis. N Engl J Med. 2012;366(9):787–798. doi:10.1056/NEJMoa1110556.
- Elliott J, Bai Z, Hsieh S-C, Kelly SE, Chen L, Skidmore B, Yousef S, Zheng C, Stewart DJ, Wells GA. ALK inhibitors for non-small cell lung cancer: a systematic review and network meta-analysis. PLoS One. 2020;15(2):e0229179. doi:10.1371/journal.pone.0229179.
- Solomon BJ, Besse B, Bauer TM, Felip E, Soo RA, Camidge DR, Chiari R, Bearz A, Lin -C-C, Gadgeel SM, et al. Lorlatinib in patients with ALK-positive non-small-cell lung cancer: results from a global phase 2 study. Lancet Oncol. 2018;19(12):1654–1667. doi:10.1016/S1470-2045(18)30649-1.
- Felip E, Shaw AT, Bearz A, Camidge DR, Solomon BJ, Bauman JR, Bauer TM, Peters S, Toffalorio F, Abbattista A, et al. Intracranial and extracranial efficacy of lorlatinib in patients with ALK-positive non-small-cell lung cancer previously treated with second-generation ALK TKIs. Ann Oncol. 2021;32(5):620–630. doi:10.1016/j.annonc.2021.02.012.
- Tevaarwerk AJ, Kolesar JM. Lapatinib: a small-molecule inhibitor of epidermal growth factor receptor and human epidermal growth factor receptor-2 tyrosine kinases used in the treatment of breast cancer. Clin Ther. 2009;31(Pt 2):2332–2348. doi:10.1016/j.clinthera.2009.11.029.
- Kroep JR, Linn SC, Boven E, Bloemendal HJ, Baas J, Mandjes IAM, van den Bosch J, Smit WM, de Graaf H, Schröder CP, et al. Lapatinib: clinical benefit in patients with HER 2-positive advanced breast cancer. Neth J Med. 2010;68(9):371–376.
- Saura C, Oliveira M, Feng Y-H, Dai M-S, Chen S-W, Hurvitz SA, Kim S-B, Moy B, Delaloge S, Gradishar W, et al. Neratinib plus capecitabine versus lapatinib plus capecitabine in HER2-positive metastatic breast cancer previously treated with ≥ 2 HER2-directed regimens: phase III NALA trial. J Clin Oncol. 2020;38(27):3138–3149. doi:10.1200/JCO.20.00147.
- Escudier B, Eisen T, Stadler WM, Szczylik C, Oudard S, Siebels M, Negrier S, Chevreau C, Solska E, Desai AA, et al. Sorafenib in advanced clear-cell renal-cell carcinoma. N Engl J Med. 2007;356(2):125–134. doi:10.1056/NEJMoa060655.
- Llovet JM, Ricci S, Mazzaferro V, Hilgard P, Gane E, Blanc J-F, de Oliveira AC, Santoro A, Raoul J-L, Forner A, et al. Sorafenib in advanced hepatocellular carcinoma. N Engl J Med. 2008;359(4):378–390. doi:10.1056/NEJMoa0708857.
- Escudier B, Worden F, Kudo M. Sorafenib: key lessons from over 10 years of experience. Expert Rev Anticancer Ther. 2019;19(2):177–189. doi:10.1080/14737140.2019.1559058.
- Demetri GD, van Oosterom AT, Garrett CR, Blackstein ME, Shah MH, Verweij J, McArthur G, Judson IR, Heinrich MC, Morgan JA, et al. Efficacy and safety of sunitinib in patients with advanced gastrointestinal stromal tumour after failure of imatinib: a randomised controlled trial. Lancet. 2006;368(9544):1329–1338. doi:10.1016/S0140-6736(06)69446-4.
- Rini BI, Plimack ER, Stus V, Gafanov R, Hawkins R, Nosov D, Pouliot F, Alekseev B, Soulières D, Melichar B, et al. Pembrolizumab plus axitinib versus sunitinib for advanced renal-cell carcinoma. N Engl J Med. 2019;380(12):1116–1127. doi:10.1056/NEJMoa1816714.
- Nassif E, Thibault C, Vano Y, Fournier L, Mauge L, Verkarre V, Timsit M-O, Mejean A, Tartour E, Oudard S. Sunitinib in kidney cancer: 10 years of experience and development. Expert Rev Anticancer Ther. 2017;17(2):129–142. doi:10.1080/14737140.2017.1272415.
- Rini BI, Escudier B, Tomczak P, Kaprin A, Szczylik C, Hutson TE, Michaelson MD, Gorbunova VA, Gore ME, Rusakov IG, et al. Comparative effectiveness of axitinib versus sorafenib in advanced renal cell carcinoma (AXIS): a randomised phase 3 trial. Lancet. 2011;378(9807):1931–1939. doi:10.1016/S0140-6736(11)61613-9.
- Villanueva MT. Urological cancer: an axis for renal cell carcinoma treatment. Nat Rev Clin Oncol. 2011;9(1):5. doi:10.1038/nrclinonc.2011.180.
- Elisei R, Schlumberger MJ, Müller SP, Schöffski P, Brose MS, Shah MH, Licitra L, Jarzab B, Medvedev V, Kreissl MC, et al. Cabozantinib in progressive medullary thyroid cancer. J Clin Oncol. 2013;31(29):3639–3646. doi:10.1200/JCO.2012.48.4659.
- Schlumberger M, Elisei R, Müller S, Schöffski P, Brose M, Shah M, Licitra L, Krajewska J, Kreissl MC, Niederle B, et al. Overall survival analysis of EXAM, a phase III trial of cabozantinib in patients with radiographically progressive medullary thyroid carcinoma. Ann Oncol. 2017;28(11):2813–2819. doi:10.1093/annonc/mdx479.
- Brose MS, Robinson B, Sherman SI, Krajewska J, Lin -C-C, Vaisman F, Hoff AO, Hitre E, Bowles DW, Hernando J, et al. Cabozantinib for radioiodine-refractory differentiated thyroid cancer (COSMIC-311): a randomised, double-blind, placebo-controlled, phase 3 trial. Lancet Oncol. 2021;22(8):1126–1138. doi:10.1016/S1470-2045(21)00332-6.
- D’Alessio A, Prete MG, Cammarota A, Personeni N, Rimassa L. The role of cabozantinib as a therapeutic option for hepatocellular carcinoma: current landscape and future challenges. J Hepatocell Carcinoma. 2021;8:177–191. doi:10.2147/JHC.S268310.
- Zhang T, Park SE, Hong C, George DJ. Cabozantinib in genitourinary malignancies. Future Oncol. 2017;13(8):755–765. doi:10.2217/fon-2016-0358.
- Abdelaziz A, Vaishampayan U. Cabozantinib for the treatment of kidney cancer. Expert Rev Anticancer Ther. 2017;17(7):577–584. doi:10.1080/14737140.2017.1344553.
- Demetri GD, Reichardt P, Kang Y-K, Blay J-Y, Rutkowski P, Gelderblom H, Hohenberger P, Leahy M, von Mehren M, Joensuu H, et al. Efficacy and safety of regorafenib for advanced gastrointestinal stromal tumours after failure of imatinib and sunitinib (GRID): an international, multicentre, randomised, placebo-controlled, phase 3 trial. Lancet. 2013;381(9863):295–302. doi:10.1016/S0140-6736(12)61857-1.
- Kudo M, Finn RS, Qin S, Han K-H, Ikeda K, Piscaglia F, Baron A, Park J-W, Han G, Jassem J, et al. Lenvatinib versus sorafenib in first-line treatment of patients with unresectable hepatocellular carcinoma: a randomised phase 3 non-inferiority trial. Lancet. 2018;391(10126):1163–1173. doi:10.1016/S0140-6736(18)30207-1.
- Vogel A, Qin S, Kudo M, Su Y, Hudgens S, Yamashita T, Yoon J-H, Fartoux L, Simon K, López C, et al. Lenvatinib versus sorafenib for first-line treatment of unresectable hepatocellular carcinoma: patient-reported outcomes from a randomised, open-label, non-inferiority, phase 3 trial. Lancet Gastroenterol Hepatol. 2021;6(8):649–658. doi:10.1016/S2468-1253(21)00110-2.
- Motzer RJ, Hutson TE, Glen H, Michaelson MD, Molina A, Eisen T, Jassem J, Zolnierek J, Maroto JP, Mellado B, et al. Lenvatinib, everolimus, and the combination in patients with metastatic renal cell carcinoma: a randomised, phase 2, open-label, multicentre trial. Lancet Oncol. 2015;16(15):1473–1482. doi:10.1016/S1470-2045(15)00290-9.
- Schlumberger M, Tahara M, Wirth LJ, Robinson B, Brose MS, Elisei R, Habra MA, Newbold K, Shah MH, Hoff AO, et al. Lenvatinib versus placebo in radioiodine-refractory thyroid cancer. N Engl J Med. 2015;372(7):621–630. doi:10.1056/NEJMoa1406470.
- Hao Z, Wang P. Lenvatinib in management of solid tumors. Oncologist. 2020;25(2):e302–e310. doi:10.1634/theoncologist.2019-0407.
- Woyach JA, Ruppert AS, Heerema NA, Zhao W, Booth AM, Ding W, Bartlett NL, Brander DM, Barr PM, Rogers KA, et al. Ibrutinib regimens versus chemoimmunotherapy in older patients with untreated CLL. N Engl J Med. 2018;379(26):2517–2528. doi:10.1056/NEJMoa1812836.
- Byrd JC, Wierda WG, Schuh A, Devereux S, Chaves JM, Brown JR, Hillmen P, Martin P, Awan FT, Stephens DM, et al. Acalabrutinib monotherapy in patients with relapsed/refractory chronic lymphocytic leukemia: updated phase 2 results. Blood. 2020;135(15):1204–1213. doi:10.1182/blood.2018884940.
- Burger JA. Bruton tyrosine kinase inhibitors: present and future. Cancer J. 2019;25(6):386–393. doi:10.1097/PPO.0000000000000412.
- Liu J, Chen C, Wang D, Zhang J, Zhang T. Emerging small-molecule inhibitors of the Bruton’s tyrosine kinase (BTK): current development. Eur J Med Chem. 2021;217:113329. doi:10.1016/j.ejmech.2021.113329.
- Rosenzweig SA. Acquired resistance to drugs targeting tyrosine kinases. Adv Cancer Res. 2018;138:71–98. doi:10.1016/bs.acr.2018.02.003.
- Lovly CM, Shaw AT. Molecular pathways: resistance to kinase inhibitors and implications for therapeutic strategies. Clin Cancer Res. 2014;20(9):2249–2256. doi:10.1158/1078-0432.CCR-13-1610.
- Quintanal-Villalonga Á, Chan JM, Yu HA, Pe’er D, Sawyers CL, Sen T, Rudin CM. Lineage plasticity in cancer: a shared pathway of therapeutic resistance. Nat Rev Clin Oncol. 2020;17(6):360–371. doi:10.1038/s41571-020-0340-z.
- Raedler LA. Opdivo (Nivolumab): second PD-1 inhibitor receives FDA approval for unresectable or metastatic melanoma. Am Health Drug Benefits. 2015;8:180–183.
- Raedler LA. Keytruda (pembrolizumab): first PD-1 inhibitor approved for previously treated unresectable or metastatic melanoma. Am Health Drug Benefits. 2015;8:96–100.
- Ascierto PA, Butterfield LH, Campbell K, Daniele B, Dougan M, Emens LA, Formenti S, Janku F, Khleif SN, Kirchhoff T, et al. Perspectives in immunotherapy: meeting report from the “Immunotherapy Bridge” (December 4th-5th, 2019, Naples, Italy). J Transl Med. 2021;19(1):13. doi:10.1186/s12967-020-02627-y.
- Hegde PS, Chen DS. Top 10 challenges in cancer immunotherapy. Immunity. 2020;52(1):17–35. doi:10.1016/j.immuni.2019.12.011.
- Tan S, Li D, Zhu X. Cancer immunotherapy: pros, cons and beyond. Biomed Pharmacother. 2020;124:109821. doi:10.1016/j.biopha.2020.109821.
- Sambi M, Bagheri L, Szewczuk MR. Current challenges in cancer immunotherapy: multimodal approaches to improve efficacy and patient response rates. J Oncol. 2019;2019:4508794. doi:10.1155/2019/4508794.
- Carretero-González A, Otero I, Lora D, Carril-Ajuria L, Castellano D, de Velasco G. Efficacy and safety of anti-PD-1/PD-L1 combinations versus standard of care in cancer: a systematic review and meta-analysis. Oncoimmunology. 2021;10(1):1878599. doi:10.1080/2162402X.2021.1878599.
- Reindl LM, Albinger N, Bexte T, Müller S, Hartmann J, Ullrich E. Immunotherapy with NK cells: recent developments in gene modification open up new avenues. Oncoimmunology. 2020;9(1):1777651. doi:10.1080/2162402X.2020.1777651.
- Naseri M, Bozorgmehr M, Zöller M, Ranaei Pirmardan E, Madjd Z. Tumor-derived exosomes: the next generation of promising cell-free vaccines in cancer immunotherapy. Oncoimmunology. 2020;9(1):1779991. doi:10.1080/2162402X.2020.1779991.
- Alcantara M, Du Rusquec P, Romano E. Current clinical evidence and potential solutions to increase benefit of CAR T-cell therapy for patients with solid tumors. Oncoimmunology. 2020;9(1):1777064. doi:10.1080/2162402X.2020.1777064.
- Tussiwand R, Onai N, Mazzucchelli L, Manz MG. Inhibition of natural type I IFN-producing and dendritic cell development by a small molecule receptor tyrosine kinase inhibitor with Flt3 affinity. J Immunol. 2005;175(6):3674–3680. doi:10.4049/jimmunol.175.6.3674.
- Petroni G, Buqué A, Zitvogel L, Kroemer G, Galluzzi L. Immunomodulation by targeted anticancer agents. Cancer Cell. 2021;39(3):310–345. doi:10.1016/j.ccell.2020.11.009.
- Comoglio PM, Trusolino L, Boccaccio C. Known and novel roles of the MET oncogene in cancer: a coherent approach to targeted therapy. Nat Rev Cancer. 2018;18(6):341–358. doi:10.1038/s41568-018-0002-y.
- Fang Y, Wang Y, Zeng D, Zhi S, Shu T, Huang N, Zheng S, Wu J, Liu Y, Huang G, et al. Comprehensive analyses reveal TKI-induced remodeling of the tumor immune microenvironment in EGFR/ALK-positive non-small-cell lung cancer. Oncoimmunology. 2021;10(1):1951019. doi:10.1080/2162402X.2021.1951019.
- Kono M, Komatsuda H, Yamaki H, Kumai T, Hayashi R, Wakisaka R, Nagato T, Ohkuri T, Kosaka A, Ohara K, et al. Immunomodulation via FGFR inhibition augments FGFR1 targeting T-cell based antitumor immunotherapy for head and neck squamous cell carcinoma. Oncoimmunology. 2022;11(1):2021619. doi:10.1080/2162402X.2021.2021619.
- Kumagai S, Koyama S, Nishikawa H. Antitumour immunity regulated by aberrant ERBB family signalling. Nat Rev Cancer. 2021;21(3):181–197. doi:10.1038/s41568-020-00322-0.
- Borg C, Terme M, Taïeb J, Ménard C, Flament C, Robert C, Maruyama K, Wakasugi H, Angevin E, Thielemans K, et al. Novel mode of action of c-kit tyrosine kinase inhibitors leading to NK cell-dependent antitumor effects. J Clin Invest. 2004;114(3):379–388. doi:10.1172/JCI21102.
- Zitvogel L, Kroemer G. Anticancer effects of imatinib via immunostimulation. Nat Med. 2011;17(9):1050–1051. doi:10.1038/nm.2429.
- Rusakiewicz S, Semeraro M, Sarabi M, Desbois M, Locher C, Mendez R, Vimond N, Concha A, Garrido F, Isambert N, et al. Immune infiltrates are prognostic factors in localized gastrointestinal stromal tumors. Cancer Res. 2013;73(12):3499–3510. doi:10.1158/0008-5472.CAN-13-0371.
- Zitvogel L, Rusakiewicz S, Routy B, Ayyoub M, Kroemer G. Immunological off-target effects of imatinib. Nat Rev Clin Oncol. 2016;13(7):431–446. doi:10.1038/nrclinonc.2016.41.
- Lin Q, Tao P, Wang J, Ma L, Jiang Q, Li J, Zhang G, Liu J, Zhang Y, Hou Y, et al. Tumor-associated tertiary lymphoid structure predicts postoperative outcomes in patients with primary gastrointestinal stromal tumors. Oncoimmunology. 2020;9(1):1747339. doi:10.1080/2162402X.2020.1747339.
- Balachandran VP, Cavnar MJ, Zeng S, Bamboat ZM, Ocuin LM, Obaid H, Sorenson EC, Popow R, Ariyan C, Rossi F, et al. Imatinib potentiates antitumor T cell responses in gastrointestinal stromal tumor through the inhibition of Ido. Nat Med. 2011;17(9):1094–1100. doi:10.1038/nm.2438.
- Seifert AM, Zeng S, Zhang JQ, Kim TS, Cohen NA, Beckman MJ, Medina BD, Maltbaek JH, Loo JK, Crawley MH, et al. PD-1/PD-L1 blockade enhances T-cell activity and antitumor efficacy of imatinib in gastrointestinal stromal tumors. Clin Cancer Res. 2017;23(2):454–465. doi:10.1158/1078-0432.CCR-16-1163.
- Schroeder BA, Kohli K, O’Malley RB, Kim TS, Jones RL, Pierce RH, Pollack SM. Durable tumor regression in highly refractory metastatic KIT/PDGFRA wild-type GIST following treatment with nivolumab. Oncoimmunology. 2020;9(1):1710064. doi:10.1080/2162402X.2019.1710064.
- Pantaleo MA, Tarantino G, Agostinelli C, Urbini M, Nannini M, Saponara M, Castelli C, Stacchiotti S, Fumagalli E, Gatto L, et al. Immune microenvironment profiling of gastrointestinal stromal tumors (GIST) shows gene expression patterns associated to immune checkpoint inhibitors response. Oncoimmunology. 2019;8(9):e1617588. doi:10.1080/2162402X.2019.1617588.
- Sugiyama E, Togashi Y, Takeuchi Y, Shinya S, Tada Y, Kataoka K, Tane K, Sato E, Ishii G, Goto K, et al. Blockade of EGFR improves responsiveness to PD-1 blockade in EGFR-mutated non-small cell lung cancer. Sci Immunol. 2020;5(43). doi:10.1126/sciimmunol.aav3937
- Lin A, Wei T, Meng H, Luo P, Zhang J. Role of the dynamic tumor microenvironment in controversies regarding immune checkpoint inhibitors for the treatment of non-small cell lung cancer (NSCLC) with EGFR mutations. Mol Cancer. 2019;18(1):139. doi:10.1186/s12943-019-1062-7.
- Tu MM, Lee FYF, Jones RT, Kimball AK, Saravia E, Graziano RF, Coleman B, Menard K, Yan J, Michaud E, et al. Targeting DDR2 enhances tumor response to anti-PD-1 immunotherapy. Sci Adv. 2019;5(2):eaav2437. doi:10.1126/sciadv.aav2437.
- Guo R, Luo J, Chang J, Rekhtman N, Arcila M, Drilon A. MET-dependent solid tumours - molecular diagnosis and targeted therapy. Nat Rev Clin Oncol. 2020;17(9):569–587. doi:10.1038/s41571-020-0377-z.
- Drilon A, Jenkins C, Iyer S, Schoenfeld A, Keddy C, Davare MA. ROS1-dependent cancers - biology, diagnostics and therapeutics. Nat Rev Clin Oncol. 2021;18(1):35–55. doi:10.1038/s41571-020-0408-9.
- Galluzzi L, Vitale I, Warren S, Adjemian S, Agostinis P, Martinez AB, Chan TA, Coukos G, Demaria S, Deutsch E, et al. Consensus guidelines for the definition, detection and interpretation of immunogenic cell death. J Immunother Cancer. 2020;8(1):e000337. doi:10.1136/jitc-2019-000337.
- Petrazzuolo A, Perez-Lanzon M, Martins I, Liu P, Kepp O, Minard-Colin V, Maiuri MC, Kroemer G. Pharmacological inhibitors of anaplastic lymphoma kinase (ALK) induce immunogenic cell death through on-target effects. Cell Death Dis. 2021;12(8):713. doi:10.1038/s41419-021-03997-x.
- Petrazzuolo A, Perez-Lanzon M, Liu P, Maiuri MC, Kroemer G. Crizotinib and ceritinib trigger immunogenic cell death via on-target effects. Oncoimmunology. 2021;10(1):1973197. doi:10.1080/2162402X.2021.1973197.
- Liu Z, Zhao K, Wei S, Liu C, Zhou J, Gou Q, Wu X, Yang Z, Yang Y, Peng Y, et al. ROS1-fusion protein induces PD-L1 expression via MEK-ERK activation in non-small cell lung cancer. Oncoimmunology. 2020;9(1):1758003. doi:10.1080/2162402X.2020.1758003.
- Liu P, Zhao L, Pol J, Levesque S, Petrazzuolo A, Pfirschke C, Engblom C, Rickelt S, Yamazaki T, Iribarren K, et al. Crizotinib-induced immunogenic cell death in non-small cell lung cancer. Nat Commun. 2019. doi:10.1038/s41467-019-09415-3.
- Chen H, Bian A, Yang L-F, Yin X, Wang J, Ti C, Miao Y, Peng S, Xu S, Liu M, et al. Targeting STAT3 by a small molecule suppresses pancreatic cancer progression. Oncogene. 2021;40(8):1440–1457. doi:10.1038/s41388-020-01626-z.
- De Martino M, Tkach M, Bruni S, Rocha D, Mercogliano MF, Cenciarini ME, Chervo MF, Proietti CJ, Dingli F, Loew D, et al. Blockade of Stat3 oncogene addiction induces cellular senescence and reveals a cell-nonautonomous activity suitable for cancer immunotherapy. Oncoimmunology. 2020;9(1):1715767. doi:10.1080/2162402X.2020.1715767.
- Lu C, Talukder A, Savage NM, Singh N, Liu K. JAK-STAT-mediated chronic inflammation impairs cytotoxic T lymphocyte activation to decrease anti-PD-1 immunotherapy efficacy in pancreatic cancer. Oncoimmunology. 2017;6(3):e1291106. doi:10.1080/2162402X.2017.1291106.
- Barone M, Catani L, Ricci F, Romano M, Forte D, Auteri G, Bartoletti D, Ottaviani E, Tazzari PL, Vianelli N, et al. The role of circulating monocytes and JAK inhibition in the infectious-driven inflammatory response of myelofibrosis. Oncoimmunology. 2020;9(1):1782575. doi:10.1080/2162402X.2020.1782575.
- Patel MR, Dash A, Jacobson BA, Ji Y, Baumann D, Ismail K, Kratzke RA. JAK/STAT inhibition with ruxolitinib enhances oncolytic virotherapy in non-small cell lung cancer models. Cancer Gene Ther. 2019;26(11–12):411–418. doi:10.1038/s41417-018-0074-6.
- Sprooten J, Agostinis P, Garg AD. Type I interferons and dendritic cells in cancer immunotherapy. Int Rev Cell Mol Biol. 2019;348:217–262. doi:10.1016/bs.ircmb.2019.06.001.
- Baluk P, Hashizume H, McDonald DM. Cellular abnormalities of blood vessels as targets in cancer. Curr Opin Genet Dev. 2005;15(1):102–111. doi:10.1016/j.gde.2004.12.005.
- Georganaki M, Ramachandran M, Tuit S, Núñez NG, Karampatzakis A, Fotaki G, van Hooren L, Huang H, Lugano R, Ulas T, et al. Tumor endothelial cell up-regulation of IDO1 is an immunosuppressive feed-back mechanism that reduces the response to CD40-stimulating immunotherapy. Oncoimmunology. 2020;9(1):1730538. doi:10.1080/2162402X.2020.1730538.
- Motz GT, Santoro SP, Wang L-P, Garrabrant T, Lastra RR, Hagemann IS, Lal P, Feldman MD, Benencia F, Coukos G. Tumor endothelium FasL establishes a selective immune barrier promoting tolerance in tumors. Nat Med. 2014;20(6):607–615. doi:10.1038/nm.3541.
- Huang Y, Kim BYS, Chan CK, Hahn SM, Weissman IL, Jiang W. Improving immune-vascular crosstalk for cancer immunotherapy. Nat Rev Immunol. 2018;18(3):195–203. doi:10.1038/nri.2017.145.
- Ziani L, Buart S, Chouaib S, Thiery J. Hypoxia increases melanoma-associated fibroblasts immunosuppressive potential and inhibitory effect on T cell-mediated cytotoxicity. Oncoimmunology. 2021;10(1):1950953. doi:10.1080/2162402X.2021.1950953.
- Hassan Venkatesh G, Abou Khouzam R, Shaaban Moustafa Elsayed W, Ahmed Zeinelabdin N, Terry S, Chouaib S. Tumor hypoxia: an important regulator of tumor progression or a potential modulator of tumor immunogenicity? Oncoimmunology. 2021;10(1):1974233. doi:10.1080/2162402X.2021.1974233.
- Guerriero JL. Macrophages: their untold story in T cell activation and function. Int Rev Cell Mol Biol. 2019;342:73–93. doi:10.1016/bs.ircmb.2018.07.001.
- Kalathil SG, Wang K, Hutson A, Iyer R, Thanavala Y. Tivozanib mediated inhibition of c-Kit/SCF signaling on Tregs and MDSCs and reversal of tumor induced immune suppression correlates with survival of HCC patients. Oncoimmunology. 2020;9(1):1824863. doi:10.1080/2162402X.2020.1824863.
- Janji B, Chouaib S. “Suffocating” tumors by blocking adaptation to hypoxia: a new headway in melanoma immunotherapy. Oncoimmunology. 2021;10(1):1968611. doi:10.1080/2162402X.2021.1968611.
- Farsaci B, Donahue RN, Coplin MA, Grenga I, Lepone LM, Molinolo AA, Hodge JW. Immune consequences of decreasing tumor vasculature with antiangiogenic tyrosine kinase inhibitors in combination with therapeutic vaccines. Cancer Immunol Res. 2014;2(11):1090–1102. doi:10.1158/2326-6066.CIR-14-0076.
- Sunay MME, Foote JB, Leatherman JM, Edwards JP, Armstrong TD, Nirschl CJ, Hicks J, Emens LA. Sorafenib combined with HER-2 targeted vaccination can promote effective T cell immunity in vivo. Int Immunopharmacol. 2017;46:112–123. doi:10.1016/j.intimp.2017.02.028.
- Martinek J, Wu T-C, Cadena D, Banchereau J, Palucka K. Interplay between dendritic cells and cancer cells. Int Rev Cell Mol Biol. 2019;348:179–215. doi:10.1016/bs.ircmb.2019.07.008.
- Läubli H, Müller P, D’Amico L, Buchi M, Kashyap AS, Zippelius A. The multi-receptor inhibitor axitinib reverses tumor-induced immunosuppression and potentiates treatment with immune-modulatory antibodies in preclinical murine models. Cancer Immunol Immunother. 2018;67(5):815–824. doi:10.1007/s00262-018-2136-x.
- Parmar S, Patel K, Pinilla-Ibarz J. Ibrutinib (imbruvica): a novel targeted therapy for chronic lymphocytic leukemia. P T. 2014;39:483–519.
- Davids MS, Brown JR. Ibrutinib: a first in class covalent inhibitor of Bruton’s tyrosine kinase. Future Oncol. 2014;10(6):957–967. doi:10.2217/fon.14.51.
- Barf T, Covey T, Izumi R, van de Kar B, Gulrajani M, van Lith B, van Hoek M, de Zwart E, Mittag D, Demont D, et al. Acalabrutinib (ACP-196): a covalent Bruton tyrosine kinase inhibitor with a differentiated selectivity and in vivo potency profile. J Pharmacol Exp Ther. 2017;363(2):240–252. doi:10.1124/jpet.117.242909.
- Kriegsmann K, Kriegsmann M, Witzens-Harig M. Acalabrutinib A second-generation Bruton’s tyrosine kinase inhibitor. Recent results in cancer research. Fortschritte der Krebsforschung. 2018;212:285–294. doi:10.1007/978-3-319-91439-8_14.
- Wu J, Zhang M, Liu D. Acalabrutinib (ACP-196): a selective second-generation BTK inhibitor. J Hematol Oncol. 2016;9(1):21. doi:10.1186/s13045-016-0250-9.
- Weaver AN, Jimeno A. Zanubrutinib: a new BTK inhibitor for treatment of relapsed/refractory mantle cell lymphoma. Drugs Today. 2020;56(8):531–539. doi:10.1358/dot.2020.56.8.3158047.
- Geethakumari PR, Awan F. An evaluation of zanubrutinib, a BTK inhibitor, for the treatment of chronic lymphocytic leukemia. Expert Rev Hematol. 2020;13(10):1039–1046. doi:10.1080/17474086.2020.1817735.
- Burger JA, Wiestner A. Targeting B cell receptor signalling in cancer: preclinical and clinical advances. Nat Rev Cancer. 2018;18(3):148–167. doi:10.1038/nrc.2017.121.
- Benner B, Scarberry L, Stiff A, Duggan MC, Good L, Lapurga G, Butchar JP, Tridandapani S, Carson WE. Evidence for interaction of the NLRP3 inflammasome and Bruton’s tyrosine kinase in tumor-associated macrophages: implications for myeloid cell production of interleukin-1beta. Oncoimmunology. 2019;8(11):1659704. doi:10.1080/2162402X.2019.1659704.
- Natarajan G, Oghumu S, Terrazas C, Varikuti S, Byrd JC, Satoskar AR. A tec kinase BTK inhibitor ibrutinib promotes maturation and activation of dendritic cells. Oncoimmunology. 2016;5(6):e1151592. doi:10.1080/2162402X.2016.1151592.
- Dubovsky JA, Beckwith KA, Natarajan G, Woyach JA, Jaglowski S, Zhong Y, Hessler JD, Liu T-M, Chang BY, Larkin KM, et al. Ibrutinib is an irreversible molecular inhibitor of ITK driving a Th1-selective pressure in T lymphocytes. Blood. 2013;122(15):2539–2549. doi:10.1182/blood-2013-06-507947.
- Long M, Beckwith K, Do P, Mundy BL, Gordon A, Lehman AM, Maddocks KJ, Cheney C, Jones JA, Flynn JM, et al. Ibrutinib treatment improves T cell number and function in CLL patients. J Clin Invest. 2017;127(8):3052–3064. doi:10.1172/JCI89756.
- Kondo K, Shaim H, Thompson PA, Burger JA, Keating M, Estrov Z, Harris D, Kim E, Ferrajoli A, Daher M, et al. Ibrutinib modulates the immunosuppressive CLL microenvironment through STAT3-mediated suppression of regulatory B-cell function and inhibition of the PD-1/PD-L1 pathway. Leukemia. 2018;32(4):960–970. doi:10.1038/leu.2017.304.
- Berg LJ, Finkelstein LD, Lucas JA, Schwartzberg PL. Tec family kinases in T lymphocyte development and function. Annu Rev Immunol. 2005;23(1):549–600. doi:10.1146/annurev.immunol.22.012703.104743.
- Xia S, Liu X, Cao X, Xu S. T-cell expression of Bruton’s tyrosine kinase promotes autoreactive T-cell activation and exacerbates aplastic anemia. Cell Mol Immunol. 2020;17(10):1042–1052. doi:10.1038/s41423-019-0270-9.
- Fraietta JA, Beckwith KA, Patel PR, Ruella M, Zheng Z, Barrett DM, Lacey SF, Melenhorst JJ, McGettigan SE, Cook DR, et al. Ibrutinib enhances chimeric antigen receptor T-cell engraftment and efficacy in leukemia. Blood. 2016;127(9):1117–1127. doi:10.1182/blood-2015-11-679134.
- Ruella M, Kenderian SS, Shestova O, Fraietta JA, Qayyum S, Zhang Q, Maus MV, Liu X, Nunez-Cruz S, Klichinsky M, et al. The addition of the BTK inhibitor ibrutinib to anti-CD19 chimeric antigen receptor T cells (CART19) improves responses against mantle cell lymphoma. Clin Cancer Res. 2016;22(11):2684–2696. doi:10.1158/1078-0432.CCR-15-1527.
- Qin JS, Johnstone TG, Baturevych A, Hause RJ, Ragan SP, Clouser CR, Jones JC, Ponce R, Krejsa CM, Salmon RA, et al. Antitumor potency of an anti-CD19 chimeric antigen receptor T-cell therapy, lisocabtagene maraleucel in combination with ibrutinib or acalabrutinib. J Immunother. 2020;43(4):107–120. doi:10.1097/CJI.0000000000000307.
- Zhao P, Zhen H, Zhao H, Zhao L, Cao B. Efficacy and safety of adjuvant EGFR-TKIs for resected non-small cell lung cancer: a systematic review and meta-analysis based on randomized control trials. BMC Cancer. 2022;22(1):328. doi:10.1186/s12885-022-09444-0.
- Derosa L, Routy B, Fidelle M, Iebba V, Alla L, Pasolli E, Segata N, Desnoyer A, Pietrantonio F, Ferrere G, et al. Gut bacteria composition drives primary resistance to cancer immunotherapy in renal cell carcinoma patients. Eur Urol. 2020;78(2):195–206. doi:10.1016/j.eururo.2020.04.044.
- Routy B, le Chatelier E, Derosa L, Duong CPM, Alou MT, Daillere R, Fluckiger A, Messaoudene M, Rauber C, Roberti MP, et al. Gut microbiome influences efficacy of PD-1-based immunotherapy against epithelial tumors. Science. 2018;359(6371):91–97. doi:10.1126/science.aan3706.
- Daillere R, Vetizou M, Waldschmitt N, Yamazaki T, Isnard C, Poirier-Colame V, Duong CPM, Flament C, Lepage P, Roberti MP, et al. Enterococcus hirae and Barnesiella intestinihominis facilitate cyclophosphamide-induced therapeutic immunomodulatory effects. Immunity. 2016;45(4):931–943. doi:10.1016/j.immuni.2016.09.009.
- Foà R, Bassan R, Vitale A, Elia L, Piciocchi A, Puzzolo M-C, Canichella M, Viero P, Ferrara F, Lunghi M, et al. Dasatinib-blinatumomab for ph-positive acute lymphoblastic leukemia in adults. N Engl J Med. 2020;383(17):1613–1623. doi:10.1056/NEJMoa2016272.
- Montemagno C, Hagege A, Borchiellini D, Thamphya B, Rastoin O, Ambrosetti D, Iovanna J, Rioux-Leclercq N, Porta C, Negrier S, et al. Soluble forms of PD-L1 and PD-1 as prognostic and predictive markers of sunitinib efficacy in patients with metastatic clear cell renal cell carcinoma. Oncoimmunology. 2020;9(1):1846901. doi:10.1080/2162402X.2020.1846901.
- Taylor MH, Schmidt EV, Dutcus C, Pinheiro EM, Funahashi Y, Lubiniecki G, Rasco D. The LEAP program: lenvatinib plus pembrolizumab for the treatment of advanced solid tumors. Future Oncol. 2021;17(6):637–648. doi:10.2217/fon-2020-0937.
- Choueiri TK, Powles T, Burotto M, Escudier B, Bourlon MT, Zurawski B, Oyervides Juárez VM, Hsieh JJ, Basso U, Shah AY, et al. Nivolumab plus cabozantinib versus sunitinib for advanced renal-cell carcinoma. N Engl J Med. 2021;384(9):829–841. doi:10.1056/NEJMoa2026982.
- Geyer MB, Rivière I, Sénéchal B, Wang X, Wang Y, Purdon TJ, Hsu M, Devlin SM, Palomba ML, Halton E, et al. Safety and tolerability of conditioning chemotherapy followed by CD19-targeted CAR T cells for relapsed/refractory CLL. JCI Insight. 2019;5(9). doi:10.1172/jci.insight.122627
- Petroni G, Galluzzi L. Impact of treatment schedule on the efficacy of cytostatic and immunostimulatory agents. Oncoimmunology. 2021;10(1):1889101. doi:10.1080/2162402X.2021.1889101.