Abstract
Tuberculosis (TB) still remains a major public health issue despite the current available vaccine for TB, Bacille Calmette Guerin (BCG). An effective vaccine against TB remains a top priority in the fight against this pandemic bacterial infection. Adequate protection against TB is associated with the development of TH1-type and CD8+ T cell responses. One alarmin cytokine, interleukin 33 (IL-33), has now been implicated in the development of both CD4+ TH1 and CD8+ T cell immunity. In this study, we determined whether the administration of IL-33 as an adjuvant, encoded in a DNA plasmid, could enhance the immunogenicity of a TB DNA vaccine. We report that the co-immunization of IL-33 with a DNA vaccine expressing the Mycobacterium Tuberculosis (Mtb) antigen 85B (Ag85B) induced robust Ag85B-specific IFNγ responses by ELISpot compared to Ag85B alone. Furthermore, these enhanced responses were characterized by higher frequencies of Ag85B-specific, multifunctional CD4+ and CD8+ T cells. Vaccination with IL-33 also increased the ability of the Ag85B-specific CD8+ T cells to undergo degranulation and to secrete IFNγ and TNFα cytokines. These finding highlights IL-33 as a promising adjuvant to significantly improve the immunogenicity of TB DNA vaccines and support further study of this effective vaccine strategy against TB.
Introduction
Tuberculosis (TB) continues to be one of the most devastating infectious diseases existing worldwide and a major threat to global health. The causative agent of TB, Mycobacterium tuberculosis (Mtb), infects as many as 8.8 million new individuals per year with active TB and is responsible for over 1.4 million deaths annually.Citation1 Against this threat, the BCG vaccine remains the only currently licensed TB vaccine approved for human use. While BCG has shown to have adequate efficacy against pediatric forms of TB, a major drawback has been its inability to protect against adult pulmonary TB.Citation2,3 The current lack of an effective vaccine necessitates the urgent development of novel vaccine strategies against TB.
Recently, DNA-based vaccines have shown promise as a therapeutic platform for treating established HPV infections, because of their ability to evoke both humoral and cell-mediated immunity.Citation4 Furthermore, the delivery of DNA vaccines, along with molecular cytokine adjuvants, by electroporation has greatly improved the effectiveness of DNA vaccines.Citation5 Together these features make DNA vaccines an ideal approach for the development of an efficacious TB vaccine. In the past several decades only a few adjuvants have been approved for human use; however, these adjuvants mainly induce humoral immunity and CD4+ T cell responses with relatively little CD8+ T cell responses.Citation6,7 Given the trending evidence that an important feature for an effective vaccine against Mtb will require both CD4+ TH1 and CD8+ T cell responses,Citation8-10 it will be imperative that new vaccine adjuvants tested induce optimal activation of both CD4+ and CD8+ T cells.
Alarmins are a unique group of endogenous molecules that initiate host defenses by inducing inflammation, activating wound healing, and perpetuating immune responses.Citation11-13 Alarmins comprise a multitude of molecules, many of which have yet to be described. However, there are several identified alarmin cytokines, which are known to differentially induce specific types of immune responses. For instance, both IL-1a and HGMB1 cytokines have been associated with promoting the generation of TH1 immune responses.Citation14,15 Specific to this paper, Interleukin 33 (IL-33), a relatively new member of the IL-1 pro-inflammatory cytokine family, has been associated with TH2-driven responses.Citation16,17 Although earlier studies have shown that IL-33 plays a role in TH2 immune responses, new confounding evidence also characterizes IL-33 as a potent TH1-polarizing alarmin.Citation18-21 Current studies show that IL-33 can contribute to the development of TH1-like CD4+ and CD8+ T cell immunity against infectious diseases and cancer.Citation20,21 We, ourselves, recently demonstrated that IL-33 could serve as an immunostimulatory molecule to generate effective immune responses by inducing potent CD4+ TH1 and CD8+ antiviral and antitumor immunity in a DNA HPV16 vaccine setting.Citation21 Moreover, a recent study by Lee et al. reported a positive correlation between IL-33 and IFNγ levels in patients with TB pleurisy.Citation22 Here, we investigated whether administration of IL-33 expressed as a DNA vaccine construct could increase the immunogenicity of an anti-TB DNA vaccine. We found that the co-immunization of IL-33 with a TB antigen 85B (Ag85B) DNA vaccine enhanced the potency of the Ag85B-specific CD4+ TH1 and CD8+ T cell responses. This data encourages further studies investigating the potential use of IL-33 as an effective immunoadjuvant for future TB DNA vaccine studies and for protection in preclinical challenge models.
Results
IL-33 enhances TB Ag85B-specific IFNγ responses after in vivo vaccination
We have previously shown that IL-33 delivered as an immunoadjuvant can induce TH1 responses in a DNA vaccine setting.Citation21 Therefore we examined whether IL-33 could increase vaccine potency when co-administered with a DNA vaccine encoding the TB antigen 85B (Ag85B). To this end, C57BL/6 mice were immunized intramuscularly with 10μg of Ag85B with or without 11μg of IL-33, immediately followed by electroporation (EP) (). One week after final immunization the magnitude of Ag85B-specific IFNγ production was measured by IFNγ ELISpot assay. As shown in , the addition of IL-33 increased the Ag85B-specific IFNγ T cell-secreting responses. Compared to the Ag85B alone-immunized group (∼333 SFC per million splenocytes), the IL-33 vaccinated group resulted in a 3-fold increase (∼1062 SFC per million splenocytes) in the frequency of Ag85B-specific responses (). These results suggest that IL-33 functions as an effective adjuvant to augment the TB antigen-specific responses during DNA vaccination.
Figure 1. IL-33 enhances TB-specific IFNγ cellular immune responses in immunized mice. (A) Immunization schedule in mice. C57BL/6 mice(n = 4) were immunized twice, with a 2-week interval between immunizations, with 10 µg Ag85B construct with or without 11 µg of IL-33 construct. (B) The total magnitude of IFNγ responses induced by isolated mice splenocytes (n = 4) stimulated ex vivo with Ag85B pooled peptides for 24 hours and measured by IFNγ ELISpot assay. The Data shows the SEM of one experiment repeated at least 2 times. **, P < 0.01
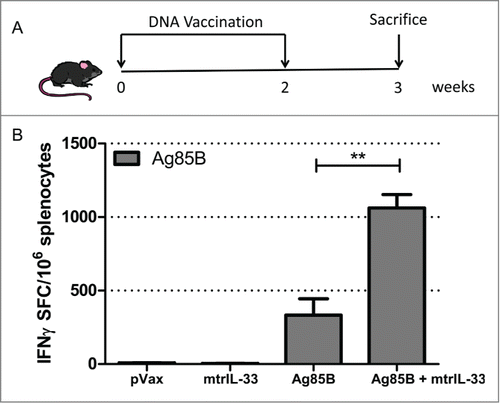
IL-33 significantly increases TB Ag85B-specific CD4+ and CD8+ splenic T cell responses
Next we were interested in whether IL-33 could enhance both the CD4+ and CD8+ T cell responses to Ag85B, so we carefully characterized the phenotype and cytokine profile of the 85B-specific T cells generated. To that end, one week after last immunization (), mural splenocytes were stimulated ex-vivo with Ag85B pooled peptides and the production of IFNγ, TNFα, and IL-2 by CD4+ and CD8+ T cells was analyzed by intracellular cytokine staining (ICS). Compared to Ag85B vaccination alone, the IL-33 adjuvant group induced significantly higher percentages of Ag85B-specific CD4+ T cells, many of which were multifunctional (). These T cells were described by total IFNγ (Ag85B: 0.8%; mtrIL-33: 1.6%), TNFα (Ag85B: 0.9%; mtrIL-33: 1.6%), and IL-2 cytokine production (Ag85B: 0.30%; mtrIL-33: 0.51%) (). As shown in , vaccination with Ag85B co-administered with IL-33 elicited substantially higher frequencies of TB-specific CD4+ T cells producing dual IFNγ+TNFα+(Ag85B: 0.43%; mtrIL-33: 0.95%) or triple-positive IFNγ+TNFα+IL-2+ (Ag85B: 0.24%; mtrIL-33: 0.47%) in the spleens (). In terms of CD8+ T cells, we saw a similar trend with the vaccinated IL-33 group, which induced higher percentages of TB-specific CD8+ T cells producing total IFNγ (Ag85B: 0.19%; mtrIL-33: 1.12%), total TNFα (Ag85B: 0.29%; mtrIL-33: 1.1%), and total IL-2 cytokine responses, although IL-2 responses were not significant (). However, we found that immunization with IL-33 predominately elicited higher frequencies of TB-specific CD8+ T cells producing dual IFNγ+TNFα+ (Ag85B: 0.12%; mtrIL-33: 0.92%)(). The administration of IL-33 produced similar amounts of Ag-specific CD4+ and CD8+ T cell responses; the majority of T cell subsets simultaneously secreted both IFNγ and TNFα. Subsequently, we analyzed the cytotoxic ability of the vaccine-induced CD8+ T cells to undergo degranulation, which was measured by staining with antibody to CD107a, a marker for degranulation. Compared to Ag85B alone-vaccinated mice (IFNγ+CD107a+: 0.2%), the CD8+ T cells isolated from mice vaccinated with IL-33 showed significantly higher percentages of Ag-specific CD8+CD107a+ T cells secreting IFNγ (IFNγ+CD107a+: 1%) (). The IL-33 immunized mice also elicited substantially higher polyfunctional CD8+ T cells co-expressing CD107+IFNγ+TNFα+(Ag85B: 0.11%; mtrIL-33: 0.91%), compared with the control groups (). Altogether, the inclusion of IL-33 can elicit robust levels of TB-specific TH1 driven cell-mediated immune responses.
Figure 2. IL-33 augments cytokine production by Ag85B-specific CD4+ (T)cells following DNA immunization. Cytokine-recall responses to TB Ag85B antigen were measured one week after last immunization by ICS and flow cytometry. (A-C), column graphs depict the total TB-specific CD4+ T cells expressing IFNγ (A), TNFα (B) and IL-2 (C). (D) Polyfunctional flow cytometry was used to determine the percentages of multifunctional CD4+ T cell cytokine profiles. The bar chart shows the percentage of Ag85B-specific CD3+CD4+ T cells displaying triple, double, or single release of the cytokines IFNγ, TNFα, and/or IL-2+. Pie charts show the proportion of each cytokine subpopulation to Ag-specific stimulation. Experiments were performed independently at least twice and data represent the mean ± SEM of 4 mice per group. *, P < 0.05 compared with Ag85B non-adjuvanted group.
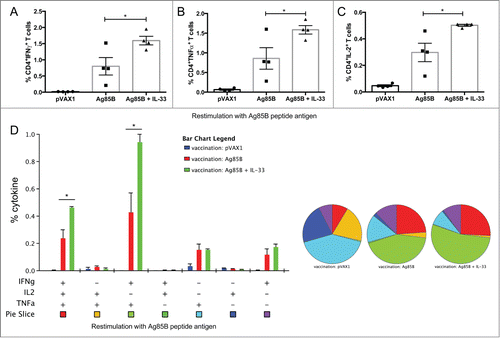
Figure 3. Induction of enhanced cytokine production of Ag85B-specific CD8+ (T)cells following DNA immunization with IL-33. Cytokine-recall responses to TB Ag85B antigen were measured one week after last immunization by ICS and flow cytometry. (A-C), column graphs depict the total TB-specific CD8+ T cells expressing total IFNγ (A), TNFα (B) and IL-2 (C). (D) Polyfunctional flow cytometry was used to determine the percentages of multifunctional CD8+ T cell cytokine profiles. The bar chart shows the percentage of Ag85B-specific CD3+CD8+ T cells displaying triple, double, or single release of the cytokines IFNγ, TNFα, and/or IL-2. Pie charts show the proportion of each cytokine subpopulation to Ag-specific stimulation. Experiments were performed independently at least twice and data represent the mean ± SEM of 4 mice per group. ***, P < 0.001; **, P < 0.01; *, P < 0.05 compared with Ag85B non-adjuvanted group.
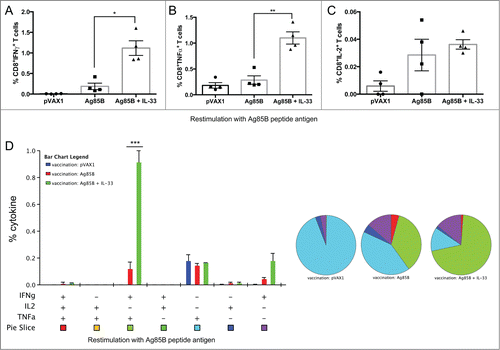
Figure 4. IL-33 promotes Ag85B-specific cytotoxic degranulating CD8+ (T)co-expressing IFNγ and TNFα. (A) Ag85B-specific, cytolytic-degranulation CD8+ T cells were measured by degranulation marker expression, CD107a and IFNγ. (B) shows the frequency of polyfunctional CD8+ T cells co-expressing CD107a. Data represent the SEM of 4 mice per group. The experiment was repeated twice with similar outcome. **P < 0.01, *P < 0.05 using Student's t-test.
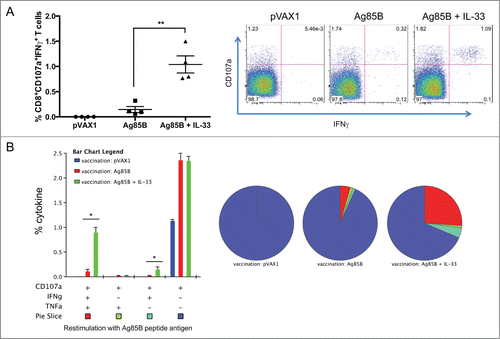
Discussion
It has been established that the development of an acquired cellular immune response (both TH1-type CD4+ and CD8+ T cells) is paramount for the control of Mtb infection. These T cell populations secrete essential cytokines (IFNγ+ and TNFα+) which activate infected macrophages to kill intracellular bacteria, which stimulate infected macrophages to kill intracellular bacteria.Citation23-25 Additionally it has been shown that knockout mice that have been genetically altered to eliminate IFNγ+ or TNFα+ production are more susceptible to mycobacterial infection.Citation23–25 Therefore, the development of new molecular adjuvants to drive these preferable anti-TB immune responses may potentially lead to a more effective vaccine against TB.
The data presented here demonstrates that IL-33 delivered as a molecular adjuvant can evoke significant Ag85B-specific cell-mediated immune responses in a TB DNA vaccine setting. We show that co-administration of IL-33 with Ag85B DNA EP immunization markedly increases the magnitude of Ag-specific IFNγ responses by ELISpot. The inclusion of IL-33 induced a ∼fold3- greater response compared with to Ag85B-alone immunization (). We find this in accordance with previous data showing that administration of IL-33 along with vaccine antigen can increase the vaccine-induced TH1 responses.Citation20,21 Furthermore, we show that IL-33 can increase the total splenic CD4+ T cells and CD8+ T cells secreting IFNγ and TNFα after Ag85B peptide pool stimulation (). Given the importance of multifunctional vaccine-induced anti-TB protective CD4+ T cell responses to prevent disease after exposure and to control Mtb in a latent state,Citation26,27 we assessed the polyfunctionality of the CD4+ T cells (). The Ag85B vaccine co-administered with IL-33 elicited a significant enhancement of the polyfunctional CD4+ T cell population simultaneously secreting both IFNγ+TNFα+ and IFNγ+TNFα+IL-2+ cytokines (). The triple positive IFNγ+TNFα+IL-2+ and double positive IFNγ+TNFα+ T cell phenotypes normally represent effector memory and central memory T cells,Citation28 indicating the induction of memory CD4+ T cell immune responses. Within the cytokine-producing CD8+ T cells, the proportional distribution of polyfunctional T cell subsets followed the order of IFNγ+TNFα+ being greater than IFNγ+ (). IL-33 not only improved the polyfunctionality of the CD8+ T cells, but also increased their antigen-specific cytolytic phenotype activity as demonstrated by the co-expression of CD107a+IFNγ+TNFα+ (). Moreover, IL-33 significantly enhanced the vaccine-induced total CD4+ T cells secreting IL-2 responses (), a cytokine that is secreted predominately by Ag-stimulated CD4+ T cells.Citation29-31 IL-2 also plays a crucial role in driving CD8+ T cell differentiation, proliferation, and activation and therefore enhancing the Ag-specific CD8+ effector functions (), such as cytolytic activity and cytokine production.Citation8,9 Altogether, the data highlight adjuvant effects of IL-33 to augment vaccine potency of both CD4 and CD8 T cells, which would be indispensible for protective immunity against TB.Citation8-10
These results are in agreement with our previously published data that demonstrated that IL-33 could enhance both CD4+ TH1 and CD8+ T cell responses with cytolytic properties.Citation21 Furthermore, this finding is consistent with the recent reports that IL-33s role extends beyond TH2 immunity; IL-33 can promote TH1 and CD8+ type cell-mediated immunity given the appropriate cytokine milieu and microenvironment.Citation16-21 Recent reports suggest that IL-33 can be a potent inducer of CD8+ T cells, as only activated effector CD8+ T cells can up-regulate the IL-33 cognate receptor, ST2, and in synergy with IL-12 can selectively enhance the expression of IFNγ responses.Citation20 The mechanism by which IL-33 promotes TH1 CD4+ and CD8+ T cell differentiation is still unknown. In our study, it is possible that the co-induction of type 1 cytokine IFNγ could be related to our selected antigen, Ag85B, which is a potent TH1 antigen.Citation32,33 Therefore, the favored TH1 cytokine milieu perpetuated by our selected TB antigen may have induced a favorable immune environment that allowed IL-33 to foster a greater TH1 cell-mediated immune response. Further studies are needed to understand exactly how IL-33 induces type-1 TH1 IFNγ+ responses in the context of an in vivo immune setting. In addition, studies are needed to understand how IL-33 bridges the innate with the adaptive immune response to evoke a pro-TH1 cell-mediated response.
Overall, in this study we further validate IL-33 as a future potent TH1- and CD8+-mediated immunoadjuvant in a DNA vaccine setting. IL-33 may be an effective strategy for increasing the efficacy of future DNA vaccines against Mtb. Since Mtb infection targets the lungs, currentstudies are evaluating the ability of IL-33 to induce polyfunctional antigen-specific T cells in the lungs. Furthermore, although IL-33 can elicit desired cell-mediated responses, it can also be detrimental to subjects at risk for HIV. The immune activation induced by IL-33 could pave the road for more efficient acquistion of HIV infection. Thus, challenge studies will be important to confirm the protective nature of IL-33 as a vaccine adjuvant in a relevant challenge system. Experiments are currently under way to test the protective efficacy of IL-33 against Mtb both as a standalone and as a prime-boost immunization regimen in combination with BCG.
Materials and Methods
DNA construct designs
The DNA constructs encoding mature IL-33 (mtrIL-33) and the TB Ag85B construct was designed as previously described.Citation21,34 The GenBank accession no. P9WQP1.1 for mouse Ag85B was used to synthesize full-length Ag85B plasmid DNA construct. All constructs had highly efficient immunoglobulin E (IgE) leader sequences inserted at the 5′ end of the gene. The constructs were commercially synthesized and optimized as described previously.Citation21,34
Animals
All animals were conducted and maintained in accordance with the NIH and the University of Pennsylvania Institutional Animal Care and Use Committee guidelines. Female C57BL/6 8-week-old mice were purchased from Jackson Laboratory (Bar Harbor, ME).
Animal immunizations
C57BL/6 mice (n = 4 per group) were immunized twice, 2 weeks apart, intramuscularly (i.m.) in the tibialis anterior muscle. In vivo electroporation (EP) was delivered, with the CELLECTRA adaptive constant current EP device (Inovio Pharmaceuticals), at the same site immediately following vaccination as previously described.Citation21,34 The mice were immunized with either 10 μg pVAX1 or 10 μg Ag85B with or without 11 μg of mtrIL-33 construct. One week after final immunization, mice were sacrificed and splenocytes were harvested to measure immune responses. All studies were repeated at least twice.
ELISPOT assay
All spleens were processed and IFNγ ELISpot assays were performed to determine antigen-specific cytokine secretion. Briefly, spleens were collected in RPMI 1640 medium (supplemented with 10% FBS, 1X Antibiotic-Antimycotic, and 1X β-ME) and splenocytes were isolated by mechanical disruption of the spleen using a Stomacher machine (Seward Laboratory Systems, Bohemia, NY). The resulting mashed spleens were filtered using a 40μm cell strainer, treated with ACK lysis buffer for 5 minutes to lyse RBCs, washed in PBS and then re-suspended in RPMI medium for use in ELISpot or Flow Cytometry assay. The IFNγ ELISPOT assays were conducted as previously described in detail.Citation21,34 Ag85B-specific T cell responses were measured by stimulating splenocytes with pooled peptides (11-mers overlapping by 8 amino acids; 2.5 μg/ml final) spanning the entire TB Ag85B antigen (Invitrogen). All peptides were synthesized from GenScript. Concavalin A (Sigma-Aldrich, St. Louis, MO) was used as positive control and complete culture medium was used as negative control. Background staining from cells stimulated with medium alone has been subtracted. Spots were enumerated using an automated ELISPOT reader (Cellular Technology, Shaker Heights, OH).
Intracellular cytokine staining flow cytometry
Intracellular cytokine staining was performed after 5 hours of ex vivo stimulation with Ag85B pooled peptides as described.Citation21,34,35 In cultures being used to measure degranulation, anti-CD107a (FITC; clone 1D4B; Biolegend) was added during time of stimulation to capture the degranulation induced by exposure to antigen stimulation by Ag-specific cells. The cells were then fixed and stained as described elsewhere.Citation21,34,35 The following antibodies were used for surface staining: LIVE/DEAD Fixable Violet Dead Cell stain kit (Invitrogen), CD4 (V500; clone RM4–5; BD Biosciences), CD8 (APC-Cy7; clone 53–6.7; BD Biosciences). For intracellular staining the following antibodies were used: IFNγ (APC; clone XMG1.2; Biolegend), TNFα (PE; clone MP6-XT22; ebioscience), CD3 (PerCP/Cy5.5; clone 145–2C11; Biolegend), and IL-2 (PeCy7; clone JES6-SH4; ebioscience). All data was collected using a LSRII flow cytometer (BD Biosciences) and analyzed using FlowJo software (Tree Star, Ashland, OR) with SPICE v5.3 (free available from http://exon.niaid.nih.gov/spice/). Boolean gating was performed using FlowJo software to examine the polyfunctionality of the T cells from vaccinated animals. For flow cytometry, cells were gated on singlets using SSC-H by SSC-A followed by gating on LIVE-DEAD, CD3+CD4+CD8− T and CD3+CD8+CD4− T cells to examine the CD4+ and CD8+ T-cell populations.
Statistical analysis
Group analyses were completed by matched, 2-tailed, unpaired student's t-tests to analyze statistical significance of all quantitative data produced in this study. A P < 0 .05 was considered statistically significant. All values are mean ± SEM and statistical analyses were performed using GraphPad Prism (La Jolla, CA).
Disclosure of Potential Conflicts of Interest
D.B.W. has grant funding, participates in industry collaborations, receives speaking honoraria, and collects fees for consulting. He serves on scientific review committees and advisory boards. Remuneration includes direct payments, stock, or stock options. In the interest of disclosure, he therefore notes potential conflicts associated with this work with Pfizer, Bristol Myers Squibb, Inovio, Touchlight, oncosec, Merck, VGXI, and possibly others. Licensing of technology from his laboratory has created over 100 jobs in the private sector in the biotech/pharma industry. The other authors declare no competing financial interests.
Acknowledgments
We would like to acknowledge all members of the Weiner Laboratory for contributions and/or critical reading of this manuscript. The authors also thank Penn CFAR and ACC core facilities for their support.
Funding
This research was supported by Inovio Pharmaceuticals and Aeras.
References
- WHO. Global tuberculosis report 2014. Geneva: World Health Organization, 2014. http://www.who.int/tb/publications/global_report/en/
- Fine PE. Variation in protection by BCG: implications of and for heterologous immunity. Lancet 1995; 346:1339-45; PMID:7475776; http://dx.doi.org/10.1016/S0140-6736(95)92348-9
- Trunz BB, Fine P, Dye C. Effect of BCG vaccination on childhood tuberculosis meningitis and military tuberculosis worldwide: a meta-analysis and assessment of cost-effectiveness. Lancet 2006; 367:1173-80; PMID:16616560; http://dx.doi.org/10.1016/S0140-6736(06)68507-3
- Bagarazzi ML, Yan J, Morrow MP, Shen X, Parker RL, Lee JC, Giffear M, Pankhong P, Khan AS, Broderick KE, et al. Immunjournalapy against HPV16/18 generates potent TH1 and cytotoxic cellular immune responses. Sci Transl Med 2012; 4:155; PMID:23052295; http://dx.doi.org/10.1126/scitranslmed.3004414
- Kalams SA, Parker SD, Elizaga M, Metch B, Edupuganti S, Hural J, De Rosa S, Carter DK, Rybczyk K, Frank I, et al. Safety and comparative immunogenicity of an HIV-1 DNA vaccine in combination with plasmid interleukin 12 and impact of intramuscular electroporation for delivery. J Infect Dis 2013; 208:818-29; PMID:23840043; http://dx.doi.org/10.1093/infdis/jit236
- Reed SG, Orr MT, Fox CB. Key roles of adjuvants in modern vaccines. Nat Med 2013; 19:1597-608; PMID:24309663; http://dx.doi.org/10.1038/nm.3409
- Schijns VEJC, Lavelle EC. Trends in vaccine adjuvants. Expert Rev 2011; 10(4):539-50; PMID:21506650; http://dx.doi.org/10.1586/erv.11.21
- Cooper AM. Cell-mediated immune responses in tuberculosis. Annu Rev Immunol 2009; 27:393-422; PMID:19302046; http://dx.doi.org/10.1146/annurev.immunol.021908.132703
- Prezzemolo T, Guggino G, Pio la Manna M, Di Liberto D, Dieli F, Caccamo N. Functional signature of human CD4 and CD8 T cell responses to Mycobacterium tuberculosis. Front Immunol 2014; 5:180; PMID:24795723; http://dx.doi.org/10.3389/fimmu.2014.00180
- Andersen P, Woodworth JS. Tuberculosis vaccines – rethinking the current paradigm. Trends Immunol 2014; 35:387-95; PMID:24875637; http://dx.doi.org/10.1016/j.it.2014.04.006
- Oppenheim JJ, Yang D. Alarmins: chemotactic activators of immune responses. Curr Opin Immunol 2005; 17:359-65; PMID:15955682; http://dx.doi.org/10.1016/j.coi.2005.06.002
- Bianchi ME. DAMPs, PAMPs and alarmins: all we need to know about danger. J Leukoc Biol 2007; 81:1-5; PMID:17032697; http://dx.doi.org/10.1189/jlb.0306164
- Chan JK, Roth J, Oppenheim JJ, Tracey KJ, Vogl T, Feldmann M, Horwood N, Nanchahal J. Alarmins: awaiting a clinical response. J Clin Invest 2012; 122:2711-9; PMID:22850880; http://dx.doi.org/10.1172/JCI62423
- Rovere-Querini P, Capobianco A, Scaffidi P, Valentinis B, Catalanotti F, Giazzon M, Dumitriu IE, Müller S, Iannacone M, Traversari C, et al. HMGB1 is an endogenous immune adjuvant released by necrotic cells. EMBO Rep 2004; 5:825-30; PMID:15272298; http://dx.doi.org/10.1038/sj.embor.7400205
- Flohe SB, Bruggemann J, Lendemans S, Nikulina M, Meierhoff G, Flohe S, Kolb H. Human heat shock protein 60 induces maturation of dendritic cells versus a Th1-promoting phenotype. J Immunol 2003; 170:2340-8; PMID:12594256; http://dx.doi.org/10.4049/jimmunol.170.5.2340
- Liew FY, Pitman NI, McInnes IB. Disease-associated functions of IL- 33: the new kid in the IL-1 family. Nat Rev Immunol 2010; 10:103-10; PMID:20081870; http://dx.doi.org/10.1038/nri2692
- Haraldsen G, Balogh J, Pollheimer J, Sponhiem J, Kuchler. Interleukin- 33—cytokine of dual function or novel alarmin? Trends Immunol 2009; 30:227-33; PMID:19359217; http://dx.doi.org/10.1016/j.it.2009.03.003
- Villarreal DO, Weiner DB. Interleukin 33: a switch-hitting cytokine. Curr Opin Immunol 2014; 28:102-6; PMID:24762410; http://dx.doi.org/10.1016/j.coi.2014.03.004
- Milovanovic M, Volarevic V, Radosavljevic, Jovanovic I, Pejnovic N, Arsenijevic N, Lukic ML. IL-33/ST2 axis in inflammation and immunopathology. Immunol Res 2012; 52:89-99; PMID:22392053; http://dx.doi.org/10.1007/s12026-012-8283-9
- Bonilla WV, Frohlich A, Senn K, Kallert S, Fernandez M, Johnson S, Kreutzfeldt M, Hegazy AN, Schrick C, Fallon PG, et al. The alarmin interleukin-33 drives protective antiviral CD8(þ) T cell responses. Science 2012; 335:984-9; PMID:22323740; http://dx.doi.org/10.1126/science.1215418
- Villarreal DO, Wise MC, Walters JN, Reuschel EL, Choi MJ, Obeng-Adjei N, Yan J, Morrow MP, Weiner DB. Alarmin IL-33 acts as an immunoadjuvant to enhance antigen-specific tumor immunity. Cancer Res 2014; 74:6; PMID:24448242; http://dx.doi.org/10.1158/0008-5472.CAN-13-2729
- Lee K-S, Kim H-R, Kwak S, Choi KH, Cho JH, Lee YJ, Lee MK, Lee JH, Park SD, Park DS. Association between elevated pleural interleukin-33 levels and tuberculous pleurisy. Ann Lab Med 2013; 33:45-51; PMID:23301222; http://dx.doi.org/10.3343/alm.2013.33.1.45
- Cooper AM Dalton DK, Stewart TA, Griffin JP, Russell DG, Orme IM. Disseminated tuberculosis in interferon gamma gene-disrupted mice. J Exp Med 1993; 168:1322-7; PMID:8245795
- Flynn JL, Chan J, Triebold J, Dalton DK Stewart TA, Bloom BR. An essential role for interferon gamma in resistance to Mycobacterium tuberculosis infection. J Exp Med 1993; 178:2249-54; PMID:7504064; http://dx.doi.org/10.1084/jem.178.6.2249
- Flynn JL, Goldstein MM, Chan J, Triebold KJ, Pfeffer K, Lowenstein CJ, Schreiber R, Mak TW, Bloom BR. Tumor necrosis factor-α Is required in the protective immune response against mycobacterium tuberculosis in mice. Immunity 1995; 2:561-72; PMID:7540941; http://dx.doi.org/10.1016/1074-7613(95)90001-2
- Derrick SC, Yabe IM, Yang A, Morris SL. Vaccine-induced anti-tuberculosis protective immunity in mice correlates with the magnitude and quality of multifunctional CD4 T cells. Vaccine 2011; 29:2902-9; PMID:21338678; http://dx.doi.org/10.1016/j.vaccine.2011.02.010
- Derrick SC, Repique C, Snoy P, Yang AL, Morris S. Immunization with a DNA vaccine cocktail protects mice lacking CD4 cells against an aerogenic infection with Mycobacterium tuberculosis. Infect Immun 2004; 72:1685-92; PMID:14977976; http://dx.doi.org/10.1128/IAI.72.3.1685-1692.2004
- Darrah PA, Patel DT, De Luca PM, Lindsay RW, Davey DF, Flynn BJ, Hoff ST, Andersen P, Reed SG, Morris SL, et al. Multifunctional Th1 cells define a correlate of vaccine-mediated protection against Leishmania major. Nat Med 2007; 13:843-850; PMID:17558415; http://dx.doi.org/10.1038/nm1592
- Boyman O, Sprent J. The role of interleukin-2 during homeostasis and activation of the immune system. Nat Rev Immunol 2012; 12:180-190; PMID:22343569
- Malek TR, Castro I. Interleukin-2 receptor signaling: at the interface between tolerance and immunity. Immunity 2010; 33:153-165; PMID:20732639; http://dx.doi.org/10.1016/j.immuni.2010.08.004
- Liao W, Lin JX, Leonard WJ: Interleukin-2 at the crossroads of effector responses, tolerance, and immunotherapy. Immunity 2013; 38:13-25; PMID:23352221; http://dx.doi.org/10.1016/j.immuni.2013.01.004
- Grover A, Ahmed MF, Singh B, Verma I, Sharma P, Khuller GK. A multivalent combination of experimental antituberculosis DNA vaccines based on Ag85B and regions of difference antigens. Microbes Infect 2006; 8:2390-9; PMID:16962360; http://dx.doi.org/10.1016/j.micinf.2006.04.025
- Weinrich Olsen A, van Pinxteren LA, Meng Okkels L, Birk Rasmussen P, Andersen P. Protection of mice with a tuberculosis subunit vaccine based on a fusion protein of antigen 85B and ESAT-6. Infec Immun 2001; 69:2773-2778; PMID:11292688; http://dx.doi.org/10.1128/IAI.69.5.2773-2778.2001
- Villarreal DO, Walters J, Laddy DJ, Yan J, Weiner DB. Multivalent TB vaccines targeting the esx gene family generate potent and broad cell-mediated immune responses superior to BCG. Hum Vaccin Immunother 2014; 10(8); PMID:25424922; http://dx.doi.org/10.4161/hv.29574. [Epub ahead of print]
- Yan J, Villarreal DO, Racine T, Chu JS, Walters JN, Morrow MP, Khan AS, Sardesai NY, Kim JJ, Kobinger GP, et al. Protective immunity to H7N9 influenza viruses elicited by synthetic DNA vaccine. Vaccine 2014; 32:2833-42; PMID:24631084; http://dx.doi.org/10.1016/j.vaccine.2014.02.038