Abstract
Respiratory syncytial virus (RSV) is a major pathogen in infants and the elderly, causing pneumonia and bronchiolitis. Despite decades of research, to date there is still no approved RSV vaccine available. In this study, we developed RSV virus-like particle (VLP) vaccines containing an RSV fusion (F) and/or attachment (G) protein with Newcastle disease virus (NDV) as the platform. The VLPs were expressed in a baculovirus system and purified by sucrose gradient centrifugation. BALB/c mice immunized intranasally (i.n.) with rNDV/RSV/F plus rNDV/RSV/G developed robust humoral, mucosal RSV-specific antibodies and cellular immune responses. Furthermore, rNDV/RSV/F plus rNDV/RSV/G provided better protection than did rNDV/RSV/F or rNDV/RSV/G alone, as shown by an obvious decrease in viral replication together with alleviation of histopathological changes in the lungs of the challenged mice. Our data demonstrate that the intranasal vaccination of combined RSV virus-like particle vaccine candidates has great potential for protection against RSV infection.
Introduction
Respiratory syncytial virus (RSV) is the leading cause of respiratory disease in infants and the elderly throughout the world. More than 70% of children infected with RSV in their first year of life have epithelial desquamation, neutrophilic bronchiolitis and pneumonia, and obstructive pulmonary disease.Citation1,2 Despite the various RSV vaccine candidates developed over the past 60 years, no vaccine is available currently. A formalin-inactivated RSV vaccine (FI-RSV), the first produced for patients, failed to protect against the virus, resulting in 2 deaths. The failure was attributed to the imbalance between Th1 and Th2 responses and the destruction of key antibody-neutralizing determinants.Citation3 To overcome such difficulties, several live-attenuated vaccine candidates have been generated and evaluated clinically.Citation4 However, it is very challenging to balance over-attenuation and under-attenuation to develop a safe yet effective vaccine.
Virus-like particle (VLP) vaccines, namely, the papillomavirus and hepatitis B virus vaccines5, have been licensed for use in humans. VLPs mimic viral morphology but lack nucleic acids; thus, their use limits the risk of live virus vaccine replication and recombination. Therefore, respiratory syncytial VLPs represent a promising approach to responding to the threat of infection. However, some studies have reported that the efficiency of RSV VLP release is significantly low.Citation6 Furthermore, it has been suggested that Newcastle disease virus (NDV) VLPs could serve as a platform for the expression of RSV proteins, resulting in NDV VLPs emerging as an effective RSV vaccine.Citation6,7 In addition, using NDV as a backbone rarely induces pre-existing immune responses in humans. Previous studies report that Cervarix, baculovirus-expressed papillomavirus VLP, has been approved and its approval is a milestone for acceptance of baculovirus as a platform for human vaccine.Citation8 In addition Cervarix vaccine contains HPV-16 and HPV-18 virus-like particles (VLPs) that are produced using the recombinant baculovirus.Citation9 Of note, since papillomavirus is non-enveloped and NDV is enveloped, these VLPs are not equivalent. We believe that NDV is the most promising backbone.
The F and G surface proteins of RSV are the major antigenic determinants of protection. The conserved F glycoprotein induces specific cytotoxic lymphocytes, transforming a primarily CD4+ and CD8+ cell T-cell response into a Th1 immune response.Citation10,11 On the other hand, the G protein elicits a Th-2-dominant immune response.Citation12
RSV infection typically initiates at the mucosal surface. There are 2 lines of defense against viruses in the mammalian respiratory tract: secretory IgA (sIgA) prevents viral infection, and serum IgG mediates protection by neutralizing the virus. A classical systemic intramuscular vaccine induces serum IgG antibodies, causing transudation in the lower respiratory tract to neutralize the virus.Citation13 In contrast, mucosal immunization evokes a better local mucosal immune response and induces local IgA productionCitation14-16, neutralizing the virus where the pathogen enters the host organism, thereby avoiding manifestation and dissemination. Mucosal immunization also stimulates systemic antibody secretion. Moreover, mucosal vaccination does not require needles and causes less pain, so there is a high acceptance of these vaccines among children.Citation17 In the current study, we used the NDV platform to develop RSV VLP vaccines containing F and/or G protein. The immune response and protective immunity against RSV were then investigated in BALB/c mice immunized intranasally (i.n.) with rNDV/RSV/F and rNDV/RSV/G particles in combination or alone.
Results
Construction and expression of rNDV/RSV/F and rNDV/RSV/G
The preparation of rNDV/RSV/F for use as an immunogen was accomplished by transfection with NDV NP and M and the RSV F cDNAs. Because individual cDNAs could not be used to produce sufficient VLPs, the fused construct was generated. To improve the transfection of rNDV/RSV/F, the RSV F gene was incorporated into the NDV genes order of NP and M to create a recombinant plasmid. The SV40 and polyhedron promoter at the junctions among the 3 genes is depicted shown in . Similarly, the RSV G protein gene was fused to the NDV genes to create rNDV/RSV/G; the SV40 and polyhedron promoter at junctions among the 3 genes are shown in . As shown in , the 2 recombinant VLPs were expressed in SF9 cells. The molecular weight of the F protein expressed from rNDV/RSV/F was 56 KD similar to that from the WT. Interestingly, the G protein expressed from rNDV/RSV/G was 60 KD, as indicated by Western blot. This finding is consistent with inefficient glycosylation.Citation18 Both of the NDV proteins in VLPs were 40 KD and 51 KD. The results demonstrated that the total proteins of VLPs were expressed properly. Moreover, NDV VLPs contains the envelope protein gp64 ().
Characteristics of VLPs
VLPs were released from cells transfected with the rNDV/RSV/F and rNDV/RSV/G plasmids (). Electron microscopy analysis of the purified VLPs revealed the morphologies of rNDV/RSV/F and rNDV/RSV/G. Both VLPs were spherical in shape with spikes on the surface. The peak of the size distribution of rNDV/RSV/F was 91–110 nm, whereas that of rNDV/RSV/G was 71–110 nm and was more heterogeneous in size. The sizes of the VLPs were consistent with that of WT RSV A2.
These data indicates that rNDV/RSV/F and rNDV/RSV/G possessed virus like morphologies and contained the antigens of interest.
Serum antibody immunity to VLPs
The levels of RSV-specific IgG antibodies in serum from i.n. immunized BALB/c mice were determined by ELISA. The highest serum IgG titer was approximately 10,000 in the rNDV/RSV/F+G group after boost. As shown in , the highest IgG1 and IgG2a titers were also seen in the rNDV/RSV/F+G group. We also used the NDV M, NP VLPs to determine the immune response to the NDV VLPs alone by ELISA (data not shown here). We found that serum possessed antibodies to NDV. The data of the 3 groups were obtained and indicated that rNDV/RSV/F+G could induce antibody response.
Figure 1. Construction of recombinant virus-like particles encoding RSV F or G and NDV M and NP, and the characteristics of rNDV/RSV/F and rNDV/RSV/G, confirmed by Western blotting and electron microscopy. The shapes and sizes of the virus-like particles were visualized. (A) A schematic of the recombinant segments of virus-like particles. The recombinant region encoding RSV F or G and NDV M and NP were connected via specific sequences, including SV40 and Pph. Blank boxes represent the NDV backbone and target protein, and lattice boxes indicate the non-translated region. Arrows indicate the connection sites. The numbers indicate the nucleotide positions of the connection sites. (B) Expression of rNDV/RSV/F and rNDV/RSV/G analyzed by Western blots. (C, D) Electron microscopy revealed the spherical shape (C) and peak size distribution (91–110 nm) (D) of the rNDV/RSV/F particles. (E, F) The spherical shape (E) and peak size distribution (71–110 nm) of the rNDV/RSV/G particles were also revealed by electron microscopy.
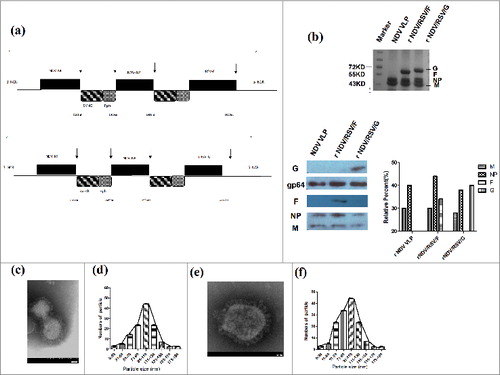
Figure 2. Female BALB/c mice vaccinated i.n. with rNDV/RSV/F, rNDV/RSV/G, or rNDV/RSV/F+G showed induced RSV-specific antibody responses. The levels of RSV-specific antibodies IgG (A), IgG1 (B) and IgG2a (C) in serum were assessed via ELISA using inactivated RSV A2. (D) The ratios of IgG1/IgG2a were determined for the 3 immunization groups and the PBS control group. The values represent the means ± SEM from 6 mice. *P < 0.05 and **P < 0.01.
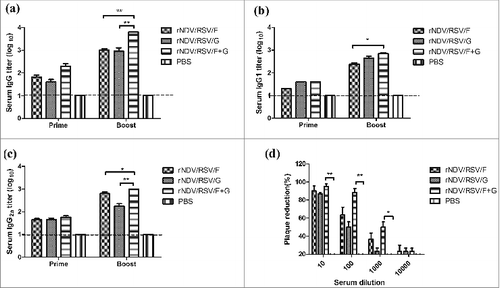
Neutralizing antibody responses
In order to detect whether neutralizing antibodies mice immunized with VLPs, the plaque reduction in vitro assay was used. As shown in , at the 1:10 serum dilutions, rNDV/RSV/G, rNDV/RSV/F and rNDV/RSV/F+G group reduced the number of plaques by 80%, while the PBS group caused no reduction. At the 1:100 serum dilutions, rNDV/RSV/F+G still reduced the number of plaques by 80% while rNDV/RSV/F and rNDV/RSV/G group reduced the number of plaques by 50–60%. We concluded that the rNDV/RSV/F, rNDV/RSV/G and rNDV/RSV/F+G VLPs could induce protective antibodies to neutralize RSV.
Cellular immunity to VLPs
ELISPOT analysis was performed to estimate the ability of VLPs to elicit cellular responses. The results demonstrated that rNDV/RSV/F+G induced a 1.7 fold higher level of IFN-γ and IL-4 than did the other groups. As illustrated in , the number of IFN-γ-secreting cells was greater than that of IL-4-secreting cells in the rNDV/RSV/F+G and rNDV/RSV/F groups. In contrast, the number of IFN-γ-secreting cells was less than that of IL-4-secreting cells in the rNDV/RSV/G group. These results suggest that the Th1-biased response was greater than that of the Th2-biased response in the rNDV/RSV/F+G and rNDV/RSV/F groups.
Figure 3. Analysis of IFN-γ and IL-4 by ELISPOT. Mice were immunized i.n. twice at a 2-week interval with rNDV/RSV/F, rNDV/RSV/G and rNDV/RSV/F+G. On day 14 after the second immunization, 6 mice in each group were sacrificed, and single-cell suspensions were prepared from the spleens. (A) IFN-γ and (B) IL-4 secretions by splenic lymphocytes were detected using ELISPOT after 48 h of culture. The data represent the means ± SEM. *P < 0.05 and **P < 0.01.
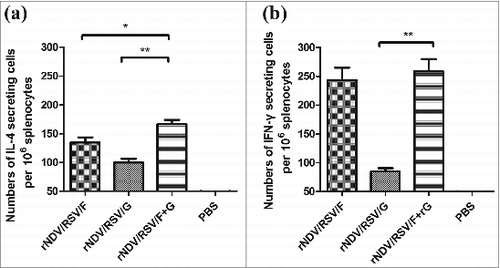
Mucosal immunity of VLPs
The sIgA levels in lung and nasal extracts of mice immunized with VLPs were determined by ELISA. As shown in , we found that the sIgA titer was higher in lung extract than in nasal extract. The lung sIgA titer of the rNDV/RSV/F group was 100, similar to that of the rNDV/RSV/F+G group. The highest nasal extract sIgA titer was approximately 100 in the rNDV/RSV/F+G group. These results showed that intranasal administration could efficiently induce mucosal antibody responses.
Figure 4. The mucosal antibody response in immunized BALB/c mice. sIgA antibodies against inactivated RSV were confirmed by ELISA in nasal (A) and lung extracts (B) from mice immunized i.n. with rNDV/RSV/F, rNDV/RSV/G, or rNDV/RSV/F+G. Nasal and lung extract samples (n = 6) were collected on day 14 after the boost. The values represent means ± SEM. *P < 0.05 and **P < 0.01.
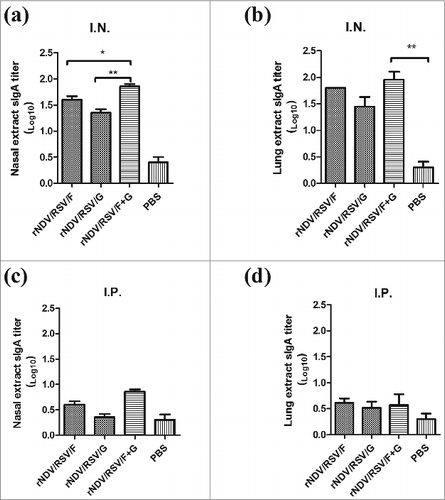
Figure 5. Protection of VLPs in BALB/c mice challenged with RSV strain A2. Groups of mice (n = 16) were immunized i.n. with rNDV/RSV/F, rNDV/RSV/G, or rNDV/RSV/F+G and then challenged with RSV A2 (1.5 × 106 PFU) by intranasal inoculation on day 14 after boost. (A) Mice were measured for body weight changes over a 2-week period. (B) The nasal extracts and lungs of 6 mice per group among the 3 vaccination and control groups were collected on day 6 post-challenge. Viral loads in tissue homogenates were determined by the plaque assay using HEp-2 cells. *P < 0.05 and **P < 0.01.
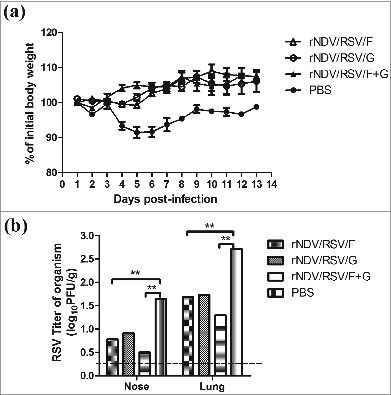
Figure 6. Attenuation of virus-induced lung pathology. After boost immunization with rNDV/RSV/F, rNDV/RSV/G, or rNDV/RSV/F+G, BALB/c mice were challenged i.n. with RSV (1.5 × 106 PFU). Six mice were sacrificed on day 6 after challenge, and lung samples were collected. Histological examination of lung tissues was performed using H&E staining: (A) Normal, (B) rNDV/RSV/F, (C) rNDV/RSV/G, (D) rNDV/RSV/F+G, and (E) PBS. (F) The numbers of inflammatory cells were counted among 100 fields within pathological sections.
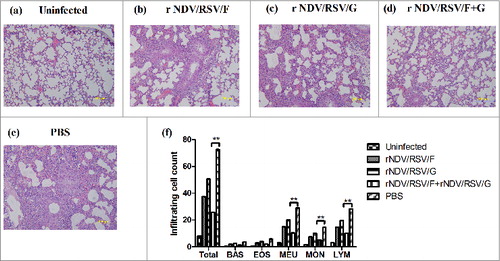
Protection of mice from RSV infection after intranasal vaccination with VLPs
The weights of the vaccinated mice were measured following challenge with 1.5 × 106 RSV A2 PFU/mouse. The lungs and noses of vaccinated mice were sampled on day 6 post-challenge to determine the viral titer. The initial body weight ratio had decreased on the first day after challenge in the vaccination groups (); however, it increased quickly from the second day post-challenge. Of note, the weight ratio showed a significant difference between the vaccination groups and the control group on day 6; whereas, no significant differences in body weight change were observed among the 3 vaccination groups. The mean viral titers were about 101.5 PFU per mouse in the rNDV/RSV/F+G group and 101.7 PFU per mouse for the rNDV/RSV/F and rNDV/RSV/G groups. There was a significant decrease greater than 50% in RSV viral titers in the lung tissue of all vaccinated groups compared with the PBS group. Furthermore, the nasal viral titer in all vaccinated groups was <10 PFU/mouse, which showed a decrease compared with the control group (). These results indicate that rNDV/RSV/F+G efficiently limited the replication of live RSV infection in respiratory tract.
Lung pathology after live RSV challenge
To observe histopathological lung damage, lung tissues were obtained on day 6 post-challenge; pathological sections stained with H&E are shown in . Compared with normal mouse lung, the PBS-immunized mice after challenge showed thickening and fragmentation of the alveolar wall and severe edema, and even infiltration of a large number of inflammatory cells (). In contrast, BALB/c mice immunized with rNDV/RSV/F+G showed light histopathological damage: thinning of the alveolar wall and decreased inflammatory cells in tissues (). Despite the fact that mice in the rNDV/RSV/F or rNDV/RSV/G group showed only mild inflammatory changes (), more severe histopathological damage was observed when compared with the rNDV/RSV/F+G group. Furthermore, the number of inflammatory cells was counted among 100 fields within pathological sections. There was a significant reduction inflammatory cell in vaccine group compared with PBS group (). Above data revealed that rNDV/RSV/F+G could induce protective immunity against live RSV infection efficiently in lung tissues.
Discussion
The WHO estimates that ∼2 million pneumonia deaths occur each year in children less than 5 y of age, mainly in Africa and South-East Asia.Citation19 RSV is considered to be an important pathogen of pediatric pneumonia and bronchiolitis. A broad range of strategies have promoted RSV vaccine development, including live-attenuated RSV, plasmid DNACitation20, and scaffold-based vaccines.Citation21 However, to date no licensed vaccine has been shown to provide efficacy as well as safe, long-term protection against RSV infection due to insufficient immunogenicity and vaccine-enhanced diseases. RSV fusion (F) and attachment (G) glycoproteins possess the majority of neutralizing and T cell epitopes. F and G proteins are recognized as the major targets for designing RSV vaccines. Virus-like particles containing RSV F and G proteins could induce protection against RSV infection without enhancing diseases due to Th-1 dominant response.Citation6,7
We focused on RSV VLPs using a NDV backbone,Citation22 because NDV shows highly efficient release and rare pre-immunity. In our study, RSV F or G proteins, fused to an NDV backbone composed of NP and M protein (rNDV/RSV/F or rNDV/RSV/G), were generated in a baculovirus expression system. Others have generated particles expressing RSV F or G fused with the transmembrane and cytoplasmic domins of other viral proteins that are the structural foundation of the particle. Although we expressed full-length F and G which contain all the neutralizing and several T-cell epitopes of RSV, the contribution of the F and G transmembrane and cytoplasmic regions to immunogenicity is unclear. Compared with other expression systems which were used to express VLPs, such as prokaryotic, yeast, and eukaryotic systems, the baculovirus system is easier for high expression of foreign genes. To construct the recombinant plasmid rNDV/RSV/F, we redesigned the target sequences combining 3 sequences (NDV NP, NDV M and RSV F); similarly, rNDV/RSV/G contained 3 sequences (NDV NP, NDV M and RSV G) end-to-end. Compared with the co-expression of the 3 proteins in mammalian cells, the recombinant sequences not only simplified the plasmid construction but also improved the efficiency of transfection. VLPs with spherical particle shapes were observed by electron microscopy. The diameter size distribution of rNDV/RSV/F was mainly in the range of 91–110 nm, and that of rNDV/RSV/G was 71–110 nm. In addition, we found that insect cell infected with recombinant Baculovirus containing F develop syncytial formation, suggesting that F protein cased syncytial formation the same as it does in mammalian cells infected with wild-type RSV. Furthermore, we compared the abundance of NDV M and NP and RSV F or G in the Baculovirus infected cells to their abundance in purified particles in order to determine the purification of the VLPs. We confirmed that the abundance of VLPs relative proteins are 85% in purified particles, while the abundance of VLPs relative proteins are 20–30% in the Baculovirus infected cells (data not shown).
We compared the quantification of M, NP, F and G proteins in the 2 VLP preparations to NDV and RSV. There are the same relative abundance of M, NP and F in the VLP preparation to NDV and RSV. However, we determined that RSV G protein was 60KD (), suggesting insufficient glycosylation in insect cells. Moreover, in the F+G preparation, the F-to-G ration was similar to that seen in wild-type RSV. Mucosal immunity played a critical role in protecting the epithelium of the nasal cavity from respiratory viral infections.Citation23 RSV infects mucosal surfaces and induces mucosal immunity in conjunction with a systemic immune response, strong humoral and cellular immune responses, to protect against infection.Citation24 Intranasal delivery allows induction of specific and functional antibody responses against RSV in the respiratory tract. Further, sIgA is the major antibody isotype in mucosal secretions that provides protective immunity against upper respiratory tract infections.Citation25 We focused on the protective mucosal immunity of a VLP RSV vaccine after intranasal immunization in BALB/c mice. Compared with the rNDV/RSV/F or rNDV/RSV/G group, BALB/c mice immunized with rNDV/RSV/F+G achieved 1.4 fold higher antibody responses and induced immunity more efficiently.
The failure of the FI-RSV vaccine was attributed to a Th2-like immune response.Citation3 Accordingly, it is necessary to maintain the Th1/Th2 response balance in protective immunity. We measured the levels of IgG, IgG2a and IgG1 RSV-specific antibodies after each immunization in mice to assess the immunogenic capabilities of recombinant VLPs. The IgG1/IgG2a serum ratio indicated that rNDV/RSV/F, as well as rNDV/RSV/F+G, triggers a Th1-based immune response. Particularly, the mice immunized i.n. with recombinant VLPs produced antibodies in mucosal secretions from nose and lung. sIgA has been detected previously in mucosal secretions. Compared with the rNDV/RSV/F+G group immunized i.p., the group immunized i.n. developed higher sIgA titers (data not shown).
Therefore, we believe that the intranasal immunization route could enhance mucosal and systemic immunity responses to clear the virus and confer resistance to infection. The PBS-vaccinated mice infected with RSV displayed a significant increase in viral loads in the nose and lung, and at the same time, their weight declined significantly during the observation period. In comparison, recombined VLP-vaccinated mice exhibited a lower viral load and mild histopathological lung damage, along with a significant increase in body weight on day 6 post-challenge. Among the 3 vaccine groups, the lowest viral titer (101.5 PFU/mouse) was observed in the rNDV/RSV/F+G group. BALB/c mice immunized with rNDV/RSV/F or rNDV/RSV/G showed mild inflammatory changes but more severe histopathological damage when compared with the rNDV/RSV/F+G group. Thus, the most effective RSV clearance was observed in BALB/c mice immunized i.n. with rNDV/RSV/F+G on day 6 post-challenge, with minimal pathological findings associated with RSV infection.
Overall, we generated Baculovirus-produced NDV VLPs containing full-length RSV F or G protein. And the rNDV/RSV/F+G intranasal vaccine conferred the best protection against RSV in BALB/c mice. We therefore conclude that rNDV/RSV/F+G is a promising vaccine candidate. However, due to the animal models limitation, we only detected efficiency and protection of rNDV/RSV/F+G in BALB/c mice. Future studies should confirm the function of the F and G transmembrane and cytoplasmic regions to immunogenicity; the immunity protection in cotton rat or monkey models; as well as the long-lasting immune response of the rNDV/RSV/F+G vaccine in mice.
Materials and Methods
Cells and viruses
HEp-2 cells were infected with the A2 strain of wild-type RSV. After freeze-thawing, the infected cell pellets were centrifuged at 1,600 g for 20 min at 4°C; the supernatants were titrated by plaque assay and then stored at −70°C. HEp-2 cells were cultured in DMEM/F12 (1:1) medium (GIBCO) containing 2% fetal bovine serum (FBS) (GIBCO) at 37°C with 5% CO2. The original virus and HEp-2 cells were obtained from the American Type Culture Collection (ATCC). SF9 cells were maintained in serum-free SF900 II medium (GIBCO) at 27°C in flasks with shaking at 120 rpm.
Construction of recombinant plasmids
Codon-optimized RSV F, RSV G, NDV M and NDV NP protein genes were introduced into baculovirus using the Bac-to-Bac system (Invitrogen). The specific sequences including SV40 and Pph were attached to RSV F and NDV NP protein genes, respectively. The constructed rNDV/RSV/F gene contained RSV F protein, SV40, Pph, NDV M protein, SV40, Pph and NP protein genes. The constructed rNDV/RSV/G gene contained RSV G protein, SV40, Pph, NDV M protein, SV40, Pph and NP protein genes. rNDV/RSV/F and rNDV/RSV/G were synthetic by Sangon (Shanghai). The synthesized target sequences were cloned into pFastbacI via the XhoI and BamHI sites within the rNDV/RSV/F and rNDV/RSV/G plasmids. The specific primers used for detection were as follows: rNDV/RSV/F, forward, 5′-TATTGGATCCATGGACTCCTCCCGTACCAT-3′, reverse 5′- GTGGAAGCTTTACATCTTGGTGGTAGCACG-3′; rNDV/RSV/F, forward, 5′-GGATCCATGGACTCCTCCCGTACCATCGGTC-3′, reverse 5′-GGGTACTCGGAGGTGG-TGGACACCTGG-3′ Recombinant bacmids were extracted using the PureLinkTM HIPure Plasmid Midiprep Kit (Invitrogen).
Generation of recombinant baculoviruses
Second-passage SF9 cells were seeded into 6-cell plates and cultured as cell monolayers. The transfection of recombinant bacmid DNA was performed according to the manufacturer's instructions (Invitrogen). Recombinant baculoviruses were harvested from cell culture medium 72 h post-transfection.
Production and purification of VLPs
At day 4 post-infection, cell supernatants were collected by centrifugation at 5,500×g. VLPs in the supernatants were concentrated by centrifugation at 28,000×g. The concentrate was resuspended in PBS. The VLPs were laid on top of a discontinuous sucrose gradient composed of 2-ml layers of 20, 35, 65 and 80% sucrose. The gradients were centrifuged for 3 h (Beckman rotor SW401, 30,000 rpm). The VLP bands found in the 35–65% sucrose gradient layers were collected, diluted with PBS, concentrated at 30,000 rpm for 2 h. VLPs were resuspended in PBS and stored at −70°C until use.
Western blotting and electron microscopy
rNDV/RSV/F and rNDV/RSV/G were identified by Western blot and electron microscopy. Anti-NDV antibody (Chinese Academy of Inspection and Quarantine) was used to detect NDV NP and NDV M proteins. Monoclonal mouse anti-RSV (Millipore) antibody was used to detect the G protein and Palivizumab (provided by Chongqing Medical University) to detect the F protein. Negative staining of VLPs was performed by transmission electron microscopy to examine their morphology and size.
Immunization and challenge in mice
Female BALB/c mice, aged 6–8 weeks (Animal Experimental Center, AMMS) were immunized i.n. twice with 40 μg VLP in 40-μl volumes at 4-week intervals. Mice were immunized i.n. with 40 μg rNDV/RSV/F, 40 μg rNDV/RSV/G, or 20 μg rNDV/RSV/F plus 20 μg rNDV/RSV/G (abbreviated as rNDV/RSV/F+G), respectively. Serum, nasal and lung extract samples were collected on day 14 after prime-boost immunization. The mice were challenged with live RSV A2 (1.5 × 106 PFU), and the lung and nasal extracts were obtained on day 6 post-challenge. All animal experiments were conducted in accordance with the guidelines of the Academy of Military Medical Sciences Institutional Animal Care and Use Committee.
RSV protein-specific antibody response
The enzyme-linked immunsorbent assay (ELISA) was performed to determine the levels of RSV-specific antibodies (IgG, IgG1, IgG2a and sIgA) in serum and in nasal and lung extracts, as described previously.Citation26
Antibody neutralization
Mouse sera immunizations with VLPs were complement inactivated at 56°C for 30 minutes. And then serially diluted sera incubated 750 PFU with Live RSV A2 at 37°C for 1 hour. And then virus-serum mixtures were added to monolayers of HEp-2 cells grown in 12-well plates. After 1 hour, the mixture was removed. The percent plaque reduction by sera compared with sera from control were determined.
ELISPOT assay
Antibodies (5 mg/ml) against mouse IL-4 and interferon-γ (BD Company) were used to coat 96-well filtration plates (Millipore) at 4°C overnight, as described previously.Citation27 The isolated splenocytes were collected on day 14 after the boost (105 cells/well) and were stimulated with 5 μg/ml purified VLPs. The plates were incubated for 48 h at 37°C, 5% CO2. Spots were computed and analyzed using the ELISPOT reader system and software (Cellular Technology).
Changes in weight, lung histopathology and viral load in mice after challenge
Immunized mice were challenged i.n. with live RSV strain A2 for protective evaluation. The individual lung tissues, lungs and nasal extracts were collected on day 6 post-challenge. The lung tissues were fixed in 4% formaldehyde, cut into pathological sections, and stained with hematoxylin and eosin (H&E) (Institute of Diseases Control and Prevention, AMMS). The inflammatory cells were counted among 100 fields selected at random within pathological sections. The viral loads of the lung tissue and nasal homogenates were determined by plaque assay.Citation26
Statistical analysis
The data were analyzed by ANOVA using SPSS version 16.0 and Tukey's Sidak's multiple comparisons test; * P < 0.05 and **P < 0.01.
Disclosure of Potential Conflicts of Interest
No potential conflicts of interest were disclosed.
References
- Mejias A, Chavez-Bueno S, Jafri HS, Ramilo O. Respiratory syncytial virus infections: old challenges and new opportunities. Pediatr Infect Dis J 2005; 24:S189-96, discussion S96-7; PMID:16378045; http://dx.doi.org/10.1097/01.inf.0000188196.87969.9a
- Stokes KL, Currier MG, Sakamoto K, Lee S, Collins PL, Plemper RK, Moore ML. The respiratory syncytial virus fusion protein and neutrophils mediate the airway mucin response to pathogenic respiratory syncytial virus infection. J Virol 2013; 87:10070-82; PMID:23843644; http://dx.doi.org/10.1128/JVI.01347-13
- Hurwitz JL. Respiratory syncytial virus vaccine development. Expert Rev Vaccines 2011; 10:1415-33; PMID:21988307; http://dx.doi.org/10.1586/erv.11.120
- Wright M, Piedimonte G. Respiratory syncytial virus prevention and therapy: past, present, and future. Pediatr Pulmonol 2011; 46:324-47; PMID:21438168; http://dx.doi.org/10.1002/ppul.21377
- Roldao A, Mellado MC, Castilho LR, Carrondo MJ, Alves PM. Virus-like particles in vaccine development. Expert Rev Vaccines 2010; 9:1149-76; PMID:20923267; http://dx.doi.org/10.1586/erv.10.115
- Murawski MR, McGinnes LW, Finberg RW, Kurt-Jones EA, Massare MJ, Smith G, Heaton PM, Fraire AE, Morrison TG. Newcastle disease virus-like particles containing respiratory syncytial virus G protein induced protection in BALB/c mice, with no evidence of immunopathology. J Virol 2010; 84:1110-23; PMID:19889768; http://dx.doi.org/10.1128/JVI.01709-09
- Quan FS, Kim Y, Lee S, Yi H, Kang SM, Bozja J, Moore ML, Compans RW. Viruslike particle vaccine induces protection against respiratory syncytial virus infection in mice. J Infect Dis 2011; 204:987-95; PMID:21881112; http://dx.doi.org/10.1093/infdis/jir474
- Mena JA, Kamen AA. Insect cell technology is a versatile and robust vaccine manufacturing platform. Expert Rev Vaccines 2011; 10:1063-81; PMID:21806400; http://dx.doi.org/10.1586/erv.11.24
- Deschuyteneer M, Elouahabi A, Plainchamp D, Plisnier M, Soete D, Corazza Y, Lockman L, Giannini S, Deschamps M. Molecular and structural characterization of the L1 virus-like particles that are used as vaccine antigens in Cervarix, the AS04-adjuvanted HPV-16 and -18 cervical cancer vaccine. Hum Vaccines 2010; 6:407-19; PMID:20953154; http://dx.doi.org/10.4161/hv.6.5.11023
- Munoz FM, Piedra PA, Glezen WP. Safety and immunogenicity of respiratory syncytial virus purified fusion protein-2 vaccine in pregnant women. Vaccine 2003; 21:3465-7; PMID:12850361; http://dx.doi.org/10.1016/S0264-410X(03)00352-9
- Singh SR, Dennis VA, Carter CL, Pillai SR, Jefferson A, Sahi SV, Moore EG. Immunogenicity and efficacy of recombinant RSV-F vaccine in a mouse model. Vaccine 2007; 25:6211-23; PMID:17629376; http://dx.doi.org/10.1016/j.vaccine.2007.05.068
- Becker Y. Respiratory syncytial virus (RSV) evades the human adaptive immune system by skewing the Th1/Th2 cytokine balance toward increased levels of Th2 cytokines and IgE, markers of allergy–a review. Virus Genes 2006; 33:235-52; PMID:16972040
- Wahl M, Hermodsson S. Intradermal, subcutaneous or intramuscular administration of hepatitis B vaccine: side effects and antibody response. Scand J Infect Dis 1987; 19:617-21; PMID:3441747; http://dx.doi.org/10.3109/00365548709117195
- Brandtzaeg P. Induction of secretory immunity and memory at mucosal surfaces. Vaccine 2007; 25:5467-84; PMID:17227687; http://dx.doi.org/10.1016/j.vaccine.2006.12.001
- Corthesy B. Role of secretory IgA in infection and maintenance of homeostasis. Autoimmu Rev 2013; 12:661-5; PMID:23201924; http://dx.doi.org/10.1016/j.autrev.2012.10.012
- van Riet E, Ainai A, Suzuki T, Hasegawa H. Mucosal IgA responses in influenza virus infections; thoughts for vaccine design. Vaccine 2012; 30:5893-900; PMID:22835738; http://dx.doi.org/10.1016/j.vaccine.2012.04.109
- Patel H, Yewale C, Rathi MN, Misra A. Mucosal immunization: a review of strategies and challenges. Crit Rev Ther Drug Carrier Syst 2014; 31:273-303; PMID:25072196; http://dx.doi.org/10.1615/CritRevTherDrugCarrierSyst.2014010102
- Kupsch J, Saizawa KM, Eichmann K. Expression of murine soluble CD4 protein in baculovirus infected insect cells. Immunobiology 1992; 186:254-67; PMID:1490731; http://dx.doi.org/10.1016/S0171-2985(11)80255-X
- Rudan I, O'Brien KL, Nair H, Liu L, Theodoratou E, Qazi S, Luksic I, Fischer Walker CL, Black RE, Campbell H, et al. Epidemiology and etiology of childhood pneumonia in 2010: estimates of incidence, severe morbidity, mortality, underlying risk factors and causative pathogens for 192 countries. J Glob Health 2013; 3:010401; PMID:23826505; http://dx.doi.org/10.7189/jogh.03.010101
- Blanco JC, Boukhvalova MS, Shirey KA, Prince GA, Vogel SN. New insights for development of a safe and protective RSV vaccine. Hum Vaccines 2010; 6:482-92; PMID:20671419; http://dx.doi.org/10.4161/hv.6.6.11562
- Correia BE, Bates JT, Loomis RJ, Baneyx G, Carrico C, Jardine JG, Rupert P, Correnti C, Kalyuzhniy O, Vittal V, et al. Proof of principle for epitope-focused vaccine design. Nature 2014; 507:201-6; PMID:24499818; http://dx.doi.org/10.1038/nature12966
- McGinnes LW, Gravel KA, Finberg RW, Kurt-Jones EA, Massare MJ, Smith G, Schmidt MR, Morrison TG. Assembly and immunological properties of Newcastle disease virus-like particles containing the respiratory syncytial virus F and G proteins. J Virol 2011; 85:366-77; PMID:20980510; http://dx.doi.org/10.1128/JVI.01861-10
- Falsey AR, Singh HK, Walsh EE. Serum antibody decay in adults following natural respiratory syncytial virus infection. J Med Virol 2006; 78:1493-7; PMID:16998887; http://dx.doi.org/10.1002/jmv.20724
- Domachowske JB, Rosenberg HF. Respiratory syncytial virus infection: immune response, immunopathogenesis, and treatment. Clin Microbiol Rev 1999; 12:298-309; PMID:10194461
- Mazanec MB, Kaetzel CS, Lamm ME, Fletcher D, Nedrud JG. Intracellular neutralization of virus by immunoglobulin A antibodies. Proc Natl Acad Sci U S A 1992; 89:6901-5; PMID:1323121; http://dx.doi.org/10.1073/pnas.89.15.6901
- Bian C, Liu S, Liu N, Zhang G, Xing L, Song Y, Duan Y, Gu H, Zhou Y, Zhang P, et al. Influenza virus vaccine expressing fusion and attachment protein epitopes of respiratory syncytial virus induces protective antibodies in BALB/c mice. Antiviral Res 2014; 104:110-7; PMID:24509239; http://dx.doi.org/10.1016/j.antiviral.2014.01.022
- Gao X, Wang W, Li Y, Zhang S, Duan Y, Xing L, Zhao Z, Zhang P, Li Z, Li R, et al. Enhanced Influenza VLP vaccines comprising matrix-2 ectodomain and nucleoprotein epitopes protects mice from lethal challenge. Antiviral Res 2013; 98:4-11; PMID:23416215; http://dx.doi.org/10.1016/j.antiviral.2013.01.010