Abstract
Leptospirosis is a bacterial zoonotic disease caused by an infection with a spirochete belonging to the genus Leptospira. In animals, leptospirosis displays a wide range of pathologies, including fever, abortion, icterus, and uveitis. Conversely, infection in humans is associated with multi-organ injury, resulting in an increased rate of fatalities. Pathogenic leptospires are able to translocate through cell monolayers at a rate significantly greater than that of non-pathogenic leptospires. Thus, vaccine approaches have been focused on targeting bacterial motility, lipopolysaccharides (LPSs), lipoproteins, outer-membrane proteins (OMPs) and other potential virulence factors. Previous studies have indicated that leptospiral proteins elicit long-lasting immunological memory in infected humans. In the study reported here, the efficacy of a synthetic consensus DNA vaccine developed against the Leptospira membrane lipoprotein LipL45 was tested. After in vivo electroporation (EP) mediated intramuscular immunization with a synthetic LipL45 DNA vaccine (pLipL45) immunized mice developed a significant cellular response along with the development of anti-LipL45-specific antibodies. Specifically, the pLipL45 vaccine induced a significant Th1 type immune response, indicated by the higher production of IL-12 and IFN-γ cytokines. The results presented here are the first demonstration that a LipL45 based DNA immunogen has potential as a anti-Leptospira vaccine.
Introduction
Leptospirosis is a serious and neglected bacterial infectious disease affecting humans as well as a wide array of other animals. The causal agent, belonging to the genus of spirochete bacteria, is Leptospira interrogans. The strains of Leptospira are antigenically and genetically diverse and are comprised of greater than 250 serovars (serotypes) and about 21 genomospecies (i.e genospecies).Citation1 The different serovars are based on the variations in the lipopolysaccahrides (LPS) of the leptospiral cell wall. In animals, leptospirosis is associated with fever, abortion, icterus and uveitis which can lead to partial or total blindness. In humans infections most frequently results in a self-limiting febrile disorder. However, more serious manifestations include Weil's disease characterized by kidney failure, icterus and hemorrhaging and is associated with a mortality rate varying between 5 and 15%. The most severe sequelae of infection is the leptosporosis associated pulmonary hemorrhage syndrome (LPHS) which has a mortality rate as high as 50–70%. Of significance is the fact that the mortality ratesCitation2,3 of both Weil's disease and LPHS are noted even with the use of antibiotic therapy.
In contemporary times, the disease has been observed with increasing incidence in both developing and developed parts of the world. The disease is predominantly transmitted by infectious urine from affected animals, with rodents being the primary hosts. Secondary hosts include, importantly, the domestic dog. The disease is predominantly of occupational significance with veterinarians, farmers and slaughterhouse workers being most susceptible, among others.Citation4,5 Recent recreational exposure to the infection in triathletes, rower and canoeists/kayackers have also been noted.Citation6
The limited success of conventional antibiotic therapy in the treatment of leptospirosis underscores the need for the development of an effective vaccine. However, the existence of over 250 serovars and 21 genomospecies, with their associated antigen variation, makes the development of an efficacious broad spectrum vaccine problematic.Citation7
Leptospires have evolved mechanisms to evade the immune defense. The rapid translocation potential of pathogenic leptospires through the tissues allows them to quickly reach the bloodstream and disseminate to multiple organs.Citation8 Thus, the rapid entry and establishment of the pathogen becomes a major challenge to the development of an highly efficacious and safe vaccine.Citation9 Although whole cell vaccine models have been developed, such vaccines have drawbacks in conferring protection against heterologous serovars.Citation10 Among several subunit recombinant vaccine trials for leptospirosis, OMP's, lipoproteins, and virulent factors have generated a considerable interest.Citation11 These vaccine candidates are known however to elicit immunity only against homologous serovars, or fail to induce long lasting immunological memory. Therefore, an effective vaccine candidate needs to induce immunity across heterologous serovars and induce long lasting immune response to control leptospirosis among humans and animals and be easy to manufacture, handle and transport to remote areas without the need for a refrigerated cold chain.Citation12-15 Historically, studies have indicated that protective immunity against Leptospira is mediated likely by humoral immune responses. The role of antibody based immunity was suggested from very early studies done shortly after the discovery of Leptospira and its implication in disease when it was demonstrated that protective immunity against Leptospira could be transferred to guinea pigs by the passive adminstration of immune sera.Citation16 Virtually all other subsequent studies have indicated the importance of humoral immunityCitation17 except for one which demonstrated a role for cellular immunity in protection.Citation18 As well, a number of different vaccine strategies against various antigens from Leptospira have been utilized including bacterins (killed preparations of bacteria), live attenuated vaccines, in addition to lipopolysaccharide and recombinant protein vaccines.Citation19
Specifically, a number of leptospiral proteins have been targeted as possible vaccine candidates including LipL32,LipL41, LipL45 and OmpL1, among others.Citation11,20-22 LipL32 has been the most extensively studied leptospiral protein and it has been suggested to be an important virulence factor for infection as well as being a potentially protective antigen.Citation23 The putative therapeutic efficacy of immune responses against this protein was based, in part, on the ability of an anti-LipL32 monoclonal antibody to protect against Leptospira infection in hamsters.Citation24 The protein is also relatively highly conserved among leptospiral serovars; and, importantly, sera from Leptospira infected patients recognize this lipoprotein.Citation25
Vaccine studies targeting LipL32 have utilized adenoviral vector deliveryCitation26 recombinant protein immunogensCitation27,28 as well as naked DNA plasmid vaccines.Citation29 A more recent strategy targeting LipL32 involved a DNA prime:recombinant protein boost regimen.Citation30 However, vaccine study results targeting LipL32 have yielded mixed findings including only partial protection as well as concerns about inadequate leprospira challenge doses and statistical methods.Citation17,19
In the study presented here our group decided to target a related leptospiral protein, designated LipL45, a 45 kDal protein which is subsequently processed to a 31 kDal membrane protein called P31-LipL45.Citation31 LipL45 has been hypothesized to be an important component of the Leptospira infection process and thus can be considered a putative virulence factor.Citation31 Likewise, hamsters infected with several different leptospiral serovars induce humoral immune responses against LipL45. In addition, the LipL45 protein is recognized by sera from human leptospirosis patients.Citation25,31
Therefore, based upon these observations LipL45 is hypothesized to be a potential vaccine target against Leptospira infection. As such, our group, based on an interest in DNA-based vaccine development, devised and performed an initial immunogenicity evaluation of a novel enhanced consensus DNA vaccine expressing LipL45. To our knowledge this is the first report of an evaluation of a DNA vaccine construct targeting the leptospiral LipL45 protein.
DNA-vaccines provide a potentially attractive immunization strategy. These vaccines induce antigen-specific immune responses following the direct injection of non-replicating plasmids into a host target tissue. Once injected, plasmids drive the synthesis of specific foreign proteins within the immunized host and, as such, mimic natural infection in terms of inducing immune responses. The host provides post-translational modifications to antigen that typically and accurately reproduce native conformations. These host-synthesized proteins then become a target for immune surveillance via both the MHC class-I and class-II pathways. These processes lead to elicitation of protective immunity against an infectious agent, primarily by activating both the humoral and cellular arms of the immune system. In particular, DNA vaccines have been demonstrated to specifically drive, when delivered by the intramuscular route, Th1 immune responses.Citation32-34 This has been hypothesized to be due to the induction by this route of inflammation and IL-12 secretion which stimulates the Th1 immune responses. Moreover, DNA vaccines can be constructed to function with many safety features as well as the specificity of a subunit vaccine. There is little risk of reversion to a disease-causing form, and there is no concern for secondary infection as the material injected is non-replicating, and non-infectious. As well, DNA vaccines are highly flexible with genes which encode multiple important immunologic epitopes being able be included in a single vaccine preparation. Also, targeted therapeutic epitopes can be included in the constructs while those that confer pathogenicity, virulence, immunologic inhibition, or cross-reactivity (i.e. autoimmunity) can be altered or deleted.
Other characteristics of DNA-based immunization strategies bring a number of advantages to this technology and further make it an attractive vaccine platform.Citation35 Specifically stimulation of pre-existing, autoimmune or inhibiting humoral or cellular immune responses has not been an issue with plasmid DNA, allowing for the boosting of antigen specific immune response by multiple immunizations while, at the same time, avoiding tolerization.Citation35,36 Plasmid DNA is also simpler to manipulate and produce than viral vector-based vaccines.Citation14 As well, they are more stable than live attenuated viral vector based vaccines, which typically require a cold-chain refrigeration method for storage. As previously indicated, DNA vaccines have been demonstrated to elicit humoral and cellular responses and confer protection in small animal models.Citation37 Taken together, these considerable advantages establish DNA-based immunogens as a potentially useful vaccine modality against infectious as well as other diseases.Citation14,38,39
Recently, the utility of DNA vaccines against various infectious diseases has gained renewed interest due, in part, to the apparent ability of delivery enhancement through in vivo electroporation (EP) to increase the immunogenicity and effectiveness of the vaccine strategy. As a result, efforts have been made to identify a more effective DNA vaccine candidate that is derived from a single antigen or multiple antigen targets.Citation37,38,40-42 DNA vaccines have been previously utilized against Leptospira, specifically targeting the OmpL1,Citation12 hemolysin associated protein 1 as well as LigA, the Leptospira immunoglobulin like protein A.Citation43 However, as indicated, another attractive vaccine target in this spirochete bacteria is the leptospiral lipoprotein LipL45 since this is an important virulence-associated protein expressed during infection.Citation31 Previous studies have demonstrated that the LipL45 can elicit long lasting immunological memory in patients infected with leptospires. However, to date, broad protective efficacy has not been effectively elicited by any of these anti-Leptospira vaccine preparations.
Considering the limitations affecting the development of a vaccine for leptospirosis, due at least in part to the existence of a large number of diverse serovars, the study reported here utilizes a consensus optimized DNA vaccine targeting the LipL45 protein with the goal of eliciting robust humoral and cellular immune responses.
Results
Construction and characterization of LipL45 plasmid and in vitro assessment of expression
Consensus sequences were generated for the gene encoding leptospiral LipL45 by techniques described in the materials and methods section. Subsequently, codon and mRNA optimization was performed. The Kozak sequence followed by a Ig-E leader sequence, which consist of 18 amino acids, were incorporated into the N terminus region. The synthesized sequences were inserted into a pVax1 expression vector as described previously.Citation44 The schematic representation of the DNA construct pLipL45 is indicated in . The insertion of LipL45 DNA was confirmed using single and double restriction enzyme digestion and agarose gel electrophoresis (). Confirmation of protein expression from pLipL45 was confirmed by an immunoblotting assay of transfected HEK293 protein extracts by SDS-PAGE. As expected, specific bands of the leprospiral LipL45 protein (45kDa) were detected in pLipL45 transfected cells () using sera from mice immunized with pLipL45.
Figure 1. Strategies utilized for LipL45 plasmid generation and confirmation of expression. (A) Schematic representation of plasmid map containing LipL45 gene, Kozak and IgE leader sequence along with restriction endonuclease sites. (B) Agarose electrophoresis gel photo indicating the LipL45 construct in the pVax1 expression vector. Lane 1: pLipL45 undigested DNA; Lane 2: LipL45 construct with single digestion of construct with KpnI; Lane 3: KpnI & NotI double digestion of the construct which releases the insert (1227bp): Lane 4: 1 kb control size markers. (C) Western blot analysis of pLipL45 expression. The expression of pLipL45 protein was analyzed 2 d post transfection by Western blotting using sera from the pLipL45 DNA vaccinated mice at 1:100 dilutions.
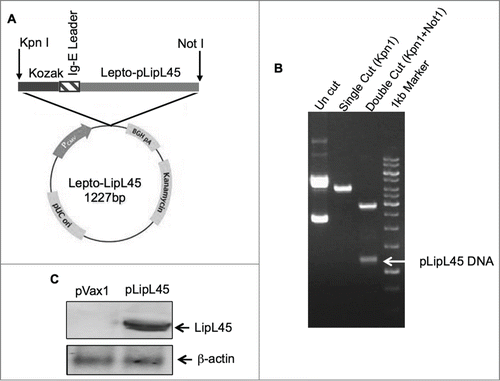
Cellular immune responses measured after immunization with pLipL45
CD8+ T lymphocytes are one of the most crucial components of antitumor and antiviral immune effector mechanisms.Citation36 To quantify anti-LipL45-specific CD8+ T-cell frequencies generated by the pLipL45 vaccine, ELISPOT assays were performed to determine the expression level of IFN-γ in splenocytes as spot forming unit (SFU). Because IFN-γ is a critical cytokine associated with the Th1 immune responses,Citation35 analysis was focused on the production of this cytokine in the vaccinated mice. Balb/C mice (n = 4/group) were vaccinated 3 times with 100 µg of pLipL45 or with 100 µg of the control plasmid pVax1 (). The vaccines were delivered in 25 µl of PRIS by in vivo EP. Animals were sacrificed on day 35 (7 d after the third vaccination) followed by isolation of splenocytes for immune analysis by IFN-γ ELISpot. The experiment was performed against a library of peptides spanning the entire LipL45 protein. The overall analysis demonstrated that the pLipL45 vaccine was able to induce high levels of IFN-γ in mice after 3 immunizations, when compared to mice in pVax1 vaccinated group. Furthermore, the breadth of T-cell responses was surmised to be important for the immune responses. Accordingly, individual peptide pool were used to confirm the matrix mapping and reaction against its homologous library. Reactions occured reacted to 17 peptide pools (15mer, 11aa overlapping) in the mix and revealed the LipL45 sequence “KSKVDIQFADGSAVR” to be the strongest immuno-dominant epitope (). The dominant peptide listed was confirmed to contain one H2-Db–restricted epitope by using Immune Epitope Database (IEDB) analysis resource Consensus tool (http://tools.immuneepitope.org), suggesting effective processing of this antigen.
Figure 2. Vaccination regimen and measurement of cellular immune responses. (A) Balb/C mice were immunized intramuscularly 3 times biweekly with 100 μg of pLipL45. Splenocytes were isolated from groups of 2 mice 1 week after the last vaccination. (B) Measurement of IFN-γ levels in pooled splenocytes pLipL45 or pVax1 vaccinated mice. Specifically, splenocytes were stimulated by individual LipL45 peptide pools followed by ELISpot assay analysis, measuring the production of IFN-γ spot forming cells (SFC). Experiments were performed twice with similar results and error bars indicated are the standard error of the mean. (C and D) Direct measurement of IFN-γ and IL-12 cytokine production by splenocyte cultures from vaccinated mice. Specifically, levels of IFN-γ (C) or IL-12p70 (D) were measured in supernatants from spleen cell cultures of mice vaccinated with pLipL45 or control pVax1 vector and restimulated in vitro with 50 μg of the LipL45 peptide pools. Cytokine production by the unstimulated cells was subtracted from that by the stimulated cells in each experiment. Splenocytes were collected from 4 mice per group one week after the final immunization to detect the levels of IFN-γ and IL-12 p70. The experiment was repeated yielding similar results. Data from 2 independent experiments were included in the analysis. Data are expressed as mean ± SD. Statistically significant differences (P < 0.0001) in levels of the cytokines were apparent, when compared with the negative control.
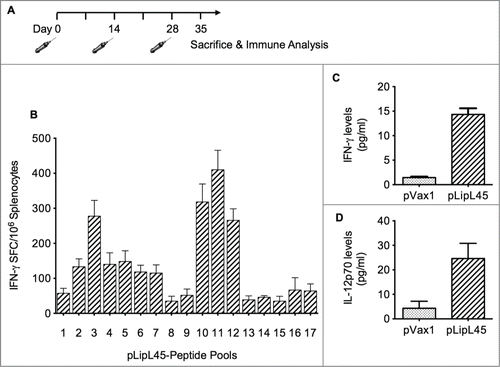
Cytokine levels in pLipL45 immunized mice
Th1 and Th2 cytokines have different roles in the polarization of immune responses. Th1 cytokines are thought to drive induction of cellular immunity, particularly CTLs, whereas Th2 cytokines preferentially drive humoral immunity. Furthermore, DNA vaccination has been demonstrated to stimulate and increase the in vitro proliferative response of splenocytes. Therefore, measurement of different cytokines released from splenocytes from pLipL45 vaccinated mice and stimulated with LipL45 peptide pools was performed. Specifically, to understand the role of the pLipL45 vaccine in the development of immune responses, the effects of this vaccine on changes in Th1 and Th2 phenotypes was evaluated. Analysis of splenocytes from mice immunized with pLipL45 secreted much higher levels of IFN-γ and IL-12 than the splenocytes from mice immunized with the pVax1 control vaccine (P < 0.0001) (). Results clearly support that vaccination with pLipL45 in mice enhanced the magnitude of IFN-γ and IL-12 production. This shows that, because both IL-12 and IFN-γ production are considered Th1 responses, the consensus leptospiral DNA vaccine induces Th1 mediated immune responses.
Induction of significant effector memory T cell responses in mice vaccinated with pLipL45
The functionality of CD8 mediated effector responses induced by vaccination with pLipL45 was evaluated. In order to characterize memory T cell responses induced by the pLipL45 vaccine, the expression of memory markers (CD44 and CD62L) in the CD8+ T cell populations was assessed. One week after the third pLipL45 immunization, CD8+ T effector memory responses () were measured, with the data indicating that pLipL45 was able to induce significant levels of effector memory CD8+ T cells, which produced type 1 cytokines.
Figure 3. Increased frequencies of memory Tcells following immunization with pLipL45. (A) Representative FACS plots measuring effector memory (TEM) and central memory (TCM) CD8+T cells from pLipL45 immunized mice. One week after the last immunization with pLipL45 or the empty vector pVax1, spleen cells from 4 Balb/c mice were cultured in the presence of pooled LipL45 peptides. Splenocytes from immunized mice were stained with surface markers CD3 (APC), CD8 (PerCP), CD44 (FITC) and CD62L (PE), which are appropriate for assessing effector and central T cell memory responses. (B) Simultaneous assessment of analysis of CD44 and CD62L expression was determined on gated CD3+CD8+ cells. Effector (CD44hi CD62Llow) T cell levels are depicted. Cells (105) from mice immunized with pVax1 or pLipL45 (n = 4) were stained, gated and analyzed using FlowJo software. Samples from individual mice were analyzed and values are expressed as mean± SE.
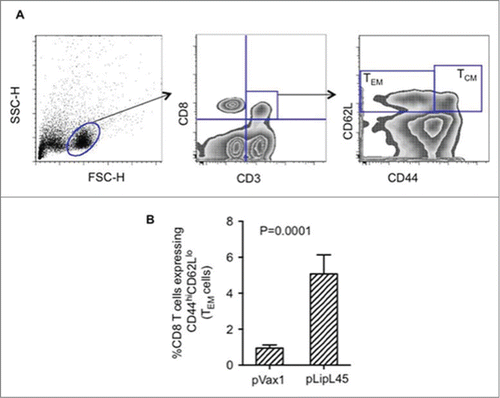
Anti-LipL45 sera IgG responses in mice after pLipL45 immunization
In order to estimate the antigen-specific humoral immune responses after immunization with pLipL45, antibody ELISA were measured on the sera collected from the mice immunized with pLipL45 or pVax1. The pLipL45 vaccine elicited humoral immune response as evidenced by the significant difference in the OD values between pLipL45 and pVax1 groups (). This profile of IgG suggested that the novel consensus DNA vaccine strategy used in this study elicited a significant antigen specific humoral immune response.
Figure 4. Measurement of anti-LipL45 specific antibody immune responses after pLipL45 vaccination. Following immunization of female BalbC mice with pLipL45 antigen specific humoral immune responses were measured. The quantitation was made one week after the final immunization. (A) Specific OD450nm ± SD values in pLipL45 and control pVax1 vaccinated mice as a function of sera dilution. (B) Ratio of antigen specific IgG2a/IgG1 levels measured in sera from pLipL45 from pVax1 vaccinated mice one week after the final immunization. The total number of mice in the analysis described for (A) and (B) above was 4. (C) Binding, measured by ELISA, sera from antibodies pLipL45 immunized mice to different LipL45 peptide pools identified a dominant MHC II LipL45 epitope.
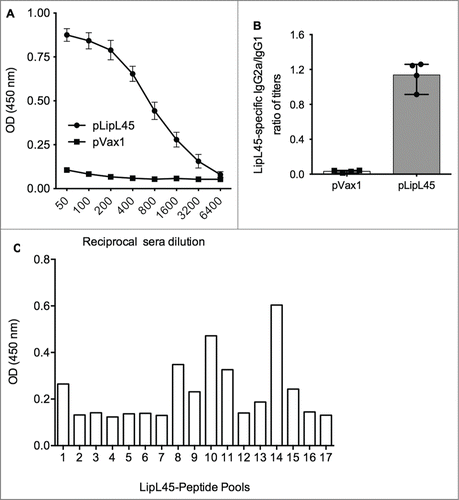
In order to determine whether a Th1 and/or Th2 response was elicited in the pLipL45 immunized mice, the specific IgG1 and IgG2a subclasses were analyzed by ELISA one week after the final immunization. As depicted in , high levels of specific anti-LipL45 IgG2a, indicated by the ratios of IgG2a to IgG1 levels, suggested that the consensus pLipL45 elicited a more Th1-type immune response. The specificity of anti-LipL45 protein binding antibodies, through identifying their cognate epitopes was also evaluated. Sera from pLipL45-vaccinated mice were tested in a peptide-based ELISA to examine the presence of peptide specific anti-LipL45 antibodies. The results of these analyses are shown in . The dominant MHC-II peptide “EKNQASVKADKSLKD” was identified. The MHC-II binding predictions were made using the IEDB analysis resource Consensus tool.
Discussion
Vaccine strategies have been demonstrated to be very successful against a number of significant pathogens. However, for some pathogen targets there are obstacles that exist which limit the development and use of vaccines. Some of these include the cost of production, delivery, and safety.Citation45 Plasmid DNA vaccine strategies have recently generated promising results against a number pathogens, due in part to the effective use of in vivo electropration as a delivery enhancement method.Citation15,46,47 DNA vaccines also induce humoral and cellular immune responses typically without the need for use of an adjuvant.Citation46 Specifically, DNA vaccine candidates have been developed against viral infection such as HIV, hepatitis B, hepatitis C, influenza, dengue and Chikungunya virus, among others. The results of these studies have demonstrated both humoral and cellular immune responses and/or protective immunity in relevant animal models.Citation13,38,41,42,48-50 However, few bacterial pathogen antigens have been effectively targeted for DNA vaccine development.
In the current study, a consensus optimized DNA construct encoding the leptospiral protein LipL45 was generated in order to determine whether this vaccine could elicit strong anti-LipL45 cellular and humoral immune responses. Historically, the leptospiral protein LipL45 has found to be immunogenic and could elicit long lasting immune responses. This conclusion was based on immunoblot data using sera collected periodically from individuals over a period of 4 y who suffered from leptospirosis (unpublished data). The constructs for the DNA vaccine used in our study was based on a consensus sequence of leptospiral proteins for LipL45 with several modifications, including codon and RNA optimization, the addition of a Kozak sequence and an IgE leader sequence.Citation51 In vitro protein expression of LipL45 was confirmed in HEK 293 cell lines by Western blot analysis. The observations made through a series of experiments such as ELISpot analysis indicates elevation of IL-12p70 levels and effector memory function of T cells in mice that were vaccinated with pLipL45. The amino acid sequence “KSKVDIQFADGSAVR” within the full length LipL45 protein was identified as an immuno-dominant epitope. These results imply that targeting LipL45 could elicit an effective cellular immune response against Leptospira that may provide a viable defense against infection.
In addition, investigations of the antigen-specific humoral responses after immunization with pLipL45 indicated a significant sera humoral response from vaccinated mice. Furthermore, by evaluating the ratio of antigen specific IgG2a/IgG1 levels, immunization with the DNA vaccine showed a trend toward a Th1 response, with a balanced ratio and secretion of IFN-γ and Th1 cytokines. This result suggested that Th1 immune responses were dominant after pLipL45 vaccination. Furthermore, memory T cells may be critical for generating long-term immunity and vaccine-induced infection control. In this study we found that after pLipL45 immunization, leptospiral specific CD8+ T cells stimulated IFN−γ and IL-12 production upon vaccination, indicative of a TEM phenotype. As well, elevation in IL-6 could support B cell growth, which is evident by the elevation in Th2 immune responses. Therefore, it can also be inferred that this construct could induce antibody response.Citation52
In conclusion, the pLipL45 plasmid based vaccine was able to induce significant antigen-specific CD8+ CTL and effector memory responses, Th1 responses, and IgG2a type antibodies. As indicated earlier the leptospiral protein LipL45 is considered a strong putative vaccine target due to it being significantly associated with the stimulation of Leptospira virulence.Citation53 This protein was found to be highly immunogenic and capable of eliciting both humoral and cell mediated immune responses. Therefore, it was concluded that a plasmid encoding LipL45 is the good candidate for the development of DNA based vaccines against leptospiral infection in humans.
Materials and Methods
Cloning of the consensus leptospiral protein LipL45 DNA vaccine
The consensus DNA sequences for the leptospiral protein LipL45 were generated by using 34 sequences of different serovars/strains available in the NCBI database. The Cluster X program (Version 1.81) was used to align the sequences and select the consensus amino acid sequence. The consensus sequence was optimized for expression, including codon and RNA optimization (Gene Optimizer™, GeneArt, Regensburg, Germany).Citation54 A Kozak sequence as well as an IgE leader sequence was incorporated in the N terminal region of the construct in order to enhance the expression and ultimately immunogenicity of the plasmid based vaccine. Specifically, the Kozak sequence (GCCACC) has been demonstrated to be necessary to preced the ATG start codon in order to drive protein translation.Citation55,56 As well, incorporation of an IgE leader sequence (MDWTWILFLVAAATRVHS) has been demonstrated to enhance translation of the construct in addition to increasing the immunogenicity of the translated product.Citation57,58 The sequence was then synthesized commerciallyCitation50,54 and cloned into the pVax1 expression vector (Invitrogen) between restriction sites KpnI and NotI. The production of the DNA construct for vaccine use was performed by Aldevron (Fargo, North Dakota, US). Purified plasmid DNA was formulated in water for immunization. The LipL45 and control expressing plasmids were designated pLIpL45 and pVax1.
Confirmation of expression of pLipL45 in HEK293T cell
Protein expression from pLipL45 was confirmed by transfection of the construct in HEK293 cells. Briefly, HEK293 cells were maintained in 25 cm2 flasks in Dulbeco's modified Eagle's medium (Life Technologies), supplemented with 100 IU/ml penicillin, 100 µg/ml streptomycin, and 10% (v/v) fetal bovine serum (Life Technologies). When adequate confluence was attained, the cells were transfected with 15 µg of pLipL45 or pVax1 plasmid DNA and pre–condensed with Lipofectamine (Invitrogen) in serum- free DMEM, as specified in the manufacturer's instructions. After 48 hours of incubation at 37°C, the cells were scraped and washed with Phosphate Buffer Saline (PBS, pH 7.2). Proteins were then extracted using lysis buffer (100 mM Tris pH7.4, 300 mM NaCl, 10% NP40, 10% Sodium Deoxycholate, 200 mM PMSF, 1X Protease inhibitor cocktail and 1X Phosphatase inhibitor cocktail). The concentration of total protein was estimated using the BCA method (Sigma Aldrich). Subsequently, 50 µg of protein was re-suspended in 2X SDS- PAGE buffer followed by fractionation on SDS–PAGE (12%) gel and then transferred to PVDF membrane (Bio-Rad, Hercules, CA). The blotted PVDF membrane was then blocked overnight (3% bovine serum albumin (BSA) in 10 mM Tris (PH 8.0), 150 mM NaCl, 0.05% Tween 20) was carried out at 4°C. Anti-LipL45 sera, collected from immunized mice was used as a detection antibody. After 1 hour of incubation at 37°C the blot was washed and a 1:2000 dilution of alkaline phosphatase conjugated anti-mice IgG (Sigma Aldrich) was added and incubated at 37°C for 1 hour. The blots were then developed and visualized through enzymatic reaction of BCIP/NBT (5-Bromo-4-chloro-3-indolyl phosphate dipotassium/nitrotetrazolium blue chloride).Citation38
Animals and immunization schedule
Balb/c female mice, aged 6–8 weeks, were used in this study. The animals were procured from National Center for Laboratory Animal Sciences (NCLAS, ICMR) Hyderabad. The experiments were conducted according to the protocol approved by the Institutional Animal Ethics Committee (IAEC). The mice were grouped into 2 categories and each group was comprised of 8 animals. The DNA constructs LipL45 and pVax1 were injected into each group of animals separately. Each animal received 100 µg of construct per dose. Subsequently, 2 booster doses on the 14th day and 28th day were also given to the respective groups of mice. All immunizations were delivered into the quadriceps muscles in a total volume of 25 μl followed by intramuscular electroporation (CELLECTRA®; Inovio Pharmaceuticals. PA, USA) by methods and pulsing conditions as previously described.Citation50 The animals were sacrificed 7 d after the last immunization, with individual groups consisting of 4 spleens each. Splenocytes were collected and prepared aseptically from the spleens and used for the ELISpot as well as quantification of cytokine expression. Sera was also collected for IgG antibody screening.
ELISpot assay
An ELISpot 96-well plate was coated with anti-mouse IFN-γ capture antibody (R&D Systems) and incubated overnight at 4°C. The plate was then washed and blocked for 2 hours with 1% BSA. Afterwards, 2 × 105 splenocytes from immunized Balb/c mice were added to the ELISpot plate. Sixty eight peptides (67 peptides of 15-mers and one 16-mer peptide) with 9 amino acid overlaps of 407 residues of LipL45 protein of Leptospira were synthesized (Eurogentec, Belgium) and used. Matrix based peptide pools were prepared and mixed with the media, splenocytes stimulated in their respective groups, and allowed to incubate at 37°C in the presence of 5% CO2. After 24 hours of incubation, the cells were washed, followed by addition of incubated overnight at 4°C in the presence of biotinylated anti-mouse IFN-γ (R&D Systems). The plates were washed and streptavidin-alkaline phosphatase conjugate (R&D Systems) was added to each well and incubated for 2 hours at room temperature (27 ± 1°C). After the wash, the chromogen BCIP/NBT was added to each well and incubated for 30 min at room temperature in the dark. The plates were rinsed with distilled water and dried at room temperature. The spots were quantified using stereo-microscopy. The raw values were normalized to spot forming units (SFU) per million splenocytes.Citation38 In order to identify the immunodominant T cell epitope, 2 different pools were identified based on the stimulation response resulting in a rise in levels of IFN-γ among the peptide pool sharing the common peptide from the matrix table.Citation59
Cytometric bead array determination of levels of relevant cytokines in tissue culture media from cultured splenocytes isolated pLipL45 vaccinated mice splenocyte
Tissue culture media from splenoytes cultured from pLipL45-vaccinated mice were analyzed for cytokine profiles by a protocol provided by the kit manufacturer (BDTM Cytometric Bead Array (CBA) Mouse Inflammation kit, Becton, Dickinson and Company). Briefly, cytokine standards were prepared and the samples diluted. The required quantities of capture beads for specific cytokines were mixed and 50 ml was distributed to the FACS tubes labeled for standards as well as for experimental (pLipL45) and control (pVax) samples. Equal volumes of detection antibody were then subsequently added to all the tubes. Finally, serially diluted standards and experimental or control samples were added to the appropriate tubes, mixed and then incubated in the dark for 2 hours at room temperature. Subsequently, the beads in all of the tubes were washed and resuspended in buffer. Samples were then measured for cytokine levels using the BD FACS Canto II (BD Biosciences) cytometer. Data from the standard, experimental and control samples were analyzed to determine the levels of cytokines using FACS DIVA software (BD Biosciences). Values were then exported and analyzed further and compared between the different groups using the FCAP array software program.
ELISA measurement of anti-LipL45 IgG responses
The humoral immune responses in pLipL45 vaccinated and control groups were determined. Anti-LipL45 antibodies were detected by an indirect ELISA test. A previously developed methodology has been adopted and used.Citation60,61 Briefly 96 well flat bottom polystyrene plates (Corning, NY) were individually coated and incubated overnight at 4°C with the LipL45 peptide pools. Plates were then washed with PBS-T (PBS, 0.05% Tween 20), blocked for 1h with 3% BSA in PBST, and diluted sera (1:100) from immunized and control mice were added in duplicate and incubated for 1 hour at 37°C. After washing, a 1:5000 dilution of rabbit anti-mouse HRP conjugated IgG (Sigma Aldrich, USA) was added and the antigen-antibody complex was detected by the addition of chromogenic substrate solution OPD (Sigma Aldrich). After incubation the enzymatic reaction was stopped by 1M H2SO4 and the optical density (OD) was measured at 490 nm by using a Teccan ELISA reader. The dominant epitope of LipL45 capable of eliciting humoral immune response was identified from the highly reactive pools on the basis of high OD values among the peptide pools sharing a common peptide, and analyzed by a matrix table.Citation59 In order to determine whether a Th1 and/or Th2 response was elicited in the immunized mice, the specific IgG1 and IgG2a subclasses were analyzed one week following the final immunization by ELISA using rabbit anti-mouse HRP conjugated IgG1 and IgG2a (Sigma Aldrich, USA).
Flow cytometric analysis
In order to determine changes in specific T cell subsets in mice immunized with the pLipL45 vaccine, a flow cytometry assay was performed. Splenocytes (1 × 106) were collected from the immunized mice one week after the third immunization and were suspended in PBS. Splenocytes were then first prestained with LIVE/DEAD Fixable Violet Dead Cell Stain Kit, and were then surface-stained for CD3 (clone 500A2; eBioscience), CD8 (clone 53–6.7; eBioscience), CD62L (clone MEL-14, eBioscience) and CD44 (clone IM7; eBioscience) and washed 3 times in PBS + 1% FBS. All data was collected using a LSRII flow cytometer (BD Biosciences) and were sorted into CD8+CD44+CD62Llo and CD8+CD44+CD62Lhi populations and analyzed using FlowJo software (Tree Star, Ashland, OR).
Statistical analysis
The results were analyzed using GraphPad Prism 5.04 (San Diego, California, United States) for Windows 7. Data was collected from cellular assays and presented as the mean ± 2 standard deviations, which was calculated from triplicate wells of pooled samples from each experimental group. Analysis of differences between groups was performed using independent samples t-test.
Disclosure of Potential Conflicts of Interest
No potential conflicts of interest were disclosed.
Funding
This work was supported by the Indian Council of Medical Research, Department of Health Research, Ministry of Health and Family Welfare, Government of India (Ref No: 30/3/31/2008/ECD-II), New Delhi, India.
References
- Levett PN. Systematics of leptospiraceae. Curr Top Microbiol Immunol 2015; 387:11-20; PMID:25388130
- Gouveia EL, Metcalfe J, de Carvalho AL, Aires TS, Villasboas-Bisneto JC, Queirroz A, Santos AC, Salgado K, Reis MG, Ko AI. Leptospirosis-associated severe pulmonary hemorrhagic syndrome, Salvador, Brazil. Emerg Infect Dis 2008; 14:505-8; PMID:18325275; http://dx.doi.org/10.3201/eid1403.071064
- Terpstra WJ. Human leptospirosis: guidance for diagnosis, surveillance and control. Geneva: WHO/ILS, WHO library; 2003:109.
- Meites E, Jay MT, Deresinski S, Shieh W-J, Zaki SR, Tompkins L, Smith DS. Reemerging Leptospirosis, California. Emerg Infect Dis 2004; 10:406-12; PMID:15109405; http://dx.doi.org/10.3201/eid1003.030431
- Vijayachari P, Sugunan A, Shriram A. Leptospirosis: an emerging global public health problem. J biosci 2008; 33:557-69; PMID:19208981; http://dx.doi.org/10.1007/s12038-008-0074-z
- Pappas G, Papadimitriou P, Siozopoulou V, Christou L, Akritidis N. The globalization of leptospirosis: worldwide incidence trends. Int J Infect Dis 2008; 12:351-7; PMID:18055245; http://dx.doi.org/10.1016/j.ijid.2007.09.011
- Cerqueira G, Picardeau M. A century of Leptospira strain typing. Infect Genet Evol 2009; 9:760-8; PMID:19540362; http://dx.doi.org/10.1016/j.meegid.2009.06.009
- Barocchi MA, Ko AI, Reis MG, McDonald KL, Riley LW. Rapid translocation of polarized MDCK cell monolayers by Leptospira interrogans, an invasive but nonintracellular pathogen. Infect Immun 2002; 70:6926-32; PMID:12438371; http://dx.doi.org/10.1128/IAI.70.12.6926-6932.2002
- Merien F, Baranton G, Perolat P. Invasion of Vero cells and induction of apoptosis in macrophages by pathogenic Leptospira interrogans are correlated with virulence. Infect Immun 1997; 65:729-38; PMID:9009336
- Sonrier C, Branger C, Michel V, Ruvoen-Clouet N, Ganiere JP, Andre-Fontaine G. Evidence of cross-protection within Leptospira interrogans in an experimental model. Vaccine 2000; 19:86-94; PMID:10924790; http://dx.doi.org/10.1016/S0264-410X(00)00129-8
- Wang Z, Jin L, Wegrzyn A. Leptospirosis vaccines. Microb Cell Fact 2007; 6:39; PMID:18072968; http://dx.doi.org/10.1186/1475-2859-6-39
- Maneewatch S, Tapchaisri P, Sakolvaree Y, Klaysing B, Tongtawe P, Chaisri U, Songserm T, Wongratanacheewin S, Srimanote P, Chongsa-nguanz M, et al. OmpL1 DNA vaccine cross-protects against heterologous Leptospira spp. challenge. Asian Pac J Allergy Immunol 2007; 25:75-82; PMID:17891923
- Ferraro B, Morrow MP, Hutnick NA, Shin TH, Lucke CE, Weiner DB. Clinical applications of DNA vaccines: current progress. Clin Infect Dis 2011; 53:296-302; PMID:21765081; http://dx.doi.org/10.1093/cid/cir334
- Kutzler MA, Weiner DB. DNA vaccines: ready for prime time? Nat Rev Genet 2008; 9:776-88; PMID:18781156; http://dx.doi.org/10.1038/nrg2432
- Gurunathan S, Klinman DM, Seder RA. DNA vaccines: immunology, application, and optimization. Annu Rev Immunol 2000; 18:927-74; PMID:10837079; http://dx.doi.org/10.1146/annurev.immunol.18.1.927
- Ido Y, Hoki R, Ito H, Wani H. The prophylaxis of Weil's disease (spirochaetosis Icterohaemorrhagica). J Exp Med 1916; 24:471-83; PMID:19868055; http://dx.doi.org/10.1084/jem.24.5.471
- Adler B, de la Pena Moctezuma A. Leptospira and leptospirosis. Vet Microbiol 2010; 140:287-96; PMID:19345023; http://dx.doi.org/10.1016/j.vetmic.2009.03.012
- Naiman BM, Alt D, Bolin CA, Zuerner R, Baldwin CL. Protective killed Leptospira borgpetersenii vaccine induces potent Th1 immunity comprising responses by CD4 and gammadelta T lymphocytes. Infect Immun 2001; 69:7550-8; PMID:11705932; http://dx.doi.org/10.1128/IAI.69.12.7550-7558.2001
- Adler B. Vaccines against leptospirosis. Curr Top Microbiol Immunol 2015; 387:251-72; PMID:25388138
- Dong H, Hu Y, Xue F, Sun D, Ojcius DM, Mao Y, Yan J. Characterization of the ompL1 gene of pathogenic Leptospira species in China and cross-immunogenicity of the OmpL1 protein. BMC Microbiol 2008; 8:223; PMID:19087358; http://dx.doi.org/10.1186/1471-2180-8-223
- Cullen PA, Xu X, Matsunaga J, Sanchez Y, Ko AI, Haake DA, Adler B. Surfaceome of Leptospira spp. Infect Immun 2005; 73:4853-63; PMID:16040999; http://dx.doi.org/10.1128/IAI.73.8.4853-4863.2005
- Haake DA, Chao G, Zuerner RL, Barnett JK, Barnett D, Mazel M, Matsunaga J, Levett PN, Bolin CA. The leptospiral major outer membrane protein LipL32 is a lipoprotein expressed during mammalian infection. Infect Immun 2000; 68:2276-85; PMID:10722630; http://dx.doi.org/10.1128/IAI.68.4.2276-2285.2000
- Murray GL. The lipoprotein LipL32, an enigma of leptospiral biology. Vet Microbiol 2013; 162:305-14; PMID:23206414; http://dx.doi.org/10.1016/j.vetmic.2012.11.005
- Maneewatch S, Sakolvaree Y, Saengjaruk P, Srimanote P, Tapchaisri P, Tongtawe P, Klaysing B, Wongratanacheewin S, Chongsa-Nguan M, Chaicumpa W. Monoclonal antibodies to LipL32 protect against heterologous Leptospira spp. challenge. Hybridoma (Larchmt) 2008; 27:453-65; PMID:19108618; http://dx.doi.org/10.1089/hyb.2008.0056
- Guerreiro H, Croda J, Flannery B, Mazel M, Matsunaga J, Galvao Reis M, Levett PN, Ko AI, Haake DA. Leptospiral proteins recognized during the humoral immune response to leptospirosis in humans. Infect Immun 2001; 69:4958-68; PMID:11447174; http://dx.doi.org/10.1128/IAI.69.8.4958-4968.2001
- Branger C, Sonrier C, Chatrenet B, Klonjkowski B, Ruvoen-Clouet N, Aubert A, André-Fontaine G, Eloit M. Identification of the hemolysis-associated protein 1 as a cross-protective immunogen of Leptospira interrogans by adenovirus-mediated vaccination. Infect Immun 2001; 69:6831-8; PMID:11598056; http://dx.doi.org/10.1128/IAI.69.11.6831-6838.2001
- Luo D, Xue F, Ojcius DM, Zhao J, Mao Y, Li L, Lin X, Yan J. Protein typing of major outer membrane lipoproteins from Chinese pathogenic Leptospira spp. and characterization of their immunogenicity. Vaccine 2009; 28:243-55; PMID:19796723; http://dx.doi.org/10.1016/j.vaccine.2009.09.089
- Grassmann AA, Felix SR, dos Santos CX, Amaral MG, Seixas Neto AC, Fagundes MQ, Seixas FK, da Silva EF, Conceição FR, Dellagostin OA. Protection against lethal leptospirosis after vaccination with LipL32 coupled or coadministered with the B subunit of Escherichia coli heat-labile enterotoxin. Clin Vaccine Immunol 2012; 19:740-5; PMID:22379066; http://dx.doi.org/10.1128/CVI.05720-11
- Branger C, Chatrenet B, Gauvrit A, Aviat F, Aubert A, Bach JM, André-Fontaine G. Protection against Leptospira interrogans sensu lato challenge by DNA immunization with the gene encoding hemolysin-associated protein 1. Infect Immun 2005; 73:4062-9; PMID:15972494; http://dx.doi.org/10.1128/IAI.73.7.4062-4069.2005
- Umthong S, Buaklin A, Jacquet A, Sangjun N, Kerdkaew R, Patarakul K, Palaga T. Immunogenicity of a DNA and Recombinant Protein Vaccine Combining LipL32 and Loa22 for Leptospirosis Using Chitosan as a Delivery System. J Microbiol Biotechnol 2015; 25:526-36; PMID:25348693
- Matsunaga J, Young TA, Barnett JK, Barnett D, Bolin CA, Haake DA. Novel 45-kilodalton leptospiral protein that is processed to a 31-kilodalton growth-phase-regulated peripheral membrane protein. Infect Immun 2002; 70:323-34; PMID:11748198; http://dx.doi.org/10.1128/IAI.70.1.323-334.2002
- Muthumani K, Wise MC, Broderick KE, Hutnick N, Goodman J, Flingai S, Yan J, Bian CB, Mendoza J, Tingey C, Wilson C, et al. HIV-1 Env DNA Vaccine plus Protein Boost Delivered by EP Expands B- and T-Cell Responses and Neutralizing Phenotype In Vivo. PLoS One 2013; 8:e84234; PMID:24391921; http://dx.doi.org/10.1371/journal.pone.0084234
- Yang JS, Kim JJ, Hwang D, Choo AY, Dang K, Maguire H, Kudchodkar S, Ramanathan MP, Weiner DB. Induction of potent Th1-type immune responses from a novel DNA vaccine for West Nile virus New York isolate (WNV-NY1999). J Infect Dis 2001; 184:809-16; PMID:11550123; http://dx.doi.org/10.1086/323395
- Kowalczyk DW, Ertl HC. Immune responses to DNA vaccines. Cell Mol Life Sci 1999; 55:751-70; PMID:10379361; http://dx.doi.org/10.1007/s000180050330
- Hokey DA, Weiner DB. DNA vaccines for HIV: challenges and opportunities. Springer Semin Immunopathol 2006; 28:267-79.
- Hutnick NA, Myles DJ, Bian CB, Muthumani K, Weiner DB. Selected approaches for increasing HIV DNA vaccine immunogenicity in vivo. Curr Opin Virol 2011; 1:233-40; PMID:22440782; http://dx.doi.org/10.1016/j.coviro.2011.08.003
- Muthumani K, Wise MC, Broderick KE, Hutnick N, Goodman J, Flingai S, Yan J, Bian CB, Mendoza J, Tingey C, et al. HIV-1 Env DNA vaccine plus protein boost delivered by EP expands B and T-cell responses and neutralizing phenotype in vivo. PloS One 2013; 8:e84234; PMID:24391921; http://dx.doi.org/10.1371/journal.pone.0084234
- Mallilankaraman K, Shedlock DJ, Bao H, Kawalekar OU, Fagone P, Ramanathan AA, Ferraro B, Stabenow J, Vijayachari P, Sundaram SG, et al. A DNA vaccine against chikungunya virus is protective in mice and induces neutralizing antibodies in mice and nonhuman primates. PLoS Negl Trop Dis 2011; 5:e928; PMID:21264351; http://dx.doi.org/10.1371/journal.pntd.0000928
- Villarreal DO, Talbott KT, Choo DK, Shedlock DJ, Weiner DB. Synthetic DNA vaccine strategies against persistent viral infections. Expert Rev Vaccines 2013; 12:537-54; PMID:23659301; http://dx.doi.org/10.1586/erv.13.33
- Bagarazzi ML, Yan J, Morrow MP, Shen X, Parker RL, Lee JC, Giffear M, Pankhong P, Khan AS, Broderick KE, et al. Immunotherapy against HPV16/18 generates potent TH1 and cytotoxic cellular immune responses. Sci Transl Med 2012; 4:155ra38; PMID:23052295; http://dx.doi.org/10.1126/scitranslmed.3004414
- Shedlock DJ, Aviles J, Talbott KT, Wong G, Wu SJ, Villarreal DO, Myles DJ, Croyle MA, Yan J, Kobinger GP, et al. Induction of broad cytotoxic T cells by protective DNA vaccination against Marburg and Ebola. Mol Ther 2013; 21:1432-44; PMID:23670573; http://dx.doi.org/10.1038/mt.2013.61
- Kalams SA, Parker S, Jin X, Elizaga M, Metch B, Wang M, Hural J, Lubeck M, Eldridge J, Cardinali M, et al. Safety and immunogenicity of an HIV-1 gag DNA vaccine with or without IL-12 and/or IL-15 plasmid cytokine adjuvant in healthy, HIV-1 uninfected adults. PLoS One 2012; 7:e29231; PMID:22242162; http://dx.doi.org/10.1371/journal.pone.0029231
- Faisal SM, Yan W, Chen CS, Palaniappan RU, McDonough SP, Chang YF. Evaluation of protective immunity of Leptospira immunoglobulin like protein A (LigA) DNA vaccine against challenge in hamsters. Vaccine 2008; 26:277-87; PMID:18055070; http://dx.doi.org/10.1016/j.vaccine.2007.10.029
- Muthumani K, Lankaraman KM, Laddy DJ, Sundaram SG, Chung CW, Sako E, Wu L, Khan A, Sardesai N, Kim JJ, et al. Immunogenicity of novel consensus-based DNA vaccines against Chikungunya virus. Vaccine 2008; 26:5128-34; PMID:18471943; http://dx.doi.org/10.1016/j.vaccine.2008.03.060
- Babiuk LA, Babiuk SL, Loehr BI, van Drunnen Littel-van den H. Nucleic acid vaccines: research tool or commercial reality. Vet Immunol Immunopathol 2000; 76:1-23; PMID:10973683; http://dx.doi.org/10.1016/S0165-2427(00)00198-7
- Forde GM. Rapid response vaccines does DNA offer a solution? Nat Biotechnol 2005; 23:1059-62; PMID:16151391; http://dx.doi.org/10.1038/nbt0905-1059
- Wolff JA, Malone RW, Williams P, Chong W, Acsadi G, Jani A, et al. Direct gene transfer into mouse muscle in vivo. Science 1990; 247:1465-68; PMID:1690918; http://dx.doi.org/10.1126/science.1690918
- Boyer JD, Robinson TM, Kutzler MA, Vansant G, Hokey DA, Kumar S, Felgner PL. Protection against simian/human immunodeficiency virus (SHIV) 89.6P in macaques after coimmunization with SHIV antigen and IL-15 plasmid. Proc Natl Acad Sci U S A 2007; 104:18648-53; PMID:18000037; http://dx.doi.org/10.1073/pnas.0709198104
- Feng GH, Liu N, Zhou Y, Zhai YZ, Li XM, Dou XG. Immunologic analysis induced by DNA vaccine encoding E protein of Beijing-1 strain derived from Japanese encephalitis virus. Intervirology 2007; 50:93-8; PMID:17139185; http://dx.doi.org/10.1159/000097395
- Laddy DJ, Yan J, Khan AS, Andersen H, Cohn A. Electroporation of synthetic DNA antigens offers protection in nonhuman primates challenged with highly pathogenic avian influenza virus. J Virol 2009; 83:4624-30; PMID:19211745; http://dx.doi.org/10.1128/JVI.02335-08
- Kumar S, Yan J, Muthumani K, Ramanathan MP, Yoon H, Pavlakis GN, Felber BK, Sidhu M, Boyer JD, Weiner DB. Immunogenicity testing of a novel engineered HIV-1 envelope gp140 DNA vaccine construct. DNA Cell Biol 2006; 25:383-92; PMID:16848679; http://dx.doi.org/10.1089/dna.2006.25.383
- Scheller J, Chalaris A, Schmidt-Arras D, Rose-John S. The pro and anti-inflammatory properties of the cytokine interleukin-6. Biochim Biophys Acta 2011; 1813:878-88; PMID:21296109; http://dx.doi.org/10.1016/j.bbamcr.2011.01.034
- Matsunaga J, Barocchi MA, Croda J, Young TA, Sanchez Y, Siqueira I, Bolin CA, Reis MG, Riley LW, Haake DA, et al. Pathogenic Leptospira species express surface-exposed proteins belonging to the bacterial immunoglobulin superfamily. Mol Microbiol 2003; 49:929-45; PMID:12890019; http://dx.doi.org/10.1046/j.1365-2958.2003.03619.x
- Muthumani K, Flingai S, Wise M, Tingey C, Ugen KE, Weiner DB. Optimized and enhanced DNA plasmid vector based in vivo construction of a neutralizing anti-HIV-1 envelope glycoprotein Fab. Hum Vaccin Immunother 2013; 9:2253-62; PMID:24045230; http://dx.doi.org/10.4161/hv.26498
- Garmory HS, Brown KA, Titball RW. DNA vaccines: improving expression of antigens. Genet Vaccines Ther 2003; 1:2; PMID:14606963; http://dx.doi.org/10.1186/1479-0556-1-2
- Kozak M. At least six nucleotides preceding the AUG initiator codon enhance translation in mammalian cells. J Mol Biol 1987; 196:947-50; PMID:3681984; http://dx.doi.org/10.1016/0022-2836(87)90418-9
- Bodles-Brakhop AM, Draghia-Akli R. DNA vaccination and gene therapy: optimization and delivery for cancer therapy. Expert Rev Vaccines 2008; 7:1085-101; PMID:18767956; http://dx.doi.org/10.1586/14760584.7.7.1085
- Hirao LA, Wu L, Khan AS, Hokey DA, Yan J, Dai A, Betts MR, Draghia-Akli R, Weiner DB. Combined effects of IL-12 and electroporation enhances the potency of DNA vaccination in macaques. Vaccine 2008; 26:3112-20; PMID:18430495; http://dx.doi.org/10.1016/j.vaccine.2008.02.036
- Boyer JD, Robinson TM, Kutzler MA, Vansant G, Hokey DA, Kumar S, Parkinson R, Wu L, Sidhu MK, Pavlakis GN, et al. Protection against simian/human immunodeficiency virus (SHIV) 89.6P in macaques after coimmunization with SHIV antigen and IL-15 plasmid. Proc Nat Acad Sci U S A 2007; 104:18648-53; PMID:18000037; http://dx.doi.org/10.1073/pnas.0709198104
- Vedhagiri K, Velineni S, Timoney JF, Shanmughapriya S, Vijayachari P, Narayanan R, Natarajaseenivasan K. Detection of LipL32-specific IgM by ELISA in sera of patients with a clinical diagnosis of leptospirosis. Pathog Glob Health 2013; 107:130-5; PMID:23683367; http://dx.doi.org/10.1179/2047773213Y.0000000088
- Heuzenroeder MW, Barton MD, Vanniasinkam T, Phumoonna T. Linear B-cell epitope mapping using enzyme-linked immunosorbent assay for libraries of overlapping synthetic peptides. Methods Mol Biol 2009; 524:137-44; PMID:19377942; http://dx.doi.org/10.1007/978-1-59745-450-6_10