ABSTRACT
Dendritic cell (DC)-based vaccination for cancer treatment has seen considerable development over recent decades. However, this field is currently in a state of flux toward niche-applications, owing to recent paradigm-shifts in immuno-oncology mobilized by T cell-targeting immunotherapies. DC vaccines are typically generated using autologous (patient-derived) DCs exposed to tumor-associated or -specific antigens (TAAs or TSAs), in the presence of immunostimulatory molecules to induce DC maturation, followed by reinfusion into patients. Accordingly, DC vaccines can induce TAA/TSA-specific CD8+/CD4+ T cell responses. Yet, DC vaccination still shows suboptimal anti-tumor efficacy in the clinic. Extensive efforts are ongoing to improve the immunogenicity and efficacy of DC vaccines, often by employing combinatorial chemo-immunotherapy regimens. In this Trial Watch, we summarize the recent preclinical and clinical developments in this field and discuss the ongoing trends and future perspectives of DC-based immunotherapy for oncological indications.
Introduction
The identification of dendritic cells (DCs) for the first time in 1973 by Ralph Steinman, who was eventually awarded a Nobel Prize for this ground-breaking discovery in 2011, laid the foundation for the field of DC immunology in health and disease.Citation1,Citation2 Since then, extensive research has been done to understand the functionality and ontology, as well as interface with CD4+/CD8+ T cells and, in recent decades, to understand how to harness their immunological potential for anti-cancer immunotherapy.
DCs are professional antigen-presenting cells (APCs), largely regarded as a crucial link between innate and adaptive immune compartments.Citation3–6 DCs specialize in antigen presentation. They are not only able to present extracellular antigens on major histocompatibility complex (MHC) class II molecules to CD4+ T helper (TH) cells but also to present extracellular antigens on MHC class I molecules to CD8+ T cells.Citation7 This phenomenon is known as cross-presentation and is crucial for efficacious anti-tumor immune responses.Citation7
DCs are a ubiquitous and highly heterogeneous group of myeloid cells, being present all over the body, and being involved in a variety of immunological functions. Due to this heterogeneity, DC classification into well-defined biological subsets based on their phenotype, ontogeny, and functions has been continuously refined and updated as advances in high-dimensional flow cytometry and single-cell transcriptomics technologies have together accelerated the knowledge garnered on DC subsets’ highly specialized features and functions.Citation8–11 Accordingly, the classification criteria have moved from the classical functional and location-based, toward classifying DCs based on lineage and ontogeny thereby resulting in three major subsets of DCs: two types of ‘classical’ or ‘conventional’ DCs (cDCs) i.e., type 1 cDC (cDC1) as well as type 2 cDC (cDC2), and plasmacytoid DC (pDC)Citation12–16. Whereas it has historically been believed that DCs arise from monocytes, cDCs or pDCs are now well established to be fully distinct, lineage-wise, from monocyte-derived DCs (moDCs).Citation10,Citation17,Citation18 Herein, while cDC1 and cDC2 arise from the same common DC progenitor (CDP), the ontogeny of pDCs is still in debate, with recent studies suggesting a lymphoid origin for this subset.Citation19–21
Human cDC1s can be identified using various highly specific cell surface markers, such as: cell adhesion molecule 1 (CADM1), thrombomodulin (THBD, also known as CD141), C-type lectin domain containing 9A (CLEC9A), as well as X-C motif chemokine receptor 1 (XCR1), which can also be used to identify cDC1 in mouse.Citation14,Citation17,Citation22–24 Defining the cDC2 subset is more complex due to the high heterogeneity and variability in surface marker expression both in humans and mice, leading to the subdivision of this subset into further clusters, distinguished based on CD5 expression: CD5+ cDC2 (DC2) and CD5− cDC2 (DC3).Citation12,Citation14 Of note, it remains unclear whether DC3 arise from CDPs.Citation9,Citation14 While other cDC2 subsets have been proposed, namely DC4 (CD1C−CD141−) and DC5 (AXL+SIGLEC6+),Citation12 their classification within the myeloid population and functional characterization still requires further studies.
The DC subsets also exhibit functional heterogeneity, with each subset specializing in distinct and assorted overlapping immunological functions, which also vary based on location.Citation6 cDC1 specialize in the activation of CD8+ T cells through cross-presentation and responses to intracellular pathogens.Citation25–28 cDC1s play a crucial role in anti-tumor immunity, being the only APCs that can efficiently prime tumor-specific CD8+ T cells.Citation28–31 In addition, cDC1s are major producers of IFN-λ, which has been contextually described to skew T cell responses toward a TH1 bias.Citation32,Citation33 In both mice and humans, cDC1 are indispensable for attracting CD8+ T cells into tumors such that, the abundance of cDC1 in the tumor microenvironment (TME) is positively correlated with patient survival.Citation25,Citation34 cDC2s’ main function is considered to be the presentation of exogenous antigens (e.g., from extracellular pathogens) to different CD4+ TH subsets, although this subset is as functionally heterogeneous as it is ontogenetically.Citation35,Citation36 In comparison to cDC1s, knowledge on cDC2s’ functions in anti-tumor responses is only starting to build up recently. It is emerging that cDC2s perform a non-redundant role in mobilizing both CD8+T cell responses and CD4+ TH cell priming.Citation9,Citation35,Citation37,Citation38 Moreover, unlike cDC1s, cDC2s can secrete high levels of interleukin-12 (IL-12),Citation39–41 which is crucial for T and natural killer (NK) cell expansion and survival.Citation41–43 pDCs excel at type I interferon (IFN) responses, mounting stronger type I IFN responses than any other DC subsets, and being the major producers of IFN-α.Citation44–47 Yet, high frequencies of pDCs in the tumor associate with poor prognosis in multiple human cancer types, likely due to frequent impairment of type I IFN production in the TME, leading to immunosuppression.Citation9,Citation48–54
In the absence of pathophysiological stimuli, both tissue-resident and circulating DCs exist in an immature or steady-state and are essential for immunosurveillance.Citation55–57 Immature DCs (iDCs) are essential to maintain tolerance to self-antigens in the periphery, having the ability to suppress or even facilitate clonal deletion of self-reactive T cells, and to promote the expansion of CD4+CD25+FOXP3+ regulatory T cells (TREG).Citation55,Citation58–62 In this state, these iDCs exhibit (1) proficient uptake of extracellular material, coupled with secretion of homeostatic cytokines and chemokines, (2) retention of MHC class II molecules in late endosomes, (3) expression of various chemokine receptors, which enable rapid chemotaxis of iDCs to inflammation sites, and (4) minimal to negligible expression of co-stimulatory molecules, such as CD80, CD83, CD86, CD70, CD40, and tumor necrosis factor (ligand) superfamily member 4 (TNFSF4, also known as OX40L).Citation56,Citation63
When iDCs encounter (potentially antigenic) inflammation accompanied by microbial stimuli – e.g., pathogen-associated molecular patterns (PAMPs)Citation64–67 – or endogenous stimuli – e.g., damage-associated molecular patterns (DAMPs)Citation68–73 – they enter a complex developmental program known as maturation.Citation74,Citation75 Mature DCs (mDCs) are phenotypically and functionally distinct from iDCs in various aspects, of which the most significant are as follows: (1) decreased phagocytic activity, (2) increased expression of co-stimulatory ligands and increased exposure of MHC molecules (both class I and II) on the cell surface, (3) altered expression of chemokine receptors, in particular chemokine (C-C motif) receptor 7 (CCR7), which is involved in homing to, and retention in the lymph nodes, and (4) abundant secretion of pro-inflammatory cytokines such as IL-12, IL-6, IL-1β, and chemokines such as C-X-C motif chemokine ligand 9, 10, and 11 (CXCL10, CXCL10, and CXCL11).Citation76–85 These modifications enable mDCs to efficiently co-stimulate and prime T cells in the lymph nodes.
DCs play a key role in anti-tumor immunity by properly priming CD8+ T cells against tumor-associated or specific antigens (TAAs or TSAs), a process which is often impaired by immunosuppressive TME.Citation5,Citation7,Citation9,Citation86–90 DCs’ potent antigen presentation and T cell activation ability have made them an important player in anticancer vaccination strategies.Citation91–95 Classically, DC vaccines in clinical studies are created by ex vivo differentiation of DCs from autologous monocytes obtained from patients with apheresis, followed by exposure to TAAs/TSAs, in the presence of maturation-inducing agents or cocktails, and finally are reinfused back into the same patient.Citation92,Citation96–99 Although this approach has shown some success in inducing TAA/TSA-specific immune responses in patients,Citation91–94 the therapeutic efficacy of DC-based vaccines in clinical studies is still limited due to the robust immunosuppressive mechanisms within the TME.Citation92,Citation100–102 Additionally, while the use of moDCs for the creation of DC vaccines is highly prevalent, due to various practical reasons, mounting evidence suggests that the use of cDCs might be worth exploring, as these subsets might be superior to moDCs in their T cell stimulatory capability.Citation94,Citation96,Citation103,Citation104 The possibility of using naturally occurring DC subsets – such as cDCs, Langerhans cells (LCs) and pDCs – as DC vaccines is being explored in clinical settings with promising early results, although protocols for the isolation or in vitro differentiation of these cells still need to be optimized.Citation105–111 Nevertheless, future clinical testing of cDC/pDC-based anticancer vaccines will answer a crucial question, i.e., is the historically low efficacy of moDC-based vaccines due to their supposedly inferior T cell-stimulatory capacity (largely suggested based on murine rather than human data), or due to other as-yet-unknown DC vaccines-specific resistance pathways.
In recent decades, the field of therapeutic DC vaccination has seen vast progress, with various strategies being explored in both preclinical and clinical settings. Broadly, DC vaccines can be classified into different groups based on the TAA/TSA delivery approach or the manipulation imposed on DCs prior to administration.Citation92,Citation112 These include (1) DCs not pulsed with TAA/TSAs, either unstimulated or stimulated with different agents (e.g., immunostimulatory cytokines or PAMP/DAMPs such as toll-like receptor (TLR) agonists);Citation41,Citation113–118 (2) DCs pulsed with TAA/TSAs ex vivo, which can be from different sources: tumor lysates, whole tumor mRNA (both of which provide a wide array of TAA/TSAs), specific TAA/TSA-based peptides or TAA/TSA-coding mRNAs (generally consisting of one or a limited amount of TAA/TSAs)Citation119–136 and (3) DCs transfected with viral or biochemical genetic vectors encoding TAA/TSAs or immunostimulatory factors.Citation136–138 Alternative DC-based therapy strategies include delivery of TAA/TSAs to DCs in vivoCitation139–144 and intra-tumoral administration of immunomodulatory molecules to stimulate DCs in situ;Citation145 as well as exosomes derived from DCs.Citation146–148
As observed within our previous Trial Watch,Citation149 the most common therapeutic approach to DC vaccines remains the pulsing of DCs with TAAs (as peptides or tumor lysate) stimulated with maturation-inducing cocktails. This can be achieved ex vivo by various methods, including but not limited to (1) co-incubation of DCs with tumor lysate,Citation119,Citation121,Citation123,Citation124,Citation150,Citation151 (2) co-incubation of DCs with recombinant TAAs or TAA-derived peptides,Citation126–128 (3) transfection of DCs with TAA-encoding plasmids or mRNA,Citation132,Citation133,Citation152–154 or (4) fusion of DCs with tumor cells.Citation155–159 Alternatively, DCs can be appropriated for therapeutic use by in vivo or in situ stimulation, through methods such as direct TAA delivery via immunoliposomes targeting DCs, genetic vectors targeting DCs, fusions of TAAs with monoclonal antibodies or other molecules (e.g., carbohydrates and polypeptides) targeting DC-specific receptors (e.g., mannose receptor, C type 1 (MRC1), CD209 (also known as DC-SIGN), and lymphocyte antigen 75 (LY75, also known as DEC-205)).Citation139–144,Citation160–162 Despite the significant amount of preclinical and clinical progress in the field, to date sipuleucel-T (sold commercially as Provenge®) remains the only tumor-targeting DC-based therapy being approved by the US Food and Drug Administration (FDA) in 2010, for the treatment of asymptomatic or minimally symptomatic metastatic castrate resistant (i.e. hormone refractory) prostate cancer.Citation163,Citation164 The marketing authorization for sipuleucel-T has since been withdrawn from the European Union. However, it is worth mentioning that sipuleucel-T was not a pure DC-based preparation but rather a mixture of various immune cells (peripheral-blood mononuclear cells), which could have been one of the reasons behind its disappointing performance.
In this Trial Watch, we review the latest preclinical and clinical progress in the development of DC vaccines for oncological indications. Immune checkpoint blockers (ICBs) and adoptive T-cell transfer (ACT) remain at the forefront of the cancer immunotherapy field,Citation165–168 yet the number of ongoing clinical studies investigating the safety and efficacy of DC vaccination for cancer treatment remains high, many of which are now focusing on multimodal therapeutic regimens combining these different approaches.
Recent preclinical developments
Since the publication of our last Trial Watch on DC vaccination for oncological treatment (February 2019),Citation149 there has been an abundance of preclinical research published on this topic, most making use of murine models of cancer. Within this, we have selected several publications of particular interest to represent the larger trends within the field (ordered in a random manner).
Zhou et al. (University of Texas MD Anderson Cancer Center, Houston, TX, USA) found that CD103+ cDC1-based vaccines were relatively superior to moDC-based vaccines in eliciting artificial OVA antigen-directed T cell responses, and resulted in complete regression of all osteosarcoma murine tumors after cytotoxic T-lymphocyte associated protein 4 (CTLA4) checkpoint blockade.Citation169 Zhu et al. (The First Hospital of Jilin University, Changchun, China) found that while a DC vaccine comprised of glioma lysate and CpG oligodeoxynucleotides (CpG) increased survival and tumor regression, it also upregulated programmed cell death 1 (PD1, also known as PDCD1) and programmed cell death ligand 1 (PDL1, also known as CD274) on effector T cells, DCs and glioma tissue in mice.Citation170 Accordingly, they found that combining the DC vaccine with anti-PDL1 antibodies had a synergistic effect and decreased TREGs in the brain.Citation170 Ashour et al. (University of Würzburg, Würzburg, Germany) showed that injected moDCs can direct T cell priming and activation of endogenous DCs but not TH type 1 (TH1) polarization, which is instead mediated by IL-12 produced from lymph-node resident cDC1s.Citation171 Hodge et al. (University of South Carolina School of Medicine, Columbia, SC, USA), demonstrated that inducing overexpression of microRNA 155 (miR-155) enhanced the anti-tumor activity of DC vaccines against breast cancer, culminating in an increase in effector T cells, a suppression of tumor growth, and a decrease in lung metastasis.Citation172 Park et al. (Konkuk University, Chungju, South Korea) identified 40S ribosomal protein S3 (RSP3) as a TLR4 ligand and as a potential adjuvant for DC vaccines, inducing activation and maturation of DCs. RSP3-treated DCs facilitated tumor prevention and regression as well as increased IFN-γ producing CD8+ T cells.Citation173 Similarly, Jang et al. (Konkuk University, Chungju, South Korea) identified another TLR4 ligand, 60S acidic ribosomal protein P2 (RPLP2), which improved DC immunogenicity, and these RPLP2-treated DC vaccines synergized with PD1 and PD-L1 blockade.Citation174 Dastmalchi et al. (University of Florida, Gainesville, FL, USA) demonstrated that sarcosine (intermediate in the metabolism of choline to glycine) improved DC vaccine migration to the lymph node and spleen after intradermal administration, and improved tumor control. CXCR2 blockade removed the effect of sarcosine on DC migration and consequently abrogated the survival benefit of this DC vaccine.Citation175 Liu et al. (Jiangxi University of Technology, Nanchang, China), showed that silencing indoleamine 2,3-dioxygenase 2 (IDO2) in DCs improved their immunogenicity, leading to enhanced cytotoxic T cell activity and decreased Tregs within the tumors post-vaccination, as well as suppression of tumor growth.Citation176 Previously, Endo et al. (Hokkaido University, Sapporo, Japan) had obtained similar results for increased antitumor effect of DC vaccines by silencing of indoleamine 2,3-dioxygenase 1 (IDO1) in DCs through administration of a siRNA via a nanodevice.Citation177
In a murine leukemia model, Stroopinsky at al. (Harvard Medical School) demonstrated that a DC/acute myeloid leukemia cell (AML) fusion vaccine, which had previously shown good results in a phase I/II trial,Citation178 synergizes with ICBs (i.e., anti-PD1, anti-hepatitis A virus cellular receptor 2 (HAVCR2, best known as TIM3), and anti- repulsive guidance molecule BMP co-receptor b (RGMb)) to induce durable leukemia-specific immunity.Citation179 Shi et al. (Guangxi Medical University, Guangxi, China) reported that a PD1-specific nanobody synergizes with DC/tumor-cell fusion vaccines in multiple tumor types.Citation180 Lau et al. (Erasmus Medical Center, Rotterdam, The Netherlands) showed that mesothelioma-lysate loaded DCs slowed tumor growth prophylactically, but in established disease only improved survival when combined with an agonistic CD40 antibody, which also induced lower expression of immune-inhibitory receptors on CD8+ T cells.Citation181 Oba et al. (Roswell Park Comprehensive Cancer Center, Buffalo, NY, USA) showed the immunotherapy potential of induced pluripotent stem cells-derived DCs and combined them with radiotherapy to overcome anti-PDL1 therapy resistance in non-immunogenic tumors.Citation182 Lapenta et al. (Istituto Superiore di Sanità, Rome, Italy) found that combining an IFNα-stimulated DC vaccine with lenalidomide leads to improved therapeutic effect in lymphoma-bearing mice.Citation183 El-Ashmawy et al. (Tanta University, Tanta, Egypt) used DC vaccination to target cancer stem cells and found that the DC vaccine loaded with tumor lysate significantly reduced the percentage of CD166+cancer stem cells when compared to cisplatin and reversed the tumorigenic effect of Benzo(a)Pyrene in the mouse lung.Citation184 Gou et al. (University of Colorado, Anschutz Medical Campus, Aurora, Colorado) screened kinase inhibitors and found that MK2206, DNA-dependent protein kinase inhibitor NU7441 and mitogen-activated protein kinase kinase enzyme (MEK) inhibitor trametinib showed the best ability to improve DC immunogenicity. This combination also improved the anti-tumor activity of a glioblastoma (GBM) DC vaccine called IC107.Citation185
The above selected studies represent only a fraction of an array of such preclinical studies on DC vaccination in the published literature. This indicates significant interest in optimizing and enabling anticancer DC vaccines.
Completed clinical studies
We identified 25 peer-reviewed research papers or clinical studies published since the release of our previous Trial Watch on this topic (February 2019),Citation149 until December 2021, that reported the safety and efficacy data of clinical trials investigating therapeutic application of DC-based vaccines for treating oncological indications.
These published studies reported results of trials spanning 13 different cancer types, of which the most common were melanoma,Citation186–189 followed by GBM/glioma,Citation190–192 prostate cancer,Citation193,Citation194 ovarian cancer,Citation195,Citation196 and pancreatic cancerCitation197,Citation198 (). Relative to our previous report in 2019Citation149, while the aforementioned tumor types remain well represented, yet we do see a shift from clinical studies focusing on ‘basket trials’ consisting of multiple tumor types, toward specific trials focusing on a particular oncological indication; such that, only two studies enrolled patients with multiple solid tumors,Citation199,Citation200 while another study focused on peritoneal metastases derived from multiple primary malignancies.Citation201
Figure 1. Overview of current strategies of dendritic cell vaccination for cancer therapy. ATRA, all-trans retinoic acid; CAR, chimeric antigen receptor; CMV-pp65, cytomegalovirus 65 kDa phosphoprotein; CTL, cytotoxic T lymphocyte; CTLA4, cytotoxic T-lymphocyte associated protein 4; DC, dendritic cell; ERBB, erb-b2 receptor tyrosine kinase; EBV, Epstein-Barr virus; fLAMP, full-length lysosome-associated membrane protein; FOLR1, folate receptor alpha; GM-CSF, granulocyte-macrophage colony-stimulating factor; gp100, glycoprotein 100; HSP70, 70 kDa heat shock protein; IFN, interferon; IL, interleukin; IL13RA2, interleukin 13 receptor subunit alpha 2; MAGE, melanoma-associated antigen; MLANA, melan-A; mTOR, mammalian target of rapamycin; MUC1, mucin 1, cell surface associated; NY-ESO-1 (official name: CTAG1B), cancer/testis antigen 1B; PD1, programmed death ligand 1; PDL1, programmed cell death 1 ligand 1; TAA, tumor-associated antigen; TBVA, tumor blood vessel antigens; TERT, telomerase reverse transcriptase; TKI, tyrosine kinase inhibitor; Tp53, tumor protein p53; TRP2, tyrosinase related protein 2; WT1, WT1 transcription factor.
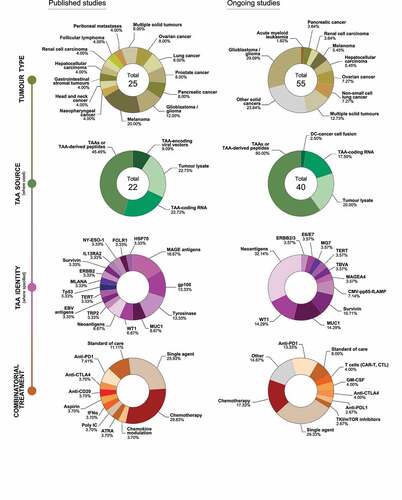
Most of these studies reported the results of clinical trials evaluating autologous DCs pulsed with TAAs or TAA-derived peptides,Citation188,Citation190,Citation191,Citation193,Citation196–199,Citation202,Citation203 TAA-coding RNAs,Citation189,Citation194,Citation204–206 and autologous cancer cell lysatesCitation187,Citation192,Citation195,Citation201,Citation207 (). This is in line with the ongoing trends in the field, as reported in our previous Trial Watch (February 2019).Citation149 Additionally, two publications reported on the safety and efficacy of TAA-coding adenovirus transfected DC vaccines.Citation186,Citation208 Finally, there were two studies reporting on autologous DCs alone (i.e. without TAAs)Citation200,Citation209 and another (Phase I) study that evaluated the safety of an allogenic DC vaccine, ilixadencel, which uses unloaded moDCs sourced from healthy donors stimulated by a cocktail of activation factors.Citation210,Citation211
Within the studies focused on specific TAAs, the most common targets were melanoma antigen family (MAGE) peptides – MAGE-A1Citation190, MAGE-A3,Citation188,Citation205 MAGE-A6,Citation186 and MAGE-C2,Citation193,Citation205 followed by glycoprotein 100 (gp100)Citation188–190,Citation205 and tyrosinaseCitation186,Citation188,Citation189,Citation205 (). These antigens are classically known as cancer-germline antigens (MAGE family) and melanocyte differentiation antigensCitation212 and, with the exception of one study targeting gp100 in GBM, and one study targeting MAGE-C2 in prostate cancer patients,Citation190,Citation193 they were used exclusively in trials involving melanoma patients. The choice of specific antigens for DC pulsing differs significantly from our previous Trial Watch;Citation149 however, the general trend remains, i.e.,, the vast majority of studies target common TAAs. Two studies deviated from this by investigating DC vaccines loaded with personalized neoantigens.Citation197,Citation199
In the above studies, DC vaccination was either assessed as a single adjuvant therapyCitation187,Citation193,Citation194,Citation196,Citation203,Citation205,Citation206,Citation210 (generally following surgery or standard-of-care), or in combination with various conventional anticancer therapies, most often chemotherapeuticsCitation189,Citation192,Citation195,Citation197–199,Citation207,Citation208 and other standard-of-care regimensCitation191,Citation202,Citation204 (). Other trials combined DC vaccination with specific immunotherapeutic agents such as ICBs (primarily, anti-PD1 or anti-CTLA4 antibodies) or immunomodulatory monoclonal antibodies (e.g., anti-CD20)Citation188,Citation197,Citation199,Citation200,Citation209 ().
It is noteworthy that most of the above publications reported results of Phase I or I/II studies (out of 25 studies, 9 were Phase I, 4 were Phase I/II and 11 were Phase II, and only 1 Phase III trial), evaluating the safety and potential adverse effects of DC vaccination regimens. Of note, in line with our previous Trial WatchCitation149 as well as available literature, DC vaccines were well tolerated, with studies reporting mostly only mild-to-moderate adverse effects (grade 1–2) such as flu-like symptoms, fatigue, and rashes in a small subset of patients. Only one Phase II study reported a significant number of severe adverse effects (36% grade 3–4); however, this is likely to be attributed to the use of ipilimumab (anti-CTLA4 ICB), as this same vaccine had been previously shown to be safe when applied as a single agent.Citation188,Citation213,Citation214
DC vaccine-induced immunogenicity in patients, as measured by increased antigen-specific T- or B-cell activity and/or lymphocyte tumor infiltration (among other parameters), was consistently observed. Of note, one study reported the results of a Phase III trial which enrolled 462 newly diagnosed renal cell carcinoma patients, and evaluated the combination of a DC vaccine pulsed with tumor RNA (Rocapuldencel-T) together with sunitinib (standard of care option at the time of the trial), compared to sunitinib alone.Citation204 The study found that Rocapuldencel-T did not improve overall survival (OS), with an OS in the combination group of 27.7 months and 32.4 months in the sunitinib group, although the magnitude of the DC vaccine-driven immune response did correlate with OS.Citation204 Most studies reported clinically relevant immune responses to DC vaccines, with two studies being worthy of note: a phase II trial which tested the efficacy and safety of a DC vaccine, ICT-107 (autologous DCs pulsed with six synthetic peptide epitopes targeting GBM TAAs), in 124 newly diagnosed GBM patients, found significant improvement in progression-free survival (PFS) in the ICT-107 group by 2.2 months, with OS also showing a (albeit non-significant) increase of 2 months;Citation190 and a phase IIb study in melanoma patients evaluating the tumor lysate, particle-loaded, DC (TLPLDC) vaccine, an autologous DC vaccine loaded with yeast cell wall particles containing tumor lysate, which showed an increased 24-month disease free survival (DFS) rate in per-treatment analysis in the TLPLDC-treated group (62.9% versus 34.8% in the control arm).Citation187
Altogether, the results of these studies convey the highly relevant clinical therapeutic potential of DC vaccination, while simultaneously indicating that there remains ample room for improvement in terms of proper patient pre-selection, cancer-type tailoring, and antigen-level personalization options.
Ongoing clinical trials
This Trial Watch covers trials ‘first posted’ in the period between February 2019 and December 2021, during which 55 clinical trials evaluating the dose, safety, and efficacy of DC-based vaccines against cancer were registered at http://www.clinicaltrials.gov/. The details of these trials are summarized in .
Table 1. Overview of clinical trials registered on clinicaltrials.gov between February 2019 and December 2021 testing dendritic cell-based immunotherapy in cancer patients.
In these ongoing clinical trials, the most common cancer type being targeted is GBM, followed by basket trials enrolling patients with various solid tumors ( and ). Cancer type-focussed studies cover a wide variety of tumor types apart from GBM, e.g., patients with ovarian cancer, non-small cell lung cancer, hepatocellular cancer, and melanoma ( and ). While most ongoing trials are in Phase I or II, there are currently at least three (active) advanced (Phase III) clinical trials testing DC vaccines for the treatment of cancer: two of these are testing TAA-loaded DC vaccines in GBM (NCT05100641 and NCT04277221), while another trial is enrolling patients with neoplasms to treat them with a combination of DCs with cytokine-induced killer cells (CIK), known as DC-CIK (NCT04292769).
Within these ongoing studies, the most common therapeutic approach to DC vaccination consists of autologous DCs pulsed with TAAs or TAA-derived peptides, followed by tumor lysate and TAA-coding RNA ( and ). Several recent studies are focusing on TAA or TAA-derived peptide mixtures as well as personalized (or pre-defined) neoantigens (). The trials targeting common TAAs show a variety of TAAs as target, contingent on the tumor type, with the most common being WT1 transcription factor (WT1), mucin 1 cell surface associated (MUC1), survivin, and the human cytomegalovirus protein 65 kDa phosphoprotein (pp65) ( and ).
To improve immunogenicity and efficacy, DC vaccination is being combined in preclinical and clinical studies with other cancer therapies such as chemo- and radiotherapy, as well as with other immunotherapeutic strategies such as adoptive cell transferCitation215 including transfer of chimeric antigen receptor (CAR) T cells,Citation216–222 CIK cellsCitation223,Citation224 and NK cells,Citation43,Citation225–227 antibody-based therapy (e.g., ICB or immunostimulatory antibodies),Citation228,Citation229 recombinant cytokines,Citation230,Citation231 or targeted therapies (e.g., tyrosine kinase inhibitors).Citation232–236 While a significant portion of ongoing trials are investigating DC vaccination as a single (adjuvant) agent (generally after completion of standard of care regimens), various other anticancer therapies are also being tested in combination with the goal of improving the efficacy of these DC vaccines ( and ). Of these, a combination of ICB stands out, with PD1 being the most common immune-checkpoint being targeted therein, followed by CTLA4 and PDL1 ( and ). The majority of these used only one ICB (alone or in combination with other cancer therapies such as chemotherapy), with only two trials combining DC vaccines with two or more ICBs (). Other common combinations within ongoing studies include (1) chemotherapy, in particular cyclophosphamide, decitabine, and temozolomide, (2) granulocyte-macrophage colony-stimulating factor (GM-CSF), and (3) adoptive transfer of T cells ( and ).
In summary, the field of clinical DC vaccines is moving toward ICB-resistant tumor landscapes (e.g., GBM), with particular emphasis on TAA-tailored or personalized approaches coupled with1e combinatorial regimen designing involving chemotherapeutics or ICBs.
Status update on clinical trials
Several clinical trials listed in our previous Trial Watch on DC-based vaccination for cancer therapy have changed status since its publication (July 2019).Citation149 NCT03688178, NCT03546426, NCT03743298 are now “Recruiting,” after being previously listed as “Not yet recruiting.” NCT03450044, NCT03152565, NCT03086564, NCT03707808, which were previously listed as “Recruiting,” have now been listed as “Completed.” NCT03657966, NCT03615404, NCT03114631changed from “Active, not recruiting” to “Completed.” The following trials have changed to “Active, not recruiting,” from “Recruiting”: NCT03325101, NCT03083054, NCT03387553, NCT03400917, NCT03406715, NCT03686683, NCT03697707. NCT03360708 changed from “Not yet recruiting” to “Active, not recruiting.” Moreover, several trials are now currently listed with “Unknown” status (due to status not being verified in the past 2 years): NCT03085966, NCT03638765, NCT03815084, NCT03214939, NCT03410732, NCT03393416, NCT03674073, NCT03282617, NCT03525652, NCT03185429, NCT03360630, NCT03190811, NCT03057340, NCT03736330, NCT03047525, NCT03815630. Lastly, the following trials have been terminated: NCT03300843 (slow accrual), NCT03735290 (decision made to not move forward to phase II), or NCT03782064 (lack of funding).
Concluding remarks
Based on our clinical trials survey, some decline in the number of published as well as ongoing clinical trials applying DC vaccines for anticancer therapy is noticeable.Citation237,Citation238 It is reasonable to believe that the rapid establishment of ICBs as standard of care for many cancers coupled with disappointing clinical performance of DC vaccines are together responsible for this decline. However, it is also clear that ICBs cannot be used for treatment of all cancers, and primary and secondary resistance to ICB remains an unresolved issue in cancer types where ICBs are used.Citation239–241 In line with this, DC vaccines are starting to occupy niche applications, e.g., GBM, which has failed to respond to ICBs across a series of high-profile clinical trials.Citation242–244 This suggests that there is great interest in delineating specific tumor niches where DC vaccines can be applied, especially in combination with other conventional (e.g., chemotherapy) or immunotherapeutic (e.g., ICBs) anticancer therapies, where the use of DC vaccines to prime tumors for subsequent T cell-based therapies is also being explored.Citation245–249
Clinical trials applying DC vaccines need to urgently demonstrate some clear survival advantages or at least reveal clear mechanisms for their failures to move the field toward actionable deliverables, like approval of DC-based vaccines by regulatory authorities or ‘next-generation’ clinical trials with novel designs and DC vaccine formulations striving to overcome previous failures. Completed Phase III clinical trials in the field are scarce and thus far have failed to show significant advantage achieved via DC-based vaccines in cancer patients.Citation204,Citation250 The results of other advanced trials that are currently ongoing or recently finished are eagerly awaited, particularly for those cancer-types that have failed to respond to currently approved ICBs.
Based on recent trends in the field of DC vaccines, there seems to be a prevalent disconnect between preclinical focus and clinical requirements. The preclinical DC vaccine research continues to focus on increasing the immunogenicity of DC vaccines (e.g., new maturating agents). However, over the last decades several DC vaccine formulations with sufficiently wide spectrum of immunogenicity have been clinically tested without sufficiently proportional clinical success.Citation101,Citation251–255 Thus, it is not clear if the lack of immunogenicity is the sole reason for clinical failure of DC vaccines or if there are also specific primary, adaptive, or acquired resistance pathways that require better attention, together with the limitations of preclinical models in accurately reflecting the clinical reality.Citation256–259 We believe that preclinical research needs to better address the previous clinical failures of DC vaccines and move forward in a definitive fashion to avoid redundancy. This will likely entail a shift from moDC-based vaccines to physiological DC subsets-based vaccination, among other avenues.Citation255,Citation260–263 Yet, as of now the difficulty in generating cDC1/2 from patients in large amounts remains a hurdle for cDC-based DC vaccinations. Simultaneously, DC vaccine research also needs to account for challenges that are also plaguing success of ICBs in the clinic, e.g., deep immunosuppressive niches within the TME of lymphocytes-depleted solid tumors,Citation249,Citation264–266 adaptive or acquired emergence of antigen-loss variant versions of cancer cells,Citation267–270 and the patient-to-patient immune heterogeneity (owing to immune haplotypes, archetypes, ethnicities, as well as microbiome variations).Citation271,Citation272 Manufacturing and production costs associated with DC vaccines also need to be considered and reduced, possibly through higher automation, to ease overall practicability.
Finally, ICBs owe a large part of their clinical success to guided application via specific immune biomarkers like tumor mutational burden or PD-L1 positivity.Citation273–282 These trends have highlighted the values of biomarker-driven patient pre-selection for the success of anticancer immunotherapy.Citation283–286 However, unfortunately, there is a severe lack of robust patient pre-selection biomarkers to guide the application of DC-based vaccines, thereby setting them up for failure. This situation needs to rapidly change. Therefore, it is urgent to use multi-omics biomarker profiling technologies to comprehensively document the biomarkers most likely to predict positive patient responses to DC vaccines. This will help support the design of clinical trials focused on patient subsets selected via specific biomarkers, thereby increasing their response probability to DC vaccines.
Despite their limited success to date, DC vaccines still exhibit immense potential as a tool in cancer immunotherapy, and novel technologies and the ever-increasing knowledge of DC biology and immune resistance mechanisms in the TME will allow for the engineering of better DC vaccines, along with increased personalization. We believe that DC vaccines stand to occupy an essential niche in the sensitization and priming of tumors to overcome ICB non-responsiveness in various cancer types and patients.
Acknowledgements
Research in ADG lab is supported by Research Foundation Flanders (FWO) (Fundamental Research Grant, G0B4620N; Excellence of Science/EOS grant, 30837538, for ‘DECODE’ consortium), KU Leuven (C1 grant, C14/19/098, C3 grant, C3/21/037, and POR award funds, POR/16/040), Kom op Tegen Kanker (Stand Up To Cancer, the Flemish Cancer Society) (KOTK/2018/11509/1 to ADG, SDV; KOTK/2019/11955/1 to AC, ADG), VLIR-UoS (iBOF grant, iBOF/21/048, for ‘MIMICRY’ consortium). JS is funded by Kom op tegen Kanker (Stand up to Cancer), the Flemish Cancer Society via Emmanuel van der Schueren (EvDS) PhD fellowship (projectID: 12699). IV is supported by FWO-SB PhD Fellowship (1S06821N). DMB is supported by KU Leuven’s Postdoctoral mandate grant (PDMT1/21/032). BB is supported by FWO Senior Clinical Investigator Fellowship (1801520N). LK is funded by Kom op tegen Kanker (Stand up to Cancer), the Flemish cancer society via Emmanuel van der Schueren (EvDS) PhD fellowship (projectID: 3328). JdV is supported by Health Holland grant LSHM18056-SGF. Research in ZB lab is supported by Kom op Tegen Kanker (Stand Up To Cancer, the Flemish Cancer Society) (including KotK_UA/2018/11465/1 and KotK_UZA/2018/11465/1), Stichting tegen Kanker (Foundation against Cancer) (CA/2016/753, CA/2020/1496), Research Foundation Flanders (FWO) (Applied Biomedical Research with a Primary Social Finality Grant, T00216N), Belgian National Cancer Plan, Stichting Semmy (Semmy Foundation), Olivia Hendrickx Research Fund vzw and UZA foundation. Research in the lab of BN and ST is supported by Kom op Tegen Kanker (Stand Up To Cancer, the Flemish Cancer Society) and by the Dutch Brain Tumor Foundation.
Disclosure statement
ADG has received speaker/lecture remuneration or consultancy fees from Boehringer Ingelheim, Miltenyi Biotec or Isoplexis. AC is a contracted researcher for Oncoinvent AS and Novocure and a consultant for Sotio a.s.
Data availability statement
All data included in this Trial Watch are publicly available at https://clinicaltrials.gov/
References
- Steinman RM, Cohn ZA. Identification of a novel cell type in peripheral lymphoid organs of mice. I. Morphology, quantitation, tissue distribution. J Exp Med. 1973;137:1142–19. doi:10.1084/jem.137.5.1142.
- Lanzavecchia A, Sallusto FR, Steinman M. 1943-2011. Cell. 2011;147:1216–1217. doi:10.1016/j.cell.2011.11.040.
- Randolph GJ. Dendritic cells: the first step. J Exp Med. 2021 Mar 1;218(3); e20202077. doi:10.1084/jem.20202077. PMID: 33600554; PMCID: PMC7888350.
- Soltani S, Mahmoudi M, Farhadi E. Dendritic cells currently under the spotlight; classification and subset based upon new markers. Immunol Invest. 2021;50:646–661. doi:10.1080/08820139.2020.1783289.
- Jhunjhunwala S, Hammer C, Delamarre L. Antigen presentation in cancer: insights into tumour immunogenicity and immune evasion. Nat Rev Cancer. 2021;21:298–312. doi:10.1038/s41568-021-00339-z.
- Eisenbarth SC. Dendritic cell subsets in T cell programming: location dictates function. Nat Rev Immunol. 2019;19:89–103. doi:10.1038/s41577-018-0088-1.
- Joffre OP, Segura E, Savina A, Amigorena S. Cross-presentation by dendritic cells. Nat Rev Immunol. 2012;12:557–569. doi:10.1038/nri3254.
- Collin M, Bigley V. Human dendritic cell subsets: an update. Immunology. 2018;154:3–20. doi:10.1111/imm.12888.
- Kvedaraite E, Ginhoux F. Human dendritic cells in cancer. Sci Immunol. 2022;7:eabm9409. doi:10.1126/sciimmunol.abm9409.
- Anderson DA, Dutertre C-A, Ginhoux F, Murphy KM. Genetic models of human and mouse dendritic cell development and function. Nat Rev Immunol. 2021;21:101–115. doi:10.1038/s41577-020-00413-x.
- Ginhoux F, Guilliams M, Merad M. Expanding dendritic cell nomenclature in the single-cell era. Nat Rev Immunol. 2022;22:67–68. doi:10.1038/s41577-022-00675-7.
- Villani A-C, Satija R, Reynolds G, Sarkizova S, Shekhar K, Fletcher J, Griesbeck M, Butler A, Zheng S, Lazo S, et al. Single-cell RNA-seq reveals new types of human blood dendritic cells, monocytes, and progenitors. Science. 2017;356. doi:10.1126/science.aah4573
- See P, Dutertre C-A, Chen J, Günther P, McGovern N, Irac SE, Gunawan M, Beyer M, Händler K, Duan K, et al. Mapping the human DC lineage through the integration of high-dimensional techniques. Science. 2017;356. doi:10.1126/science.aag3009
- Brown CC, Gudjonson H, Pritykin Y, Deep D, Lavallée V-P, Mendoza A, Fromme R, Mazutis L, Ariyan C, Leslie C, et al. Transcriptional basis of mouse and human dendritic cell heterogeneity. Cell. 2019;179:846–863.e24. doi:10.1016/j.cell.2019.09.035.
- Collin M, McGovern N, Haniffa M. Human dendritic cell subsets. Immunology. 2013;140:22–30. doi:10.1111/imm.12117.
- Gerhard GM, Bill R, Messemaker M, Klein AM, and Pittet MJ. Tumor-infiltrating dendritic cell states are conserved across solid human cancers. J Exp Med. 2021 Jan 4;218(1): e20200264. doi:10.1084/jem.20200264. PMID: 33601412; PMCID: PMC7754678.
- Schlitzer A, Sivakamasundari V, Chen J, Sumatoh HRB, Schreuder J, Lum J, Malleret B, Zhang S, Larbi A, Zolezzi F, et al. Identification of cDC1- and cDC2-committed DC progenitors reveals early lineage priming at the common DC progenitor stage in the bone marrow. Nat Immunol. 2015;16:718–728. doi:10.1038/ni.3200.
- Guilliams M, Ginhoux F, Jakubzick C, Naik SH, Onai N, Schraml BU, Segura E, Tussiwand R, Yona S. Dendritic cells, monocytes and macrophages: a unified nomenclature based on ontogeny. Nat Rev Immunol. 2014;14:571–578. doi:10.1038/nri3712.
- Rodrigues PF, Alberti-Servera L, Eremin A, Grajales-Reyes GE, Ivanek R, Tussiwand R. Distinct progenitor lineages contribute to the heterogeneity of plasmacytoid dendritic cells. Nat Immunol. 2018;19:711–722. doi:10.1038/s41590-018-0136-9.
- Dress RJ, Dutertre C-A, Giladi A, Schlitzer A, Low I, Shadan NB, Tay A, Lum J, Kairi MFBM, Hwang YY, et al. Plasmacytoid dendritic cells develop from Ly6D+ lymphoid progenitors distinct from the myeloid lineage. Nat Immunol. 2019;20:852–864. doi:10.1038/s41590-019-0420-3.
- Rodrigues PF, Tussiwand R. Novel concepts in plasmacytoid dendritic cell (pDC) development and differentiation. Mol Immunol. 2020;126:25–30. doi:10.1016/j.molimm.2020.07.006.
- Carpentier S, Vu Manh T-P, Chelbi R, Henri S, Malissen B, Haniffa M, Ginhoux F, Dalod M. Comparative genomics analysis of mononuclear phagocyte subsets confirms homology between lymphoid tissue-resident and dermal XCR1(+) DCs in mouse and human and distinguishes them from Langerhans cells. J Immunol Methods. 2016;432:35–49. doi:10.1016/j.jim.2016.02.023.
- Audsley KM, McDonnell AM, Waithman J. Cross-presenting XCR1+ dendritic cells as targets for cancer immunotherapy. Cells. 2020;9:565. doi:10.3390/cells9030565.
- Pipperger L, Riepler L, Kimpel J, Siller A, Stoitzner P, Bánki Z, von Laer D. Differential infection of murine and human dendritic cell subsets by oncolytic vesicular stomatitis virus variants. Oncoimmunology. 2021;10:1959140. doi:10.1080/2162402X.2021.1959140.
- Böttcher JP, Reise Sousa C. The role of type 1 conventional dendritic cells in cancer immunity. Trends Cancer. 2018;4:784–792. doi:10.1016/j.trecan.2018.09.001.
- Hildner K, Edelson BT, Purtha WE, Diamond M, Matsushita H, Kohyama M, Calderon B, Schraml BU, Unanue ER, Diamond MS, et al. Batf3 deficiency reveals a critical role for CD8alpha+ dendritic cells in cytotoxic T cell immunity. Science. 2008;322:1097–1100.
- Cancel J-C, Crozat K, Dalod M, Mattiuz R. Are conventional type 1 dendritic cells critical for protective antitumor immunity and how? Front Immunol. 2019;10:9. doi:10.3389/fimmu.2019.00009.
- Qiu CC, Kotredes KP, Cremers T, Patel S, Afanassiev A, Slifker M, Gallucci S, Gamero AM. Targeted Stat2 deletion in conventional dendritic cells impairs CTL responses but does not affect antibody production. Oncoimmunology. 2020;10:1860477. doi:10.1080/2162402X.2020.1860477.
- Salmon H, Idoyaga J, Rahman A, Leboeuf M, Remark R, Jordan S, Casanova-Acebes M, Khudoynazarova M, Agudo J, Tung N, et al. Expansion and activation of CD103(+) dendritic cell progenitors at the tumor site enhances tumor responses to therapeutic PD-L1 and BRAF inhibition. Immunity. 2016;44:924–938. doi:10.1016/j.immuni.2016.03.012.
- Broz ML, Binnewies M, Boldajipour B, Nelson AE, Pollack JL, Erle DJ, Barczak A, Rosenblum MD, Daud A, Barber DL, et al. Dissecting the tumor myeloid compartment reveals rare activating antigen-presenting cells critical for T cell immunity. Cancer Cell. 2014;26:638–652. doi:10.1016/j.ccell.2014.09.007.
- Pilones KA, Charpentier M, Garcia-Martinez E, Demaria S. IL15 synergizes with radiotherapy to reprogram the tumor immune contexture through a dendritic cell connection. Oncoimmunology. 2020;9:1790716. doi:10.1080/2162402X.2020.1790716.
- Lauterbach H, Bathke B, Gilles S, Traidl-Hoffmann C, Luber CA, Fejer G, Freudenberg MA, Davey GM, Vremec D, Kallies A, et al. Mouse CD8alpha+ DCs and human BDCA3+ DCs are major producers of IFN-lambda in response to poly IC. J Exp Med. 2010;207:2703–2717. doi:10.1084/jem.20092720.
- Lazear HM, Nice TJ, Diamond MS. Interferon-λ: immune functions at barrier surfaces and beyond. Immunity. 2015;43:15–28. doi:10.1016/j.immuni.2015.07.001.
- Horton BL, Fessenden TB, Spranger S. Tissue site and the cancer immunity cycle. Trends Cancer. 2019;5:593–603. doi:10.1016/j.trecan.2019.07.006.
- Binnewies M, Mujal AM, Pollack JL, Combes AJ, Hardison EA, Barry KC, Tsui J, Ruhland MK, Kersten K, Abushawish MA, et al. Unleashing Type-2 dendritic cells to drive protective antitumor CD4+ T cell immunity. Cell. 2019;177:556–571.e16. doi:10.1016/j.cell.2019.02.005.
- Saito Y, Komori S, Kotani T, Murata Y, Matozaki T. The role of Type-2 conventional dendritic cells in the regulation of tumor immunity. Cancers (Basel). 2022;14:1976. doi:10.3390/cancers14081976.
- Bennett SR, Carbone FR, Karamalis F, Miller JF, Heath WR. Induction of a CD8+ cytotoxic T lymphocyte response by cross-priming requires cognate CD4+ T cell help. J Exp Med. 1997;186:65–70. doi:10.1084/jem.186.1.65.
- Blasio HD. DCs are critical cellular mediators of immune responses induced by immunogenic cell death. Oncoimmunology. 2016;5(8): e1192739
- Nizzoli G, Krietsch J, Weick A, Steinfelder S, Facciotti F, Gruarin P, Bianco A, Steckel B, Moro M, Crosti M, et al. Human CD1c+ dendritic cells secrete high levels of IL-12 and potently prime cytotoxic T-cell responses. Blood. 2013;122:932–942. doi:10.1182/blood-2013-04-495424.
- Balan S, Radford KJ, Bhardwaj N. Unexplored horizons of cDC1 in immunity and tolerance. Adv Immunol. 2020;148:49–91.
- Jeon D, McNeel DG. Toll-like receptor agonist combinations augment mouse T-cell anti-tumor immunity via IL-12- and interferon ß-mediated suppression of immune checkpoint receptor expression. Oncoimmunology. 2022;11:2054758. doi:10.1080/2162402X.2022.2054758.
- Li Q, Li Y, Wang Y, Xu L, Guo Y, Wang Y, Wang L, Guo C. Oral administration of Bifidobacterium breve promotes antitumor efficacy via dendritic cells-derived interleukin 12. Oncoimmunology. 2021;10:1868122. doi:10.1080/2162402X.2020.1868122.
- Reindl LM, Albinger N, Bexte T, Müller S, Hartmann J, Ullrich E. Immunotherapy with NK cells: recent developments in gene modification open up new avenues. Oncoimmunology. 2020;9:1777651. doi:10.1080/2162402X.2020.1777651.
- Cella M, Facchetti F, Lanzavecchia A, Colonna M. Plasmacytoid dendritic cells activated by influenza virus and CD40L drive a potent TH1 polarization. Nat Immunol. 2000;1:305–310. doi:10.1038/79747.
- Sprooten J, Agostinis P, Garg AD. Type I interferons and dendritic cells in cancer immunotherapy. Int Rev Cell Mol Biol. 2019;348:217–262.
- Gilliet M, Boonstra A, Paturel C, Antonenko S, Xu X-L, Trinchieri G, O’Garra A, Liu Y-J. The development of murine plasmacytoid dendritic cell precursors is differentially regulated by FLT3-ligand and granulocyte/macrophage colony-stimulating factor. J Exp Med. 2002;195:953–958. doi:10.1084/jem.20020045.
- Sprooten J, Garg AD. Type I interferons and endoplasmic reticulum stress in health and disease. Int Rev Cell Mol Biol. 2020;350:63–118. doi:10.1016/bs.ircmb.2019.10.004.
- Labidi-Galy SI, Sisirak V, Meeus P, Gobert M, Treilleux I, Bajard A, Combes J-D, Faget J, Mithieux F, Cassignol A, et al. Quantitative and functional alterations of plasmacytoid dendritic cells contribute to immune tolerance in ovarian cancer. Cancer Res. 2011;71:5423–5434. doi:10.1158/0008-5472.CAN-11-0367.
- Demoulin S, Herfs M, Somja J, Roncarati P, Delvenne P, Hubert P. HMGB1 secretion during cervical carcinogenesis promotes the acquisition of a tolerogenic functionality by plasmacytoid dendritic cells. Int J Cancer. 2015;137:345–358. doi:10.1002/ijc.29389.
- Hartmann E, Wollenberg B, Rothenfusser S, Wagner M, Wellisch D, Mack B, Giese T, Gires O, Endres S, Hartmann G. Identification and functional analysis of tumor-infiltrating plasmacytoid dendritic cells in head and neck cancer. Cancer Res. 2003;63:6478–6487.
- Sisirak V, Vey N, Goutagny N, Renaudineau S, Malfroy M, Thys S, Treilleux I, Labidi-Galy SI, Bachelot T, Dezutter-Dambuyant C, et al. Breast cancer-derived transforming growth factor-β and tumor necrosis factor-α compromise interferon-α production by tumor-associated plasmacytoid dendritic cells. Int J Cancer. 2013;133:771–778. doi:10.1002/ijc.28072.
- Aspord C, Leccia M-T, Charles J, Plumas J. Plasmacytoid dendritic cells support melanoma progression by promoting Th2 and regulatory immunity through OX40L and ICOSL. Cancer Immunol Res. 2013;1:402–415. doi:10.1158/2326-6066.CIR-13-0114-T.
- Gong W, Donnelly CR, Heath BR, Bellile E, Donnelly LA, Taner HF, Broses L, Brenner JC, Chinn SB, R-R J, et al. Cancer-specific type-I interferon receptor signaling promotes cancer stemness and effector CD8+ T-cell exhaustion. Oncoimmunology. 2021;10:1997385. doi:10.1080/2162402X.2021.1997385.
- Zhang X, Wang S, Zhu Y, Zhang M, Zhao Y, Yan Z, Wang Q, Li X. Double-edged effects of interferons on the regulation of cancer-immunity cycle. Oncoimmunology. 2021;10:1929005. doi:10.1080/2162402X.2021.1929005.
- Galluzzi L, Yamazaki T, Kroemer G. Linking cellular stress responses to systemic homeostasis. Nat Rev Mol Cell Biol. 2018;19:731–745. doi:10.1038/s41580-018-0068-0.
- Mahnke K, Schmitt E, Bonifaz L, Enk AH, Jonuleit H. Immature, but not inactive: the tolerogenic function of immature dendritic cells. Immunol Cell Biol. 2002;80:477–483. doi:10.1046/j.1440-1711.2002.01115.x.
- Angelova M, Mascaux C, Galon J. Evasion before invasion: pre-cancer immunosurveillance. Oncoimmunology. 2021;10:1912250. doi:10.1080/2162402X.2021.1912250.
- Yu H, Tian Y, Wang Y, Mineishi S, Zhang Y. Dendritic cell regulation of Graft-Vs.-Host disease: immunostimulation and tolerance. Front Immunol. 2019;10:93. doi:10.3389/fimmu.2019.00093.
- Volkmann A, Zal T, Stockinger B. Antigen-presenting cells in the thymus that can negatively select MHC class II-restricted T cells recognizing a circulating self antigen. J Immunol. 1997;158:693–706.
- Idoyaga J, Fiorese C, Zbytnuik L, Lubkin A, Miller J, Malissen B, Mucida D, Merad M, Steinman RM. Specialized role of migratory dendritic cells in peripheral tolerance induction. J Clin Invest. 2013;123:844–854. doi:10.1172/JCI65260.
- Roncarolo MG, Levings MK, Traversari C. Differentiation of T regulatory cells by immature dendritic cells. J Exp Med. 2001;193:F5–9. doi:10.1084/jem.193.2.F5.
- Mahnke K, Enk AH. Dendritic cells: key cells for the induction of regulatory T cells? Curr Top Microbiol Immunol. 2005;293:133–150. doi:10.1007/3-540-27702-1_7.
- Palucka K, Banchereau J. Cancer immunotherapy via dendritic cells. Nat Rev Cancer. 2012;12:265–277. doi:10.1038/nrc3258.
- Liu S, Zhang Y, Ren J, Li J. Microbial DNA recognition by cGAS-STING and other sensors in dendritic cells in inflammatory bowel diseases. Inflamm Bowel Dis. 2015;21:901–911. doi:10.1097/MIB.0000000000000299.
- Reise Sousa C, Sher A, Kaye P. The role of dendritic cells in the induction and regulation of immunity to microbial infection. Curr Opin Immunol. 1999;11:392–399. doi:10.1016/S0952-7915(99)80066-1.
- Sistigu A, Yamazaki T, Vacchelli E, Chaba K, Enot DP, Adam J, Vitale I, Goubar A, Baracco EE, Remédios C, et al. Cancer cell-autonomous contribution of type I interferon signaling to the efficacy of chemotherapy. Nat Med. 2014;20:1301–1309. doi:10.1038/nm.3708.
- Oth T, Vanderlocht J, Elssen CHMJ V, Bos GMJ, Germeraad WTV. Pathogen-associated molecular patterns induced crosstalk between Dendritic Cells, T Helper Cells, and Natural Killer Helper Cells can improve dendritic cell vaccination. Mediators Inflamm. 2016;2016:5740373. doi:10.1155/2016/5740373.
- Yatim N, Cullen S, Albert ML. Dying cells actively regulate adaptive immune responses. Nat Rev Immunol. 2017;17:262–275. doi:10.1038/nri.2017.9.
- Garg AD, Dudek AM, Agostinis P. Cancer immunogenicity, danger signals, and DAMPs: what, when, and how? Biofactors. 2013;39:355–367. doi:10.1002/biof.1125.
- Nace G, Evankovich J, Eid R, Tsung A. Dendritic cells and damage-associated molecular patterns: endogenous danger signals linking innate and adaptive immunity. J Innate Immun. 2012;4:6–15. doi:10.1159/000334245.
- Sprooten J, De Wijngaert P, Vanmeerbeerk I, Martin S, Vangheluwe P, Schlenner S, Krysko DV, Parys JB, Bultynck G, Vandenabeele P, et al. Necroptosis in immuno-oncology and cancer immunotherapy. Cells. 2020;9:1823. doi:10.3390/cells9081823.
- Cirone M, Di Renzo L, Lotti LV, Conte V, Trivedi P, Santarelli R, Gonnella R, Frati L, Faggioni A. Activation of dendritic cells by tumor cell death. Oncoimmunology. 2012;1:1218–1219. doi:10.4161/onci.20428.
- Kroemer G, Galassi C, Zitvogel L, Galluzzi L. Immunogenic cell stress and death. Nat Immunol. 2022;23:487–500. doi:10.1038/s41590-022-01132-2.
- West MA, Prescott AR, Chan KM, Zhou Z, Rose-John S, Scheller J, Watts C. TLR ligand-induced podosome disassembly in dendritic cells is ADAM17 dependent. J Cell Biol. 2008;182:993–1005. doi:10.1083/jcb.200801022.
- Granucci F, Ferrero E, Foti M, Aggujaro D, Vettoretto K, Ricciardi-Castagnoli P. Early events in dendritic cell maturation induced by LPS. Microbes Infect. 1999;1:1079–1084. doi:10.1016/S1286-4579(99)00209-9.
- Reise Sousa C. Dendritic cells in a mature age. Nat Rev Immunol. 2006;6:476–483. doi:10.1038/nri1845.
- Mellman I, Steinman RM. Dendritic cells: specialized and regulated antigen processing machines. Cell. 2001;106:255–258. doi:10.1016/S0092-8674(01)00449-4.
- Merad M, Sathe P, Helft J, Miller J, Mortha A. The dendritic cell lineage: ontogeny and function of dendritic cells and their subsets in the steady state and the inflamed setting. Annu Rev Immunol. 2013;31:563–604. doi:10.1146/annurev-immunol-020711-074950.
- Dearman RJ, Cumberbatch M, Maxwell G, Basketter DA, Kimber I. Toll-like receptor ligand activation of murine bone marrow-derived dendritic cells. Immunology. 2009;126:475–484. doi:10.1111/j.1365-2567.2008.02922.x.
- Majumder S, Bhattacharjee S, Paul Chowdhury B, Majumdar S, Rodrigues MM. CXCL10 is critical for the generation of protective CD8 T cell response induced by antigen pulsed CpG-ODN activated dendritic cells. PLoS One. 2012;7:e48727. doi:10.1371/journal.pone.0048727.
- Lutz MB, Backer RA, Clausen BE. Revisiting current concepts on the tolerogenicity of steady-state dendritic cell subsets and their maturation stages. J Immunol. 2021;206:1681–1689. doi:10.4049/jimmunol.2001315.
- Garg AD, Romano E, Rufo N, Agostinis P. Immunogenic versus tolerogenic phagocytosis during anticancer therapy: mechanisms and clinical translation. Cell Death Differ. 2016;23:938–951. doi:10.1038/cdd.2016.5.
- Fang S, Agostinis P, Salven P, Garg AD. Decoding cancer cell death-driven immune cell recruitment: an in vivo method for site-of-vaccination analyses. Meth Enzymol. 2020;636:185–207.
- Spranger S, Dai D, Horton B, Gajewski TF. Tumor-residing Batf3 dendritic cells are required for effector T Cell trafficking and adoptive T Cell therapy. Cancer Cell. 2017;31:711–723.e4. doi:10.1016/j.ccell.2017.04.003.
- de Mingo Pulido Á, Hänggi K, Celias DP, Gardner A, Li J, Batista-Bittencourt B, Mohamed E, Trillo-Tinoco J, Osunmakinde O, Peña R, et al. The inhibitory receptor TIM-3 limits activation of the cGAS-STING pathway in intra-tumoral dendritic cells by suppressing extracellular DNA uptake. Immunity. 2021;54:1154–1167.e7. doi:10.1016/j.immuni.2021.04.019.
- Wang Y, Xiang Y, Xin VW, Wang X-W, Peng X-C, Liu X-Q, Wang D, Li N, Cheng J-T, Lyv Y-N, et al. Dendritic cell biology and its role in tumor immunotherapy. J Hematol Oncol. 2020;13:107. doi:10.1186/s13045-020-00939-6.
- Fu C, Jiang A. Dendritic cells and CD8 T cell immunity in tumor microenvironment. Front Immunol. 2018;9:3059. doi:10.3389/fimmu.2018.03059.
- Tormoen GW, Crittenden MR, Gough MJ. Role of the immunosuppressive microenvironment in immunotherapy. Adv Radiat Oncol. 2018;3:520–526. doi:10.1016/j.adro.2018.08.018.
- Wang Y-M, Qiu -J-J, X-Y Q, Peng J, Lu C, Zhang M, Zhang M-X, X-L Q, Lv B, Guo -J-J, et al. Accumulation of dysfunctional tumor-infiltrating PD-1+ DCs links PD-1/PD-L1 blockade immunotherapeutic response in cervical cancer. Oncoimmunology. 2022;11:2034257. doi:10.1080/2162402X.2022.2034257.
- Hassan Venkatesh G, Abou Khouzam R, Shaaban Moustafa Elsayed W, Ahmed Zeinelabdin N, Terry S, Chouaib S. Tumor hypoxia: an important regulator of tumor progression or a potential modulator of tumor immunogenicity? Oncoimmunology. 2021;10:1974233. doi:10.1080/2162402X.2021.1974233.
- Lu Y, Shi Y, You J. Strategy and clinical application of up-regulating cross presentation by DCs in anti-tumor therapy. J Control Release. 2022;341:184–205. doi:10.1016/j.jconrel.2021.11.011.
- Sadeghzadeh M, Bornehdeli S, Mohahammadrezakhani H, Abolghasemi M, Poursaei E, Asadi M, Zafari V, Aghebati-Maleki L, Shanehbandi D. Dendritic cell therapy in cancer treatment; the state-of-the-art. Life Sci. 2020;254:117580. doi:10.1016/j.lfs.2020.117580.
- Gardner A, de Mingo Pulido Á, Ruffell B. Dendritic cells and their role in immunotherapy. Front Immunol. 2020;11:924. doi:10.3389/fimmu.2020.00924.
- Wculek SK, Cueto FJ, Mujal AM, Melero I, Krummel MF, Sancho D. Dendritic cells in cancer immunology and immunotherapy. Nat Rev Immunol. 2020;20:7–24. doi:10.1038/s41577-019-0210-z.
- Harari A, Graciotti M, Bassani-Sternberg M, Kandalaft LE. Antitumour dendritic cell vaccination in a priming and boosting approach. Nat Rev Drug Discov. 2020;19:635–652. doi:10.1038/s41573-020-0074-8.
- Y-z G, Zhao X, Song X-R. Ex vivo pulsed dendritic cell vaccination against cancer. Acta Pharmacol Sin. 2020;41:959–969. doi:10.1038/s41401-020-0415-5.
- Han P, Hanlon D, Sobolev O, Chaudhury R, Edelson RL. Ex vivo dendritic cell generation-A critical comparison of current approaches. Int Rev Cell Mol Biol. 2019;349:251–307. doi:10.1016/bs.ircmb.2019.10.003.
- Giri B, Sharma P, Jain T, Ferrantella A, Vaish U, Mehra S, Garg B, Iyer S, Sethi V, Malchiodi Z, et al. Hsp70 modulates immune response in pancreatic cancer through dendritic cells. Oncoimmunology. 2021;10:1976952. doi:10.1080/2162402X.2021.1976952.
- DeVette CI, Gundlapalli H, Lai S-CA, McMurtrey CP, Hoover AR, Gurung HR, Chen WR, Welm AL, Hildebrand WH. A pipeline for identification and validation of tumor-specific antigens in a mouse model of metastatic breast cancer. Oncoimmunology. 2020;9:1685300. doi:10.1080/2162402X.2019.1685300.
- Sutherland SIM, Ju X, Horvath LG, Clark GJ. Moving on from sipuleucel-T: new dendritic cell vaccine strategies for prostate cancer. Front Immunol. 2021;12:641307. doi:10.3389/fimmu.2021.641307.
- Schaller TH, Sampson JH. Advances and challenges: dendritic cell vaccination strategies for glioblastoma. Expert Rev Vaccines. 2017;16:27–36. doi:10.1080/14760584.2016.1218762.
- Petroni G, Buqué A, Coussens LM, Galluzzi L. Targeting oncogene and non-oncogene addiction to inflame the tumour microenvironment. Nat Rev Drug Discov. 2022;21:440–462. doi:10.1038/s41573-022-00415-5.
- Laoui D, Keirsse J, Morias Y, Van Overmeire E, Geeraerts X, Elkrim Y, Kiss M, Bolli E, Lahmar Q, Sichien D, et al. The tumour microenvironment harbours ontogenically distinct dendritic cell populations with opposing effects on tumour immunity. Nat Commun. 2016;7:13720. doi:10.1038/ncomms13720.
- Filin IY, Kitaeva KV, Rutland CS, Rizvanov AA, Solovyeva VV. Recent advances in experimental dendritic cell vaccines for cancer. Front Oncol. 2021;11:730824. doi:10.3389/fonc.2021.730824.
- Schreibelt G, Bol KF, Westdorp H, Wimmers F, Aarntzen EHJG, Duiveman-de Boer T, van de Rakt MWMM, Scharenborg NM, de Boer AJ, Pots JM, et al. Effective clinical responses in metastatic melanoma patients after vaccination with primary myeloid dendritic cells. Clin Cancer Res. 2016;22:2155–2166. doi:10.1158/1078-0432.CCR-15-2205.
- Chung DJ, Carvajal RD, Postow MA, Sharma S, Pronschinske KB, Shyer JA, Singh-Kandah S, Dickson MA, D’Angelo SP, Wolchok JD, et al. Langerhans-type dendritic cells electroporated with TRP-2 mRNA stimulate cellular immunity against melanoma: results of a phase I vaccine trial. Oncoimmunology. 2017;7:e1372081. doi:10.1080/2162402X.2017.1372081.
- Tel J, Aarntzen EHJG, Baba T, Schreibelt G, Schulte BM, Benitez-Ribas D, Boerman OC, Croockewit S, Oyen WJG, van Rossum M, et al. Natural human plasmacytoid dendritic cells induce antigen-specific T-cell responses in melanoma patients. Cancer Res. 2013;73:1063–1075. doi:10.1158/0008-5472.CAN-12-2583.
- Huber A, Dammeijer F, Jgjv A, Vroman H. Current state of dendritic cell-based immunotherapy: opportunities for in vitro antigen loading of different DC subsets? Front Immunol. 2018;9:2804. doi:10.3389/fimmu.2018.02804.
- Johnson P, Rosendahl N, Radford KJ. Conventional type 1 dendritic cells (cDC1) as cancer therapeutics: challenges and opportunities. Expert Opin Biol Ther. 2022;22:465–472. doi:10.1080/14712598.2022.1994943.
- Charles J, Chaperot L, Hannani D, Bruder Costa J, Templier I, Trabelsi S, Gil H, Moisan A, Persoons V, Hegelhofer H, et al. An innovative plasmacytoid dendritic cell line-based cancer vaccine primes and expands antitumor T-cells in melanoma patients in a first-in-human trial. Oncoimmunology. 2020;9:1738812. doi:10.1080/2162402X.2020.1738812.
- Bloemendal M, Bol KF, Boudewijns S, Gorris MAJ, de Wilt JHW, Croockewit SAJ, van Rossum MM, de Goede AL, Petry K, and Koornstra RHT, et al. Immunological responses to adjuvant vaccination with combined CD1c+ myeloid and plasmacytoid dendritic cells in stage III melanoma patients. Oncoimmunology. 2022;11:1. doi:10.1080/2162402X.2021.2015113.
- Santos PM, Butterfield LH. Dendritic Cell-based cancer vaccines. J Immunol. 2018;200:443–449. doi:10.4049/jimmunol.1701024.
- Satoh Y, Esche C, Gambotto A, Shurin GV, Yurkovetsky ZR, Robbins PD, Watkins SC, Todo S, Herberman RB, Lotze MT, et al. Local administration of IL-12-transfected dendritic cells induces antitumor immune responses to colon adenocarcinoma in the liver in mice. J Exp Ther Oncol. 2002;2:337–349. doi:10.1046/j.1359-4117.2002.01050.x.
- Yang S-C, Hillinger S, Riedl K, Zhang L, Zhu L, Huang M, Atianzar K, Kuo BY, Gardner B, Batra RK, et al. Intratumoral administration of dendritic cells overexpressing CCL21 generates systemic antitumor responses and confers tumor immunity. Clin Cancer Res. 2004;10:2891–2901. doi:10.1158/1078-0432.CCR-03-0380.
- Nishioka Y, Hirao M, Robbins PD, Lotze MT, Tahara H. Induction of systemic and therapeutic antitumor immunity using intratumoral injection of dendritic cells genetically modified to express interleukin 12. Cancer Res. 1999;59:4035–4041.
- Hu J, Yuan X, Belladonna ML, Ong JM, Wachsmann-Hogiu S, Farkas DL, Black KL, Yu JS. Induction of potent antitumor immunity by intratumoral injection of interleukin 23-transduced dendritic cells. Cancer Res. 2006;66:8887–8896. doi:10.1158/0008-5472.CAN-05-3448.
- Endo H, Saito T, Kenjo A, Hoshino M, Terashima M, Sato T, Anazawa T, Kimura T, Tsuchiya T, Irisawa A, et al. Phase I trial of preoperative intratumoral injection of immature dendritic cells and OK-432 for resectable pancreatic cancer patients. J Hepatobiliary Pancreat Sci. 2012;19:465–475. doi:10.1007/s00534-011-0457-7.
- Jeong H, Lee S-Y, Seo H, Kim DH, Lee D, Kim B-J. Potential of Mycobacterium tuberculosis chorismate mutase (Rv1885c) as a novel TLR4-mediated adjuvant for dendritic cell-based cancer immunotherapy. Oncoimmunology. 2022;11:2023340. doi:10.1080/2162402X.2021.2023340.
- Aerts JGJV, de Goeje PL, Cornelissen R, Kaijen-Lambers MEH, Bezemer K, van der Leest CH, Mahaweni NM, Kunert A, Eskens FALM, Waasdorp C, et al. Autologous dendritic cells pulsed with allogeneic tumor cell lysate in mesothelioma: from mouse to human. Clin Cancer Res. 2018;24:766–776. doi:10.1158/1078-0432.CCR-17-2522.
- Chen Z, Moyana T, Saxena A, Warrington R, Jia Z, Xiang J. Efficient antitumor immunity derived from maturation of dendritic cells that had phagocytosed apoptotic/necrotic tumor cells. Int J Cancer. 2001;93:539–548. doi:10.1002/ijc.1365.
- Fields RC, Shimizu K, Mulé JJ. Murine dendritic cells pulsed with whole tumor lysates mediate potent antitumor immune responses in vitro and in vivo. Proc Natl Acad Sci USA. 1998;95:9482–9487. doi:10.1073/pnas.95.16.9482.
- Albert ML, Sauter B, Bhardwaj N. Dendritic cells acquire antigen from apoptotic cells and induce class I-restricted CTLs. Nature. 1998;392:86–89. doi:10.1038/32183.
- González FE, Gleisner A, Falcón-Beas F, Osorio F, López MN, Salazar-Onfray F. Tumor cell lysates as immunogenic sources for cancer vaccine design. Hum Vaccin Immunother. 2014;10:3261–3269. doi:10.4161/21645515.2014.982996.
- Rojas-Sepúlveda D, Tittarelli A, Gleisner MA, Ávalos I, Pereda C, Gallegos I, González FE, López MN, Butte JM, Roa JC, et al. Tumor lysate-based vaccines: on the road to immunotherapy for gallbladder cancer. Cancer Immunol Immunother. 2018;67:1897–1910. doi:10.1007/s00262-018-2157-5.
- Ding Z, Li Q, Zhang R, Xie L, Shu Y, Gao S, Wang P, Su X, Qin Y, Wang Y, et al. Personalized neoantigen pulsed dendritic cell vaccine for advanced lung cancer. Signal Transduct Target Ther. 2021;6:26. doi:10.1038/s41392-020-00448-5.
- Zhang R, Yuan F, Shu Y, Tian Y, Zhou B, Yi L, Zhang X, Ding Z, Xu H, Yang L. Personalized neoantigen-pulsed dendritic cell vaccines show superior immunogenicity to neoantigen-adjuvant vaccines in mouse tumor models. Cancer Immunol Immunother. 2020;69:135–145. doi:10.1007/s00262-019-02448-z.
- Hoffmann J-M, Schmitt M, Ni M, Schmitt A. Next-generation dendritic cell-based vaccines for leukemia patients. Immunotherapy. 2017;9:173–181. doi:10.2217/imt-2016-0116.
- Geskin LJ, Damiano JJ, Patrone CC, Butterfield LH, Kirkwood JM, Falo LD. Three antigen-loading methods in dendritic cell vaccines for metastatic melanoma. Melanoma Res. 2018;28:211–221. doi:10.1097/CMR.0000000000000441.
- Benencia F, Courrèges MC, Coukos G. Whole tumor antigen vaccination using dendritic cells: comparison of RNA electroporation and pulsing with UV-irradiated tumor cells. J Transl Med. 2008;6:21. doi:10.1186/1479-5876-6-21.
- Mayordomo JI, Loftus DJ, Sakamoto H, De Cesare CM, Appasamy PM, Lotze MT, Storkus WJ, Appella E, DeLeo AB. Therapy of murine tumors with p53 wild-type and mutant sequence peptide-based vaccines. J Exp Med. 1996;183:1357–1365. doi:10.1084/jem.183.4.1357.
- Paglia P, Chiodoni C, Rodolfo M, Colombo MP. Murine dendritic cells loaded in vitro with soluble protein prime cytotoxic T lymphocytes against tumor antigen in vivo. J Exp Med. 1996;183:317–322. doi:10.1084/jem.183.1.317.
- Boczkowski D, Nair SK, Snyder D, Gilboa E. Dendritic cells pulsed with RNA are potent antigen-presenting cells in vitro and in vivo. J Exp Med. 1996;184:465–472. doi:10.1084/jem.184.2.465.
- Ashley DM, Faiola B, Nair S, Hale LP, Bigner DD, Gilboa E. Bone marrow-generated dendritic cells pulsed with tumor extracts or tumor RNA induce antitumor immunity against central nervous system tumors. J Exp Med. 1997;186:1177–1182. doi:10.1084/jem.186.7.1177.
- Boczkowski D, Nair SK, Nam JH, Lyerly HK, Gilboa E. Induction of tumor immunity and cytotoxic T lymphocyte responses using dendritic cells transfected with messenger RNA amplified from tumor cells. Cancer Res. 2000;60:1028–1034.
- Wan Y, Emtage P, Zhu Q, Foley R, Pilon A, Roberts B, Gauldie J. Enhanced immune response to the melanoma antigen gp100 using recombinant adenovirus-transduced dendritic cells. Cell Immunol. 1999;198:131–138. doi:10.1006/cimm.1999.1585.
- Tüting T, Steitz J, Brück J, Gambotto A, Steinbrink K, DeLeo AB, Robbins P, Knop J, Enk AH. Dendritic cell-based genetic immunization in mice with a recombinant adenovirus encoding murine TRP2 induces effective anti-melanoma immunity. J Gene Med. 1999;1:400–406. doi:10.1002/(SICI)1521-2254(199911/12)1:6<400::AID-JGM68>3.0.CO;2-D.
- Fotaki G, Jin C, Kerzeli IK, Ramachandran M, Martikainen -M-M, Karlsson-Parra A, Yu D, Essand M. Cancer vaccine based on a combination of an infection-enhanced adenoviral vector and pro-inflammatory allogeneic DCs leads to sustained antigen-specific immune responses in three melanoma models. Oncoimmunology. 2018;7:e1397250. doi:10.1080/2162402X.2017.1397250.
- Wu Q, Xia D, Carlsen S, Xiang J. Adenovirus-mediated transgene-engineered dendritic cell vaccine of cancer. Curr Gene Ther. 2005;5:237–247. doi:10.2174/1566523053544272.
- Kranz LM, Diken M, Haas H, Kreiter S, Loquai C, Reuter KC, Meng M, Fritz D, Vascotto F, Hefesha H, et al. Systemic RNA delivery to dendritic cells exploits antiviral defence for cancer immunotherapy. Nature. 2016;534:396–401. doi:10.1038/nature18300.
- Cruz LJ, Tacken PJ, Pots JM, Torensma R, Buschow SI, Figdor CG. Comparison of antibodies and carbohydrates to target vaccines to human dendritic cells via DC-SIGN. Biomaterials. 2012;33:4229–4239. doi:10.1016/j.biomaterials.2012.02.036.
- Tacken PJ, de Vries IJM, Gijzen K, Joosten B, Wu D, Rother RP, Faas SJ, Punt CJA, Torensma R, Adema GJ, et al. Effective induction of naive and recall T-cell responses by targeting antigen to human dendritic cells via a humanized anti-DC-SIGN antibody. Blood. 2005;106:1278–1285. doi:10.1182/blood-2005-01-0318.
- Bonifaz L, Bonnyay D, Mahnke K, Rivera M, Nussenzweig MC, Steinman RM. Efficient targeting of protein antigen to the dendritic cell receptor DEC-205 in the steady state leads to antigen presentation on major histocompatibility complex class I products and peripheral CD8+ T cell tolerance. J Exp Med. 2002;196:1627–1638. doi:10.1084/jem.20021598.
- Liu H, Dumont C, Johnston APR, Mintern JD. Analysis of intracellular trafficking of dendritic cell receptors for antigen targeting. Methods Mol Biol. 2016;1423:199–209.
- Chappell CP, Giltiay NV, Dresch C, Clark EA. Controlling immune responses by targeting antigens to dendritic cell subsets and B cells. Int Immunol. 2014;26:3–11. doi:10.1093/intimm/dxt059.
- Hammerich L, Bhardwaj N, Kohrt HE, Brody JD. In situ vaccination for the treatment of cancer. Immunotherapy. 2016;8:315–330. doi:10.2217/imt.15.120.
- Zitvogel L, Regnault A, Lozier A, Wolfers J, Flament C, Tenza D, Ricciardi-Castagnoli P, Raposo G, Amigorena S. Eradication of established murine tumors using a novel cell-free vaccine: dendritic cell-derived exosomes. Nat Med. 1998;4:594–600. doi:10.1038/nm0598-594.
- Théry C, Regnault A, Garin J, Wolfers J, Zitvogel L, Ricciardi-Castagnoli P, Raposo G, Amigorena S. Molecular characterization of dendritic cell-derived exosomes. Selective accumulation of the heat shock protein hsc73. J Cell Biol. 1999;147:599–610. doi:10.1083/jcb.147.3.599.
- Viaud S, Théry C, Ploix S, Tursz T, Lapierre V, Lantz O, Zitvogel L, Chaput N. Dendritic cell-derived exosomes for cancer immunotherapy: what’s next? Cancer Res. 2010;70:1281–1285. doi:10.1158/0008-5472.CAN-09-3276.
- Sprooten J, Ceusters J, Coosemans A, Agostinis P, De Vleeschouwer S, Zitvogel L, Kroemer G, Galluzzi L, Garg AD. Trial watch: dendritic cell vaccination for cancer immunotherapy. Oncoimmunology. 2019;8:e1638212. doi:10.1080/2162402X.2019.1638212.
- Fučíková J, Rožková D, Ulčová H, Budinský V, Sochorová K, Pokorná K, Bartůňková J, Špíšek R. Poly I: c-activated dendritic cells that were generated in CellGro for use in cancer immunotherapy trials. J Transl Med. 2011;9:223. doi:10.1186/1479-5876-9-223.
- Bercovici N, Haicheur N, Massicard S, Vernel-Pauillac F, Adotevi O, Landais D, Gorin I, Robert C, Prince HM, Grob -J-J, et al. Analysis and characterization of antitumor T-cell response after administration of dendritic cells loaded with allogeneic tumor lysate to metastatic melanoma patients. J Immunother. 2008;31:101–112. doi:10.1097/CJI.0b013e318159f5ba.
- Irvine AS, Trinder PK, Laughton DL, Ketteringham H, McDermott RH, Reid SC, Haines AM, Amir A, Husain R, Doshi R, et al. Efficient nonviral transfection of dendritic cells and their use for in vivo immunization. Nat Biotechnol. 2000;18:1273–1278. doi:10.1038/82383.
- Wan Y, Bramson J, Carter R, Graham F, Gauldie J. Dendritic cells transduced with an adenoviral vector encoding a model tumor-associated antigen for tumor vaccination. Hum Gene Ther. 1997;8:1355–1363. doi:10.1089/hum.1997.8.11-1355.
- Ishida T, Chada S, Stipanov M, Nadaf S, Ciernik FI, Gabrilovich DI, Carbone DP. Dendritic cells transduced with wild-type p53 gene elicit potent anti-tumour immune responses. Clin Exp Immunol. 1999;117:244–251. doi:10.1046/j.1365-2249.1999.00913.x.
- Celluzzi CM, Falo LD. Physical interaction between dendritic cells and tumor cells results in an immunogen that induces protective and therapeutic tumor rejection. J Immunol. 1998;160:3081–3085.
- Wang J, Saffold S, Cao X, Krauss J, Chen W. Eliciting T cell immunity against poorly immunogenic tumors by immunization with dendritic cell-tumor fusion vaccines. J Immunol. 1998;161:5516–5524.
- Orentas RJ, Schauer D, Bin Q, Johnson BD. Electrofusion of a weakly immunogenic neuroblastoma with dendritic cells produces a tumor vaccine. Cell Immunol. 2001;213:4–13. doi:10.1006/cimm.2001.1864.
- Kjaergaard J, Shimizu K, Shu S. Electrofusion of syngeneic dendritic cells and tumor generates potent therapeutic vaccine. Cell Immunol. 2003;225:65–74. doi:10.1016/j.cellimm.2003.09.005.
- Tanaka H, Shimizu K, Hayashi T, Shu S. Therapeutic immune response induced by electrofusion of dendritic and tumor cells. Cell Immunol. 2002;220:1–12. doi:10.1016/S0008-8749(03)00009-1.
- Copland MJ, Baird MA, Rades T, McKenzie JL, Becker B, Reck F, Tyler PC, Davies NM. Liposomal delivery of antigen to human dendritic cells. Vaccine. 2003;21:883–890. doi:10.1016/S0264-410X(02)00536-4.
- van Broekhoven CL, Parish CR, Demangel C, Britton WJ, Altin JG. Targeting dendritic cells with antigen-containing liposomes: a highly effective procedure for induction of antitumor immunity and for tumor immunotherapy. Cancer Res. 2004;64:4357–4365. doi:10.1158/0008-5472.CAN-04-0138.
- Badiee A, Davies N, McDonald K, Radford K, Michiue H, Hart D, Kato M. Enhanced delivery of immunoliposomes to human dendritic cells by targeting the multilectin receptor DEC-205. Vaccine. 2007;25:4757–4766. doi:10.1016/j.vaccine.2007.04.029.
- Kantoff PW, Higano CS, Shore ND, Berger ER, Small EJ, Penson DF, Redfern CH, Ferrari AC, Dreicer R, Sims RB, et al. Sipuleucel-T immunotherapy for castration-resistant prostate cancer. N Engl J Med. 2010;363:411–422. doi:10.1056/NEJMoa1001294.
- Handy CE, Antonarakis ES. Sipuleucel-T for the treatment of prostate cancer: novel insights and future directions. Future Oncol. 2018;14:907–917. doi:10.2217/fon-2017-0531.
- Dhar R, Seethy A, Singh S, Pethusamy K, Srivastava T, Talukdar J, Rath GK, Karmakar S. Cancer immunotherapy: recent advances and challenges. J Cancer Res Ther. 2021;17:834–844. doi:10.4103/jcrt.JCRT_1241_20.
- Chen S-J, Wang S-C, Chen Y-C, Romero MP. The immunotherapy for colorectal cancer, lung cancer and pancreatic cancer. Int J Mol Sci. 2021;23:22. doi:10.3390/ijms23010022.
- Vanmeerbeek I, Borras DM, Sprooten J, Bechter O, Tejpar S, Garg AD. Early memory differentiation and cell death resistance in T cells predicts melanoma response to sequential anti-CTLA4 and anti-PD1 immunotherapy. Genes Immun. 2021;22:108–119. doi:10.1038/s41435-021-00138-4.
- Hendrickson PG, Olson M, Luetkens T, Weston S, Han T, Atanackovic D, Fine GC. The promise of adoptive cellular immunotherapies in hepatocellular carcinoma. Oncoimmunology. 2020;9:1673129. doi:10.1080/2162402X.2019.1673129.
- Zhou Y, Slone N, Chrisikos TT, Kyrysyuk O, Babcock RL, Medik YB, Li HS, Kleinerman ES, and Watowich SS. Vaccine efficacy against primary and metastatic cancer with in vitro-generated CD103+ conventional dendritic cells. J Immunother Cancer. 2020 Apr;8(1): e000474. doi:10.1136/jitc-2019-000474. PMID: 32273347; PMCID: PMC7254126.
- Zhu S, Lv X, Zhang X, Li T, Zang G, Yang N, Wang X, Wu J, Chen W, Liu Y-J, et al. An effective dendritic cell-based vaccine containing glioma stem-like cell lysate and CpG adjuvant for an orthotopic mouse model of glioma. Int J Cancer. 2019;144:2867–2879. doi:10.1002/ijc.32008.
- Ashour D, Arampatzi P, Pavlovic V, Förstner KU, Kaisho T, Beilhack A, Erhard F, Lutz MB. IL-12 from endogenous cDC1, and not vaccine DC, is required for Th1 induction. JCI Insight. 2020;5. doi:10.1172/jci.insight.135143.
- Hodge J, Wang F, Wang J, Liu Q, Saaoud F, Wang Y, Singh UP, Chen H, Luo M, Ai W, et al. Overexpression of microRNA-155 enhances the efficacy of dendritic cell vaccine against breast cancer. Oncoimmunology. 2020;9:1724761. doi:10.1080/2162402X.2020.1724761.
- Park HJ, Jang G-Y, Kim YS, Park JH, Lee SE, Vo M-C, Lee -J-J, Han HD, Jung ID, Kang TH, et al. A novel TLR4 binding protein, 40S ribosomal protein S3, has potential utility as an adjuvant in a dendritic cell-based vaccine. J Immunother Cancer. 2019;7:60. doi:10.1186/s40425-019-0539-7.
- Jang G-Y, Kim YS, Lee SE, Lee JW, Han HD, Kang TH, Park Y-M. Improvement of DC-based vaccines using adjuvant TLR4-binding 60S acidic ribosomal protein P2 and immune checkpoint inhibitors. Cancer Immunol Immunother. 2021;70:1075–1088. doi:10.1007/s00262-020-02759-6.
- Dastmalchi F, Karachi A, Yang C, Azari H, Sayour EJ, Dechkovskaia A, Vlasak AL, Saia ME, Lovaton RE, Mitchell DA, et al. Sarcosine promotes trafficking of dendritic cells and improves efficacy of anti-tumor dendritic cell vaccines via CXC chemokine family signaling. J Immunother Cancer. 2019;7:321. doi:10.1186/s40425-019-0809-4.
- Liu Y, Xu P, Liu H, Fang C, Guo H, Chen X, Tan M, Zhang Y, Min W. Silencing IDO2 in dendritic cells: a novel strategy to strengthen cancer immunotherapy in a murine lung cancer model. Int J Oncol. 2020;57:587–597. doi:10.3892/ijo.2020.5073.
- Endo R, Nakamura T, Kawakami K, Sato Y, Harashima H. The silencing of indoleamine 2,3-dioxygenase 1 (IDO1) in dendritic cells by siRNA-loaded lipid nanoparticles enhances cell-based cancer immunotherapy. Sci Rep. 2019;9:11335. doi:10.1038/s41598-019-47799-w.
- Rosenblatt J, Stone RM, Uhl L, Neuberg D, Joyce R, Levine JD, Arnason J, McMasters M, Luptakova K, Jain S, et al. Individualized vaccination of AML patients in remission is associated with induction of antileukemia immunity and prolonged remissions. Sci Transl Med. 2016;8:368ra171. doi:10.1126/scitranslmed.aag1298.
- Stroopinsky D, Liegel J, Bhasin M, Cheloni G, Thomas B, Bhasin S, Panchal R, Ghiasuddin H, Rahimian M, Nahas M, et al. Leukemia vaccine overcomes limitations of checkpoint blockade by evoking clonal T cell responses in a murine acute myeloid leukemia model. Haematologica. 2021;106:1330–1342. doi:10.3324/haematol.2020.259457.
- Shi W, Yang X, Xie S, Zhong D, Lin X, Ding Z, Duan S, Mo F, Liu A, Yin S, et al. A new PD-1-specific nanobody enhances the antitumor activity of T-cells in synergy with dendritic cell vaccine. Cancer Lett. 2021;522:184–197. doi:10.1016/j.canlet.2021.09.028.
- Lau SP, van Montfoort N, Kinderman P, Lukkes M, Klaase L, van Nimwegen M, van Gulijk M, Dumas J, Mustafa DAM, and Lievense SLA, et al. Dendritic cell vaccination and CD40-agonist combination therapy licenses T cell-dependent antitumor immunity in a pancreatic carcinoma murine model. J Immunother Cancer. 2020 Jul;8(2): e000772. doi:10.1136/jitc-2020-000772. PMID: 32690771; PMCID: PMC7373331.
- Oba T, Makino K, Kajihara R, Yokoi T, Araki R, Abe M, Minderman H, Chang AE, Odunsi K, Ito F. In situ delivery of iPSC-derived dendritic cells with local radiotherapy generates systemic antitumor immunity and potentiates PD-L1 blockade in preclinical poorly immunogenic tumor models. J Immunother Cancer. 2021;9:e002432. doi:10.1136/jitc-2021-002432.
- Lapenta C, Donati S, Spadaro F, Lattanzi L, Urbani F, Macchia I, Sestili P, Spada M, Cox MC, Belardelli F, et al. Lenalidomide improves the therapeutic effect of an interferon-α-dendritic cell-based lymphoma vaccine. Cancer Immunol Immunother. 2019;68:1791–1804. doi:10.1007/s00262-019-02411-y.
- El-Ashmawy NE, Salem ML, Abd El-Fattah EE, Khedr EG. Targeting CD166+ lung cancer stem cells: molecular study using murine dendritic cell vaccine. Toxicol Appl Pharmacol. 2021;429:115699. doi:10.1016/j.taap.2021.115699.
- Guo J, Muse E, Christians AJ, Swanson SJ, Davila E. An anticancer drug cocktail of three kinase inhibitors improved response to a dendritic cell-based cancer vaccine. Cancer Immunol Res. 2019;7:1523–1534. doi:10.1158/2326-6066.CIR-18-0684.
- Butterfield LH, Vujanovic L, Santos PM, Maurer DM, Gambotto A, Lohr J, Li C, Waldman J, Chandran U, Lin Y, et al. Multiple antigen-engineered DC vaccines with or without IFNα to promote antitumor immunity in melanoma. J Immunother Cancer. 2019;7:113. doi:10.1186/s40425-019-0552-x.
- Vreeland TJ, Clifton GT, Hale DF, Chick RC, Hickerson AT, Cindass JL, Adams AM, Bohan PMK, Andtbacka RHI, Berger AC, et al. A phase iib randomized controlled trial of the TLPLDC vaccine as adjuvant therapy after surgical resection of stage III/IV melanoma: a primary analysis. Ann Surg Oncol. 2021;28:6126–6137. doi:10.1245/s10434-021-09709-1.
- De Keersmaecker B, Claerhout S, Carrasco J, Bar I, Corthals J, Wilgenhof S, Neyns B, and Thielemans K. TriMix and tumor antigen mRNA electroporated dendritic cell vaccination plus ipilimumab: link between T-cell activation and clinical responses in advanced melanoma. J Immunother Cancer. 2020 Feb;8(1): e000329. doi:10.1136/jitc-2019-000329. PMID: 32114500; PMCID: PMC7057443.
- Boudewijns S, Bloemendal M, de Haas N, Westdorp H, Bol KF, Schreibelt G, Aarntzen EHJG, Lesterhuis WJ, Gorris MAJ, Croockewit A, et al. Autologous monocyte-derived DC vaccination combined with cisplatin in stage III and IV melanoma patients: a prospective, randomized phase 2 trial. Cancer Immunol Immunother. 2020;69:477–488. doi:10.1007/s00262-019-02466-x.
- Wen PY, Reardon DA, Armstrong TS, Phuphanich S, Aiken RD, Landolfi JC, Curry WT, Zhu -J-J, Glantz M, Peereboom DM, et al. A randomized double-blind placebo-controlled phase II trial of dendritic cell vaccine ICT-107 in newly diagnosed patients with glioblastoma. Clin Cancer Res. 2019;25:5799–5807. doi:10.1158/1078-0432.CCR-19-0261.
- Mitsuya K, Akiyama Y, Iizuka A, Miyata H, Deguchi S, Hayashi N, Maeda C, Kondou R, Kanematsu A, Watanabe K, et al. Alpha-type-1 polarized dendritic cell-based vaccination in newly diagnosed high-grade glioma: a phase II clinical trial. Anticancer Res. 2020;40:6473–6484. doi:10.21873/anticanres.14669.
- Rudnick JD, Sarmiento JM, Uy B, Nuno M, Wheeler CJ, Mazer MJ, Wang H, Hu JL, Chu RM, Phuphanich S, et al. A phase I trial of surgical resection with Gliadel Wafer placement followed by vaccination with dendritic cells pulsed with tumor lysate for patients with malignant glioma. J Clin Neurosci. 2020;74:187–193. doi:10.1016/j.jocn.2020.03.006.
- Westdorp H, Creemers JHA, van Oort IM, Schreibelt G, Gorris MAJ, Mehra N, Simons M, de Goede AL, van Rossum MM, Croockewit AJ, et al. Blood-derived dendritic cell vaccinations induce immune responses that correlate with clinical outcome in patients with chemo-naive castration-resistant prostate cancer. J Immunother Cancer. 2019;7:302. doi:10.1186/s40425-019-0787-6.
- Tryggestad AMA, Axcrona K, Axcrona U, Bigalke I, Brennhovd B, Inderberg EM, Hønnåshagen TK, Skoge LJ, Solum G, Saebøe-Larssen S, et al. Long-term first-in-man Phase I/II study of an adjuvant dendritic cell vaccine in patients with high-risk prostate cancer after radical prostatectomy. Prostate. 2022;82:245–253. doi:10.1002/pros.24267.
- Cibula D, Rob L, Mallmann P, Knapp P, Klat J, Chovanec J, Minar L, Melichar B, Hein A, Kieszko D, et al. Dendritic cell-based immunotherapy (DCVAC/OvCa) combined with second-line chemotherapy in platinum-sensitive ovarian cancer (SOV02): a randomized, open-label, phase 2 trial. Gynecol Oncol. 2021;162:652–660. doi:10.1016/j.ygyno.2021.07.003.
- Block MS, Dietz AB, Gustafson MP, Kalli KR, Erskine CL, Youssef B, Vijay GV, Allred JB, Pavelko KD, Strausbauch MA, et al. Th17-inducing autologous dendritic cell vaccination promotes antigen-specific cellular and humoral immunity in ovarian cancer patients. Nat Commun. 2020;11:5173. doi:10.1038/s41467-020-18962-z.
- Bassani-Sternberg M, Digklia A, Huber F, Wagner D, Sempoux C, Stevenson BJ, Thierry A-C, Michaux J, Pak H, Racle J, et al. A phase Ib study of the combination of personalized autologous dendritic cell vaccine, aspirin, and standard of care adjuvant chemotherapy followed by nivolumab for resected pancreatic adenocarcinoma-A proof of antigen discovery feasibility in three patients. Front Immunol. 2019;10:1832. doi:10.3389/fimmu.2019.01832.
- Nagai K, Adachi T, Harada H, Eguchi S, Sugiyama H, Miyazaki Y. Dendritic Cell-based immunotherapy pulsed with Wilms tumor 1 Peptide and Mucin 1 as an adjuvant therapy for pancreatic ductal adenocarcinoma after curative resection: a phase I/IIa clinical trial. Anticancer Res. 2020;40:5765–5776. doi:10.21873/anticanres.14593.
- Wang Q-T, Nie Y, Sun S-N, Lin T, Han R-J, Jiang J, Li Z, J-q L, Xiao Y-P, Fan -Y-Y, et al. Tumor-associated antigen-based personalized dendritic cell vaccine in solid tumor patients. Cancer Immunol Immunother. 2020;69:1375–1387. doi:10.1007/s00262-020-02496-w.
- Schwarze JK, Awada G, Cras L, Tijtgat J, Forsyth R, Dufait I, Tuyaerts S, Van Riet I, Neyns B, Jarrold MF. Intratumoral combinatorial administration of CD1c (BDCA-1)+ myeloid dendritic cells plus ipilimumab and avelumab in combination with intravenous low-dose nivolumab in patients with advanced solid tumors: a phase IB clinical trial. Vaccines (Basel). 2020;9:8. doi:10.3390/vaccines9010008.
- Ramanathan R, Choudry H, Jones H, Girgis M, Gooding W, Kalinski P, Bartlett DL. Phase II trial of adjuvant dendritic cell vaccine in combination with celecoxib, interferon-α, and rintatolimod in patients undergoing cytoreductive surgery and hyperthermic intraperitoneal chemotherapy for peritoneal metastases. Ann Surg Oncol. 2021;28:4637–4646. doi:10.1245/s10434-020-09464-9.
- Ogasawara M, Miyashita M, Yamagishi Y, Ota S. Phase I/II pilot study of Wilms’ tumor 1 peptide-pulsed dendritic cell vaccination combined with conventional chemotherapy in patients with head and neck cancer. Ther Apher Dial. 2019;23:279–288. doi:10.1111/1744-9987.12831.
- Nickles E, Dharmadhikari B, Yating L, Walsh RJ, Koh LP, Poon M, Tan LK, Wang L-Z, Ang Y, Asokumaran Y, et al. Dendritic cell therapy with CD137L-DC-EBV-VAX in locally recurrent or metastatic nasopharyngeal carcinoma is safe and confers clinical benefit. Cancer Immunol Immunother. 2022;71:1531–1543. doi:10.1007/s00262-021-03075-3.
- Figlin RA, Tannir NM, Uzzo RG, Tykodi SS, Chen DYT, Master V, Kapoor A, Vaena D, Lowrance W, Bratslavsky G, et al. Results of the ADAPT phase 3 study of rocapuldencel-T in combination with sunitinib as first-line therapy in patients with metastatic renal cell carcinoma. Clin Cancer Res. 2020;26:2327–2336. doi:10.1158/1078-0432.CCR-19-2427.
- Jansen Y, Kruse V, Corthals J, Schats K, van Dam P-J, Seremet T, Heirman C, Brochez L, Kockx M, Thielemans K, et al. A randomized controlled phase II clinical trial on mRNA electroporated autologous monocyte-derived dendritic cells (TriMixDC-MEL) as adjuvant treatment for stage III/IV melanoma patients who are disease-free following the resection of macrometastases. Cancer Immunol Immunother. 2020;69:2589–2598. doi:10.1007/s00262-020-02618-4.
- Matsui HM, Hazama S, Nakajima M, Xu M, Matsukuma S, Tokumitsu Y, Shindo Y, Tomochika S, Yoshida S, Iida M, et al. Novel adjuvant dendritic cell therapy with transfection of heat-shock protein 70 messenger RNA for patients with hepatocellular carcinoma: a phase I/II prospective randomized controlled clinical trial. Cancer Immunol Immunother. 2021;70:945–957. doi:10.1007/s00262-020-02737-y.
- Zhong R, Ling X, Cao S, Xu J, Zhang B, Zhang X, Wang H, Han B, Zhong H. Safety and efficacy of dendritic cell-based immunotherapy (DCVAC/LuCa) combined with carboplatin/pemetrexed for patients with advanced non-squamous non-small-cell lung cancer without oncogenic drivers. ESMO Open. 2022;7:100334. doi:10.1016/j.esmoop.2021.100334.
- Chiappori AA, Williams CC, Gray JE, Tanvetyanon T, Haura EB, Creelan BC, Thapa R, Chen D-T, Simon GR, Bepler G, et al. Randomized-controlled phase II trial of salvage chemotherapy after immunization with a TP53-transfected dendritic cell-based vaccine (Ad.p53-DC) in patients with recurrent small cell lung cancer. Cancer Immunol Immunother. 2019;68:517–527. doi:10.1007/s00262-018-2287-9.
- Cox MC, Castiello L, Mattei M, Santodonato L, D’Agostino G, Muraro E, Martorelli D, Lapenta C, Di Napoli A, Di Landro F, et al. Clinical and antitumor immune responses in relapsed/refractory follicular lymphoma patients after intranodal injections of ifnα-dendritic cells and rituximab: a phase I clinical trial. Clin Cancer Res. 2019;25:5231–5241. doi:10.1158/1078-0432.CCR-19-0709.
- Fröbom R, Berglund E, Berglund D, Nilsson I-L, Åhlén J, von Sivers K, Linder-Stragliotto C, Suenaert P, Karlsson-Parra A, Bränström R. Phase I trial evaluating safety and efficacy of intratumorally administered inflammatory allogeneic dendritic cells (ilixadencel) in advanced gastrointestinal stromal tumors. Cancer Immunol Immunother. 2020;69:2393–2401. doi:10.1007/s00262-020-02625-5.
- Karlsson-Parra A, Kovacka J, Heimann E, Jorvid M, Zeilemaker S, Longhurst S, Suenaert P. Ilixadencel - an allogeneic cell-based anticancer immune primer for intratumoral administration. Pharm Res. 2018;35:156. doi:10.1007/s11095-018-2438-x.
- Ralli M, Botticelli A, Visconti IC, Angeletti D, Fiore M, Marchetti P, Lambiase A, de Vincentiis M, Greco A. Immunotherapy in the treatment of metastatic melanoma: current knowledge and future directions. J Immunol Res. 2020;2020:9235638. doi:10.1155/2020/9235638.
- Wilgenhof S, Van Nuffel AMT, Benteyn D, Corthals J, Aerts C, Heirman C, Van Riet I, Bonehill A, Thielemans K, Neyns B. A phase IB study on intravenous synthetic mRNA electroporated dendritic cell immunotherapy in pretreated advanced melanoma patients. Ann Oncol. 2013;24:2686–2693. doi:10.1093/annonc/mdt245.
- Shulgin B, Kosinsky Y, Omelchenko A, Chu L, Mugundu G, Aksenov S, Pimentel R, DeYulia G, Kim G, Peskov K, et al. Dose dependence of treatment-related adverse events for immune checkpoint inhibitor therapies: a model-based meta-analysis. Oncoimmunology. 2020;9:1748982. doi:10.1080/2162402X.2020.1748982.
- Lövgren T, Wolodarski M, Wickström S, Edbäck U, Wallin M, Martell E, Markland K, Blomberg P, Nyström M, Lundqvist A, et al. Complete and long-lasting clinical responses in immune checkpoint inhibitor-resistant, metastasized melanoma treated with adoptive T cell transfer combined with DC vaccination. Oncoimmunology. 2020;9:1792058. doi:10.1080/2162402X.2020.1792058.
- Hong M, Clubb JD, Chen YY. Engineering CAR-T cells for next-generation cancer therapy. Cancer Cell. 2020;38:473–488. doi:10.1016/j.ccell.2020.07.005.
- Alcantara M, Du Rusquec P, Romano E. Current clinical evidence and potential solutions to increase benefit of CAR T-cell therapy for patients with solid tumors. Oncoimmunology. 2020;9:1777064. doi:10.1080/2162402X.2020.1777064.
- Feldmann A, Hoffmann A, Bergmann R, Koristka S, Berndt N, Arndt C, Rodrigues Loureiro L, Kittel-Boselli E, Mitwasi N, Kegler A, et al. Versatile chimeric antigen receptor platform for controllable and combinatorial T cell therapy. Oncoimmunology. 2020;9:1785608. doi:10.1080/2162402X.2020.1785608.
- Li L, Zhu X, Qian Y, Yuan X, Ding Y, Hu D, He X, Wu Y. Chimeric antigen receptor T-Cell therapy in glioblastoma: current and future. Front Immunol. 2020;11:594271. doi:10.3389/fimmu.2020.594271.
- Wang L, Yao R, Zhang L, Fan C, Ma L, Liu J. Chimeric antigen receptor T cell therapy and other therapeutics for malignancies: combination and opportunity. Int Immunopharmacol. 2019;70:498–503. doi:10.1016/j.intimp.2019.01.010.
- Cha SE, Kujawski M, Yazaki J, Brown P, Shively C. Tumor regression and immunity in combination therapy with anti-CEA chimeric antigen receptor T cells and anti-CEA-IL2 immunocytokine. Oncoimmunology. 2021;10:1899469. doi:10.1080/2162402X.2021.1899469.
- Grote S, Mittelstaet J, Baden C, Chan KC-H, Seitz C, Schlegel P, Kaiser A, Handgretinger R, Schleicher S. Adapter chimeric antigen receptor (AdCAR)-engineered NK-92 cells: an off-the-shelf cellular therapeutic for universal tumor targeting. Oncoimmunology. 2020;9:1825177. doi:10.1080/2162402X.2020.1825177.
- Wang S, Wang X, Zhou X, Lyerly HK, Morse MA, Ren J. DC-CIK as a widely applicable cancer immunotherapy. Expert Opin Biol Ther. 2020;20:601–607. doi:10.1080/14712598.2020.1728250.
- Pan Q-Z, Zhao -J-J, Yang C-P, Zhou Y-Q, Lin J-Z, Tang Y, J-M G, Wang Q-J, Y-Q L, He J, et al. Efficacy of adjuvant cytokine-induced killer cell immunotherapy in patients with colorectal cancer after radical resection. Oncoimmunology. 2020;9:1752563. doi:10.1080/2162402X.2020.1752563.
- Hodgins JJ, Khan ST, Park MM, Auer RC, Ardolino M. Killers 2.0: NK cell therapies at the forefront of cancer control. J Clin Invest. 2019;129:3499–3510. doi:10.1172/JCI129338.
- Yamazaki T, Wennerberg E, Hensler M, Buqué A, Kraynak J, Fucikova J, Zhou XK, Sveinbjørnsson B, Rekdal Ø, Demaria S, et al. LTX-315-enabled, radiotherapy-boosted immunotherapeutic control of breast cancer by NK cells. Oncoimmunology. 2021;10:1962592. doi:10.1080/2162402X.2021.1962592.
- Voisin A, Grinberg-Bleyer Y. The many-sided contributions of NF-κB to T-cell biology in health and disease. Int Rev Cell Mol Biol. 2021;361:245–300.
- Tsao L-C, Force J, Hartman ZC. Mechanisms of therapeutic antitumor monoclonal antibodies. Cancer Res. 2021;81:4641–4651. doi:10.1158/0008-5472.CAN-21-1109.
- van Willigen WW, Bloemendal M, Boers-Sonderen MJ, de Groot JWB, Koornstra RHT, van der Veldt AAM, Haanen JBAG, Boudewijns S, Schreibelt G, Gerritsen WR, et al. Response and survival of metastatic melanoma patients treated with immune checkpoint inhibition for recurrent disease on adjuvant dendritic cell vaccination. Oncoimmunology. 2020;9:1738814. doi:10.1080/2162402X.2020.1738814.
- Propper DJ, Balkwill FR. Harnessing cytokines and chemokines for cancer therapy. Nat Rev Clin Oncol. 2022;19:237–253. doi:10.1038/s41571-021-00588-9.
- Berraondo P, Sanmamed MF, Ochoa MC, Etxeberria I, Aznar MA, Pérez-Gracia JL, Rodríguez-Ruiz ME, Ponz-Sarvise M, Castañón E, Melero I. Cytokines in clinical cancer immunotherapy. Br J Cancer. 2019;120:6–15. doi:10.1038/s41416-018-0328-y.
- Mongre RK, Mishra CB, Shukla AK, Prakash A, Jung S, Ashraf-Uz-Zaman M, Lee M-S. Emerging importance of tyrosine kinase inhibitors against cancer: quo vadis to cure? Int J Mol Sci. 2021;23:22.
- Hua H, Kong Q, Zhang H, Wang J, Luo T, Jiang Y. Targeting mTOR for cancer therapy. J Hematol Oncol. 2019;12:71. doi:10.1186/s13045-019-0754-1.
- Alzahrani AS. PI3K/Akt/mTOR inhibitors in cancer: at the bench and bedside. Semin Cancer Biol. 2019;59:125–132. doi:10.1016/j.semcancer.2019.07.009.
- Petrazzuolo A, Maiuri MC, Zitvogel L, Kroemer G, Kepp O. Trial Watch: combination of tyrosine kinase inhibitors (TKIs) and immunotherapy. Oncoimmunology. 2022;11:2077898. doi:10.1080/2162402X.2022.2077898.
- Petroni G, Buqué A, Zitvogel L, Kroemer G, Galluzzi L. Immunomodulation by targeted anticancer agents. Cancer Cell. 2021;39:310–345. doi:10.1016/j.ccell.2020.11.009.
- Garg AD, Coulie PG, Van den Eynde BJ, and Agostinis P. Integrating Next-Generation Dendritic Cell Vaccines into the Current Cancer Immunotherapy Landscape. Trends Immunol. 2017 Aug;38(8): 577–593. doi:10.1016/j.it.2017.05.006. Epub 2017 Jun 10. PMID: 28610825.
- Garg AD, Vara Perez M, Schaaf M, Agostinis P, Zitvogel L, Kroemer G, Galluzzi L. Trial watch: dendritic cell-based anticancer immunotherapy. Oncoimmunology. 2017;6:e1328341. doi:10.1080/2162402X.2017.1328341.
- Fucikova J, Coosemans A, Orsulic S, Cibula D, Vergote I, Galluzzi L, Spisek R. Immunological configuration of ovarian carcinoma: features and impact on disease outcome. J Immunother Cancer. 2021;9:e002873. doi:10.1136/jitc-2021-002873.
- Jiang Y-Q, Wang Z-X, Zhong M, Shen L-J, Han X, Zou X, Liu X-Y, Deng Y-N, Yang Y, Chen G-H, et al. Investigating mechanisms of response or resistance to immune checkpoint inhibitors by analyzing cell-cell communications in tumors before and after programmed cell death-1 (PD-1) targeted therapy: an integrative analysis using single-cell RNA and Bulk-RNA Sequencing Data. Oncoimmunology. 2021;10:1908010. doi:10.1080/2162402X.2021.1908010.
- Kato S, Okamura R, Kumaki Y, Ikeda S, Nikanjam M, Eskander R, Goodman A, Lee S, Glenn ST, Dressman D, et al. Expression of TIM3/Vista checkpoints and the CD68 macrophage-associated marker correlates with anti-PD1/PDL1 resistance: implications of immunogram heterogeneity. Oncoimmunology. 2020;9:1708065. doi:10.1080/2162402X.2019.1708065.
- Reardon DA, Brandes AA, Omuro A, Mulholland P, Lim M, Wick A, Baehring J, Ahluwalia MS, Roth P, Bähr O, et al. Effect of nivolumab vs bevacizumab in patients with recurrent glioblastoma: the checkmate 143 phase 3 randomized clinical trial. JAMA Oncol. 2020;6:1003–1010. doi:10.1001/jamaoncol.2020.1024.
- Lim M, Weller M, Idbaih A, Steinbach J, Finocchiaro G, Raval RR, Ansstas G, Baehring J, Taylor JW, Honnorat J, et al. Phase 3 trial of chemoradiotherapy with temozolomide plus nivolumab or placebo for newly diagnosed glioblastoma with methylated MGMT promoter. Neuro Oncol. 2022. doi:10.1093/neuonc/noac116.
- Yuan B, Wang G, Tang X, Tong A, Zhou L. Immunotherapy of glioblastoma: recent advances and future prospects. Hum Vaccin Immunother. 2022;1–16. doi:10.1080/21645515.2022.2055417.
- Vanmeerbeek I, Sprooten J, De Ruysscher D, Tejpar S, Vandenberghe P, Fucikova J, Spisek R, Zitvogel L, Kroemer G, Galluzzi L, et al. Trial watch: chemotherapy-induced immunogenic cell death in immuno-oncology. Oncoimmunology. 2020;9:1703449. doi:10.1080/2162402X.2019.1703449.
- Yang J, Eresen A, Shangguan J, Ma Q, Yaghmai V, Zhang Z. Irreversible electroporation ablation overcomes tumor-associated immunosuppression to improve the efficacy of DC vaccination in a mice model of pancreatic cancer. Oncoimmunology. 2021;10:1875638. doi:10.1080/2162402X.2021.1875638.
- Kandalaft LE, Harari A, Tzelepi V. Vaccines as priming tools for T cell therapy for epithelial cancers. Cancers (Basel). 2021;14:13. doi:10.3390/cancers14010013.
- Fucikova J, Hensler M, Kasikova L, Lanickova T, Pasulka J, Rakova J, Drozenova J, Fredriksen T, Hraska M, Hrnciarova T, et al. An autologous dendritic cell vaccine promotes anticancer immunity in ovarian cancer patients with low mutational burden and cold tumors. Clin Cancer Res. pp.OF1–OF13. 2022. doi:10.1158/1078-0432.CCR-21-4413
- Liang T, Tong W, Ma S, Chang P. Standard therapies: solutions for improving therapeutic effects of immune checkpoint inhibitors on colorectal cancer. Oncoimmunology. 2020;9:1773205. doi:10.1080/2162402X.2020.1773205.
- Vogelzang NJ, Beer TM, Gerritsen W, Oudard S, Wiechno P, Kukielka-Budny B, Samal V, Hajek J, Feyerabend S, Khoo V, et al. Efficacy and safety of autologous dendritic cell-based immunotherapy, docetaxel, and prednisone vs placebo in patients with metastatic castration-resistant prostate cancer: the VIABLE phase 3 randomized clinical trial. JAMA Oncol. 2022;8:546–552. doi:10.1001/jamaoncol.2021.7298.
- Verheye E, Bravo Melgar J, Deschoemaeker S, Raes G, Maes A, De Bruyne E, Menu E, Vanderkerken K, Laoui D, and De Veirman K. dendritic cell-based immunotherapy in multiple myeloma: challenges, opportunities, and future directions. Int J Mol Sci. 2022;23(2): 904. doi:10.3390/ijms23020904.
- Jung N-C, Lee J-H, Chung K-H, Kwak YS, Lim D-S. Dendritic cell-based immunotherapy for solid tumors. Transl Oncol. 2018;11:686–690. doi:10.1016/j.tranon.2018.03.007.
- Tesfatsion DA. Dendritic cell vaccine against leukemia: advances and perspectives. Immunotherapy. 2014;6:485–496. doi:10.2217/imt.14.12.
- Anguille S, Smits EL, Lion E, van Tendeloo VF, Berneman ZN. Clinical use of dendritic cells for cancer therapy. Lancet Oncol. 2014;15:e257–67. doi:10.1016/S1470-2045(13)70585-0.
- Constantino J, Gomes C, Falcão A, Cruz MT, Neves BM. Antitumor dendritic cell-based vaccines: lessons from 20 years of clinical trials and future perspectives. Transl Res. 2016;168:74–95. doi:10.1016/j.trsl.2015.07.008.
- Celus W, Oliveira AI, Rivis S, Van Acker HH, Landeloos E, Serneels J, Cafarello ST, Van Herck Y, Mastrantonio R, Köhler A, et al. Plexin-A4 mediates Cytotoxic T-cell trafficking and exclusion in cancer. Cancer Immunol Res. 2022;10:126–141. doi:10.1158/2326-6066.CIR-21-0061.
- Marabelle A, Kohrt H, Sagiv-Barfi I, Ajami B, Axtell RC, Zhou G, Rajapaksa R, Green MR, Torchia J, Brody J, et al. Depleting tumor-specific Tregs at a single site eradicates disseminated tumors. J Clin Invest. 2013;123:2447–2463. doi:10.1172/JCI64859.
- Wouters R, Bevers S, Riva M, De Smet F, and Coosemans A. Immunocompetent mouse models in the search for effective immunotherapy in glioblastoma. Cancers (Basel). 2020 Dec;13(1): 19. doi:10.3390/cancers13010019. PMID: 33374542; PMCID: PMC7793150.
- Vitale I, Shema E, Loi S, Galluzzi L. Intratumoral heterogeneity in cancer progression and response to immunotherapy. Nat Med. 2021;27:212–224. doi:10.1038/s41591-021-01233-9.
- Balan S, Ollion V, Colletti N, Chelbi R, Montanana-Sanchis F, Liu H, Vu Manh T-P, Sanchez C, Savoret J, Perrot I, et al. Human XCR1+ dendritic cells derived in vitro from CD34+ progenitors closely resemble blood dendritic cells, including their adjuvant responsiveness, contrary to monocyte-derived dendritic cells. J Immunol. 2014;193:1622–1635. doi:10.4049/jimmunol.1401243.
- Bol KF, Schreibelt G, Rabold K, Wculek SK, Schwarze JK, Dzionek A, Teijeira A, Kandalaft LE, Romero P, Coukos G, et al. The clinical application of cancer immunotherapy based on naturally circulating dendritic cells. J Immunother Cancer. 2019;7:109. doi:10.1186/s40425-019-0580-6.
- Crozat K, Guiton R, Guilliams M, Henri S, Baranek T, Schwartz-Cornil I, Malissen B, Dalod M. Comparative genomics as a tool to reveal functional equivalences between human and mouse dendritic cell subsets. Immunol Rev. 2010;234:177–198. doi:10.1111/j.0105-2896.2009.00868.x.
- Borst J, Ahrends T, Bąbała N, Melief CJM, Kastenmüller W. CD4+ T cell help in cancer immunology and immunotherapy. Nat Rev Immunol. 2018;18:635–647. doi:10.1038/s41577-018-0044-0.
- Saleh R, Elkord E. Acquired resistance to cancer immunotherapy: role of tumor-mediated immunosuppression. Semin Cancer Biol. 2020;65:13–27. doi:10.1016/j.semcancer.2019.07.017.
- Jackson CM, Choi J, Lim M. Mechanisms of immunotherapy resistance: lessons from glioblastoma. Nat Immunol. 2019;20:1100–1109. doi:10.1038/s41590-019-0433-y.
- Gondhowiardjo SA, Handoko JVF, Apriantoni R, Barata AR, Senoaji F, Utami IJW, Maubere F, Nuryadi E, and Giselvania A. Tackling resistance to cancer immunotherapy: what do we know? Molecules. 2020 Sep 8;25(18): 4096. doi:10.3390/molecules25184096. PMID: 32911646; PMCID: PMC7570938.
- O’Donnell JS, Teng MWL, Smyth MJ. Cancer immunoediting and resistance to T cell-based immunotherapy. Nat Rev Clin Oncol. 2019;16:151–167. doi:10.1038/s41571-018-0142-8.
- Schoenfeld AJ, Hellmann MD. Acquired resistance to immune checkpoint inhibitors. Cancer Cell. 2020;37:443–455. doi:10.1016/j.ccell.2020.03.017.
- Dhatchinamoorthy K, Colbert JD, Rock KL. Cancer immune evasion through loss of MHC class I antigen presentation. Front Immunol. 2021;12:636568. doi:10.3389/fimmu.2021.636568.
- Zhou T, Wang H-W. Antigen loss after targeted immunotherapy in hematological malignancies. Clin Lab Med. 2021;41:341–357. doi:10.1016/j.cll.2021.04.005.
- Gao Y, Chen L, Cai G, Xiong X, Wu Y, Ma D, Li SC, Gao Q. Heterogeneity of immune microenvironment in ovarian cancer and its clinical significance: a retrospective study. Oncoimmunology. 2020;9:1760067. doi:10.1080/2162402X.2020.1760067.
- Van den Eynde M, Mlecnik B, Bindea G, Galon J. Multiverse of immune microenvironment in metastatic colorectal cancer. Oncoimmunology. 2020;9:1824316. doi:10.1080/2162402X.2020.1824316.
- Sprooten J, Coosemans A, Garg AD. A first-in-class, non-invasive, immunodynamic biomarker approach for precision immuno-oncology. Oncoimmunology. 2022;11:2024692. doi:10.1080/2162402X.2021.2024692.
- Sprooten J, Vankerckhoven A, Vanmeerbeek I, Borras DM, Berckmans Y, Wouters R, Laureano RS, Baert T, Boon L, Landolfo C, et al. Peripherally-driven myeloid NFkB and IFN/ISG responses predict malignancy risk, survival, and immunotherapy regime in ovarian cancer. J Immunother Cancer. 2021;9:e003609. doi:10.1136/jitc-2021-003609.
- Chan TA, Yarchoan M, Jaffee E, Swanton C, Quezada SA, Stenzinger A, Peters S. Development of tumor mutation burden as an immunotherapy biomarker: utility for the oncology clinic. Ann Oncol. 2019;30:44–56. doi:10.1093/annonc/mdy495.
- Sha D, Jin Z, Budczies J, Kluck K, Stenzinger A, Sinicrope FA. Tumor mutational burden as a predictive biomarker in solid tumors. Cancer Discov. 2020;10:1808–1825. doi:10.1158/2159-8290.CD-20-0522.
- Klempner SJ, Fabrizio D, Bane S, Reinhart M, Peoples T, Ali SM, Sokol ES, Frampton G, Schrock AB, Anhorn R, et al. Tumor mutational burden as a predictive biomarker for response to immune checkpoint inhibitors: a review of current evidence. Oncologist. 2020;25:e147–e159. doi:10.1634/theoncologist.2019-0244.
- Patel SP, Kurzrock R. PD-L1 expression as a predictive biomarker in cancer immunotherapy. Mol Cancer Ther. 2015;14:847–856. doi:10.1158/1535-7163.MCT-14-0983.
- Doroshow DB, Bhalla S, Beasley MB, Sholl LM, Kerr KM, Gnjatic S, Wistuba II, Rimm DL, Tsao MS, Hirsch FR. PD-L1 as a biomarker of response to immune-checkpoint inhibitors. Nat Rev Clin Oncol. 2021;18:345–362. doi:10.1038/s41571-021-00473-5.
- Zouein J, Kesrouani C, Kourie HR. PD-L1 expression as a predictive biomarker for immune checkpoint inhibitors: between a dream and a nightmare. Immunotherapy. 2021;13:1053–1065. doi:10.2217/imt-2020-0336.
- de Coaña Y P, Wolodarski M, van der Haar Àvila I, Nakajima T, Rentouli S, Lundqvist A, Masucci G, Hansson J, Kiessling R. PD-1 checkpoint blockade in advanced melanoma patients: NK cells, monocytic subsets and host PD-L1 expression as predictive biomarker candidates. Oncoimmunology. 2020;9:1786888. doi:10.1080/2162402X.2020.1786888.
- Evrard D, Hourseau M, Couvelard A, Paradis V, Gauthier H, Raymond E, Halimi C, Barry B, Faivre S. PD-L1 expression in the microenvironment and the response to checkpoint inhibitors in head and neck squamous cell carcinoma. Oncoimmunology. 2020;9:1844403. doi:10.1080/2162402X.2020.1844403.
- Naulaerts S, Borras DM, Martinez AA, Messiaen J, Van Herck Y, Gelens L, Venken T, Vanmeerbeek I, More S, and Sprooten J, et al. Immunogenomic, single-cell and spatial dissection of CD8+T cell exhaustion reveals critical determinants of cancer immunotherapy. BioRxiv. 2021. doi:10.1101/2021.11.22.468617.
- Vaes RDW, Reynders K, Sprooten J, Nevola KT, Rouschop KMA, Vooijs M, Garg AD, Lambrecht M, Hendriks LEL, Rucevic M, et al. Identification of potential prognostic and predictive immunological biomarkers in patients with stage I and Stage III Non-Small Cell Lung Cancer (NSCLC): a prospective exploratory study. Cancers (Basel). 2021;14:13.
- Peng J, Zou D, Gong W, Kang S, Han L. Deep neural network classification based on somatic mutations potentially predicts clinical benefit of immune checkpoint blockade in lung adenocarcinoma. Oncoimmunology. 2020;9:1734156. doi:10.1080/2162402X.2020.1734156.
- Guo L, Wang X, Wang S, Hua L, Song N, Hu B, Tong Z. Efficacy of immune-checkpoint inhibitors in PD-L1 selected or unselected patients vs. control group in patients with advanced or metastatic urothelial carcinoma. Oncoimmunology. 2021;10:1887551. doi:10.1080/2162402X.2021.1887551.