Abstract
Metabolic analysis for medium optimization represents a very useful strategy in the process development of production of vaccines in cells. During influenza vaccine production, viruses hijack host cells and take advantage of host's metabolism. As a consequence, the nutritional demand of host cells should undergo a profound change, and usually more nutrients such as glucose and amino acids should be consumed. As such, the maintaining media used in virus production processes often cannot provide sufficient nutrients, and novel methods are urged to be established to address this severe issue of nutritional limitation. A detailed study on impacts of influenza virus on cell death and metabolism, with a profound analysis of nutritional requirements during virus production process, followed by a rational medium optimization is expected to be the most straightfoward and effective strategy. This would ensure a balanced and adequate nutritional supply, which should minimize cell death and improve both cell-specific virus yield and total influenza virus production. Such a metabolic analysis-based medium optimization would lay a solid foundation for the development of cell culture technology in influenza vaccine production.
Introduction
In recent years, the global demand of influenza vaccine is increasing rapidly. However, current production capacity by using cell culture technology is seriously insufficient, which requests a further improvement in virus productivity by host cells. Among various approaches exploited, the metabolic analysis represents a very promising one.Citation1-3
For influenza vaccine production, viruses utilize a variety of strategies to penetrate cells and take over the synthesis machinery to build up their own components and produce progeny. Hence, the nutritional demand of host cells should undergo a profound and complex shift. For instance, the glucose demand can be affected by virus infection and replication,Citation4-6 various proteins need to be synthesized for virus replication, which apparently exert significant influences on the demand for amino acids,Citation7,8 and lipid rafts are also badly needed during the infection and replication process.Citation9-11 Not surprisingly, the common maintaining media used in virus production processes cannot provide sufficient nutrients in general and additional supplementation of nutrients must be performed. Currently, several strategies have been exploited to address this issue of nutritional limitation, including fresh medium replenishment, medium-exchanging, fed-batch, perfusion, etc..Citation12-18 However, a rational design of medium supplementation based on comprehensive nutritional analyses for cells during virus production phase is still missing. Only through a detailed analysis on the differences in the cellular metabolism before and after viral infection, an insight understanding of real nutritional requirements during virus production phase can be achieved, and a rational design of medium supplementation can be solid.
Effects of influenza virus infection on host cell growth and metabolism
Viruses must hijack and exploit their host cells for survival. In order to take full advantage of resources in their hosts for effective propagation, they have evolved various sophisticated strategies that would profoundly affect the hosts. They enslave cellular signaling pathways and transcription factors, and control them to their own advantage. In particular, influenza virus can alter host cell growth and metabolism characteristic via viral proteins significantly ().Citation19
Figure 1. Release and uptake of metabolites: (A) (▪) glucose, (▴) lactate; (B) (○) total glutamine, (•) glutamine without chemical decomposition and (▪) ammonium during growth of MDCK cells on microcarriers in a 5 L stirred bioreactor (0–92 h) and during influenza virus production after medium exchange (96–140 h). Adapted from Genzel et al.Citation19
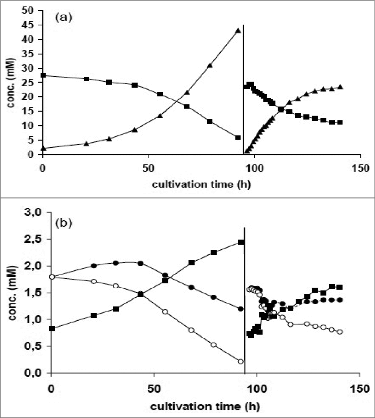
Viruses have been known for a long time to utilize a variety of strategies to take over host cells' nucleic acid and protein synthesis machineries to build up their own components and produce viral progenyies. Moreover, influenza virus replication and viral proteins can potentially trigger the apoptosis pathway and anti- and pro-viral molecular mechanisms in host cells,Citation20-24 which must also have exerted a great influence on cell growth and death. Therefore, it is highly possible that the anti- and pro-viral molecular mechanisms and apoptosis, together with the replication of influenza virus, take over energy and biomass for the synthesis of macromolecules like proteins, RNA and lipids, and thus exert profound influences on host cell metabolism. Besides, cell apoptosis leads to breakdown of mitochondrial membrane and then cellular respiration is terminated, resulting in a drastic shortage of ATP. Accordingly, relevant metabolism pathways must be adjusted to compensate for such energetic deficiencies.Citation25
Given all these, the nutritional requirements of cells post influenza virus infection can be drastically different from those of non-infected cells. With the objective to obtain a great influenza vaccine productivity, an analysis of nutritional requirements for cells post infection becomes necessary to develop an appropriate nutritional replenishment strategy for cell culture.
Current strategies to overcome nutritional limitation
As stated, the intracellular free nutrients like glucose and amino acids can be the bottleneck for virus production, as a higher virus yield requires the uptake and synthesis of more resources during the infection process. To address this issue, , current strategies include both simple and straightforward processes of fresh medium additionCitation12 and medium exchanging, and the relatively complex processes like fed-batch and perfusion.Citation13-19 In particular, one reported approach utilizes an alternating tangential flow (ATF) system.Citation18 With eight successful high cell-density ATF perfusion runs for the production of H1N1 virus, it was demonstrated that cell-specific virus yields could be kept constant and cell-specific infectious virus titers were even higher than those of batch cultures ().Citation18
Figure 2. Virus production in a 1 L batch (A: HA titer and B: TCID50) and perfusion (C: HA titer and D: TCID50) stirred tank bioreactor (ATF). Adapted from Genzel et al.Citation18
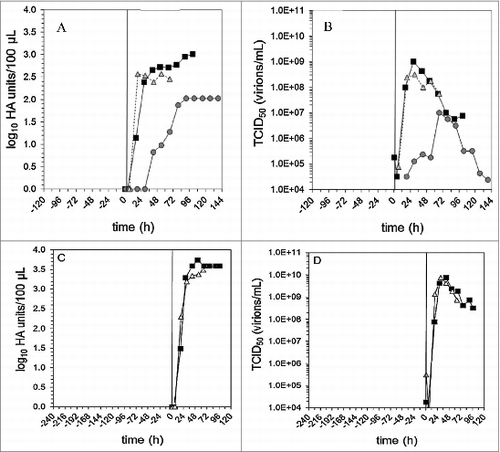
However, whether the nutritional supplementation is entirely dedicated to influenza virus production in these studies remains unclear. It is highly possible that not all nutrients consumed by cells enter the pro-viral pathway. Instead, some nutrients may even contribute to the anti-viral responses in host cells. Consequently, and such empirical supplementation of nutrients might even be counter-productive for influenza vaccines. In addition, these nutritional supplementation modes usually make the culture processes very complicated.
Metabolic analysis-based medium optimization for influenza virus production
We had performed a metabolic analysis for cells during influenza virus production. The essentiality and necessity of Glucose, Glutamine and other amino acids including Serine, Glycine, Histidine, Threonine, Arginine, Tyrosine, Cystine, Valine, Methionine, Ileucine, Leucine, Lysine and Phenylalanine in cells were demonstrated ().Citation26 Within a certain concentration range, Glucose delayed cell death, Glutamine could not only slow down cell death, but also contribute to a high yield of virus, and addition of a mixture of amino acids including Serine, Glycine, Histidine, Threonine, Arginine, Tyrosine, Cystine, Valine, Methionine, Ileucine, Leucine, Lysine and Phenylalanine only enhanced the virus yield. This demonstrates that a detailed metabolic analysis makes nutrient supplementation more quick and accurate. As reported, by studying the impacts of influenza virus on host cell death and metabolism, dissecting nutritional and metabolic demands of host cells during virus production phase were analyzed in detail, a rational nutritional replenishment strategy could be designed whereupon.Citation23 With such a rational nutritional replenishment strategy, cell death post infection could be slowed down, and both total and cell specific influenza virus yield had been enhanced.
Figure 3. Specific metabolism rates (Q) of glutamine, glucose, lactate acid and ammonia and Qi/Qm of amino acids except glutamine. (□) mock; (▪) infected; (

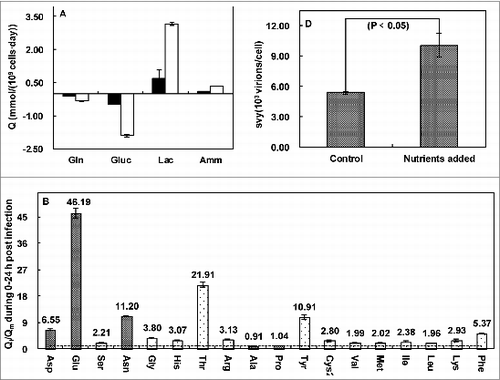
It was demonstrated virus production can be efficiently improved through a rational optimization of nutritional components in culture medium on the basis of metabolism analysis and nutritional demand investigation during production phase.
Conclusions and perspectives
The remarkable potential of metabolic analysis and nutritional optimization as a useful strategy to improve productivity of influenza vaccines in cell technology is highlighted.
An important issue is whether the antigenicity of progeny influenza virus produced is same to that of parent virus. Recently, the quality of influenza vaccine has recently been a great concern, especially including glycosylation and antigenicity. It has been pointed out that the antigenicity of progeny influenza virus is largely determined by the glycosylation of HA, and culture conditions including nutritional components in the medium have no influence on the glycosylation.Citation27 In contrast, some reported that metabolic by-product accumulation of lactate and ammonia derived from glucose and amino acids could impact the glycosylation,Citation28–30 implicating the influence of nutrients on the antigenicity of progeny influenza virus.
Taken together, an insight understanding of the interaction between influenza virus and its host cell should be gained, wherein nutrients for the pro-viral process should be differentiated from those for the anti-viral responses. As such, the production processes could become more effective based on an optimized medium supplementation. Future research on effects of culture environment (e.g. host cells, nutrients, culture conditions, and etc.) on viruses, especially on their surface protein antigenicity, should be emphasized, the outcome of which would make cell-based influenza vaccine production more efficient and saft.
Disclosure of Potential Conflicts of Interest
No potential conflicts of interest were disclosed.
Funding
This work was supported by the National Natural Science Foundation of China (Nos. 21206040, 21406066), the National High Technology Research and Development Program of China (863 Program) (No. 2012AA02A303), the National Science and Technology Major Project (No.2013ZX10004003-003-003), and the Fundamental Research Funds for the Central Universities (WF1214035).
References
- Niklas J, Heinzle E. Metabolic flux analysis in systems biology of mammalian cells. Springer: Genomics and Systems Biology of Mammalian Cell Culture; 2012:109-32.
- Quek L-E, Dietmair S, Krömer JO, Nielsen LK. Metabolic flux analysis in mammalian cell culture. Metab Eng 2010; 12:161-71; PMID:19833223; http://dx.doi.org/10.1016/j.ymben.2009.09.002
- Çelik E, Çalık P, Oliver SG. Metabolic flux analysis for recombinant protein production by Pichia pastoris using dual carbon sources: Effects of methanol feeding rate. Biotechnol Bioeng 2010; 105:317-29; PMID:19777584; http://dx.doi.org/10.1002/bit.22543
- Fisher TN, Ginsberg HS. The reaction of influenza viruses with guinea pig polymorphonuclear leucocytes. II. The reduction of white blood cell glycolysis by influenza viruses and receptor-destroying enzyme (RDE). Virology 1956; 2:637-55; PMID:13371724; http://dx.doi.org/10.1016/0042-6822(56)90044-7
- Genzel Y, Olmer R, Schäfer B, Reichl U. Wave microcarrier cultivation of MDCK cells for influenza virus production in serum containing and serum-free media. Vaccine 2006; 24:6074-87; PMID:16781022; http://dx.doi.org/10.1016/j.vaccine.2006.05.023
- Klemperer H. Glucose breakdown in chick embryo cells infected with influenza virus. Virology 1961; 13:68-77; PMID:13756751; http://dx.doi.org/10.1016/0042-6822(61)90033-2
- Genzel Y, Behrendt I, König S, Sann H, Reichl U. Metabolism of MDCK cells during cell growth and influenza virus production in large-scale microcarrier culture. Vaccine 2004; 22:2202-8; PMID:15149778; http://dx.doi.org/10.1016/j.vaccine.2003.11.041
- Genzel Y, Fischer M, Reichl U. Serum-free influenza virus production avoiding washing steps and medium exchange in large-scale microcarrier culture. Vaccine 2006; 24:3261-72; PMID:16472544; http://dx.doi.org/10.1016/j.vaccine.2006.01.019
- Billharz R, Zeng H, Proll SC, Korth MJ, Lederer S, Albrecht R, Goodman AG, Rosenzweig E, Tumpey TM, García-Sastre A, et al. The NS1 protein of the 1918 pandemic influenza virus blocks host interferon and lipid metabolism pathways. J Virol 2009; 83:10557-70; PMID:19706713; http://dx.doi.org/10.1128/JVI.00330-09
- Munger J, Bennett BD, Parikh A, Feng X-J, McArdle J, Rabitz HA, Shenk T, Rabinowitz JD. Systems-level metabolic flux profiling identifies fatty acid synthesis as a target for antiviral therapy. Nat Biotechnol 2008; 26:1179-86; PMID:18820684; http://dx.doi.org/10.1038/nbt.1500
- Lin S, Liu N, Yang Z, Song W, Wang P, Chen H, Lucio M, Schmitt-Kopplin P, Chen G, Cai Z. GC/MS-based metabolomics reveals fatty acid biosynthesis and cholesterol metabolism in cell lines infected with influenza A virus. Talanta 2010; 83:262-8; PMID:21035673; http://dx.doi.org/10.1016/j.talanta.2010.09.019
- Peschel B, Frentzel S, Laske T, Genzel Y, Reichl U. Comparison of influenza virus yields and apoptosis-induction in an adherent and a suspension MDCK cell line. Vaccine 2013; 31:5693-9; PMID:24113260; http://dx.doi.org/10.1016/j.vaccine.2013.09.051
- Bock A, Schulze Horsel J, Schwarzer J, Rapp E, Genzel Y, Reichl U. High density microcarrier cell cultures for influenza virus production. Biotechnol Prog 2011; 27:241-50; PMID:21312371; http://dx.doi.org/10.1002/btpr.539
- Maranga L, Goochee CF. Metabolism of PER. C6TM cells cultivated under fed batch conditions at low glucose and glutamine levels. Biotechnol Bioeng 2006; 94:139-50; PMID:16523524; http://dx.doi.org/10.1002/bit.20890
- Pohlscheidt M, Langer U, Minuth T, Bödeker B, Apeler H, Hörlein H-D, Paulsen D, Rübsamen-Waigmann H, Henzler HJ, Reichl U. Development and optimisation of a procedure for the production of Parapoxvirus ovis by large-scale microcarrier cell culture in a non-animal, non-human and non-plant-derived medium. Vaccine 2008; 26:1552-65; PMID:18295380; http://dx.doi.org/10.1016/j.vaccine.2008.01.032
- Pohlscheidt M, Bodeker B, Langer U, Apeler H, Horlein HD, Rubsamen-Waigmann H, Reichl U, Henzler HJ. Interpretation data for bioreactors for the increase of scale of a microcarrier-based virus production process. Chemie Ingenieur Technik 2008; 80:821-30; http://dx.doi.org/10.1002/cite.200700125
- Henry O, Dormond E, Perrier M, Kamen A. Insights into adenoviral vector production kinetics in acoustic filter based perfusion cultures. Biotechnol Bioeng 2004; 86:765-74; PMID:15162452; http://dx.doi.org/10.1002/bit.20074
- Genzel Y, Vogel T, Buck J, Behrendta I, Ramireza DV, Schiednerc G, Jordand I, Reichla U. High cell density cultivations by alternating tangential flow (ATF) perfusion for influenza A virus production using suspension cells. Vaccine 2014; 32:2770-2781; PMID:24583003; http://dx.doi.org/10.1016/j.vaccine.2014.02.016
- Genzel Y, Behrendt I, König S, Sann H, Reichl U. Metabolism of MDCK cells during cell growth and influenza virus production in large-scale microcarrier culture. Vaccine 2004; 22:2202-2208; PMID:15149778; http://dx.doi.org/10.1016/j.vaccine.2003.11.041
- Lin C, Holland RE, Jr, Donofrio JC, McCoy MH, Tudor LR, Chambers TM. Caspase activation in equine influenza virus induced apoptotic cell death. Vet Microbiol 2002; 84:357-65; PMID:11750143; http://dx.doi.org/10.1016/S0378-1135(01)00468-0
- Lin C, Zimmer SG, Lu Z, Holland RE, Dong Q, Chambers TM. The involvement of a stress-activated pathway in equine influenza virus-mediated apoptosis. Virology 2001; 287:202-13; PMID:11504555; http://dx.doi.org/10.1006/viro.2001.1010
- Zhirnov O, Konakova T, Garten W, Klenk H-D. Caspase-dependent N-terminal cleavage of influenza virus nucleocapsid protein in infected cells. J Virol 1999; 73:10158-63; PMID:10559331
- Horníčková Z. Different progress of MDCK cell death after infection by two different influenza virus isolates. Cell Biochem Funct 1997; 15:87-93; PMID:9253160; http://dx.doi.org/10.1002/(SICI)1099-0844(19970601)15:2%3c87::AID-CBF726%3e3.0.CO;2-6
- Mersich S, Baumeister E, Riva D, Lewis A, Cadario M, Pontoriero A, Savy VL. Influenza circulating strains in Argentina exhibit differential induction of cytotoxicity and caspase-3 in vitro. J Clin Virol 2004; 31:134-9; PMID:15364270; http://dx.doi.org/10.1016/j.jcv.2004.01.004
- Ritter JB, Wahl AS, Freund S, Genzel Y, Reichl U. Metabolic effects of influenza virus infection in cultured animal cells: Intra-and extracellular metabolite profiling. BMC Syst Biol 2010; 4:61; PMID:20465796; http://dx.doi.org/10.1186/1752-0509-4-61
- Huang D, Xia-Hou K, Liu X-P, Zhao L, Fan L, Ye Z, Tan WS, Luo J, Chen Z. Rational design of medium supplementation strategy for improved influenza viruses production based on analyzing nutritional requirements of MDCK Cells. Vaccine 2014; 32:7091-7; PMID:25444832; http://dx.doi.org/10.1016/j.vaccine.2014.10.067
- Rödig JV, Rapp E, Bohne J, Kampe M, Kaffka H, Bock A, Genzel Y, Reichl U. Impact of cultivation conditions on N‐glycosylation of influenza virus a hemagglutinin produced in MDCK cell culture. Biotechnol Bioeng 2013; 110(6):1691-703; PMID:23297157
- Yang M, Butler M. Effects of ammonia on CHO cell growth, erythropoietin production, and glycosylation. Biotechnol Bioeng 2000; 68(4):370-80; PMID:10745205
- Yang M, Butler M. Effect of ammonia on the glycosylation of human recombinant erythropoietin in culture. Biotechnol Prog 2000; 16:751-9; PMID:11027166; http://dx.doi.org/10.1021/bp000090b
- Gillmeister MP, Tomiya N, Jacobia SJ, Lee YC, Gorfien SF, Betenbaugh MJ. An HPLC-MALDI MS method for N-glycan analyses using smaller size samples: application to monitor glycan modulation by medium conditions. Glycoconj J 2009; 26:1135-49; PMID:19412663; http://dx.doi.org/10.1007/s10719-009-9235-z