Abstract
In order to develop a cell-based vaccine against the Parkinson disease (PD) associated protein α-synuclein (α-Syn) 3 peptides were synthesized based upon predicted B cell epitopes within the full length α-Syn protein sequence. These peptide fragments as well as the full length recombinant human α-Syn (rh- α-Syn) protein were used to sensitize mouse bone marrow-derived dendritic cells (DC) ex vivo, followed by intravenous delivery of these sensitized DCs into transgenic (Tg) mice expressing the human A53T variant of α-Syn. ELISA analysis and testing of behavioral locomotor function by rotometry were performed on all mice after the 5th vaccination as well as just prior to euthanasia. The results indicated that vaccination with peptide sensitized DCs (PSDC) as well as DCs sensitized by rh-α-Syn induced specific anti-α-Syn antibodies in all immunized mice. In terms of rotometry performance, a measure of locomotor activity correlated to brain dopamine levels, mice vaccinated with PSDC or rh- α-Syn sensitized DCs performed significantly better than non-vaccinated Tg control mice during the final assessment (i.e. at 17 months of age) before euthanasia. As well, measurement of levels of brain IL-1α, a cytokine hypothesized to be associated with neuroinflammation, demonstrated that this proinflammatory molecule was significantly reduced in the PSDC and rh- α-Syn sensitized DC vaccinated mice compared to the non-vaccinated Tg control group. Overall, α-Syn antigen-sensitized DC vaccination was effective in generating specific anti- α-Syn antibodies and improved locomotor function without eliciting an apparent general inflammatory response, indicating that this strategy may be a safe and effective treatment for PD.
Introduction
Parkinson disease (PD) is a neurodegenerative disease caused by the death of dopaminergic neurons in the substantia nigra (SN), a region of the midbrain.Citation1,2 Progressive accumulation of abnormal intracellular aggregates of the protein α synuclein (α-Syn) occurs within inclusions/structures known as Lewy bodies which are one of the pathologic hallmarks of PD.Citation3 These structures first appear in olfactory bulb and medulla, and then gradually spread to the midbrain, at which time the initial motor signs of PD develop.Citation4 Concomitantly, inflammatory responses from resident microglia result in T-cell recruitment.Citation5 Together, these events lead to the progressive death of nigro-striatal dopaminergic neurons, resulting in the classical clinical signs of bradykinesia, resting tremor and rigidity.Citation6 Symptomatic relief for PD is provided by dopamine replacement; however, the underlying disease process continues unabated. Advances in research on the evaluation of amyloid beta (Aβ) targeted immunotherapies against Alzheimer disease (AD) have provided new opportunities for the expansion of these strategies for the potential treatment of PD. In fact, vaccines developed against α-Syn, as well as passive antibody immunotherapies targeting α-Syn, have been studied by several research groups.Citation7-9,Citation10-14
Dendritic cell based (DC) vaccination is a cell-based therapy that elicits an immune response through the use of antigen-loaded/sensitized DCs as the vehicle for immunization. DCs have a central role in initiating primary immune responses, through the presentation of antigen to T-cells.Citation15,16 Moreover, studies have revealed that DCs can induce proliferation of B-cells, directly stimulate production of antibodis,Citation17,18 as well as influence immunoglobulin class-switching.Citation19 These findings suggest that DCs can regulate the humoral immune response.Citation20
Antigen-sensitized DCs have been evaluated as potential vaccines for cancer treatment.Citation21-26 Other clinical trials have also been performed to evaluate their potential utility against other disorders such as infectious diseases.Citation27,28 In fact, in animal studies, DCs sensitized with mutant Aβ peptides were used to vaccinate different mouse models of AD, without eliciting a generalized inflammatory response.Citation29-31 However, to date the only approved and licensed therapeutic vaccine using a DC based strategy is Sipuleucel-T (Provenge), used for the treatment of hormone resistant metastatic prostate carcinoma.Citation32-34
Based on past work, the novel study reported here was undertaken to develop and evaluate, immunologically and therapeutically, a DC-based vaccine against human-α-Syn in a Tg mouse model of PD/synucleinopathies. An important advantage of DCs is their “self adjuvant” activity in eliciting an immune response without causing generalized inflammation which typically occurs with vaccines administered with conventional adjuvants.Citation35 Moreover, peptide-sensitized DC (PSDC) vaccines trigger longer lasting antigen-specific immune responses in comparison to traditional vaccines.Citation36 Despite the fact that peptide-sensitized DC vaccination has many advantages, this approach has not yet been explored in a PD-related study. In the present investigationhuman-α-Syn (rh-α-Syn) and α-Syn peptides, containing the B cell epitopes, were used to sensitize DCs . These sensitized DCs were then used as a vaccine to evaluate immune responses in a Tg mouse model of PD that expresses a 140 amino acid full length human A53T variant α-Syn (B6;C3-Tg (Prnp-SNCA*A53T)83Vle/J), under the control of the mouse prion protein promoter. Results from this study indicate the ability of the α-Syn protein/peptide sensitized DC vaccine to elicit specific anti- α-Syn protein/peptide antibody responses. As well, these DC vaccines ameliorated the locomotor deficits which are characteristic of the α-Syn expressing Tg PD mouse model used in this investigation.
Results
In the results presented in the subsequent figures, 3 of the vaccination/treatment groups, as indicated, utilized the Tg mouse model expressing human α-Syn, the genotype of which is described and referenced in the Materials and Methods section. indicates the schedule for α-Syn peptide/ α-Syn recombinant protein or control sensitized DC vaccinations as well as the time points when locomotor (i.e., rotometry) and immune analysis was performed. indicates the location (amino acid numbers) and sequence of the 3 identified B cell epitopes within α-Syn from which the 3 DC sensitizing peptides were generated.
Figure 1. Vaccination and rotometry testing schedule and amino acid sequences of DC sensitizing peptides. (A) Schedule for vaccination, rotometry testing and euthanasia as described further in the Materials and Methods section. The initial vaccination with sensitized or non-sensitized DCs was made in 3.5 month old α− Syn expressing Tg mice. (B) Amino acid sequence and residue numbers for the 3 α-Syn specific B cell epitope-containing peptides used as DC sensitizers.
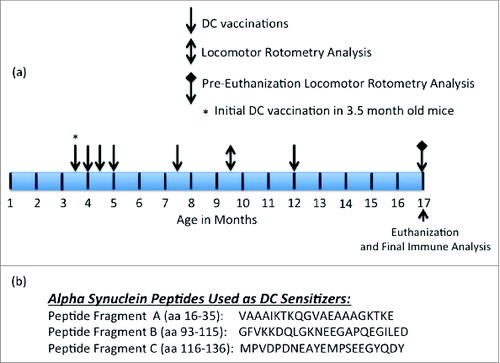
Figure 2. Anti-α− Syn antibody responses elicited by PSDC or rh- α− Syn or rh- sensitized DC vaccines as measured by ELISA. (A) Ten days following the initial vaccination PSDC administered Tg (i.e. Tg PSDC) mice demonstrated a significantly higher anti-α−Syn antibody response, measured by OD450nm values, than did Tg mice vaccinated with rh-α− Syn sensitized DCs (i.e., Tg rh-α− Syn). OD450nm binding values are also provided for non-sensitized PSDC vaccinated mice (i.e. Tg DC Control). (B) Time course of anti-α− Syn antibody responses from analysis of sera from either PSDC (i.e., Tg PSDC) or rh-α− Syn DC sensitized (i.e. Tg rh-α− Syn DC) vaccinated mice. OD450nm binding values are also provided for non-sensitized DC vaccinated mice (i.e., Tg DC Control) (C) Demonstration that sensitization of DCs with peptide fragment C resulted in the highest anti-α− Syn peptide antibody responses of the 3 peptides tested. For analysis of data presented in (A), (B) and (C) above statistical significance was determined by the student t test with differences being significant at the 0.05 level and are indicated by *.
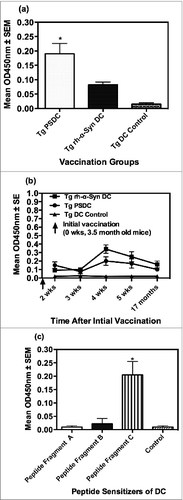
Figure 3. Rotometric locomotor performance of Tg mice at age 17 months after the vaccination regimen with either PSDC or rh-α− Syn sensitized DCs. The PSDC (i.e. Tg PSDC) or rh-α− Syn sensitized (i.e., Tg rh-α− Syn) DC vaccinated Tg mouse groups performed significantly better (i.e. higher latency values) on the rotorod test than did Tg mice vaccinated with non-sensitized DCs (i.e., Tg DC Control). Latency values are also provided for WT (wild type) control mice (i.e. WT Control).
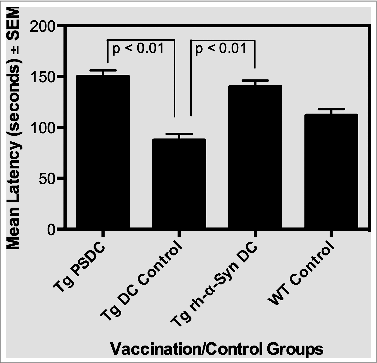
PSDC, administered by intravenous (i.v.) injection into Tg α-Syn mice (Tg-PSDC), induced a more vigorous (p < 0.05) antibody response measured by ELISA against rh- α-Syn (i.e. 0.20 ± 0.04 OD450nm units) after one immunization (quantitated 10 d post-immunization) than the did rh-α-Syn-sensitized DCs (Tg-rh- α-Syn DC) vaccinated group (i.e., 0.08 ± 0.01 OD450nm units). Background antibody binding levels in sera from non-sensitized DC vaccinated mice were at 0.015 ± 0.005 OD450nm units. These data are presented in . However, antibody levels against rh- α-Syn in mice vaccinated with rh-α-Syn sensitized DCs increased after the 3rd vaccination and remained slightly higher or comparable for the duration of the study compared to the antibodies generated in the Tg-PSDC group (). The results indicate that the DC vaccine sensitized with the pooled peptides induced levels of anti- α-Syn specific antibodies more rapidly than the DC vaccine sensitized with rh-α-Syn, even though with time the levels of antibodies in the 2 groups became comparable. Epitope mapping of the anti-sera generated from the vaccinated animals revealed that antibodies produced by PSDC DC vaccination exhibited significant (p < 0.05) binding (0.21 ± 0.05 OD450nm units) only to α-Syn peptide fragment C. Binding to α-Syn peptide fragments A and B was 0.01 ± 0.005 and 0.02 ± 0.002 OD450nm units respectively (). Negative control binding was at 0.012 ± 0.005 OD450nm units. Therefore, binding of antisera to peptides A and B was at background levels. These data suggest that peptide fragment C contained the major B cell epitope which mediated effective DC sensitization.
There was no difference in locomotor performance on the rotorod between the 4 groups of mice after 5 vaccinations that is, at 9.5 months of age (data not shown). However testing of the mice shortly before euthanasia (at 17 months), after they had received a total 6 immunizations revealed that Tg-PSDC and Tg-rh- α-Syn vaccinated mice exhibited (p < 0.05), locomotor performance (i.e., lateney times) scores (150 ± 6 and 140 ± 6 seconds respectively), compared to the Tg DC control or wild type (WT) control groups scores (87 ± 6 and 112 ± 6 seconds respectively). These data are presented in . There was no difference in the mean lateney time scores between the Tg-PSDC and Tg-rh- α-Syn vaccinated mice, suggesting that both α-Syn peptide and α-Syn recombinant protein sensitization of DCs for use as a cell based vaccine/therapy, were comparably effective in ameliorating some of the locomotor behavioral defects characteristic of the α-Syn expressing Tg mice.
Upon euthanization of mice at the 17 month time point, brains of mice from the different vaccinated and control groups were prepared for measurement of the pro-inflammatory cytokine IL-1α. The IL-1 family of cytokines have a significant role in neuroinflammation, with levels of IL-1α and β as well as other pro-inflammatory cytokines being elevated in the brains of AD and PD patients.Citation37-39 As such, IL-1α levels in brain lysates, prepared as indicated above, were measured in the non-Tg WT as well as experimental and control vaccinated α−Syn expressing Tg mice. Data on levels of IL-1α are indicated in and are expressed as mean pg/ml brain lysate ± SEM. Specifically, mean IL-1α levels were 25 ± 1 , 172 ± 13, 70 ± 10 and 100 ± 10 pg/ml brain lysate in WT control, Tg DC control,Tg rh-α−Syn DC and Tg PSDC mouse groups, respectively. Importantly, it was determined that the levels of IL-1 α in the brains of Tg rh-α−Syn DC and Tg PSDC mice were significantly (p < 0.05) decreased compared to the Tg DC control mice. These results suggest that the DC vaccines sensitized with either α−Syn peptides or full-length α−Syn protein mediated a decrease in the brain levels of IL-1α, a cytokine that, as indicated, is associated with neuroinflammation and is often elevated in the brains of PD patients. Future studies will expand analysis of other inflammatory markers in the context of the α-Syn sensitized DC vaccination strategy.
Figure 4. Levels of the pro-inflammatory cytokine IL-1α in brain lysates from PSDC or rh-α− Syn sensitized DC vaccinated mice. Levels of IL-1α in brain lysates are expressed as pg/ml ± SEM. The results indicate that significantly lower levels of IL-1α were measured in the brain lysates from PSDC or rh-α− Syn sensitized DC vaccinated Tg mice than in non-sensitized DC vaccinated Tg controls. IL-1α levels are also provided for WT (wild type) control mice (i.e., WT Control).
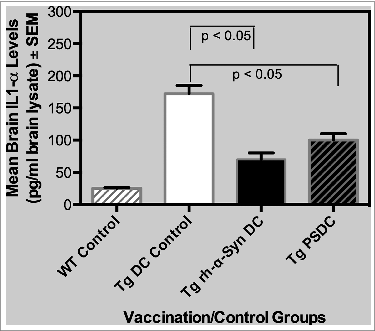
In a further analysis of the data parameters generated in this study, Pearson correlation (r) determinations were performed, which assessed potential associations between sera anti-α-Syn antibody levels, brain IL-1 α levels and locomotor rotometry latency values. Specifically, r-values comparing (a) antibody levels to IL-1α levels, (b) antibody levels to latency values and (c) latency values to IL-1α levels were −0.94, 0.99 and −0.91, respectively. Importantly, these analyses indicate a positive correlation between anti-α-Syn antibody levels and latency values, suggestive of a causal relationship between antigen specific antibody levels and protection against the locomotor. Likewise, there was a negative correlation between levels of anti-α-Syn antibodies or lateney values to IL-1α levels. Overall, the results of these determinations are further supportive evidence of the ability of the α-Syn peptide/protein sensitized DC vaccines to induce antigen specific immune responses which reduces (a) the inflammatory profile in these Tg mice as well as (b) the locomotor deficits characteristic of the α-Syn expressing Tg mouse strain.
Discussion
The application of immune based interventions against Alzheimer disease (AD) has recently being applied to Parkinson disease (PD), the second most common neurodegenerative disorder. Several years ago, the first antigen-loaded DC vaccine against AD was developed and tested in a mouse model.Citation29 This cell-based approach to vaccination generated a long-lasting antibody response without eliciting significant inflammation. Specifically then in the study reported here a similar cell based DC vaccine approach was applied to a PD mouse model Tg which targeted α-Syn. As such, the results presented here indicated that DCs sensitized with full length rh-α-Syn or peptide fragments from h-α-Syn, were effective in triggering the generation of anti-α-Syn antibodies in a Tg α-Syn expressing mouse model of PD synucleinopathy.
The α-Syn peptide fragments used in this study as sensitizers of DCs were generated based on B cell epitope antigenicity analysis of full human α-Syn amino acid sequence. Specifically 3 major B cell epitopes were identified followed by the synthesis of 3 peptide fragments, each containing one of these epitopes. These peptides were combined as a peptide/antigen mixture and used to stimulate mouse bone marrow-derived DCs. The full-length recombinant protein (rh-α-Syn) was also used to sensitize DCs, as a putative positive control. DCs sensitized with the peptide fragment pool (PSDC), when used as a cell based vaccine, elicited a temporally more rapid antigen specific antibody response than did DCs sensitized with full-length rh-α-Syn. This is hypothesized to be due to bypassing of the typical antigen processing steps which generate small peptides from protein antigens, which in turn binds to MHC molecules with subsequent binding of this complex to the TCR of T cells leading ultimately to activation.
Epitope mapping data using anti-sera generated from the DC vaccinated animals verified the antigenicity predicted by analysis with DNAStar 8.1 software, as described in the Materials and Methods. One of the epitopes evaluated (i.e. within peptide C) appears to be the critical antigen that elicits a functional antibody response. Therefore, the epitope displayed in peptide C may be an important target for the development of immune based preventatives/therapies.
Rotometry locomotor behavioral testing demonstrated that both peptide and rh- α-Syn sensitized DC vaccinated Tg mice performed better, in terms of ability to remain on the rod longer (i.e., higher lateney values), than the non-sensitized DC control vaccinated Tg mice. Despite the improvement in locomotor activity in the vaccinated mice, levels of soluble brain α-Syn measured after euthanasia were not different between the groups (data not shown). While it is possible that the assay fails to measure total (insoluble and soluble) α-Syn, a more likely explanation may relate to the mouse model used. Specifically, the PD model used in this study greatly overexpresses α-Syn, to levels considerably higher than those observed in physiological or pathophysiological circumstances. When used in a system that expresses more moderately elevated levels of α-Syn consistent with typical pathology, the cell based DC vaccine used in this study may, in fact, demonstrate a reduction in total α-Syn levels. Another possibility is that the animals were not provided sufficient vaccinations, especially after 12 months of age. In this study the last treatment was at 12 months of age, with euthanasia and post-mortem analyses being performed at 17 months of age. As well, the mouse model of synucleinopathy utilized in this study has limitations, the most important being that the animals do not demonstrate pathology or locomotor dysfunction until an advanced age.Citation40
As indicated, a range of immune-based interventions, including vaccination with the Aβ peptide,Citation41 and passive delivery of antibodies against Aβ have all been demonstrated to be effective, to some extent, in APP/PS1 Tg mouse models of AD, in terms of decreasing Aβ deposition and ameliorating memory deficits in this murine system.Citation42 However, transition of findings in these animal models to human clinical trials has resulted in the development of adverse effects including hemorrhages and encephalitis, which obviously need to be prevented if this vaccination is to have any clinical utility. As well, immune tolerance to Aβ as well as immunization, in the context of an aging immune system, are likewise limitations of vaccine-based interventions.Citation43,44 Therefore, as indicated, in order for immune based therapies to be utilized, a number of different challenges need to be overcome. To compensate for age related diminution of immunity with age a potent immune stimulating adjuvant is often necessary to be included in the vaccine preparation. Unfortunately, such adjuvants often result in over-activation of the immune system with concomitant elicitation of a massive T cell response. These T cells may then infiltrate the brain through a leaky blood brain barrier culminating in neurologic adverse events including encephalitis. Specifically, as indicated above, this observation was made in a subset of subjects in an Aβ vaccine clinical trial.Citation45 Interestingly however, a followup on individuals in this clinical trial revealed that those exhibiting anti-Aβ antibodies had, to some degree, attenuation of clinical symptoms.Citation45 This finding suggests that irrespective of some of the adverse effects noted in the trial, an immune based strategies against neurodegenerative diseases such as AD are worth pursuing.
Using studies on AD as a precedent, vaccines and immunotherapies targeting the intracellular protein α-Syn have also been evaluated in animal models of PD.Citation12,14,46 A traditional vaccination approach against PD will have similar challenges and safety concerns as noted with immune based strategies against AD. Among the challenges with targeting α-Syn in vaccine and immunotherapeutic strategies, as is the case with Aβ for AD, is that α-Syn typically functions as a self-protein. Therefore, this protein will likely exhibit immune tolerance, particularly in the context of the α-Syn expressing Tg mouse model used in the study. However, several studies have indicated that antigen sensitized DCs, when used as a vaccine, can overcome (i.e., break) immune tolerance. This has been specifically reported in a mouse model of scrapie induced by prion proteins,Citation47 as well as in mouse models of tumor inductionCitation48 and hepatitis B infection.Citation49 Also, as previously indicated both α-Syn and Aβ have successfully been able to stimulate antibody responses in appropriate Tg animal models.Citation13,50 Therefore, the potential problem of immune tolerance to α-Syn can be overcome by various strategies including the use of antigen sensitized DC vaccines. Cell based DC vaccines may provide safer and more effective approach against neurodegenerative diseases such as AD and PD. Dendritic cell vaccines have been widely studied and are currently being applied for treatment of cancers.Citation21-26 Most investigations have focused on the major ability of DC based vaccines to stimulate CD8+ T cells.Citation51,52 However, there is evidence as well that DC based vaccines can stimulate antigen specific B cells with the concomitant release of antibody.Citation51-54 As such, it is hypothesized that the protective biological activity of the DC vaccine used in this study and noted in the α-Syn expressing Tg mouse model is likely mediated by α-Syn specific antibodies. In fact, previous published data from our group indicate that DC vaccines are able to redirect the immune response toward a Th2 anti-inflammatory response which decreases the likelihood for the generation of adverse effects often noted associated with pro-inflammatory Th1 immune responses.Citation55,56
However, since cellular immune responses were not directly measured in our study, the possibity of some role of cell- mediated immunity on the effects noted cannot be completely excluded. In addition, and importantly, there are significant advantages with the use of dendritic cell vaccines including: (a) ability to serve as self-adjuvants with no additional stimulation of the immune system required and therefore likely decreasing the chances for developing adverse inflammatory responses and (b) the fact that DCs are collected autologously from patient effectively eliminating the chance of tissue/cell rejection.
Overall, this report has identified the most effective peptide fragment of α-Syn with which to vaccinate mice to successfully produce protective anti α-Syn antibodies in the context of a cell-based DC vaccine. In addition, this study demonstrated that the DC vaccination strategy reduced levels of the pro-inflammatory cytokine IL-1α, which suggests that the α-Syn peptide/protein DC sensitized vaccine approach is both effective in inducing anti- α-Syn immune responses as well as reducing some clinical manifestation of PD in an appropriate Tg model. The mechanism for the reduction in brain levels of IL-1α after α-Syn sensitized DC vaccination is unclear since experiments were not performed in this study to directly address this issue. However, there is evidence that IL-1 stimulates the generation of inflammation inducing Th17 cells through downregulation of anti-inflammatory regulatory T cells.Citation57 This observation underscores the importance of the balance between Th17 cells and regulatory T cells in autoimmune and inflammatory diseases.Citation58 Therefore, it is possible that the α-Syn sensitized DC vaccine can decrease brain IL-1 α levels through the generation and activity of regulatory T cells. This hypothesis will be addressed in future studies.
In summary, the results presented in this paper suggested that the α-Syn recombinant protein and peptide sensitized DC vaccine tested stimulated immune responses as well as an anti-inflammatory phenotype that protected an α-Syn expressing Tg mouse model from locomotor defects. Overall, the results presented in this report indicate the potential clinical utility of this α-Syn DC sensitized cell based vaccine approach against PD.
Materials and Methods
Ethics Statement: The University of South Florida (USF) IACUC reviewed and approved the animal component of this research project, which was conducted in accordance with the United States Public Health Service's Policy on Humane Care and Use of Laboratory Animals.
Murine model: Human α-Syn Tg mice were purchased from Jackson Laboratory (B6;C3-Tg(Prnp-SNCA*A53T)83Vle/J, Bar Harbor, Maine stock number 004479) and were bred at the USF animal facility. Mice homozygous for the Tg insert are viable and normal in size. These Tg mice express human A53T variant α-Syn (full-length, 140 amino acid isoform) under the control of the mouse prion protein promoter. This Tg mouse strain was originally developed by Giasson and colleagues.Citation59 Some of the characteristics documenting the rationale for the use of this Tg mouse strain in the studies in this report are listed as follows. In this Tg strain at 8 months of age, some homozygous mice begin to develop a progressively impaired locomotor phenotype. Full presentation of this phenotype typically manifests at 14–15 months of age on average. Manifestations of minimal grooming, weight loss and diminished mobility precede movement impairment, partial limb paralysis, trembling and inability to stand. Immunohistochemistry analysis of mutants between 8 to 12 months of age indicates widely distributed α-Syn inclusions, with dense accumulation in the spinal cord, brainstem, cerebellum and thalamus. The appearance of α-Syn aggregate inclusions parallels the onset of the motor impairment phenotype. As well, axons and myelin sheaths exhibit progressive ultrastructural degeneration. Immunoelectron microscopy and biochemical analysis of this α-Syn expressing Tg strain demonstrate that the inclusions in neurons are comprised primarily of 10–16 nm fibrils of α-Syn. The structure, location and onset of the inclusions observed in the mutant mice resemble characteristics noted in human neuronal α-synucleinopathies, such as familial PD.
α-Syn recombinant proteins and peptides and immunological assay reagents: Recombinant human α-Syn (rh-α-Syn) was generated by Panoab Inc.. (Tampa, FL). The α-Syn peptides used in this study were synthesized at the USF Peptide Center (Tampa, FL) and Biomer Technology (Pleasanton, CA). All of the antibodies used in the ELISA assay were provided by Panoab Inc.. (Tampa FL).The cytokine/immune stimulators GM-CSF and IL-4 were purchased from R&D (Minneapolis, MN).
Identification of B cell epitope domains and peptide generation: The full length human α-Syn protein was analyzed using DNAStar 8.1 software in order to identify relevant B cell epitope domains. From this analysis 3 major B-cell domains were predicted from which the 3 peptides were generated for this study. The amino acid sequence and residue numbers within the α-Syn protein are presented in .
Preparation and administration of α-Syn sensitized DC based vaccines: DCs were obtained and purified from bone marrow of 10-week-old female non-Tg (C57Bl6) littermates from the α-Syn Tg mouse-breeding colony. Bone marrow isolation followed an established protocol, as published previously.Citation30,60 Briefly, femurs were cleanly excised followed by immersion in cold phosphate-buffered saline (PBS), with subsequent treatment with ethanol and subsequently flushed from the cut femur with media (99% RPMI and 1% antibiotics). Bone marrow was then gently re-suspended and passed through 70 μm sieves into a centrifuge tube. The mixture was centrifuged for 10 min at 1100 rpm, followed by removal of the supernatant followed by brief vortexing of the pellet. Five ml of ACK buffer (0.15 M NH4Cl, 1 mM KHCO3, 0.1 mM EDTA, pH 7.3 at room temperature) was added for 30min, with shaking, in order to lyse red blood cells. Following centrifugation at 1,100 rpm (10°C for 10 min), cells were suspended at 106 cells/ml in medium (RPMI with+10%-FBS + 1% antibiotics). Ten ng/ml of GM-CSF and IL-4 each (BD-PharMingen, San Jose CA) were added to the media, and cells were cultured in 6-well plates (3 ml/well). On the second day of culturing, the medium was completely aspirated to remove all non-adherent cells (lymphocytes etc.), followed by the addition of 3 ml of fresh DC culture medium. The cells were permitted to grow in a CO2 incubator (5% CO2) and on day 4 were treated as follows: 1 ml/well old medium was aspirated and replaced by 1 ml/well fresh DC culture medium containing 60 μg/ml peptide (diluted to a final concentration 20 μg/ml). On the 8th day, DCs were harvested, and the supernatant collected for analysis.Citation29,56 The vaccine was prepared by sensitizing the antigen-presenting DCs with rh-α-Syn or a mixture of the α-Syn peptides at 10 μg/ml for 7 d After DCs were cultured, they were washed with 1xPBS twice and the concentration adjusted to 5.0×106 cells, followed by centrifugation Two hundred microliters of 106 cells were then injected intravenously (i.v.). There were 4 groups used in the study. The Tg α-Syn expressing mice were grouped into the initial 2 treatment cohorts, the first being DCs sensitized with recombinant full length human α-Syn (designated Tg hr- α-Syn DC) and the second being DCs sensitized with the α-Syn peptides (designated Tg PSDC). The third group was a Tg mouse control group, which was vaccinated only with non-sensitized DCs (designated Tg DC control). A fourth and final group consisted of non-Tg littermate mice, which were used as a non-Tg, non-treated control (designated WT control). Each group of mice consisted of an n of 7. The treatment/vaccination schedule and schema is illustrated in . Specifically, vaccinations were initiated in 3.5-month-old Tg mice, which functioned as the primary immunization (i.e. also designated as week 0). The subsequent initial 3 booster vaccinations/treatments were performed at 2-week intervals i.e., at 4,4.5 and 5 months of age, corresponding to 2,4 and 6 weeks following the initial vaccination. Two additional boosters were performed at 7.5 and 12 months of age, which were 16 and 34 weeks after the primary vaccination, respectively.
Blood Sample Collection: Blood samples were collected, at time points indicated in the results section and figure legends, via the submandibular vein into an EDTA-containing tube after each vaccination. Plasma was separated by centrifugation at 9300 rcf for 2 minutes, and then aliquoted into screw-capped tubes for long -term storage at −80˚C. All mice were euthanized after the last test of locomotor activity followed by collection of plasma and brain tissue for subsequent analyses.
Measurement of locomotor behavioral activity in vaccinated and control a-Syn expressing Tg mice: Coordinated locomotor activity was measured on a 47600 - Mouse Rota-Rod rotometer purchased from Ugo Basile (Comerio VA, Italy). Briefly for this test, mice were placed on a rotating rod, which gradually accelerated from a rate of 4 to-40 rpms over a period of 5 minutes until the mice could no longer remain on the rod. The duration of time (seconds), designated as latency, that the mice remained on the rod was recorded, and each mouse was tested 5 times each day for 3 d. Data was expressed as mean latency (seconds ± SEM) for each group of animals. A decrease in latency times in α-Syn Tg mice compared to the non-Tg control animals is indicative of depletion of SN levels of dopamine, the loss of which is correlated with the development of symptoms of PD.Citation61,62 As such, in these experiments higher latency values in vaccinated α-Syn Tg mice, compared to non-vaccinated Tg animals would be indicative of a protective effect of DC vaccination against dopamine depletion. A one-way ANOVA was performed and followed by Fisher's LSD test for the difference between groups.
Measurement of specific anti- α-Syn antibody responses: Humoral immune responses against the α synuclein peptides were measured using methods previously reported, with some modifications.Citation63 Briefly, either single synthetic α-Syn peptides or rh- α-Syn were immobilized at a concentration of 5 μg/ml (50 μl/well) in 96 well Immulon 4 plates (Dynatech Inc..) and incubated overnight at 4°C. Plates were then rinsed with washing buffer (0.45% NaCl in distilled/ deionized water containing 0.05% Tween-20). After incubation, plates were washed again and subsequently incubated with the various dilutions of sera from vaccinated mice. After an hour of incubation, plates were again washed and incubated with 100 μl of a goat anti-mouse IgG–HRP conjugate diluted in blocking buffer for 1 h at 37°C. Following incubation plates were again washed with a 1-mg/ml solution of TMB substrate. After color development, the reaction was stopped by addition of 25 μl of 2N H2SO4 per well. Plates were then read on an ELISA plate reader/spectrophotometer at 450 nm. OD values were then graphed versus dilution of sera.
Brain tissue preparation: For preparation of brain lysates mice were deeply anesthetized followed by intracardiac perfusion with PBS solution. The brain was sectioned into 2 and each was rapidly frozen in dry ice for subsequent chemical/immunological analysis. The brain tissue was then lysed with RIPA buffer containing a protease inhibitor and the lysates stored at −70C until subsequent analysis.
Cytokine level determinations: Milliplex MAP Kits for mouse interleukin-1 α (IL-1α) from Millipore Corp. (Billerica, MA) were used for quantification of the cytokine level in brain tissue lysate, the preparation of which was described above.
Data analysis: All data in this manuscript were analyzed for statistical significance using the Graph Pad 5.0 software, α-Sy and cytokine quantitation results were analyzed with a one–way ANOVA, followed by post-hoc Tukey test between groups. Locomotor behavioral activity differences between the groups were measured by Fishers LSD test. Antibody binding differences were measured by AIVOVA followed by post-hoc Tukey test. The level for statistical significance was set at 0.05 (2-sided).
Disclosure of Potential Conflicts of Interest
No potential conflicts of interest were disclosed.
Acknowledgments
Dr. David Morgan has provided constructive suggestion for this project; the Byrd Alzheimer Institute has provided a supportive research environment for this study.
Funding
This work was supported by a grant from the Parkinson Research Foundation and the USF-Health Byrd Alzheimer Institute Start-up Fund.
References
- Damier P, Hirsch EC, Agid Y, Graybiel AM. The substantia nigra of the human brain. II. Patterns of loss of dopamine-containing neurons in Parkinson disease. Brain 1999; 122 ( Pt 8):1437-48; PMID:10430830; http://dx.doi.org/10.1093/brain/122.8.1437
- Bernheimer H, Birkmayer W, Hornykiewicz O, Jellinger K, Seitelberger F. Brain dopamine and the syndromes of Parkinson and Huntington. Clinical, morphological and neurochemical correlations. J Neurol Sci 1973; 20:415-55; PMID:4272516; http://dx.doi.org/10.1016/0022-510X(73)90175-5
- Spillantini MG, Crowther RA, Jakes R, Hasegawa M, Goedert M. α-Synuclein in filamentous inclusions of Lewy bodies from Parkinson disease and dementia with lewy bodies. Proc Natl Acad Sci U S A 1998; 95:6469-73; PMID:9600990; http://dx.doi.org/10.1073/pnas.95.11.6469
- Braak H, Del Tredici K, Bratzke H, Hamm-Clement J, Sandmann-Keil D, Rub U. Staging of the intracerebral inclusion body pathology associated with idiopathic Parkinson disease (preclinical and clinical stages). J Neurol 2002; 249 Suppl 3):III/1-5; PMID:11954855; http://dx.doi.org/10.1007/s00415-002-1301-4
- Brochard V, Combadiere B, Prigent A, Laouar Y, Perrin A, Beray-Berthat V, Bonduelle O, Alvarez-Fischer D, Callebert J, Launay JM, et al. Infiltration of CD4+ lymphocytes into the brain contributes to neurodegeneration in a mouse model of Parkinson disease. J Clin Invest 2009; 119:182-92; PMID:19104149
- Berardelli A, Rothwell JC, Thompson PD, Hallett M. Pathophysiology of bradykinesia in Parkinson disease. Brain 2001; 124:2131-46; PMID:11673316; http://dx.doi.org/10.1093/brain/124.11.2131
- Tran HT, Chung CH, Iba M, Zhang B, Trojanowski JQ, Luk KC, Lee VM. Alpha-synuclein immunotherapy blocks uptake and templated propagation of misfolded α-synuclein and neurodegeneration. Cell Rep 2014; 7:2054-65; PMID:24931606; http://dx.doi.org/10.1016/j.celrep.2014.05.033
- Masliah E, Rockenstein E, Mante M, Crews L, Spencer B, Adame A, Patrick C, Trejo M, Ubhi K, Rohn TT, et al. Passive immunization reduces behavioral and neuropathological deficits in an α-synuclein transgenic model of Lewy body disease. PloS One 2011; 6:e19338; PMID:21559417; http://dx.doi.org/10.1371/journal.pone.0019338
- Games D, Valera E, Spencer B, Rockenstein E, Mante M, Adame A, Patrick C, Ubhi K, Nuber S, Sacayon P, et al. Reducing C-terminal-truncated α-synuclein by immunotherapy attenuates neurodegeneration and propagation in Parkinson disease-like models. J Neurosci 2014; 34:9441-54; PMID:25009275; http://dx.doi.org/10.1523/JNEUROSCI.5314-13.2014
- Schneeberger A, Mandler M, Mattner F, Schmidt W. Vaccination for Parkinson disease. Parkinsonism Relat Disord 2012; 18 Suppl 1:S11-3; PMID:22166404; http://dx.doi.org/10.1016/S1353-8020(11)70006-2
- Sanchez-Guajardo V, Annibali A, Jensen PH, Romero-Ramos M. α-Synuclein vaccination prevents the accumulation of parkinson disease-like pathologic inclusions in striatum in association with regulatory T cell recruitment in a rat model. J Neuropathol Exp Neurol 2013; 72:624-45; PMID:23771222; http://dx.doi.org/10.1097/NEN.0b013e31829768d2
- Mougenot AL, Betemps D, Hogeveen KN, Kovacs GG, Chouaf-Lakhdar L, Milhavet O, Lehmann S, Legastelois S, Pin JJ, Baron TG. Production of a monoclonal antibody, against human α-synuclein, in a subpopulation of C57BL/6J mice, presenting a deletion of the α-synuclein locus. J Neurosci Methods 2010; 192:268-76; PMID:20709102; http://dx.doi.org/10.1016/j.jneumeth.2010.08.010
- Masliah E, Rockenstein E, Adame A, Alford M, Crews L, Hashimoto M, Seubert P, Lee M, Goldstein J, Chilcote T, et al. Effects of α-synuclein immunization in a mouse model of Parkinson disease. Neuron 2005; 46:857-68; PMID:15953415; http://dx.doi.org/10.1016/j.neuron.2005.05.010
- Hirsch E, Ruberg M, Dardenne M, Portier MM, Javoy-Agid F, Bach JF, Agid Y. Monoclonal antibodies raised against Lewy bodies in brains from subjects with Parkinson disease. Brain Res 1985; 345:374-8; PMID:2994847; http://dx.doi.org/10.1016/0006-8993(85)91020-0
- Banchereau J, Steinman RM. Dendritic cells and the control of immunity. Nature 1998; 392:245-52; PMID:9521319; http://dx.doi.org/10.1038/32588
- Steinman RM. The dendritic cell system and its role in immunogenicity. Annual Rev Immunol 1991; 9:271-96; PMID:1910679; http://dx.doi.org/10.1146/annurev.iy.09.040191.001415
- Dubois B, Bridon JM, Fayette J, Barthelemy C, Banchereau J, Caux C, Briere F. Dendritic cells directly modulate B cell growth and differentiation. J Leukoc Biol 1999; 66:224-30; PMID:10449158
- Dubois B, Vanbervliet B, Fayette J, Massacrier C, Van Kooten C, Briere F, Banchereau J, Caux C. Dendritic cells enhance growth and differentiation of CD40-activated B lymphocytes. J Exp Med 1997; 185:941-51; PMID:9120400; http://dx.doi.org/10.1084/jem.185.5.941
- Fayette J, Dubois B, Vandenabeele S, Bridon JM, Vanbervliet B, Durand I, Banchereau J, Caux C, Briere F. Human dendritic cells skew isotype switching of CD40-activated naive B cells towards IgA1 and IgA2. J Exp Med 1997; 185:1909-18; PMID:9166420; http://dx.doi.org/10.1084/jem.185.11.1909
- Clark EA. Regulation of B lymphocytes by dendritic cells. J Exp Med 1997; 185:801-3; PMID:9120385; http://dx.doi.org/10.1084/jem.185.5.801
- Barrou B, Benoit G, Ouldkaci M, Cussenot O, Salcedo M, Agrawal S, Massicard S, Bercovici N, Ericson ML, Thiounn N. Vaccination of prostatectomized prostate cancer patients in biochemical relapse, with autologous dendritic cells pulsed with recombinant human PSA. Cancer Immunol Immunother 2004; 53:453-60; PMID:14760510; http://dx.doi.org/10.1007/s00262-003-0451-2
- Cohen S, Haimovich J, Hollander N. B-cell lymphoma and myeloma protection induced by idiotype vaccination with dendritic cells is mediated entirely by T cells in mice. J Immunother 2005; 28:461-6; PMID:16113602; http://dx.doi.org/10.1097/01.cji.0000171312.16171.77
- Gajewski TF, Fallarino F, Ashikari A, Sherman M. Immunization of HLA-A2+ melanoma patients with MAGE-3 or MelanA peptide-pulsed autologous peripheral blood mononuclear cells plus recombinant human interleukin 12. Clin Cancer Res 2001; 7:895s-901s; PMID:11300489
- Loveland BE, Zhao A, White S, Gan H, Hamilton K, Xing PX, Pietersz GA, Apostolopoulos V, Vaughan H, Karanikas V, et al. Mannan-MUC1-pulsed dendritic cell immunotherapy: a phase I trial in patients with adenocarcinoma. Clin Cancer Res 2006; 12:869-77; PMID:16467101; http://dx.doi.org/10.1158/1078-0432.CCR-05-1574
- Mittendorf EA, Storrer CE, Foley RJ, Harris K, Jama Y, Shriver CD, Ponniah S, Peoples GE. Evaluation of the HER2/neu-derived peptide GP2 for use in a peptide-based breast cancer vaccine trial. Cancer 2006; 106:2309-17; PMID:16596621; http://dx.doi.org/10.1002/cncr.21849
- Satthaporn S, Eremin O. Dendritic cells (II): Role and therapeutic implications in cancer. J R Coll Surg Edinb 2001; 46:159-67; PMID:11478013
- Ide F, Nakamura T, Tomizawa M, Kawana-Tachikawa A, Odawara T, Hosoya N, Iwamoto A. Peptide-loaded dendritic-cell vaccination followed by treatment interruption for chronic HIV-1 infection: a phase 1 trial. J Med Virol 2006; 78:711-8; PMID:16628588; http://dx.doi.org/10.1002/jmv.20612
- Pellegatta S, Poliani PL, Corno D, Grisoli M, Cusimano M, Ubiali F, Baggi F, Bruzzone MG, Finocchiaro G. Dendritic cells pulsed with glioma lysates induce immunity against syngeneic intracranial gliomas and increase survival of tumor-bearing mice. Neurol Res 2006; 28:527-31; PMID:16808884; http://dx.doi.org/10.1179/016164106X116809
- Cao C, Lin X, Zhang C, Wahi MM, Wefes I, Arendash G, Potter H. Mutant amyloid-β-sensitized dendritic cells as Alzheimer disease vaccine. J Neuroimmunol 2008; 200:1-10; PMID:18649951; http://dx.doi.org/10.1016/j.jneuroim.2008.05.017
- Luo Z, Li J, Nabar NR, Lin X, Bai G, Cai J, Zhou SF, Cao C, Wang J. Efficacy of a therapeutic vaccine using mutated β-amyloid sensitized dendritic. J Neuroimmune Pharmacol 2012; 7:640-55; PMID:22684353; http://dx.doi.org/10.1007/s11481-012-9371-2
- Nabar NR, Yuan F, Lin X, Wang L, Bai G, Mayl J, Li Y, Zhou SF, Wang J, Cai J, et al. Cell therapy: a safe and efficacious therapeutic treatment for Alzheimer disease in APP+PS1 mice. PloS One 2012; 7:e49468; PMID:23226497; http://dx.doi.org/10.1371/journal.pone.0049468
- Wesley JD, Whitmore J, Trager J, Sheikh N. An overview of sipuleucel-T: autologous cellular immunotherapy for prostate cancer. Hum Vaccin Immunother 2012; 8:520-7; PMID:22370520; http://dx.doi.org/10.4161/hv.18769
- Small EJ, Fratesi P, Reese DM, Strang G, Laus R, Peshwa MV, Valone FH. Immunotherapy of hormone-refractory prostate cancer with antigen-loaded dendritic cells. J Clin Oncol 2000; 18:3894-903; PMID:11099318
- Sims RB. Development of sipuleucel-T: autologous cellular immunotherapy for the treatment of metastatic castrate resistant prostate cancer. Vaccine 2012; 30:4394-7; PMID:22122856; http://dx.doi.org/10.1016/j.vaccine.2011.11.058
- Hart DN. Dendritic cells: unique leukocyte populations which control the primary immune response. Blood 1997; 90:3245-87; PMID:9345009
- Steinman RM. Dendritic cells and the control of immunity: enhancing the efficiency of antigen presentation. Mt Sinai J Med 2001; 68:160-6; PMID:11373688
- Shaftel SS, Griffin WS, O'Banion MK. The role of interleukin-1 in neuroinflammation and Alzheimer disease: an evolving perspective. J Neuroinflammation 2008; 5:7; PMID:18302763; http://dx.doi.org/10.1186/1742-2094-5-7
- Hirsch EC, Hunot S. Neuroinflammation in Parkinson disease: a target for neuroprotection? Lancet Neurol 2009; 8:382-97; PMID:19296921; http://dx.doi.org/10.1016/S1474-4422(09)70062-6
- Basu A, Krady JK, Levison SW. Interleukin-1: a master regulator of neuroinflammation. J Neurosci Res 2004; 78:151-6; PMID:15378607; http://dx.doi.org/10.1002/jnr.20266
- Fernagut P-O, Chesselet M-F. Alpha-synuclein and transgenic mouse models. Neurobiol Dis 2004; 17:123-30; PMID:15474350; http://dx.doi.org/10.1016/j.nbd.2004.07.001
- Morgan D, Diamond DM, Gottschall PE, Ugen KE, Dickey C, Hardy J, Duff K, Jantzen P, DiCarlo G, Wilcock D, et al. A[β] peptide vaccination prevents memory loss in an animal model of Alzheimer disease. Nature, 2000; 408:982-5; PMID:11140686
- McLaurin J, Cecal R, Kierstead ME, Tian X, Phinney AL, Manea M, French JE, Lambermon MH, Darabie AA, Brown ME; et al. Therapeutically effective antibodies against amyloid-[β] peptide target amyloid-[β] residues 4-10 and inhibit cytotoxicity and fibrillogenesis. Nat Med 2002; 8:1263-9.
- Vasilevko V, Head E. Immunotherapy in a natural model of Abeta pathogenesis: the aging beagle. CNS Neurol Disord Drug Targets 2009; 8:98-113; PMID:19355931; http://dx.doi.org/10.2174/187152709787847333
- Cicin-Sain L, Smyk-Pearson S, Currier N, Byrd L, Koudelka C, Robinson T, Swarbrick G, Tackitt S, Legasse A, Fischer M, et al. Loss of naive T cells and repertoire constriction predict poor response to vaccination in old primates. J Immunol 2010; 184:6739-45; http://dx.doi.org/10.4049/jimmunol.0904193
- Sela M, Arnon R, Schechter B. Therapeutic vaccines: realities of today and hopes for the future. Drug Discov Today 2002; 7:664-73; PMID:12110243; http://dx.doi.org/10.1016/S1359-6446(02)02296-1
- Masliah E, Rockenstein E, Adame A, Alford M, Crews L, Hashimoto M, Seubert P, Lee M, Goldstein J, Chilcote T, et al. Effects of α-synuclein immunization in a mouse model of Parkinson disease. Neuron 2005; 46:857-68; PMID:15953415; http://dx.doi.org/10.1016/j.neuron.2005.05.010
- Bachy V, Ballerini C, Gourdain P, Prignon A, Iken S, Antoine N, Rosset M, Carnaud C. Mouse vaccination with dendritic cells loaded with prion protein peptides overcomes tolerance and delays scrapie. J Gen Virol 2010; 91:809-20; PMID:19864503; http://dx.doi.org/10.1099/vir.0.013417-0
- Okano F, Merad M, Furumoto K, Engleman EG. In vivo manipulation of dendritic cells overcomes tolerance to unmodified tumor-associated self antigens and induces potent antitumor immunity. J Immunol 2005; 174:2645-52; http://dx.doi.org/10.4049/jimmunol.174.5.2645
- Farag MM, Tedjokusumo R, Flechtenmacher C, Asen T, Stremmel W, Muller M, Protzer U, Weigand K. Immune tolerance against HBV can be overcome in HBV transgenic mice by immunization with dendritic cells pulsed by HBVsvp. Vaccine 2012; 30:6034-9; PMID:22867720; http://dx.doi.org/10.1016/j.vaccine.2012.07.057
- Morgan D, Diamond DM, Gottschall PE, Ugen KE, Dickey C, Hardy J, Duff K, Jantzen P, DiCarlo G, Wilcock D, et al. A β peptide vaccination prevents memory loss in an animal model of Alzheimer disease. Nature 2000; 408:982-5; PMID:11140686; http://dx.doi.org/10.1038/35050116
- Yu JS, Liu G, Ying H, Yong WH, Black KL, Wheeler CJ. Vaccination with tumor lysate-pulsed dendritic cells elicits antigen-specific, cytotoxic T-cells in patients with malignant glioma. Cancer Res 2004; 64:4973-9; PMID:15256471; http://dx.doi.org/10.1158/0008-5472.CAN-03-3505
- Nair SK, Heiser A, Boczkowski D, Majumdar A, Naoe M, Lebkowski JS, Vieweg J, Gilboa E. Induction of cytotoxic T cell responses and tumor immunity against unrelated tumors using telomerase reverse transcriptase RNA transfected dendritic cells. Nat Med 2000; 6:1011-7; PMID:10973321; http://dx.doi.org/10.1038/79519
- Gruber A, Chalmers AS, Rasmussen RA, Ong H, Popov S, Andersen J, Hu SL, Ruprecht RM. Dendritic cell-based vaccine strategy against human immunodeficiency virus clade C: skewing the immune response toward a helper T cell type 2 profile. Viral Immunol 2007; 20:160-9; PMID:17425430; http://dx.doi.org/10.1089/vim.2006.0052
- Boscardin SB, Hafalla JC, Masilamani RF, Kamphorst AO, Zebroski HA, Rai U, Morrot A, Zavala F, Steinman RM, Nussenzweig RS, et al. Antigen targeting to dendritic cells elicits long-lived T cell help for antibody responses. J Exp Med 2006; 203:599-606; PMID:16505139; http://dx.doi.org/10.1084/jem.20051639
- Nabar NR, Yuan F, Lin X, Wang L, Bai G, Mayl J, Li Y, Zhou SF, Wang J, Cai J, et al. Cell therapy: a safe and efficacious therapeutic treatment for Alzheimer disease in APP+PS1 mice. PLoS One, 2012;7:e49468; http://dx.doi.org/10.1371/journal.pone.0049468
- Luo Z, Li J, Nabar NR, Lin X, Bai G, Cai J, Zhou SF, Cao C, Wang J. Efficacy of a therapeutic vaccine using mutated β-amyloid sensitized dendritic cells in Alzheimer mice. J Neuroimmune Pharmacol 2012; 7:640-55; PMID:22684353; http://dx.doi.org/10.1007/s11481-012-9371-2
- Ikeda S, Saijo S, Murayama MA, Shimizu K, Akitsu A, Iwakura Y. Excess IL-1 signaling enhances the development of Th17 cells by downregulating TGF-β-induced Foxp3 expression. J Immunol 2014; 192:1449-58; http://dx.doi.org/10.4049/jimmunol.1300387
- Noack M, Miossec P. Th17 and regulatory T cell balance in autoimmune and inflammatory diseases. Autoimmun Rev 2014; 13:668-77; PMID:24418308; http://dx.doi.org/10.1016/j.autrev.2013.12.004
- Giasson BI, Duda JE, Quinn SM, Zhang B, Trojanowski JQ, Lee VM. Neuronal α-synucleinopathy with severe movement disorder in mice expressing A53T human α-synuclein. Neuron 2002; 34:521-33; PMID:12062037; http://dx.doi.org/10.1016/S0896-6273(02)00682-7
- Cao C, Lin X, Zhang C, Wahi M, Wefes I, Arendash G, Potter H. Mutant amyloid-β-sensitized dendritic cells as Alzheimer disease vaccine. J Neuroimmunol 2008; 200:1-10; PMID:18649951; http://dx.doi.org/10.1016/j.jneuroim.2008.05.017
- Rozas G, Guerra MJ, Labandeira-Garcia JL. An automated rotarod method for quantitative drug-free evaluation of overall motor deficits in rat models of parkinsonism. Brain Res Brain Res Protoc 1997; 2:75-84; PMID:9438075; http://dx.doi.org/10.1016/S1385-299X(97)00034-2
- Meredith GE, Kang UJ. Behavioral models of Parkinson disease in rodents: a new look at an old problem. Mov Disord 2006; 21:1595-606; PMID:16830310; http://dx.doi.org/10.1002/mds.21010
- Cao C, Lin X, Wahi MM, Jackson EA, Potter H, Jr. Successful adjuvant-free vaccination of BALB/c mice with mutated amyloid β peptides. BMC Neurosci. England, 2008; 9:25.