Abstract
HIV vaccine strategies are expected to be a crucial component for controlling the HIV epidemic. Despite the large spectrum of potential candidate vaccines for both prophylactic and therapeutic use, the overall development process of an efficacious HIV vaccine strategy is lengthy. The design of clinical trials and the progression of a candidate strategy through the different clinical development stages remain methodologically challenging, mainly due to the lack of validated correlates of protection. In this review, we describe recent advances in clinical trial designs to increase the efficiency of the clinical development of candidate HIV vaccine strategies. The methodological aspects of the designs for early- (phase I and II) and later –stage (phase IIB and III) development are discussed, taking into account the specificities of both prophylactic and therapeutic HIV vaccine development.
Background
Despite considerable advances in HIV prevention and treatment, HIV infection still remains a threatening infectious disease with important public health consequences. To control the HIV epidemic, 2 principal issues would need to be resolved: i) efficacious and feasible large-scale measures for HIV prevention, and ii) strategies for a cure from infection. To achieve each of these aims, complex integrated strategies may be required, of which HIV vaccines (prophylactic vaccines for the first issue and therapeutic vaccines for the second one) are expected to be crucial components.Citation1
No prophylactic or therapeutic HIV vaccine strategies with satisfactory efficacy are available to date, but the research field has been encouraged by the first report of a protective vaccine effect against HIV in 2009. Results of a randomized phase IIB trial in 16402 healthy volunteers in Thailand (RV144 trial), evaluating a combination of 2 HIV candidate vaccines in a prime-boost strategy against placebo, showed promising results with a reduction of HIV acquisition in the active vaccine arm.Citation2 However, estimated vaccine efficacy was modest (approximately 30%) and seemed to wane over time.Citation2-4
In therapeutic approaches, the efficacy of vaccine strategies to control viral replication or to reduce the viral reservoir has been evaluated in proof-of-concept trials with varying results (reviewed in Citation5,6).
To disentangle the results of the RV144 trial and to improve the efficacy of HIV candidate vaccines, research is currently very active, with a multitude of potential vaccine strategies under development for both prophylactic and therapeutic applications. Candidate vaccines against HIV include protein or sub-unit vaccines, recombinant viral vector, deoxyribonucleic acid (DNA) and dendritic-cell based vaccines.Citation7 As the results of the RV144 trial suggest that heterologous prime-boost strategies are a promising approach, the number of strategies to be evaluated is potentially large.
Despite this spectrum of candidate vaccines, the development of efficacious HIV vaccine strategies remains a major scientific challenge. In addition to aspects related to viral diversity and escape, HIV vaccine development is complicated as there is limited knowledge about the potential immunological correlates of clinical vaccine efficacy, that is protection from infection or post-infection viral load in the case of a prophylactic strategy or virological control or stable disease without antiretroviral treatment in the case of a therapeutic strategy. This means that it is not yet possible to predict these clinical outcomes based on immunogenicity markers. The overall development process is lengthy, and it is difficult to correctly appreciate the worthiness of a new HIV vaccine strategy before the first clinical efficacy trials.Citation8 This has resulted in only 6 prophylactic HIV vaccine efficacy trials implemented so far, with the phase IIB RV144 trial in Thailand mentioned above being the only trial with a promising result. The remaining trials showed disappointing results with no evidence for a protective vaccine effect ().
Table 1. Overview of phase IIB and III trials of prophylactic HIV vaccine strategies
Results of an exploratory case-control analysis nested in the RV144 trial suggested that the modest clinical efficacy of the prime-boost strategy under evaluation may have been mediated by humoral responses,Citation9 but cellular responses may also contribute to this association.Citation10 Despite the encouraging results of the RV144 trial, it is, however, premature to conclude which components of cellular or humoral immunity should be generated by a HIV vaccine strategy. Moreover, it is likely that the immunological correlates of HIV vaccine efficacy will be different for prophylactic vaccine strategies versus therapeutic vaccine strategies.
Principles of Clinical Development in HIV Vaccine Research
HIV vaccine development currently relies on iterative loops between animal studies and clinical studies in humans. Phase I and II clinical trials are conducted to determine the safety and to assess the immunogenicity of the vaccine strategies in early clinical development. As correlates of protection are not well known to date, a large number of immunogenicity markers are measured. This implies a considerable uncertainty about the interpretation of immunogenicity results and the potential value of a vaccine strategy as it proceeds through early clinical development. Immunogenicity results in early clinical development stages in humans often entail a return to the animal model to optimize tested strategies.
To obtain preliminary evidence based on a clinical endpoint (that is HIV acquisition or post-infection viremia), HIV vaccine development nowadays usually includes phase IIB test-of-concept trials before deciding whether further clinical efficacy testing is reasonable.Citation11 In contrast to large phase III trials, phase IIB trials do not aim at directly supporting a licensure decision. Endpoint definitions and allowable type I and II error rates may be different between phase IIB and III trials. In particular, in test-of-concept trials higher false positive rates (type I error) and lower false negative rates (type II error) than in confirmatory trials may be desirable in order to increase the probability to detect a vaccine effect.Citation11,12 However, even these phase IIB trials typically require a large sample size with several thousand participants and prolonged follow-up.Citation2,13,14 If results of a phase IIB trial are promising enough, phase III trials are conducted to confirm clinical vaccine efficacy with stringent methods aiming at licensure requests.
To evaluate potential surrogate markers the first step is to assess correlates of risk, i.e. immunological markers that correlate with the clinical endpoint of interest.Citation15-17 Evaluation of correlates of risk requires variability in the marker and the clinical endpoint, but not necessarily a protective vaccine effect. Correlates of risk can thus be analyzed in various datasets, such as observational data from HIV controllers,Citation18-20 single arm vaccine trials or randomized trials.
Once correlates of risks are identified, they can be evaluated with regards to their value as correlates of protection, which implies their evaluation as surrogate endpoints. Plotkin and Gilbert have defined a correlate of protection as an immune marker statistically associated with clinical vaccine efficacy.Citation15 In other words, a correlate of protection must capture the vaccine effect on the clinical endpoint. This definition incorporates “surrogate endpoints” in the terminology used by Prentice.Citation21 For the analysis of a surrogate endpoint, one needs data sets of randomized phase IIB or III trials having demonstrated a protective vaccine effect.
As RV144 is the only trial having shown a modest protective effect of a prophylactic HIV vaccine strategy so far, this dataset constitutes a unique opportunity to search for correlates of protection for prophylactic HIV vaccine strategies,Citation2,9 although vaccine studies in non-human primates may also contribute to this search.Citation22,23
The sample size of the RV144 trial (16402 participants) and the trial's timelines, with approximately 10 y between trial planning and the publication of the main correlate results also illustrate the efforts required to conduct prophylactic HIV vaccine efficacy trials.Citation24
Trial Designs to Accelerate Clinical Development of HIV Vaccine Strategies
A relevant impetus for rethinking HIV vaccine trial designs today is the demand to accelerate the clinical development of candidate vaccine strategies. In the methodological literature numerous clinical trial designs exist that allow for increased efficiency, by reducing sample size or allowing more rapid decision making within the clinical development plan than with classical designs, while maintaining desired operating characteristics and trial validity. Many of these designs have been in longstanding use in fields other than HIV vaccine research, especially in cancer research.Citation25-27
Multi-arm trials have more than 2 arms, which can increase efficiency in terms of sample size, for example by comparing more than one active vaccine arm to a common control arm or by using factorial designs ().
Figure 1. Schematic illustration of different trial designs. (A) Multi-arm design, Example of a design, comparing 3 different vaccine strategies to a common placebo arm. (B) Two-by-2 factorial 4-arm design. Example of a design evaluating a vaccine strategy, another intervention and the combination of both. The other intervention could for instance be pre-exposure prophylaxis (PrEP) in case of a HIV prevention trial or a drug to mobilize the viral reservoir in case of a therapeutic trial. (C) Two-arm group-sequential design Example of a design with one interim analysis and stopping rule (D) Seamless adaptive design. Example of an adaptive design with a seamless progression between phase II and phase III, integrating a selection of trial arms during phase II and additional design adaptations at the beginning of phase III.
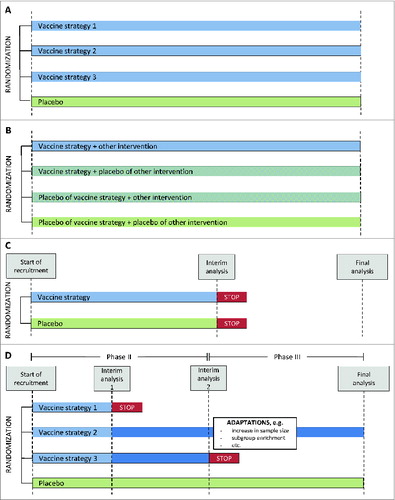
Group-sequential or sequential trials allow for stopping the trial early in case of efficacy, futility (that is lack of efficacy), or harm. This requires interim analyses, appropriate statistical methods to define the stopping rules, and review by an Independent Data Monitoring Committee.Citation28 Recent prophylactic HIV vaccine efficacy trials used such designs, which resulted in the STEP and HVTN 505 trials, respectively, being stopped for futility.Citation13,29
More sophisticated adaptive designs accommodate greater flexibility for modifications during trial conduct while controlling bias and the rates of erroneous conclusions, in particular the type I error rate.Citation30 Design modifications in adaptive trials are prospectively planned and based on analyses of data accumulated during the trial and can concern different adaptations, such as selecting a treatment dose, stopping or adding a trial arm, enriching a participant sub-group, modifying the sample size or changing the random allocation ratio.Citation31 Seamless adaptive designs allow for combining several development phases in one single trial. Well-defined endpoints but also extensive simulation studies are usually necessary to set up complex adaptive designs with adequate operating characteristics.Citation32 The conduct of such trials also requires thorough planning of all operational aspects, for example the practical implementation of the design modifications in the trial's logistics and the timing of unblinding. As is also the case in group-sequential trials, a proficient Independent Data Monitoring Committee plays a key role in the rigorous conduct of adaptive designs. Development of adaptive designs is currently a vast field of statistical research with a multitude of methods proposed in the recent literature, although the scientific and ethical challenges of such trials are also subject to debate,Citation33,34 and acceptability by regulatory authorities may need upfront consultations.
To accelerate the clinical development of efficacious prophylactic HIV vaccine strategies several authors have recently called for the use of adaptive trial designs.Citation8,24,35,36 As most adaptive designs existing in the literature have been devised for other indications, mainly oncology trials, thorough methodological consideration is needed before being adapted to HIV vaccine research. Many differences distinguish cancer and HIV vaccine research, including but not limited to the acceptable toxicity rates of interventions and the rates of clinical efficacy endpoints.
Designs for Clinical Efficacy Vaccine Trials
The only adaptive design in the literature developed for HIV vaccine trials relates to a multi-arm 2-stage phase IIB design, in which adaptive decisions during the trial are based on a clinical HIV acquisition endpoint.Citation12 This design allows for evaluating the efficacy of several prophylactic vaccine strategies in parallel with a common placebo arm. Interim analyses monitor futility of vaccine efficacy compared to placebo and aim at stopping inefficacious strategies during the first stage, that is in the first 18 months of follow-up. For all strategies that are not stopped, adaptations in the proposed design include i) the assessment of durability of vaccine efficacy in the second stage, ii) preparations to analyze correlates of protection in a timely manner, and iii) head-to-head comparisons of promising vaccine strategies. Furthermore, interim analyses are planned to monitor for high efficacy, albeit it is unlikely that this type of stopping criterion be met with the currently developed HIV vaccine strategies, as well as continuous monitoring of harm. This design has thus many desirable features to accelerate HIV vaccine efficacy trials, but it remains yet to be implemented in practice.
Specific methods for correlate discovery called “augmented designs” have been developed independently from adaptive trial designs, but can be combined with the latter. Such augmentations aim at deducing immune responses to the vaccine in placebo recipients, which are required for the statistical evaluation of surrogate endpoints in a counterfactual model. This can be achieved by imputing the non-observed immune response using baseline variables as predictors (baseline immunogenicity predictor approach) and/or administrating the vaccine to a sample of placebo recipients at the end of the trial (close-out placebo vaccination).Citation37
To evaluate prophylactic HIV vaccine strategies together with other available partially effective prevention measures, such as pre-exposure prophylaxis (PrEP), several types of multi-arm efficacy trial designs have been proposed in the literature.Citation38,39 These include a 2-by-2 factorial 4-arm design, with a first group receiving a combination of the vaccine and the other prevention measure, a second and third group receiving either the vaccine or the prevention measure, respectively, and the relevant placebo, and the fourth group receiving double placebo, which allows to assess not only the efficacy of each intervention but also possible synergies if the trial is adequately powered. Design options accommodating for the willingness of the participants to adhere to the non-vaccine prevention measure also exist, performing randomization in 2 stages. In the first stage, all participants are randomized to either vaccine or placebo. In the second stage, use of the other prevention measure is randomized only for those being willing. However, this increases the required sample sizes.Citation39 Designs including only a subset of the above mentioned arms also exist (reviewed in Citation40). Some of these multi-arm designs could also be used should a non-inferiority hypothesis of a vaccine strategy alone compared to the combination strategy become relevant.Citation39 How to best perform group-sequential monitoring, or even adaptive modifications, in these combination trials requires further consideration.
Therapeutic HIV vaccine efficacy trials require their own specific methodological considerations. Availability of highly effective antiretroviral treatment as standard of care raises questions about the acceptability and risk-benefit ratio of analytical treatment interruption to assess the vaccine effect on virological control in proof-of-concept trials.Citation41 Whether virological control or disease progression events should be the primary endpoint in confirmatory trials would also need scrutiny. The level of intracellular HIV DNA, which is a measure of the 'reservoir' of the latently infected cells, could also be considered as an endpoint. As therapeutic HIV vaccine development is currently mainly in early development stages, to our knowledge, few specific methodological papers related to efficacy trials have been published to date.
Immunogenicity Assessments in Early Stage Clinical HIV Vaccine Development
In early stage clinical HIV vaccine development, that is phase I and II trials, the main limitation is currently the lack of validated surrogate endpoints that could be used to reliably explore vaccine efficacy based on immunogenicity markers. As adaptive designs require a clear consensual endpoint definition for progression between stages, application of such designs to early clinical development stages in HIV vaccine research is difficult. Nevertheless, phase II immunogenicity trials could benefit from other features of design optimizations but few authors have so far addressed design considerations in early stage HIV vaccine trials beyond endpoint definitions.
Randomized multi-arm trials are valuable in early stage trials to allow for an unbiased assessment of the safety and immunogenicity of several candidate vaccine strategies in parallel, with the standardized immunogenicity assays and endpoint definitions across arms. For instance, a non-comparative multi-arm phase IB selection design can be used to screen several strategies and prioritize among them for further development the most promising one.Citation42 In this type of design, the arms are not compared directly to each other by statistical testing but are ranked based on a binary immunogenicity endpoint, and the arm(s) with the top-rank is/are selected. This allows for having adequate statistical power for selecting the strategy with the highest immunological response rate of the defined endpoint, while keeping the sample size reasonable for a phase II trial, usually comprising between 20 and 50 participants per arm. A minimum required response rate of the selected strategy can also be incorporated in the design.
Another type of non-comparative multi-arm design aims at moving all strategies with immunogenicity response rate above such a minimum required rate forward to the next development stage. The minimum required rate is defined a priori, and the one-sided 95% confidence interval of the observed response rate in a trial arm must lie above this minimal rate in order to consider it promising. Such a design has recently been proposed in the literature for a phase II prophylactic HIV vaccine trial of 4 heterologous prime-boost strategies. Furthermore, as one of the vaccines to be studied in this design also required a phase I evaluation, the possibility to integrate a phase I safety stage in one arm of the randomized phase II design has been established (). This is achieved by a sequential analyses of an early safety endpoint of the concerned vaccine with the aim to stop the vaccine before the boost phase should it be unsafe.Citation43 The applicability of the integrated phase I evaluation relies on prior knowledge of the safety of similar vaccines and quick participant accrual in the trial.
Figure 2. Illustration of a multi-arm phase I-II design evaluating 4 vaccine strategies in parallel and integrating an early safety decision rule for one of the vaccines (vaccine 1).
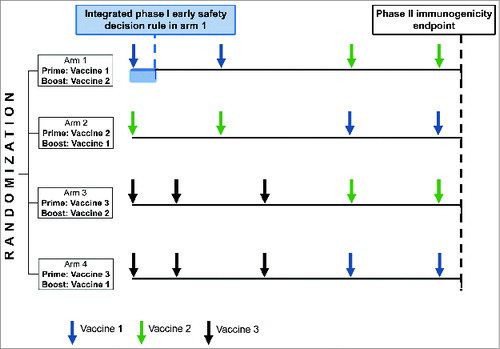
After such non-comparative trials, a randomized comparative phase II design can be set up as the next development step with direct statistical head-to-head comparisons of immunogenicity between strategies. Inclusion of a placebo arm is debatable in early stage prophylactic HIV vaccine trials in low-risk populations, where HIV-specific immune responses can be considered close to zero in the absence of exposure or vaccination for assays with high specificity. As a vaccine strategy proceeds further through clinical development stages, a randomized placebo arm will become important for head-to-head comparisons.
In the absence of validated correlates of protection, endpoint definitions in immunogenicity trials are subject to more or less empirical choices. Several authors have put forward statistical methods for the creation of binary endpoints from IFN-γ ELISpot and intracellular cytokine staining data,Citation44-48 but the use of binary endpoints is subject to debate.Citation49 Analysis methods of quantitative immunogenicity variables have been proposed for IFN-γ ELISpot data and multiplex bead array assays,Citation50,51 and summary measures have also been developed for multivariate broadly neutralizing antibody data.Citation52
Nevertheless, all currently established designs for early stage HIV vaccine trials, be they comparative or not, rely on the definition of a single immunogenicity primary endpoint.Citation43 Secondary immunogenicity endpoints thus play an important role in these designs.
The definition of appropriate statistical analysis methods is crucial for HIV vaccine immunogenicity trials, which are characterized by a relatively small sample size and a broad spectrum of assessed immunogenicity markers. In particular, the analysis of markers of HIV-specific cellular response is intricate due to their multidimensionality (numerous markers assessed in different stimulation conditions). The statistical analysis of multidimensional data requires careful considerations related to the large numbers of variables measured and the correlations between different markers. This also implies questions regarding the adjustment for test multiplicity and the use of analysis methods allowing to integrate and summarize the immune responses across different markers, such as statistical methods for dimension-reduction and systems biology approaches.Citation53
For the early stage development of therapeutic HIV vaccine strategies, additional aspects need to be taken into account. As these strategies are administered to HIV-infected persons, virological endpoints are assessable in addition to immunogenicity endpoints from early development on. Although these are not validated surrogates for clinical endpoints, HIV RNA or DNA can be measured. If HIV RNA is measured after short-term antiretroviral treatment interruption, methodological consideration is required for defining a standardized viral load endpoint. Viral load set point, maximum viral load, area under the curve of viral load, time to rebound etc. are examples of endpoint definitions, the potential clinical relevance of which needs to be discussed. The underlying assumption of the vaccine effect varies according to the chosen endpoint. For instance, establishing an immune response that delays the start of the viral rebound is not the same as limiting the maximum observed value of viral load.Citation54 The handling of patients having restarted antiretroviral treatment before measurement of the endpoint is critical, as these could correspond to the ones with the weakest viro-immunological control. Randomized placebo groups are also important from early development on, as HIV-specific immune responses exist prior to trial entry and regardless of vaccination in HIV-infected participants. The integrative analysis of the data in therapeutic HIV vaccine research requires the consideration of viral parameters in addition to the immunological measures.Citation5 A recently published example used several statistical approaches for the dimension-reduction in a phase I therapeutic HIV vaccine trial.Citation54
Definition of Dose and Schedules in HIV Vaccine Trials
In first-in-human phase I trials the safety of different doses of a single vaccine or of a combination is often assessed in a dose-escalation design. In contrast to phase I trials of therapeutic drugs, the estimation of the maximum tolerated dose is likely not the relevant paradigm in phase I trials of vaccines, as dose-toxicity and dose-immunogenicity relationships may not both be sigmoid shaped. The low expected rate of vaccine-related toxicity events requires a trade-off between risk minimization, commensurability and statistical operating characteristics. Ten participants per dose level have been recommended in vaccine dose escalation trials,Citation42,55 and the number of potential doses is often limited. More than one safe dose level can be selected after phase I dose escalation and be moved forward to randomized phase II trials to search for the optimal biologically active and safe dose.
However, dose finding and selection of candidate vaccines is only one aspect when defining a complex vaccine strategy, and it is likely that the timing of the repeated vaccine injections also plays a role. As the large number of possible injection schedules makes it prohibitive to test them all in clinical trials, the timing has often been defined empirically. Recently, mathematical models have been used to predict the effect of an exogenous IL-7 immunotherapy on CD4 T-cells in HIV infected persons and to define the number of treatment cycles to be tested in future trials of this therapy.Citation56 HIV vaccine research could also benefit from such modeling to narrow down the best time points for vaccine injections and also for immunogenicity assessments. These so-called in-silico trials have already been proposed in other areas.Citation57,58
Conclusion
Despite the large number of potential candidate strategies, the selection of HIV vaccine strategies for clinical efficacy testing remains a major methodological challenge, related to the lack of validated correlates of protection and the considerable human and financial resources required for efficacy trials. Although several clinical trials designs have recently been proposed to make vaccine evaluation more efficient at different development stages, the progression from phase II immunogenicity trials to phase IIB efficacy trials remains the most critical point. Decision criteria for this progression can currently not be based on a clear surrogate marker but take into account a large spectrum of immunogenicity variables and are thus implicitly multivariate. Standardisation of various immune assays as well as the use of statistical methods for multivariate data are thus relevant areas for progress in decision making in HIV vaccine development in the absence of well established surrogate immunogenicity markers.
Disclosure of Potential Conflicts of Interest
No potential conflicts of interest were disclosed.
References
- Fauci AS, Folkers GK, Marston HD. Ending the Global HIV/AIDS Pandemic: The Critical Role of an HIV Vaccine. Clin Infect Dis 2014; 59 Suppl 2: S80-4; PMID:25151483; http://dx.doi.org/10.1093/cid/ciu420.
- Rerks-Ngarm S, Pitisuttithum P, Nitayaphan S, Kaewkungwal J, Chiu J, Paris R, Premsri N, Namwat C, de Souza M, Adams E, et al. Vaccination with ALVAC and AIDSVAX to prevent HIV-1 infection in Thailand. N Engl J Med 2009; 361(23): 2209-20; PMID:19843557; http://dx.doi.org/10.1056/NEJMoa0908492.
- Gilbert PB, Berger JO, Stablein D, Becker S, Essex M, Hammer SM, Kim JH, Degruttola VG. Statistical interpretation of the RV144 HIV vaccine efficacy trial in Thailand: a case study for statistical issues in efficacy trials. J Infect Dis 2011; 203(7): 969-75; PMID:21402548; http://dx.doi.org/10.1093/infdis/jiq152.
- Robb ML, Rerks-Ngarm S, Nitayaphan S, Pitisuttithum P, Kaewkungwal J, Kunasol P, Khamboonruang C, Thongcharoen P, Morgan P, Benenson M, et al. Risk behaviour and time as covariates for efficacy of the HIV vaccine regimen ALVAC-HIV (vCP1521) and AIDSVAX B/E: a post-hoc analysis of the Thai phase 3 efficacy trial RV 144. Lancet Infect Dis 2012; 12(7): 531-7; PMID:22652344; http://dx.doi.org/10.1016/S1473-3099(12)70088-9.
- Pantaleo G, Levy Y. Vaccine and immunotherapeutic interventions. Curr Opin HIV AIDS 2013; 8(3): 236-42; PMID:23478912; http://dx.doi.org/10.1097/COH.0b013e32835fd5cd.
- Vanham G, Van Gulck E. Can immunotherapy be useful as a “functional cure” for infection with Human Immunodeficiency Virus-1? Retrovirology 2012; 9: 72; PMID:22958464; http://dx.doi.org/10.1186/1742-4690-9-72.
- O'Connell RJ, Kim JH, Corey L, Michael NL. Human immunodeficiency virus vaccine trials. Cold Spring Harb Perspect Med 2012; 2(12): a007351; PMID:23209178; http://dx.doi.org/10.1101/cshperspect.a007351.
- Koup RA, Graham BS, Douek DC. The quest for a T cell-based immune correlate of protection against HIV: a story of trials and errors. Nat Rev Immunol 2011; 11(1): 65-70; PMID:21164527; http://dx.doi.org/10.1038/nri2890.
- Haynes BF, Gilbert PB, McElrath MJ, Zolla-Pazner S, Tomaras GD, Alam SM, Evans DT, Montefiori DC, Karnasuta C, Sutthent R, et al. Immune-correlates analysis of an HIV-1 vaccine efficacy trial. N Engl J Med 2012; 366(14): 1275-86; PMID:22475592; http://dx.doi.org/10.1056/NEJMoa1113425.
- Gartland AJ, Li S, McNevin J, Tomaras GD, Gottardo R, Janes H, Fong Y, Morris D, Geraghty DE, Kijak GH, et al. Analysis of HLA A*02 Association with Vaccine Efficacy in the RV144 HIV-1 Vaccine Trial. J Virol 2014; 88(15): 8242-55; PMID:24829343; http://dx.doi.org/10.1128/JVI.01164-14.
- Gilbert PB. Some design issues in phase 2B vs phase 3 prevention trials for testing efficacy of products or concepts. Stat Med 2010; 29(10): 1061-71; PMID:20419758; http://dx.doi.org/10.1002/sim.3676.
- Gilbert PB, Grove D, Gabriel E, Huang Y, Gray G, Hammer SM, Buchbinder SP, Kublin J, Corey L, Self SG. A sequential phase 2b trial design for evaluating vaccine efficacy and immune correlates for multiple HIV vaccine regimens. Stat Commun Infect Dis 2011; 3(1):1037; PMID:23181167.
- Buchbinder SP, Mehrotra DV, Duerr A, Fitzgerald DW, Mogg R, Li D, Gilbert PB, Lama JR, Marmor M, Del Rio C, et al. Efficacy assessment of a cell-mediated immunity HIV-1 vaccine (the Step Study): a double-blind, randomised, placebo-controlled, test-of-concept trial. Lancet 2008; 372(9653): 1881-93; PMID:19012954; http://dx.doi.org/10.1016/S0140-6736(08)61591-3.
- Gray GE, Allen M, Moodie Z, Churchyard G, Bekker LG, Nchabeleng M, Mlisana K, Metch B, de Bruyn G, Latka MH, et al. Safety and efficacy of the HVTN 503/Phambili study of a clade-B-based HIV-1 vaccine in South Africa: a double-blind, randomised, placebo-controlled test-of-concept phase 2b study. Lancet Infect Dis 2011; 11(7): 507-15; PMID:21570355; http://dx.doi.org/10.1016/S1473-3099(11)70098-6.
- Plotkin SA, Gilbert PB. Nomenclature for immune correlates of protection after vaccination. Clin Infect Dis 2012; 54(11): 1615-7; PMID:22437237; http://dx.doi.org/10.1093/cid/cis238.
- Qin L, Gilbert PB, Corey L, McElrath MJ, Self SG. A framework for assessing immunological correlates of protection in vaccine trials. J Infect Dis 2007; 196(9): 1304-12; PMID:17922394; http://dx.doi.org/10.1086/522428.
- Gilbert PB, Qin L, Self SG. Evaluating a surrogate endpoint at three levels, with application to vaccine development. Stat Med 2008; 27(23): 4758-78; PMID:17979212; http://dx.doi.org/10.1002/sim.3122.
- Hua S, Lecuroux C, Saez-Cirion A, Pancino G, Girault I, Versmisse P, Boufassa F, Taulera O, Sinet M, Lambotte O, et al. Potential role for HIV-specific CD38-/HLA-DR+ CD8+ T cells in viral suppression and cytotoxicity in HIV controllers. PloS one 2014; 9(7): e101920; PMID:25000587; http://dx.doi.org/10.1371/journal.pone.0101920.
- Saez-Cirion A, Lacabaratz C, Lambotte O, Versmisse P, Urrutia A, Boufassa F, Barré-Sinoussi F, Delfraissy JF, Sinet M, Pancino G, et al. HIV controllers exhibit potent CD8 T cell capacity to suppress HIV infection ex vivo and peculiar cytotoxic T lymphocyte activation phenotype. Proc Natl Acad Sci USA 2007; 104(16): 6776-81; PMID:17428922; http://dx.doi.org/10.1073/pnas.0611244104.
- Potter SJ, Lacabaratz C, Lambotte O, Perez-Patrigeon S, Vingert B, Sinet M, Colle JH, Urrutia A, Scott-Algara D, Boufassa F, et al. Preserved central memory and activated effector memory CD4+ T-cell subsets in human immunodeficiency virus controllers: an ANRS EP36 study. J Virol 2007; 81(24): 13904-15; PMID:17928341; http://dx.doi.org/10.1128/JVI.01401-07.
- Prentice RL. Surrogate endpoints in clinical trials: definition and operational criteria. Stat Med 1989; 8(4): 431-40; PMID:2727467; http://dx.doi.org/10.1002/sim.4780080407.
- Barouch DH, Liu J, Li H, Maxfield LF, Abbink P, Lynch DM, Iampietro MJ, SanMiguel A, Seaman MS, Ferrari G, et al. Vaccine protection against acquisition of neutralization-resistant SIV challenges in rhesus monkeys. Nature 2012; 482(7383): 89-93; PMID:22217938; http://dx.doi.org/10.1038/nature10766.
- Hansen SG, Vieville C, Whizin N, Coyne-Johnson L, Siess DC, Drummond DD, Legasse AW, Axthelm MK, Oswald K, Trubey CM, et al. Effector memory T cell responses are associated with protection of rhesus monkeys from mucosal simian immunodeficiency virus challenge. Nat Med 2009; 15(3): 293-9; PMID:19219024; http://dx.doi.org/10.1038/nm.1935.
- Burgers WA, Manrique A, Masopust D, McKinnon LR, Reynolds MR, Rolland M, Blish C, Chege GK, Curran R, Fischer W, et al. Measurements of immune responses for establishing correlates of vaccine protection against HIV. AIDS Res Hum Retroviruses 2012; 28(7): 641-8; PMID:21861777; http://dx.doi.org/10.1089/aid.2011.0239.
- Berry DA. Adaptive clinical trials in oncology. Nat Rev Clin Oncol 2012; 9(4): 199-207; http://dx.doi.org/10.1038/nrclinonc.2011.165.
- Brown SR, Gregory WM, Twelves CJ, Buyse M, Collinson F, Parmar M, Seymour MT, Brown JM, et al. Designing phase II trials in cancer: a systematic review and guidance. Br J Cancer 2011; 105(2): 194-9; PMID:21712822; http://dx.doi.org/10.1038/bjc.2011.235.
- Todd S. A 25-year review of sequential methodology in clinical studies. Stat Med 2007; 26(2): 237-52; PMID:17139691; http://dx.doi.org/10.1002/sim.2763.
- Jennison C, Turnbull BW. Group Sequential Methods with Applications to Clinical Trials. Boca Raton: Chapman & Hall/CRC; 2000.
- Hammer SM, Sobieszczyk ME, Janes H, Karuna ST, Mulligan MJ, Grove D, Koblin BA, Buchbinder SP, Keefer MC, Tomaras GD, et al. Efficacy Trial of a DNA/rAd5 HIV-1 Preventive Vaccine. N Engl J Med 2013; 369(22): 2083-92; PMID:24099601; http://dx.doi.org/10.1056/NEJMoa1310566.
- Commitee for medicinal products for human use (CHMP). Draft reflection paper on methodological issues in confirmatory clinical trials with flexible design and analysis plan. London, UK: European Medicines Agency; 2006. http://www.ema.europa.eu/docs/en_GB/document_library/Scientific_guideline/2009/09/WC500003617.pdf, last accessed March 4, 2015.
- Kairalla JA, Coffey CS, Thomann MA, Muller KE. Adaptive trial designs: a review of barriers and opportunities. Trials 2012; 13: 145; PMID:22917111; http://dx.doi.org/10.1186/1745-6215-13-145.
- US. Department of Health and Human Services. Draft guidance for industry: adaptive design clinical trials for drugs and biologics. Silver Spring, Maryland: Food and Drug Administration (FDA); 2010. http://www.fda.gov/downloads/Drugs/Guidances/ucm201790.pdf, last accessed March 4, 2015.
- Meurer WJ, Lewis RJ, Berry DA. Adaptive clinical trials: a partial remedy for the therapeutic misconception? JAMA 2012; 307(22): 2377-8; PMID:22692168; http://dx.doi.org/10.1001/jama.2012.4174.
- van der Graaf R, Roes KC, van Delden JJ. Adaptive trials in clinical research: scientific and ethical issues to consider. JAMA 2012; 307(22): 2379-80; PMID:22692169; http://dx.doi.org/10.1001/jama.2012.6380.
- Corey L, Nabel GJ, Dieffenbach C, Gilbert P, Haynes BF, Johnston M, Kublin J, Lane HC, Pantaleo G, Picker LJ, et al. HIV-1 vaccines and adaptive trial designs. Sci Transl Med 2011; 3(79): 79ps13; PMID:21508308; http://dx.doi.org/10.1126/scitranslmed.3001863.
- Nabel GJ. Designing tomorrow's vaccines. N Engl J Med 2013; 368(6): 551-60; PMID:23388006; http://dx.doi.org/10.1056/NEJMra1204186.
- Follmann D. Augmented designs to assess immune response in vaccine trials. Biometrics 2006; 62(4): 1161-9; PMID:17156291; http://dx.doi.org/10.1111/j.1541-0420.2006.00569.x.
- Excler JL, Rida W, Priddy F, Gilmour J, McDermott AB, Kamali A, Anzala O, Mutua G, Sanders EJ, Koff W, et al. AIDS vaccines and preexposure prophylaxis: is synergy possible? AIDS Res Hum Retroviruses 2011; 27(6): 669-80; PMID:21043994; http://dx.doi.org/10.1089/aid.2010.0206.
- Janes H, Gilbert P, Buchbinder S, Kublin J, Sobieszczyk ME, Hammer SM. In pursuit of an HIV vaccine: designing efficacy trials in the context of partially effective nonvaccine prevention modalities. AIDS Res Hum Retroviruses 2013; 29(11): 1513-23; PMID:23597282; http://dx.doi.org/10.1089/aid.2012.0385.
- Moodie Z, Janes H, Huang Y. New clinical trial designs for HIV vaccine evaluation. Curr Opin HIV AIDS 2013; 8(5): 437-42; PMID:23872613; http://dx.doi.org/10.1097/COH.0b013e328363d46a.
- Eyal N, Kuritzkes DR. Challenges in clinical trial design for HIV-1 cure research. Lancet 2013; 382(9903): 1464-5; PMID:24182529; http://dx.doi.org/10.1016/S0140-6736(13)62040-1.
- Moodie Z, Rossini AJ, Hudgens MG, Gilbert PB, Self SG, Russell ND. Statistical evaluation of HIV vaccines in early clinical trials. Contemp Clin Trials 2006; 27(2): 147-60; PMID:16426900; http://dx.doi.org/10.1016/j.cct.2005.11.008.
- Richert L, Doussau A, Lelievre JD, Arnold V, Rieux V, Bouakane A, Lévy Y, Chêne G, Thiébaut R; Vaccine Research Institute (VRI). Accelerating clinical development of HIV vaccine strategies: methodological challenges and considerations in constructing an optimised multi-arm phase I/II trial design. Trials 2014; 15: 68; PMID:24571662; http://dx.doi.org/10.1186/1745-6215-15-68.
- Horton H, Thomas EP, Stucky JA, Frank I, Moodie Z, Huang Y, Chiu YL, McElrath MJ, De Rosa SC. Optimization and validation of an 8-color intracellular cytokine staining (ICS) assay to quantify antigen-specific T cells induced by vaccination. J Immunol Methods 2007; 323(1): 39-54; PMID:17451739; http://dx.doi.org/10.1016/j.jim.2007.03.002.
- Hudgens MG, Self SG, Chiu YL, Russell ND, Horton H, McElrath MJ. Statistical considerations for the design and analysis of the ELISpot assay in HIV-1 vaccine trials. J Immunol Methods 2004; 288(1-2): 19-34; PMID:15183082; http://dx.doi.org/10.1016/j.jim.2004.01.018.
- Moodie Z, Huang Y, Gu L, Hural J, Self SG. Statistical positivity criteria for the analysis of ELISpot assay data in HIV-1 vaccine trials. J Immunol Methods 2006; 315(1-2): 121-32; PMID:16959262; http://dx.doi.org/10.1016/j.jim.2006.07.015.
- Alexander N, Fox A, Lien VT, Dong T, Lee LY, Hang Nle K, Mai le Q, Horby P. Defining ELISpot cut-offs from unreplicated test and control wells. J Immunol Methods 2013; 392(1-2): 57-62; PMID:23500146; http://dx.doi.org/10.1016/j.jim.2013.02.014.
- Finak G, McDavid A, Chattopadhyay P, Dominguez M, De Rosa S, Roederer M, Gottardo R. Mixture models for single-cell assays with applications to vaccine studies. Biostatistics 2014; 15(1): 87-101; PMID:23887981; http://dx.doi.org/10.1093/biostatistics/kxt024.
- Richert L, Thiebaut R. Is the current use of 'positivity' thresholds meaningful for evaluating HIV-vaccine immunogenicity endpoints? AIDS 2013; 27(8): 1362-5; PMID:23759712; http://dx.doi.org/10.1097/QAD.0b013e328360d52e.
- Gilbert PB, Sato A, Sun X, Mehrotra DV. Efficient and robust method for comparing the immunogenicity of candidate vaccines in randomized clinical trials. Vaccine 2009; 27(3): 396-401; PMID:19022314; http://dx.doi.org/10.1016/j.vaccine.2008.10.083.
- Fong Y, Wakefield J, De Rosa S, Frahm N. A robust bayesian random effects model for nonlinear calibration problems. Biometrics 2012; 68(4): 1103-12; PMID:22551415; http://dx.doi.org/10.1111/j.1541-0420.2012.01762.x.
- Huang Y, Gilbert PB, Montefiori DC, Self SG. Simultaneous Evaluation of the Magnitude and Breadth of a Left and Right Censored Multivariate Response, with Application to HIV Vaccine Development. Stat Biopharm Res 2009; 1(1): 81-91; PMID:20072667; http://dx.doi.org/10.1198/sbr.2009.0008.
- De Gregorio E, Rappuoli R. From empiricism to rational design: a personal perspective of the evolution of vaccine development. Nat Rev Immunol 2014; 14(7): 505-14; PMID:24925139; http://dx.doi.org/10.1038/nri3694.
- Levy Y, Thiebaut R, Montes M, Lacabaratz C, Sloan L, King B, Pérusat S, Harrod C, Cobb A, Roberts LK, et al. Dendritic cell-based therapeutic vaccine elicits polyfunctional HIV-specific T-cell immunity associated with control of viral load. Eur J Immunol 2014; 44(9):2802-10; PMID: 25042008; Epub ahead of print.
- Saul A. Models of Phase 1 vaccine trials: optimization of trial design to minimize risks of multiple serious adverse events. Vaccine 2005; 23(23): 3068-75; PMID:15811654; http://dx.doi.org/10.1016/j.vaccine.2004.10.048.
- Thiebaut R, Drylewicz J, Prague M, Lacabaratz C, Beq S, Jarne A, Croughs T, Sekaly RP, Lederman MM, Sereti I, et al. Quantifying and predicting the effect of exogenous interleukin-7 on CD4+ T cells in HIV-1 infection. PLoS Comput Biol 2014; 10(5): e1003630; PMID:24853554; http://dx.doi.org/10.1371/journal.pcbi.1003630.
- Magni L, Raimondo DM, Bossi L, Man CD, De Nicolao G, Kovatchev B, Cobelli C. Model predictive control of type 1 diabetes: an in silico trial. J Diabetes Sci Technol 2007; 1(6): 804-12; PMID:19885152; http://dx.doi.org/10.1177/193229680700100603.
- Roelofs E, Engelsman M, Rasch C, Persoon L, Qamhiyeh S, de Ruysscher D, Verhaegen F, Pijls-Johannesma M, Lambin P; ROCOCO Consortium. Results of a multicentric in silico clinical trial (ROCOCO): comparing radiotherapy with photons and protons for non-small cell lung cancer. J Thorac Oncol 2012; 7(1): 165-76; PMID:22071782; http://dx.doi.org/10.1097/JTO.0b013e31823529fc.
- Pitisuttithum P, Gilbert P, Gurwith M, Heyward W, Martin M, van Griensven F, Hu D, Tappero JW, Choopanya K; Bangkok Vaccine Evaluation Group. Randomized, double-blind, placebo-controlled efficacy trial of a bivalent recombinant glycoprotein 120 HIV-1 vaccine among injection drug users in Bangkok, Thailand. J Infect Dis 2006; 194(12): 1661-71; PMID:17109337; http://dx.doi.org/10.1086/508748.
- Flynn NM, Forthal DN, Harro CD, Judson FN, Mayer KH, Para MF. Placebo-controlled phase 3 trial of a recombinant glycoprotein 120 vaccine to prevent HIV-1 infection. J Infect Dis 2005; 191(5): 654-65; PMID:15688278; http://dx.doi.org/10.1086/428404.