Abstract
Vaccines containing multiple antigens may induce broader immune responses and provide better protection against Mycobacterium tuberculosis (Mtb) infection as compared to a single antigen. However, strategies for incorporating multiple antigens into a single vector and the immunization routes may affect their immunogenicity. In this study, we utilized recombinant adenovirus type 5 (rAd5) as a model vaccine vector, and Ag85A (Rv3804c) and Mtb32 (Rv0125) as model antigens, to comparatively evaluate the influence of codon usage optimization, signal sequence, fusion linkers, and immunization routes on the immunogenicity of tuberculosis (TB) vaccine containing multiple antigens in C57BL/6 mice. We showed that codon-optimized Ag85A and Mtb32 fused with a GSG linker induced the strongest systemic and pulmonary cell-mediated immune (CMI) responses. Strong CMI responses were characterized by the generation of a robust IFN-γ ELISPOT response as well as antigen-specific CD4+ T and CD8+ T cells, which secreted mono-, dual-, or multiple cytokines. We also found that subcutaneous (SC) and intranasal (IN)/oral immunization with this candidate vaccine exhibited the strongest boosting effects for Mycobacterium bovis bacille Calmette-Guérin (BCG)-primed systemic and pulmonary CMI responses, respectively. Our results supported that codon optimized Ag85A and Mtb32 fused with a proper linker and immunized through SC and IN/oral routes can generate the strongest systemic and pulmonary CMI responses in BCG-primed mice, which may be particularly important for the design of TB vaccines containing multiple antigens.
Abbreviations:
- Mtb, Mycobacterium tuberculosis
- TB, tuberculosis
- BCG, Mycobacterium bovis bacille Calmette-Guérin
- rAd5, recombinant adenovirus type 5
- CMI, cell-mediated immune responses
- IM, intramuscular
- IN, intranasal
- SC, subcutaneous
- IFN-γ, interferon gamma
- ELISPOT, Enzyme-linked immune-sorbent spot
- SFC, spot-forming cells
- tPA, tissue plasminogen activator
- HA tag, hemagglutinin tag
- SDS-PAGE, sodium dodecyl sulfate polyacrylamide gel electrophoresis
- IL-2, Interleukin 2
- TNF-α, tumor necrosis factor α
- ICS, Intracellular cytokine staining
- PCR, polymerase chain reaction
- HEK, human embryo kidney
- PBS, Phosphate Buffered Saline
- NBT/BCIP, Nitro blue tetrazolium/ 5-Bromo-4-chloro-3-indolyl phosphate
- DMSO, Dimethyl sulfoxide
- BSA, bovine serum album
- FITC, fluorescein isothiocyanate
- PE, Phycoerythrin
- DAPI, 4′,6-diamidino-2-phenylindole
- RPMI, Roswell Park Memorial Institute
- APC, Allophycocyanin
- PerCP, Peridinin-ChlorophylL-Protein Complex
- FACS, Fluorescence Activated Cell Sorter
- FBS, fetal bovine serum
- vp, viral particles
Introduction
Tuberculosis (TB) remains one of the most threatening infectious diseases in the world. Approximately 8.6 million newly-infected cases and 1.3 million deaths were recorded in 2012.Citation1 Although Mycobacterium bovis bacille Calmette-Guérin (BCG), the only available vaccine against Mycobacterium tuberculosis (Mtb) infection, provides effective protection against the most severe form of childhood TB, its protection efficacy against adult pulmonary TB is variable.Citation2,3 With the emergence and increased prevalence of drug-resistant TB strains, the development of new vaccines that can either replace BCG or boost and prolong protection efficacy are urgently needed.
Several TB vaccine candidates are currently undergoing clinical trials. Among these, the recombinant viral vector-based vaccines AdAg85a, MV85A, and Aeras-402 (Crucell Ad35), showed some promising potentials in inducing cell-mediated immune (CMI) responses.Citation4-6 Although Ag85A, a component of Mtb mycolyl transferase complex, has shown to be immune-dominant and protective in animal models, the disappointing results of the recent MVA85A trial has raised the issue of incorporating multiple antigens into TB vaccines.Citation7,8 However, the strategies utilized for constructing fusion antigens varied widely. AERAS-402 is a recombinant adenoviral vector harboring a fused coding sequence of 3 Mtb proteins, Ag85A, Ag85B, and TB10.4, whose codon usage was optimized and native signal sequences were deleted.Citation9 However, V-2A, a multicistronic DNA vaccine, contained the wild-type coding sequence of Rv3407, Ag85A, and HspX linked with the self-cleavage 2A peptide and the native signal sequence was replaced with that of human tissue plasminogen activator (tPA).Citation10 These strategies were also applied to the construction of other genetic vector based TB vaccines.Citation11,12 However, the influence of codon optimization, fusion linker, and signal sequence on the immunogenicity of vaccines containing multiple TB antigens has not been fully determined.
The immunization route also affects the immunogenicity and protective efficiency of a TB vaccine, especially in the context of viral vector based vaccines.Citation13 Parenteral administration of DNA- or viral-vectored TB vaccines reduced the bacterial load in the spleen rather than in the lung, due to the lack of specific T cells in the lung.Citation14-17 Mucosal delivery of adenoviral-based vaccines generated specific T cells, especially CD8+ T cells, in the lung and thus provided protection against pulmonary TB.Citation18 Furthermore, airway exposure to Mtb antigens or nonspecific inflammatory agonists recruited and retained systemically activated specific T cells.Citation19 Therefore, it is desirable to understand the systemic and pulmonary immune responses that are boosted by a candidate TB vaccine containing multiple antigens through various delivery routes after BCG immunization.
In this study, we evaluated the influence of codon usage optimization, signal sequence, fusion linker, and delivery routes on the immunogenicity of TB vaccine candidates containing multiple antigens using 2 previously reported Mtb antigens, Ag85A and Mtb32,Citation20,21 and the replication-defective Ad5 vector.
Results
Construction and characterization of rAd5 vectors carrying 4 forms of Ag85A-Mtb32 fusion antigens
To evaluate the influence of codon usage optimization, signal sequence, and fusion linker on the immunogenicity of Ag85A-Mtb32 fusion antigens, 4 forms of Ag85A-Mtb32 fusion (AM) genes harbored in rAd5 vectors were generated (). Ad5-wtAM carried Ag85A-Mtb32 with wild-type sequences, consisting of tPA signal sequence, HA tag, Ag85A, 2A auto-cleavage linker, Mtb32, and Flag tag (from Napos; to COOHapos; termini). The 2A linker facilitates the post-translational cleavage of the fusion protein into separate Ag85A and Mtb32 peptides (); Ad5-optAM was assembled in an analogous fashion as Ad5-wtAM except that the coding sequences of Ag85A and Mtb32 were optimized. Based on Ad5-optAM, Ad5-gsgAM and Ad5-ΔsAM were constructed with the replacement of 2A linker with GSG linker and the deletion of the tPA signal sequence, respectively.
Figure 1. Construction and Characterization of Recombinant Ad5 Vectors Carrying Four Forms of Ag85A-Mtb32 Fusion Genes. (A). Schematic presentation of Ad5 vectors carrying 4 forms of Ag85A-Mtb32 fusion genes. An HA tag and a Flag tag were added to the Napos; terminus of Ag85A and the COOHapos; terminus of Mtb32 for expression detection. (B). The function mechanism of 2A auto-cleavage peptide in the expression of Ag85A-Mtb32 fusion antigens. (C). The expression of Ag85A and Mtb32 by recombinant Ad5 vectors. Vero cells were infected with Ad5-optAM (Lane 1, 2), Ad5-wtAM (Lane 3, 4), Ad5-gsgAM (Lane 5,6), Ad5-ΔsAM (Lane 7, 8) and a control vector Ad5-Empty (Lane 9,10), and the cell lysates (Lane 1, 3, 5, 7, 9) and the culture medium (Lane 2, 4, 6, 8, 10) were subjected to SDS-PAGE followed by western blot analysis using anti-HA tag (upper), anti-Flag tag (middle), and anti-β actin antibodies (bottom).
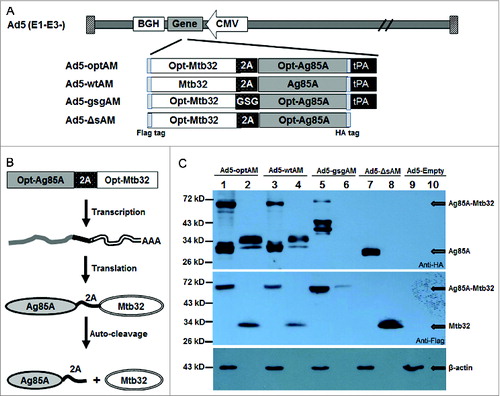
To confirm the expression of these AM fusion antigens, Vero cells were infected with these vectors using equivalent viral particles and a rAd5-empty was used as negative control. The cell lysates and culture medium were collected and subjected to SDS-PAGE and subsequent western blot analysis. The results showed that all 4 fusion antigens were efficiently expressed. The expression levels of optAM () were higher than wtAM () in either cell lysates or culture medium. The gsgAM underwent partial degradation (). In the absence of tPA signal, Ag85A was primarily retained in the cytoplasm as expected (). Surprisingly, a band with a molecular weight similar to that of the Ag85A-Mtb32 fusion protein appeared in the lysates of Ad5-wtAM- and Ad5-optAM-infected cells, indicating that the auto-cleavage effects of 2A were not 100% in the presence of tPA signal (). Most of the separated Mtb32 was secreted into the culture medium due to the presence of a natural signal sequence (). Taken together, the 4 Ag85A-Mtb32 fusion antigens were all efficiently expressed by recombinant Ad5 vectors in infected cells.
To determine whether the subcellular localization of Ag85A and Mtb32 was altered by the various fusion strategies, Vero cells were infected with these vectors and subjected to immunofluorescence assay. The results showed that Ag85A and Mtb32, either as separated or as fused proteins, were co-localized primarily in the cytoplasm (), indicating that codon optimization, signal sequence, and protein linker did not significantly alter the subcellular distribution patterns of Ag85A and Mtb32.
Figure 2. Subcellular Localization of Ag85A and Mtb32. Vero cells were seeded and infected with respective rAd5 vectors carrying different forms of Ag85A-Mtb32 fusion genes, an Ad5-Empty was used as a control. After 48 hours, the cells were labeled with FITC-labeled anti-Flag antibody and PE-labeled anti-HA antibody and subsequently stained with DAPI. Images were viewed and photographed using a fluorescence microscope.
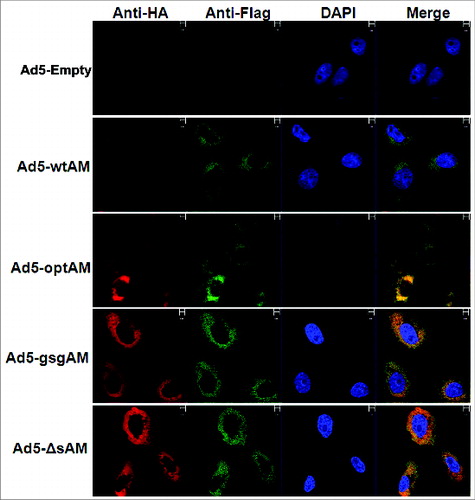
Immunization with gsgAM generated the highest level of IFN-γ ELISPOT responses in both the spleen and the lung
To compare the immunogenicity of the 4 forms of Ag85A-Mtb32 fusion antigens, C57BL/6 mice were immunized intramuscularly followed by an intranasal boost ( and ). Two weeks after prime or boost, the splenocytes and lung lymphocytes were isolated and subjected to IFN-γ ELISPOT analysis. The results showed that all 4 Ag85A-Mtb32 fusions generated robust CMI responses in both the spleen and the lung. gsgAM generated the strongest cellular responses in the spleen at 2 weeks after prime, compared to the other 3 fusion antigens (). The Ag85A in ΔsAM induced significantly more spot-forming splenocytes than in optAM (, p < 0.05). The Ag85A in wtAM and optAM induced a comparable response, as did the Mtb32 in wtAM, optAM, and ΔsAM (). However, a single IM injection of any Ag85A-Mtb32 fusion vaccines was unable to generate any obvious IFN-γ+ spot-forming cells in the lung (). Interestingly, after an intranasal boost immunization, the IFN-γ+ spot-forming cells in the spleen were not expanded in any groups (), while robust Ag85A- and Mtb32-specific IFN-γ+ spot-forming cells were generated in the lung (). Similar to the case in the spleen, gsgAM induced the strongest specific responses for both Ag85A and Mtb32 in the lung (, p < 0.01). These data indicated the following: 1) codon usage optimization in general did not significantly enhance immune responses with the exception for the Mtb32 specific response in the lung after IN boost (p < 0.05); 2) deletion of the signal sequence did not significantly affect antigen-specific CMI responses after IM prime and boost; 3) gsgAM induced the strongest cell mediated IFN-γ ELISPOT responses in both the spleen and the lung after IM prime or after IN boost immunizations.
Figure 3. Ag85A and Mtb32 Specific IFN-γ+ Spot-forming Cells Generated by Various Ag85A-Mtb32 Fusion Antigens. (A). The schedule of immunization and detection. (B). The frequency of IFN-γ+ spot-forming splenocytes at 2 weeks after IM prime. (C). The frequency of IFN-γ+ spot-forming lung lymphocytes at 2 weeks post IM prime. (D). The frequency of IFN-γ+ spot-forming splenocytes at 2 weeks post IN boost. (E). The frequency of IFN-γ+ spot-forming lung lymphocytes at 2 weeks post IN boost. C57BL/6 mice were immunized with control Ad5-empty or rAd5 vectors carrying Ag85A-Mtb32 fusion genes using an IM prime plus IN boost strategy. At 2 weeks after prime or boost, splenocytes and lung lymphocytes were harvested and cultured with peptide pools for each antigen and subjected to an IFN-γ ELISPOT assay. The data were analyzed by 2-way ANOVA. The bars represent the standard error of the mean. *p < 0.05; **p < 0.01; ***p < 0.001.
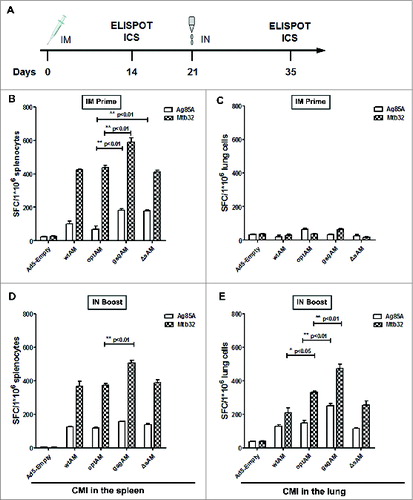
Table 1. Immunization and detection schedule for the evaluation of Ad5 vectors carrying various Ag85A-Mtb32 fusion antigens
Table 2. Schedule for Ad5-gsgAM boost following BCG primary immunization
Cytokine secretion profiling of antigen-specific CD4+ and CD8+ T cells induced by immunization with Ag85A-Mtb32 fusions
To evaluate the quantity and quality of cytokine-producing CD4+ T and CD8+ T cells induced by Ag85A-Mtb32 fusions, splenocytes and lung lymphocytes were stimulated with Ag85A and Mtb32 peptide pools. The production of IFN-γ, TNF-α, and IL-2, the major Type 1 cytokines important for the suppression and clearance of Mtb infection,Citation22,23 were analyzed using intracellular cytokine staining (ICS). The results showed that all 4 Ag85A-Mtb32 fusion antigens induced significant Ag85A- and Mtb32-specific CD4+ and CD8+ T cells, both in the spleen and in the lung (). Primary immunization with all fusion antigens via IM injection generated low level of antigen specific CD4+ T cells and CD8+ T cells in the spleen but not in the lung (data not shown). After IN boost, antigen-specific CD4+ T and CD8+ T cell responses were significantly elevated, not only in the spleen but also in the lung (). gsgAM appeared to generate the highest levels of Ag85A-specific CD4+ T cells and Mtb32-specific CD8+ T cells in both the spleen and the lung, while ΔsAM was showed to elicit more Mtb32-specific CD4+ and CD8+ T cells than optAM only in the spleen. Interestingly, a significant proportion of antigen-specific CD4+ T and CD8+ T cells secreted dual or triple cytokines (, pie charts). These results indicate the following: 1) gsgAM generated the strongest Ag85A-specific CD4+ and Mtb32-specific CD8+ T cell responses in both the spleen and the lung after IN boost; 2) a significant proportion of antigen-specific CD4+ and CD8+ T cells secreted dual- or triple cytokines, which may be especially important for the protective function of TB vaccines.Citation24-26
Figure 4. Cytokine secretion profiling of Antigen-specific CD4+ and CD8+ T Cells. C57BL/6 mice were immunized with Ad5 vectors carrying Ag85A-Mtb32 fusion genes using an IM prime followed by IN boost immunization strategy as shown in . At 2 weeks after boost immunization, splenocytes (A and C) and lung lymphocytes (B and D) were harvested and cultured with peptide pools for each antigen. A flow cytometry assay based on intracellular cytokine staining was used to quantify antigen-specific cytokine-secreting CD4+ and CD8+ T cells. The percentage of Ag85A specific CD4+ T cell and Mtb32 specific CD8+T cell which secreted mono-(dark blue), dual-(green), and triple-(red) cytokines are shown in the pie charts. The data represents one of the 2 independent experiments.
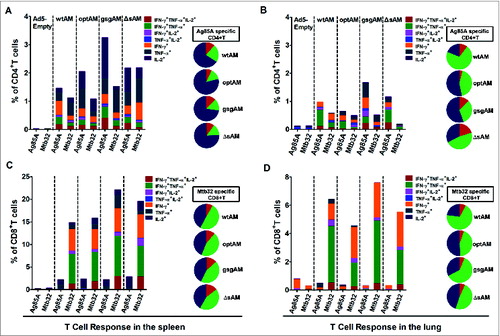
SC and IN/oral inoculations with gsgAM can efficiently boost BCG-primed systemic and pulmonary CMI responses
Next, we sought to determine the delivery route through which gsgAM can most efficiently boost the CMI responses induced by BCG immunization. C57Bl/6 mice were subcutaneously primed with BCG and then boosted with gsgAM through IM, SC, oral, and IN routes. Splenocytes and lung lymphocytes were isolated and subjected to IFN-γ ELISPOT and ICS assays (Table 2). ELISPOT assay showed that splenic CMI responses were significantly boosted through IM and SC immunization, with the SC route generating the strongest response (, p < 0.001 for Ag85A, and p < 0.01 for Mtb32). Only moderate splenic responses were boosted by oral and IN immunization with gsgAM (). Robust Mtb32-specific responses were boosted by oral and IN immunization in the lung (). Interestingly, SC immunization was able to boost moderate but statistically significant antigen specific responses in the lung (, p < 0.05).
Figure 5. Boosting Effects of Ad5-gsgAM on BCG-primed Cell-mediated Immune Responses through Various Immunization Routes. (A). The frequency of IFN-γ spot-forming splenocytes specific for Ag85A and Mtb32 at 2 weeks post-boost. (B). The frequency of IFN-γ spot-forming lung lymphocytes specific for Ag85A and Mtb32 at 2-weeks post boost. (C). The percentage of antigen-specific cytokine-secreting CD4+ T cells of total CD4+ T cells in the spleen. (D). The percentage of antigen-specific cytokine-secreting CD4+ T cells of total CD4+ T cells in the lung. (E). The percentage of antigen-specific cytokine-secreting CD8+ T cells of total CD8+ T cells in the spleen. (F). The percentage of antigen-specific cytokine-secreting CD8+ T cells of total CD8+ T cells in the lung. C57BL/6 mice were first primed with BCG and then immunized with Ad5-gsgAM through 4 routes: IM, SC, IN, and oral. Splenocytes and lung lymphocytes were isolated and subjected to IFN-γ ELISPOT assay and intracellular cytokine staining followed by flow cytometry analysis. The data represents one of 2 independent experiments.
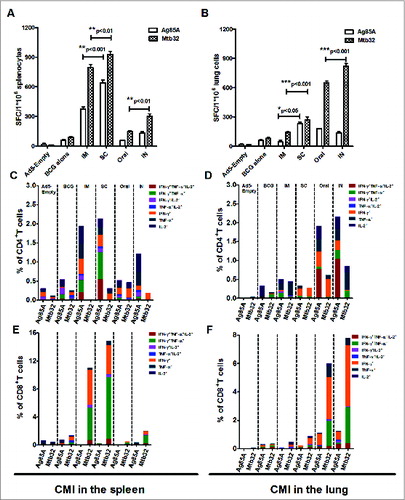
The results of the ICS assay showed a trend similar to that observed in the ELISPOT assay. Robust Ag85A- and Mtb32-specific CD4+ T cells were induced in the spleen by IM and SC boost, which were able to produce single, dual, and even triple cytokines (). CD8+ T cells in the spleen were mainly directed to Mtb32, which produced IFN-γ, IFN-γ and TNF-α, as well as triple cytokines (). No significant Mtb32-specific CD4+ and CD8+ T cells were elicited in the spleen by either IN or oral immunization (). Moreover, a high frequency of Ag85A-specific CD4+ and Mtb32-specific CD8+ T cells secreting cytokines were generated in the lung by oral and IN immunization ().
These data indicate that although primary immunization with BCG only generated a moderate CMI response, a booster immunization with Ad5 vectored vaccine carrying a Ag85A-Mtb32 fusion antigen via IM and SC injections could significantly boost systemic immune responses. Moreover, a booster immunization via mucosal routes, such as oral and IN inoculations, could significantly boost immune responses in the lung.
Discussion
Incorporating multiple antigens into TB vaccine candidates could be beneficial for generating broader immune responses.Citation7 There are various strategies for constructing a fusion antigen.Citation5,7,10,11 The influence of codon usage optimization, signal sequence, fusion linker, and immunization routes on the immunogenicity of fusion antigens has not yet been well defined. In this study, by using the 2 Mtb antigens Ag85A and Mtb32 as model antigens, we compared the immunogenicity of 4 Ag85A-Mtb32 fusions to induce systemic and pulmonary immune responses. We found that: 1) the subcellular localizations of Ag85A and Mtb32 were not significantly altered by the various fusion strategies; 2) codon usage optimization slightly elevated the expression level of Ag85A-Mtb32 fusion protein in Ad5 infected cells, but not the immunogenicity in the animals; 3) deletion of the secretory signal peptide slightly enhanced the immunogenicity of Ag85A; 4) gsgAM fused by a GSG linker elicited stronger CMI responses than separated Ag85A and Mtb32 generated by a 2A auto-cleavage. Moreover, we also found that SC and IN immunizations with gsgAM efficiently boosted specific CMI responses primed by BCG in the spleen and lung respectively.
Codon optimization of the wild-type coding sequence has been reported to significantly improve antigen expression and thereby enhance immunogenicity, especially in a heterologous expression system.Citation27–29 However, our results showed that the expression of Ag85A and Mtb32 with codons optimized for mammalian usage was only slightly elevated (), and that immunogenicity was not significantly enhanced (). We found that almost 65% and 53% of codons in wild-type sequences encoding Ag85A and Mtb32, respectively, were also the preferred codons used in mammalian genes, which may partly explain why a complete codon optimization did not dramatically enhanced the level of protein expression.
Ag85A and Mtb32 are both secreted proteins with native secretory signal peptides. However, these signal sequences were either deleted or replaced by tPA signal sequence when incorporated into DNA or viral vectors.Citation7,9,10,15,21,30 In this study, we compared the antigen protein expression and immunogenicity of codon-optimized Ag85A with or without tPA signal sequence. The subcellular localization of Ag85A and Mtb32 were not obviously altered. Similar as a previous report,Citation31 the secretory signal sequence appeared to weaken the cleavage efficiency of the 2A linker which resulted in the presence of a proportion of uncleaved Ag85A-Mtb32 fusion (). Although more Ag85A-specific IFN-γ+ spot-forming splenocytes were generated by ΔsAM after primary immunization (, p < 0.01), the specific CD4+T and CD8+T cell response were not significantly elevated by the deletion of signal sequence (). Therefore, the deletion of secreted signal only showed slight enhancement, if any, on the immunogenicity of Ag85A.
Protein linkers can have an important role in the design of fusion antigens expressed from a single cassette. Proper linkers may improve the folding, stability, bioactivity, and expression level of fusion antigens.Citation10,32,33 Here, we comparatively studied 2 protein linkers, GSG linker and auto-cleavage 2A linker, which were used in optAM and gsgAM, respectively. gsgAM induced a significantly higher level of CMI responses, which were characterized by a stronger IFN-γ ELISPOT response and more antigen-specific CD4+ and CD8+ T cells in both the spleen and the lung (, compared gsgAM with optAM immunization group). Therefore, GSG linker was better than 2A linker for Ag85A and Mtb32 fusion antigen in the respect of generating stronger systemic and pulmonary CMI response. However, whether GSG linker is better than 2A linker in the construction of other fusion antigens and the mechanism behind this phenomenon need to be evaluated in the future study.
We observed that the Ad5 vectored Ag85A appeared to largely induce CD4+ T cell response and the Mtb32 largely induce CD8+ T cell response (), which is in accordance with another study using DNA plasmids harboring Ag85A and Mtb32 in C57/BL6 mice.Citation21 However, another study showed that both CD4+ and CD8+ T cell responses were elicited to Ag85A in C57/BL6 mice by Ad35-TBS which contains Ag85A, Ag85B, and TB10.4.Citation34 Previous studies have reported dominant CD8+ T cell epitopes of Mtb32 in H-2b MHC background.Citation35,36 We speculated that antigenic competition may happen when the dominant CD8+ T cell epitopes of Mtb32 is co-administered with Ag85A in H-2b MHC background,Citation37,38 however, this remains to be elucidated in the future.
The magnitude, quality, and protective efficacy of vaccine-induced CMI responses may be dramatically affected by immunization routes.Citation15-17,39,40 Administering vaccines into subcutaneous tissue may be more immunogenic due to the presence of more professional antigen-presenting cells,Citation41 while immunization through mucosal surfaces was especially important for inducing protective responses against respiratory infectious diseases such as TB.Citation15,16,19,40,42 In the context of rAd5-based vaccines, mucosal immunization may potentially circumvent the dampening effects of pre-existing anti-vector immunity.Citation43,44 Consistent with previous reports, we also found that administering Ad5-gsgAM through SC and mucosal routes efficiently boosted BCG-primed CMI responses in the spleen and lung, respectively (). A significant proportion of dual- and multi-functional CD4+ and CD8+ T cells was increased by SC and IM immunization in the spleen, and by IN and oral administration in the lung, respectively (). Interestingly, comparable pulmonary CMI responses were elicited by a boost immunization via either IN or oral administration (). As the epithelium of the sublingual mucosa is readily accessible to adenovirus infection, Ad5-gsgAM inoculated into oral cavity may efficiently infect the sublingual epithelium cells, so that the antigens can be expressed and presented to T cells by the resident antigen presenting cells.Citation45,46 Considering the convenience of oral administration, this route should be further exploited for the development of Ad5 vector-based TB vaccines, especially as the boost after BCG priming. Future studies will be carried out to evaluate oral, IN and SC routes in in vivo challenge models, and if possible, in non-human primates. Ag85A is one of the 3 mycolyl transferases essential for Mtb survival in macrophages, while Mtb32 is a HtrA-like serine protease whose biological function is still undetermined.Citation47,48 Although they both have shown strong immunogenicity as vaccine components in preclinical or clinical trials, their use in combination has not been reported yet.Citation4,6,21,49 We have demonstrated that robust CMI responses were generated either by IM prime plus IN boost with Ad5-gsgAM or by BCG prime plus Ad5-gsgAM boost in mice. And consistent with previous reports,Citation50,51 a high frequency of Ag85A-specific IFN-γ+TNF-α+ and IFN-γ+TNF-α+IL-2+ CD4+ T cells (), and of Mtb32-specific IFN-γ+TNF-α+ CD8+T cells () was elicited. Whether the enhanced CMI responses induced by Ad5-gsgAM can provide better protection against Mtb infection needs to be determined in in vivo challenge models and ultimately in clinical trials. Earlier studies showed that vaccine induced Mtb specific CD4+ and CD8+ T cells play critical roles in protection against Mtb infection in animals.Citation24,52 However, Mtb specific polyfunctional CD4+ T cells have been shown to correlate with disease progression in studies of individuals with active TB disease and latent TB infection.Citation53,54 Recently, Ag85A specific polyfunctional CD4+ T cells induced by MVA-Ag85A vaccine did not show any evidence of protection in infants in a clinical trial.Citation8 Therefore, the functions of Mtb specific polyfunctional CD4+ and CD8+ T cells in the context of vaccination and Mtb infection remained to be resolved.Citation55 Importantly, the combination of Ag85A and Mtb32 or multiple antigens as potential TB vaccine deserves further evaluation in an in vivo challenge model.
Taken together, we have provided a direct comparison of 4 Ag85A-Mtb32 fusion antigens in the induction of systemic and pulmonary immune responses and reinforced the importance of protein linker in the design of TB vaccines containing multiple antigens. We have also demonstrated that SC and IN immunization with Ad5 vector carrying the Ag85A-Mtb32 fusion antigen generated the strongest systemic and pulmonary immune responses in BCG-primed mice, respectively. Our results provided new insights for the design of genetic vector-based TB vaccines that contain multiple antigens. Future studies will be conducted in an in vivo Mtb challenge model to elucidate the relationship of the enhanced CMI responses and the protection efficacy.
Materials and Methods
Replication-defective recombinant adenoviral vectors
The construction of replication-defective Ad5 vectors was performed according to previously described methods.Citation38 Briefly, the fusion gene encoding Ag85A and Mtb32, named optAM, was optimized according to mammalian codon usage and synthesized with the addition of a Kozak consensus sequence, a tPA signal sequence, and an auto-cleave 2A linker. An HA-tag and a Flag-tag were fused to the Napos; terminus of Ag85A and to the COOH terminus of Mtb32, respectively. This fusion gene was inserted into a shuttle vector, pGA-1. Then, the wild-type sequences of Ag85A and Mtb32 were obtained using PCR amplification from the BCG genome, and the counterparts in pGA1-optAM were substituted to obtain pGA1-wtAM. pGA1-gsgAM and pGA1-ΔsAM were constructed by replacing the 2A-Mtb32-Flag fragment and the tPA-HA-Ag85A fragment in pGA1-optAM with Mtb32-Flag and HA-Ag85A obtained by PCR, respectively. Subsequently, the shuttle vectors were linearized and subjected to homologous recombination with the linearized Ad5 backbone (deletion of the E1 and E3 genes) in the E. coli BJ5183 strain. The pAd5 plasmids were amplified in E. coli strain XLI-Blue and prepared with a Qiagen Plasmid Midi kit (Qiagen, Cat. No. 12145) according to the manufacturer's protocol. pAd5-optAM, pAd5-wtAM, pAd5-gsgAM, and pAd5-ΔsAM were then linearized and transfected into HEK-293 cells to produce the respective recombinant adenovirus. To confirm the expression of Ag85A and Mtb32, Vero cells were infected with purified Ad5-optAM, Ad5-wtAM, Ad5-gsgAM, Ad5-ΔsAM, and Ad5-empty. The cell lysates and culture media were harvested at 48 hours post-infection. The expression levels of Ag85A and Mtb32 were further analyzed by SDS-PAGE and Western blot analyses by using monoclonal anti-HA (Sino Biological Inc.., Cat. No. 50124-RP02) and anti-Flag (Abcam, Cat. No. ab47069) antibodies, respectively.
Fluorescence microscopy
Vero cells were seeded onto glass slides and infected with corresponding recombinant Ad5 vectors. After 48 hours, the cells were washed with PBS and then fixed with 4% paraformaldehyde in PBS for 15 min at 4°C. The cells were permeabilized with 0.05% Triton X-100 in PBS for 5 min and blocked with 5% bovine serum albumin (BSA) in PBS for 1 h. The cells were incubated with a 1:50 dilution of FITC-labeled anti-Flag antibody (Cat. No. bs-0965R-FITC) and a 1:100 dilution of PE-labeled anti-HA antibody (Cat. No. bsm-0966m-PE) in blocking buffer for 1 h at room temperature. The cells were then stained with DAPI. Finally, images of the slides were viewed and photographed using a fluorescence microscope (LEICA, DMI6000B).
Animals and immunization strategies
Six- to 8-week-old female C57BL/6 mice were obtained and housed at the Experimental Animals Center of the Guangzhou Institutes of Biomedicine and Health. All the animal experiments in this study were approved by the Institutional Animal Care and Use Committee of the Guangzhou Institutes of Biomedicine and Health (Permit No. 2012028).
To comparatively evaluate the immunogenicity of 4 Ag85A-Mtb32 fusion antigens, 50 mice were divided randomly into 5 groups and were immunized intramuscularly with 2.5 × 109 viral particles (vp) of recombinant Ad5 vectors. Each mouse received 2 injections of 50ul in each thigh quadriceps muscle of hind legs at week 0. Then, the mice were boosted intranasally with a total dose of 2.5 × 109 vp in 2 aliquots with a fine-tip pipette at week 3. At 2 weeks after prime and boost immunization, 5 mice in each group were sacrificed, and the lymphocytes of the spleen and the lung were isolated and subjected to subsequent immunological assays.
To evaluate the boosting efficiency of Ad5-gsgAM on the BCG-primed CMI responses, 30 mice were divided randomly into 6 groups and were immunized subcutaneously at week 0 with BCG at 1 × 105 colony-forming units (Shanghai Institute of Biological Products Co., Ltd.). At week 6, the mice were boosted with 2.5 × 109 vp Ad5-gsgAM through 4 routes: IM, SC, oral, and IN. At 2 weeks after boost immunization, the animals were sacrificed, and the lymphocytes of the spleen and the lung were isolated and subjected to subsequent immunological assays.
Lymphocyte preparation
Splenocytes were obtained as described previously.Citation38 Lung lymphocytes were prepared as follows: first, the lungs were exhaustively perfused by PBS through the right ventricle of the heart and cut into pieces. Then, the homogenates were subjected to digestion with collagenase Type 1 (Catalog No. 2195109.1) for 1 h at 37°C, and were crushed through a 75-µm filter. The lung cells were washed with RPMI 1640 medium and then suspended in R10 medium supplemented with 10% fetal bovine serum and 100 µg/ml penicillin and streptomycin.
IFN-γ enzyme-linked immunospot (ELISPOT) assay
To test the CMI responses induced by rAd5 vectors carrying different Ag85A-Mtb32 fusions, an IFN-γ ELISPOT assay was performed as previously described. Briefly, 96-well plates (Millipore, Immobilon-P membrane) were activated with 75% alcohol, washed with PBS, and coated with a mouse IFN-γ capture antibody (BD PharMingen) overnight at 4°C. Then, the plates were blocked with R10 medium for 2 hours at 37°C. Splenocytes and lung cells were seeded at 5 × 105 cells per well and incubated for 24 hours at 37°C with or without stimulation with the synthesized peptide pools for Ag85A and Mtb32 (Hangzhou Chinese Peptide Co., Ltd.). Subsequently, the plates were developed with the biotinylated polyclonal anti-mouse IFN-γ (BD PharMingen) and NBT/BCIP reagent (Pierce). Finally, the number of IFN-γ-releasing cells was counted by an ELISPOT reader (Bioreader 4000, BIOSYS, Germany).
Intracellular cytokine staining (ICS) and multicolor flowcytometry
To evaluate the quantity and quality of immunization-induced cytokine-producing T cells, ICS and multicolor flow cytometry were performed as previously described. Briefly, freshly isolated splenocytes and lung cells were seeded into 96-well plates at 2 × 106 cells per well and stimulated with either DMSO (Sigma) or peptide pools for Ag85A or Mtb32 (2 µg/ml per peptide). After 1 hour incubation at 37°C, the cells were mixed with brefeldin A (BD PharMingen) to inhibit cytokine secretion and were incubated for 16 hours at 37°C. The cells were stained with surface antibodies (CD3-PerCP, CD4-FITC, CD8-APC; BD Biosciences) for 1 hour, and then were washed and permeabilized in FACS Perm buffer (BD PharMingen) for 20 minutes. Subsequently, the cells were stained with intracellular antibodies (IFN-γ-PE, TNF-α-PE-cy7, IL-2-APC-cy7; BD Pharmingen). Finally, the stained cells were analyzed with a BD Accuri™ C6 (BD Biosciences).
Data collection and statistical analysis
The flow cytometry data were analyzed with FlowJo software (version 7.6.2; Tree Star, Inc.., Ashland, OR). Statistical analysis and graphical presentations were generated with GraphPad Prism software (version 5.01; GraphPad Software Inc.., La Jolla, CA). Mean and standard deviations were used to describe the immune response in each group. A comparison between the various groups was performed using a 2-tailed Student's t-test (p < 0.05 was considered statistically significant).
Disclosure of Potential Conflicts of Interest
No potential conflicts of interest were disclosed.
Acknowledgments
We thank Dr. Tianyu ZHANG for helpful suggestions and the review of the manuscript draft. We thank Yi ZHENG, Zheyu CAI, Xiangjie FENG, Weimin ZHANG and Jianfu TAO and the staff at the Animal Center of GIBH for their excellent technical assistance.
Funding
This study was supported by the National Natural Science Foundation of China (31200693, 31470892, 31370923), and the grant of CAS Youth Innovation Promotion Association to Dr. Liqiang Feng. This study was partly supported by a collaborative grant from the municipal government of Guangzhou. The funders had no role in study design, data collection and analysis, decision to publish, or preparation of the manuscript.
References
- Organization WH. Global Tuberculosis Report 2013. http://www.who.int/tb/publications/global_report/en/2013.
- Montagnani C, Chiappini E, Galli L, de Martino M. Vaccine against tuberculosis: what's new? BMC Infect Dis 2014; 14 Suppl 1:S2; PMID:24564340; http://dx.doi.org/10.1186/1471-2334-14-S1-S2
- Mittrucker HW, Steinhoff U, Kohler A, Krause M, Lazar D, Mex P, Miekley D, Kaufmann SH. Poor correlation between BCG vaccination-induced T cell responses and protection against tuberculosis. P Natl Acad Sci USA 2007; 104:12434-9; http://dx.doi.org/10.1073/pnas.0703510104
- Smaill F, Jeyanathan M, Smieja M, Medina MF, Thanthrige-Don N, Zganiacz A, Yin C, Heriazon A, Damjanovic D, Puri L, et al. A Human Type 5 Adenovirus-Based Tuberculosis Vaccine Induces Robust T Cell Responses in Humans Despite Preexisting Anti-Adenovirus Immunity. Sci Transl Med 2013; 5:205ra134
- Abel B, Tameris M, Mansoor N, Gelderbloem S, Hughes J, Abrahams D, Makhethe L, Erasmus M, de Kock M, van der Merwe L, et al. The novel tuberculosis vaccine, AERAS-402, induces robust and polyfunctional CD4+ and CD8+ T cells in adults. Am J Respir Crit Care Med 2010; 181:1407-17; PMID:20167847; http://dx.doi.org/10.1164/rccm.200910-1484OC
- Scriba TJ, Tameris M, Mansoor N, Smit E, van der Merwe L, Isaacs F, Keyser A, Moyo S, Brittain N, Lawrie A, et al. Modified vaccinia Ankara-expressing Ag85A, a novel tuberculosis vaccine, is safe in adolescents and children, and induces polyfunctional CD4+ T cells. Eur J Immunol 2010; 40:279-90; PMID:20017188; http://dx.doi.org/10.1002/eji.200939754
- Mu J, Jeyanathan M, Small CL, Zhang X, Roediger E, Feng X, Chong D, Gauldie J, Xing Z. Immunization with a bivalent adenovirus-vectored tuberculosis vaccine provides markedly improved protection over its monovalent counterpart against pulmonary tuberculosis. Mol Ther 2009; 17:1093-100; PMID:19319120; http://dx.doi.org/10.1038/mt.2009.60
- Tameris MD, Hatherill M, Landry BS, Scriba TJ, Snowden MA, Lockhart S, Shea JE, McClain JB, Hussey GD, Hanekom WA, et al. Safety and efficacy of MVA85A, a new tuberculosis vaccine, in infants previously vaccinated with BCG: a randomised, placebo-controlled phase 2b trial. Lancet 2013; 381:1021-8; http://dx.doi.org/10.1016/S0140-6736(13)60177-4
- Havenga M, Vogels R, Zuijdgeest D, Radosevic K, Mueller S, Sieuwerts M, Weichold F, Damen I, Kaspers J, Lemckert A, et al. Novel replication-incompetent adenoviral B-group vectors: high vector stability and yield in PER.C6 cells. J Gen Virol 2006; 87:2135-43; PMID:16847108; http://dx.doi.org/10.1099/vir.0.81956-0
- Mir FA, Kaufmann SH, Eddine AN. A multicistronic DNA vaccine induces significant protection against tuberculosis in mice and offers flexibility in the expressed antigen repertoire. Clin Vaccine Immunol 2009; 16:1467-75; PMID:19656992; http://dx.doi.org/10.1128/CVI.00237-09
- Yuan W, Dong N, Zhang L, Liu J, Lin S, Xiang Z, Qiao H, Tong W, Qin C. Immunogenicity and protective efficacy of a tuberculosis DNA vaccine expressing a fusion protein of Ag85B-Esat6-HspX in mice. Vaccine 2012; 30:2490-7; PMID:21704108; http://dx.doi.org/10.1016/j.vaccine.2011.06.029
- Perez de Val B, Vidal E, Villarreal-Ramos B, Gilbert SC, Andaluz A, Moll X, Martín M, Nofrarías M, McShane H, Vordermeier HM, et al. A multi-antigenic adenoviral-vectored vaccine improves BCG-induced protection of goats against pulmonary tuberculosis infection and prevents disease progression. PloS one 2013; 8:e81317; PMID:24278420; http://dx.doi.org/10.1371/journal.pone.0081317
- Whelan A, Court P, Xing Z, Clifford D, Hogarth PJ, Vordermeier M, Villarreal-Ramos B. Immunogenicity comparison of the intradermal or endobronchial boosting of BCG vaccinates with Ad5-85A. Vaccine 2012; 30:6294-300; PMID:22885013; http://dx.doi.org/10.1016/j.vaccine.2012.07.086
- McCluskie MJ, Brazolot Millan CL, Gramzinski RA, Robinson HL, Santoro JC, Fuller JT, Widera G, Haynes JR, Purcell RH, Davis HL. Route and method of delivery of DNA vaccine influence immune responses in mice and non-human primates. Mol Med 1999; 5:287-300; PMID:10390545; http://dx.doi.org/10.1007/s0089490050287
- Wang J, Thorson L, Stokes RW, Santosuosso M, Huygen K, Zganiacz A, Hitt M, Xing Z. Single mucosal, but not parenteral, immunization with recombinant adenoviral-based vaccine provides potent protection from pulmonary tuberculosis. J Immunol 2004; 173:6357-65; http://dx.doi.org/10.4049/jimmunol.173.10.6357
- Xing Z, McFarland CT, Sallenave JM, Izzo A, Wang J, McMurray DN. Intranasal mucosal boosting with an adenovirus-vectored vaccine markedly enhances the protection of BCG-primed guinea pigs against pulmonary tuberculosis. PloS one 2009; 4:e5856; PMID:19516906; http://dx.doi.org/10.1371/journal.pone.0005856
- Forbes EK, Sander C, Ronan EO, McShane H, Hill AV, Beverley PC, Tchilian EZ. Multifunctional, high-level cytokine-producing Th1 cells in the lung, but not spleen, correlate with protection against Mycobacterium tuberculosis aerosol challenge in mice. J Immunol 2008; 181:4955-64; http://dx.doi.org/10.4049/jimmunol.181.7.4955
- Santosuosso M, Zhang X, McCormick S, Wang J, Hitt M, Xing Z. Mechanisms of mucosal and parenteral tuberculosis vaccinations: adenoviral-based mucosal immunization preferentially elicits sustained accumulation of immune protective CD4 and CD8 T cells within the airway lumen. J Immunol 2005; 174:7986-94; http://dx.doi.org/10.4049/jimmunol.174.12.7986
- Santosuosso M, McCormick S, Roediger E, Zhang X, Zganiacz A, Lichty BD, Xing Z. Mucosal luminal manipulation of T cell geography switches on protective efficacy by otherwise ineffective parenteral genetic immunization. J Immunol 2007; 178:2387-95; http://dx.doi.org/10.4049/jimmunol.178.4.2387
- Vordermeier HM, Villarreal-Ramos B, Cockle PJ, McAulay M, Rhodes SG, Thacker T, Gilbert SC, McShane H, Hill AV, Xing Z, et al. Viral booster vaccines improve Mycobacterium bovis BCG-induced protection against bovine tuberculosis. Infect Immun 2009; 77:3364-73; PMID:19487476; http://dx.doi.org/10.1128/IAI.00287-09
- Ahn SS, Jeon BY, Kim KS, Kwack JY, Lee EG, Park KS, Sung YC, Cho SN. Mtb32 is a promising tuberculosis antigen for DNA vaccination in pre- and post-exposure mouse models. Gene Ther 2012; 19:570-5; PMID:21956689; http://dx.doi.org/10.1038/gt.2011.140
- Cooper AM, Mayer-Barber KD, Sher A. Role of innate cytokines in mycobacterial infection. Mucosal Immunol 2011; 4:252-60; PMID:21430655; http://dx.doi.org/10.1038/mi.2011.13
- Redford PS, Boonstra A, Read S, Pitt J, Graham C, Stavropoulos E, Bancroft GJ, O'Garra A. Enhanced protection to Mycobacterium tuberculosis infection in IL-10-deficient mice is accompanied by early and enhanced Th1 responses in the lung. Eur J Immunol 2010; 40:2200-10; PMID:20518032; http://dx.doi.org/10.1002/eji.201040433
- Derrick SC, Yabe IM, Yang A, Morris SL. Vaccine-induced anti-tuberculosis protective immunity in mice correlates with the magnitude and quality of multifunctional CD4 T cells. Vaccine 2011; 29:2902-9; PMID:21338678; http://dx.doi.org/10.1016/j.vaccine.2011.02.010
- Caccamo N, Meraviglia S, La Mendola C, Guggino G, Dieli F, Salerno A. Phenotypical and functional analysis of memory and effector human CD8 T cells specific for mycobacterial antigens. J Immunol 2006; 177:1780-5; http://dx.doi.org/10.4049/jimmunol.177.3.1780
- Caccamo N, Guggino G, Meraviglia S, Gelsomino G, Di Carlo P, Titone L, Bocchino M, Galati D, Matarese A, Nouta J, et al. Analysis of Mycobacterium tuberculosis-specific CD8 T-cells in patients with active tuberculosis and in individuals with latent infection. PloS one 2009; 4:e5528; PMID:19436760; http://dx.doi.org/10.1371/journal.pone.0005528
- Cid-Arregui A, Juarez V, zur Hausen H. A synthetic E7 gene of human papillomavirus type 16 that yields enhanced expression of the protein in mammalian cells and is useful for DNA immunization studies. J Virol 2003; 77:4928-37; PMID:12663798; http://dx.doi.org/10.1128/JVI.77.8.4928-4937.2003
- Ramakrishna L, Anand KK, Mohankumar KM, Ranga U. Codon optimization of the tat antigen of human immunodeficiency virus type 1 generates strong immune responses in mice following genetic immunization. J Virol 2004; 78:9174-89; PMID:15308713; http://dx.doi.org/10.1128/JVI.78.17.9174-9189.2004
- Ko HJ, Ko SY, Kim YJ, Lee EG, Cho SN, Kang CY. Optimization of codon usage enhances the immunogenicity of a DNA vaccine encoding mycobacterial antigen Ag85B. Infect Immun 2005; 73:5666-74; PMID:16113284; http://dx.doi.org/10.1128/IAI.73.9.5666-5674.2005
- Wen B, Deng Y, Guan J, Yan W, Wang Y, Tan W, Gao J. Signal peptide replacements enhance expression and secretion of hepatitis C virus envelope glycoproteins. Acta biochimica et biophysica Sinica 2011; 43:96-102; PMID:21196448; http://dx.doi.org/10.1093/abbs/gmq117
- de Felipe P, Luke GA, Brown JD, Ryan MD. Inhibition of 2A-mediated ‘cleavage’ of certain artificial polyproteins bearing N-terminal signal sequences. Biotechnol J 2010; 5:213-23; PMID:19946875; http://dx.doi.org/10.1002/biot.200900134
- Chia MY, Hsiao SH, Chan HT, Do YY, Huang PL, Chang HW, Tsai YC, Lin CM, Pang VF, Jeng CR. The immunogenicity of DNA constructs co-expressing GP5 and M proteins of porcine reproductive and respiratory syndrome virus conjugated by GPGP linker in pigs. Veterinary Microbiol 2010; 146:189-99; PMID:20570063; http://dx.doi.org/10.1016/j.vetmic.2010.05.007
- Chen X, Zaro JL, Shen WC. Fusion protein linkers: property, design and functionality. Adv Drug Deliv Rev 2013; 65:1357-69; PMID:23026637; http://dx.doi.org/10.1016/j.addr.2012.09.039
- Radosevic K, Wieland CW, Rodriguez A, Weverling GJ, Mintardjo R, Gillissen G, Vogels R, Skeiky YA, Hone DM, Sadoff JC, et al. Protective immune responses to a recombinant adenovirus type 35 tuberculosis vaccine in two mouse strains: CD4 and CD8 T-cell epitope mapping and role of gamma interferon. Infection and immunity 2007; 75:4105-15; PMID:17526747; http://dx.doi.org/10.1128/IAI.00004-07
- Skeiky YA, Alderson MR, Ovendale PJ, Guderian JA, Brandt L, Dillon DC, Campos-Neto A, Lobet Y, Dalemans W, Orme IM, et al. Differential immune responses and protective efficacy induced by components of a tuberculosis polyprotein vaccine, Mtb72F, delivered as naked DNA or recombinant protein. J Immunol 2004; 172:7618-28; http://dx.doi.org/10.4049/jimmunol.172.12.7618
- Irwin SM, Izzo AA, Dow SW, Skeiky YA, Reed SG, Alderson MR, Orme IM. Tracking antigen-specific CD8 T lymphocytes in the lungs of mice vaccinated with the Mtb72F polyprotein. Infect Immun 2005; 73:5809-16; PMID:16113299; http://dx.doi.org/10.1128/IAI.73.9.5809-5816.2005
- Hel Z, Tsai WP, Tryniszewska E, Nacsa J, Markham PD, Lewis MG, Pavlakis GN, Felber BK, Tartaglia J, Franchini G. Improved vaccine protection from simian AIDS by the addition of nonstructural simian immunodeficiency virus genes. J Immunol 2006; 176:85-96; http://dx.doi.org/10.4049/jimmunol.176.1.85
- Zhang Y, Sun C, Feng L, Xiao L, Chen L. Enhancement of Gag-specific but reduction of Env- and Pol-specific CD8+ T cell responses by simian immunodeficiency virus nonstructural proteins in mice. AIDS Res hum Retroviruses 2012; 28:374-83; PMID:21736424; http://dx.doi.org/10.1089/aid.2011.0061
- Beverley PC, Sridhar S, Lalvani A, Tchilian EZ. Harnessing local and systemic immunity for vaccines against tuberculosis. Mucosal Immunol 2014; 7:20-6; PMID:24253104; http://dx.doi.org/10.1038/mi.2013.99
- Santosuosso M, McCormick S, Zhang X, Zganiacz A, Xing Z. Intranasal boosting with an adenovirus-vectored vaccine markedly enhances protection by parenteral Mycobacterium bovis BCG immunization against pulmonary tuberculosis. Infect Immun 2006; 74:4634-43; PMID:16861651; http://dx.doi.org/10.1128/IAI.00517-06
- Koblin BA, Casapia M, Morgan C, Qin L, Wang ZM, Defawe OD, Baden L, Goepfert P, Tomaras GD, Montefiori DC, et al. Safety and immunogenicity of an HIV adenoviral vector boost after DNA plasmid vaccine prime by route of administration: a randomized clinical trial. PloS one 2011; 6:e24517; PMID:21931737; http://dx.doi.org/10.1371/journal.pone.0024517
- Ma C, Li Y, Wang L, Zhao G, Tao X, Tseng CT, Zhou Y, Du L, Jiang S. Intranasal vaccination with recombinant receptor-binding domain of MERS-CoV spike protein induces much stronger local mucosal immune responses than subcutaneous immunization: Implication for designing novel mucosal MERS vaccines. Vaccine 2014; 32:2100-8; PMID:24560617; http://dx.doi.org/10.1016/j.vaccine.2014.02.004
- Richardson JS, Pillet S, Bello AJ, Kobinger GP. Airway delivery of an adenovirus-based Ebola virus vaccine bypasses existing immunity to homologous adenovirus in nonhuman primates. JVirol 2013; 87:3668-77; PMID:23302894; http://dx.doi.org/10.1128/JVI.02864-12
- Xiang ZQ, Gao GP, Reyes-Sandoval A, Li Y, Wilson JM, Ertl HC. Oral vaccination of mice with adenoviral vectors is not impaired by preexisting immunity to the vaccine carrier. J Virol 2003; 77:10780-9; PMID:14512528; http://dx.doi.org/10.1128/JVI.77.20.10780-10789.2003
- Appledorn DM, Aldhamen YA, Godbehere S, Seregin SS, Amalfitano A. Sublingual administration of an adenovirus serotype 5 (Ad5)-based vaccine confirms Toll-like receptor agonist activity in the oral cavity and elicits improved mucosal and systemic cell-mediated responses against HIV antigens despite preexisting Ad5 immunity. Clin Vaccine Immunol 2011; 18:150-60; PMID:21084461; http://dx.doi.org/10.1128/CVI.00341-10
- Hovav AH. Dendritic cells of the oral mucosa. Mucosal Immunol 2014; 7:27-37; PMID:23757304; http://dx.doi.org/10.1038/mi.2013.42
- Skeiky YA, Lodes MJ, Guderian JA, Mohamath R, Bement T, Alderson MR, Reed SG. Cloning, expression, and immunological evaluation of two putative secreted serine protease antigens of Mycobacterium tuberculosis. Infect Immun 1999; 67:3998-4007.; PMID:10417166
- Armitige LY, Jagannath C, Wanger AR, Norris SJ. Disruption of the genes encoding antigen 85A and antigen 85B of Mycobacterium tuberculosis H37Rv: effect on growth in culture and in macrophages. Infect Immun 2000; 68:767-78; PMID:10639445; http://dx.doi.org/10.1128/IAI.68.2.767-778.2000
- Ahn SS, Jeon BY, Park SJ, Choi DH, Ku SH, Cho SN, Sung YC. Nonlytic Fc-fused IL-7 synergizes with Mtb32 DNA vaccine to enhance antigen-specific T cell responses in a therapeutic model of tuberculosis. Vaccine 2013; 31:2884-90; PMID:23624092; http://dx.doi.org/10.1016/j.vaccine.2013.04.029
- Romano M, Roupie V, Wang XM, Denis O, Jurion F, Adnet PY, Laali R, Huygen K. Immunogenicity and protective efficacy of tuberculosis DNA vaccines combining mycolyl-transferase Ag85A and phosphate transport receptor PstS-3. Immunology 2006; 118:321-32; PMID:16827893; http://dx.doi.org/10.1111/j.1365-2567.2006.02373.x
- Tzelepis F, Verway M, Daoud J, Gillard J, Hassani-Ardakani K, Dunn J, Downey J, Gentile ME, Jaworska J, Sanchez AM, et al. Annexin1 regulates DC efferocytosis and cross-presentation during Mycobacterium tuberculosis infection. J Clin Investig 2015; 125:752-68; PMID:25562320; http://dx.doi.org/10.1172/JCI77014
- Chen CY, Huang D, Wang RC, Shen L, Zeng G, Yao S, Shen Y, Halliday L, Fortman J, McAllister M, et al. A critical role for CD8 T cells in a nonhuman primate model of tuberculosis. PLoS Pathogens 2009; 5:e1000392; PMID:19381260; http://dx.doi.org/10.1371/journal.ppat.1000392
- Qiu Z, Zhang M, Zhu Y, Zheng F, Lu P, Liu H, Graner MW, Zhou B, Chen X. Multifunctional CD4 T cell responses in patients with active tuberculosis. Sci Rep 2012; 2:216.; PMID:22355730
- Caccamo N, Guggino G, Joosten SA, Gelsomino G, Di Carlo P, Titone L, Galati D, Bocchino M, Matarese A, Salerno A, et al. Multifunctional CD4(+) T cells correlate with active Mycobacterium tuberculosis infection. Eur J Immunol 2010; 40:2211-20; PMID:20540114; http://dx.doi.org/10.1002/eji.201040455
- Prezzemolo T, Guggino G, La Manna MP, Di Liberto D, Dieli F, Caccamo N. Functional Signatures of Human CD4 and CD8 T Cell Responses to Mycobacterium tuberculosis. Front Immunol 2014; 5:180; PMID:24795723; http://dx.doi.org/10.3389/fimmu.2014.00180