ABSTRACT
Rotavirus (RV) and norovirus (NoV) are the 2 leading causes of acute viral gastroenteritis worldwide. We have developed a non-live NoV and RV vaccine candidate consisting of NoV virus-like particles (VLPs) and recombinant polymeric RV VP6 protein produced in baculovirus-insect cell expression system. Both components have been shown to induce strong potentially protective immune responses. As VP6 nanotubes are highly immunogenic, we investigated here a possible adjuvant effect of these structures on NoV-specific immune responses in vivo. BALB/c mice were immunized intramuscularly with a suboptimal dose (0.3 μg) of GII.4 or GI.3 VLPs either alone or in a combination with 10 μg dose of VP6 and induction of NoV-specific antibodies in sera of experimental animals were measured. Blocking assay using human saliva or synthetic histo-blood group antigens was employed to test NoV blocking antibodies. Suboptimal doses of the VLPs alone did not induce substantial anti-NoV antibodies. When co-administered with the VP6, considerable titers of not only type-specific but also cross-reactive IgG antibodies against NoV VLP genotypes not included in the vaccine composition were induced. Most importantly, NoV-specific blocking antibodies, a surrogate for neutralizing antibodies, were generated. Our results show that RV VP6 protein has an in vivo adjuvant effect on NoV-specific antibody responses and support the use of VP6 protein as a part of the NoV-RV combination vaccine, especially when addition of external adjuvants is not desirable.
Introduction
Some viral capsid proteins are highly polymorphic with ability to self-assemble into different types of structures including sheets, nanotubes and icosahedra used in vaccine design as well as chimeric delivery platforms.Citation1-4 Rotaviruses (RV) are nonenveloped dsRNA viruses with triple-layered concentric capsid composed of VP2, VP6, VP7 and VP4 proteins.Citation5 The middle layer is composed of 780 molecules of VP6 protein approximately 45 kD in size, which are distributed as trimers. VP6 self-assembles into polymeric tubular or spherical structures when expressed in baculovirus (BV)Citation6 or bacterial recombinant expression systems.Citation7, 8 The assembly conformation depends on various conditions such as pH, ionic strength and divalent cation concentration.Citation9 The polymeric protein structures, in contrast to the soluble monomeric proteins, are highly immunogenic because of their particulate nature and size (˜20 nm to 2 μm) which readily activate antigen presenting cells (APC), as well as multivalent expression of antigenic epitopes to the immune cells.Citation10-13
RV VP6 protein is highly conserved, abundant and the most immunogenic protein in humans naturally infected with RV.Citation6,14-16 Antibodies directed against VP6 interfere with the replication cycle of RVCitation17-19 making it a promising next generation vaccine candidate against RV.Citation15,20-22 Licensed live oral RV vaccines are part of the routine childhood immunization in many countries. However, for various reasons including safety and low efficacy in developing countries, alternative non-live RV vaccines are being considered.Citation23-27 We have developed subunit protein based combination vaccine candidate consisting of norovirus (NoV) virus-like particles (VLPs) and RV VP6 nanotubes, against both NoV and RV gastroenteritis.Citation28,29 Most NoVs infecting humans belong to 2 heterologous and distant genogroups, namely GI and GII, that induce hardly any inter-genogroup immunity.Citation30 Although there are more than 30 genotypes within the GI and GII genogroups, the variants of GII.4 genotype have dominated since the mid 1990s,Citation31,32 being responsible for >70% of NoV infections worldwide. To induce broader NoV-specific immune responses, GII.4 and GI.3 VLPs as representatives of both genogroups have been included into our vaccine candidate.Citation29 We have previously published that NoV-RV combination vaccine induced strong type-specific and cross-reactive NoV- and RV-specific B and T cell immune responses and protected mice against murine RV challenge.Citation28,29,33 These earlier studies also suggested a possible adjuvant effect of RV VP6 on NoV-specific immune responses.Citation28 In addition, other investigators have reported that RV VP6 spheric formations used as an immunological carrier improved responses to the heterologous peptides or proteins delivered.Citation34-36 Due to the highly immunogenic properties of RV VP6, we investigated in the present study whether VP6 in the form of nanotubes has an adjuvant effect on the generation of NoV-specific immunity in mice. For the first time, using suboptimal doses of NoV GII.4 and GI.3 VLPs, our results show that VP6 acts as a strong adjuvant sparing the dose of NoV VLPs in a NoV-RV combination vaccine.
Results
Identity and purity of proteins used for immunization
Assembly and integrity of highly purified GII.4, GI.3 and VP6 proteins were confirmed by electron microscopy (EM) (). NoV VLPs 30 – 40 nm in size and VP6 tubes with the length of ∼0,2 to 1 μm were observed. Each product was >90% pure when analyzed by silver staining and densitometry (data not shown). Moreover, no impurities such as DNA (<10 ng dsDNA/10 μg of protein) or endotoxin (<0.1 endotoxin unit/10 μg of protein) were detected. Importantly, the protein preparations did not contain live BV (0 pfu/ml) or BV gp64 protein.
RV VP6 adjuvant effect on NoV type-specific serum antibodies
Groups of BALB/c mice were immunized according to the experimental design shown in and NoV type-specific IgG antibodies were analyzed from the termination sera (). Very high and uniform antibody responses were induced with 3 μg doses of GII.4 (geometric mean antibody titer, GMT 22,807) () or GI.3 VLPs (GMT 91,228) () alone. In contrast, suboptimal 0.3 μg doses of GII.4 () or GI.3 VLPs () alone induced very low levels of IgG antibodies. When the suboptimal doses of the VLPs were co-administered with RV VP6 protein (groups III and VII, ) the antibody responses were raised to the similar levels as detected with the 3 μg VLP doses and significantly higher compared to the responses obtained with 0.3 μg VLP dose () (all p < 0.002). Similar adjuvant effect on the NoV antibody responses was seen when 30 μg dose of VP6 (, group IV) was used instead of 10 μg (data not shown). Serum samples of the groups receiving GII.4 VLPs in a combination with VP6 were further analyzed for GII.4-specific IgG subtypes. The adjuvant effect was seen on both, GII.4-specific IgG1 antibodies (GMT 11,404) as well as IgG2a antibodies (GMT 6,400). All six control mice were completely negative for NoV-specific antibodies ().
Figure 2. NoV GII.4 (A, B) and GI.3 (C, D) genotype-specific serum IgG antibody responses induced with 0.3 μg or 3 μg of GII.4 or GI.3 VLPs alone or with 0.3 μg doses in a combination with 10 μg of RV VP6. Control (Ctrl) mice received carrier (PBS) only. OD490nm values of GII.4- (A) and GI.3-specific (C) antibodies in 1:100 diluted sera of individual mice are shown with the horizontal line representing the mean OD490nm value of the experimental group. GII.4- (B) and GI.3-specific (D) end-point titers of the groups of mice expressed as the geometric mean titers of the reciprocal of the highest sample dilution giving a positive reading. Error bars represent 95% confidence intervals, CIs. Groups were compared by Mann-Whitney U-test or Fisher's exact test and p values determined.
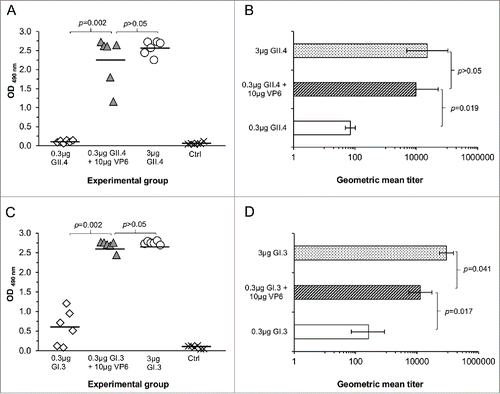
Table 1. Experimental and control groups of immunized mice. Mice were immunized intramuscularly (IM) at day 0 and 21 with the indicated dose and terminated at day 35.
RV VP6 adjuvant effect on NoV cross-reactive serum antibodies
Cross-reactive serum antibodies to NoV VLPs not used for immunization were also tested (). The sera of NoV GII.4 immunized mice were analyzed for the responses to the most novel GII.4 variants, GII.4 New Orleans (NO) () and GII.4 Sydney () and sera of GI.3 immunized mice to the prototype NoV GI.1 (). Considerable levels of cross-reactive NoV IgG antibodies were detected in groups of mice immunized with the combination of the NoV VLPs and VP6 but not with the suboptimal NoV VLP doses alone.
Figure 3. Cross-reactivity of serum IgG antibodies to NoV GII.4 NO (A), GII.4 Sydney (B) and GI.1 (C) in mice immunized with 0.3 μg of GII.4 or GI.3 VLPs alone or in a combination with 10 μg of RV VP6. Control (Ctrl) mice received carrier only (PBS). Mean ODs of the experimetal groups with standard errors are shown.
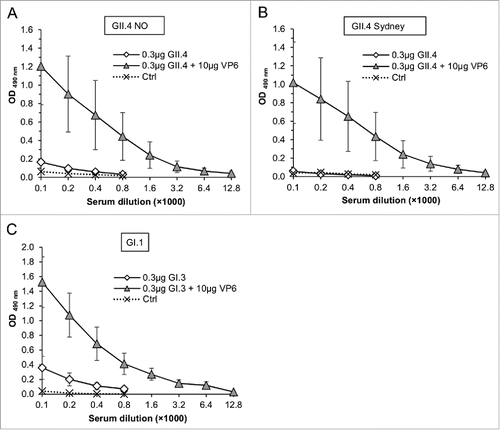
In order to determine success of immunization with recombinant VP6 protein, serum VP6-specific IgG antibodies were measured. Each mice receiving 10 μg dose of VP6 in a combination with NoV GII.4 or GI.3 VLPs developed considerable high antibody titer to VP6 (). The 30 μg dose of VP6 in a combination with GII.4 VLPs induced similar titer of antibodies (GMT 32,253) compared to 10 μg dose (GMT 36,203). None of the control mice developed VP6-specific antibodies ().
RV VP6 adjuvant effect on NoV-specific blocking antibodies
Serum antibodies of all immunized and control mice were tested for blocking of NoV VLP binding to the saliva type A (). To confirm the results, sera of all GII.4 VLP immunized mice were also tested for blocking synthetic H type 1 binding () and sera of GI.3 immunized mice for blocking Lewisa (Lea) histo-blood group antigen (HBGA) binding (). None of the controls or mice immunized with suboptimal 0.3 μg VLP dose developed any blocking antibodies (). In contrast, mice receiving 0.3 μg GII.4 or GI.3 VLPs combined with the 10 μg VP6 generated antibodies able to block binding of GII.4 VLPs () and GI.3 VLPs () to the saliva and synthetic HBGAs.
Figure 5. Blocking of NoV GII.4 VLP (A and B) and GI.3 VLP (C and D) binding to human type A saliva (A and C) and synthetic HBGAs (B and D) by the sera of mice immunized with 0.3 μg or 3 μg of GII.4 or GI.3 VLPs alone or with 0.3 μg doses in a combination with 10 μg of RV VP6. Synthetic HBGA H type 1 was employed for GII.4 (B) and Lea HBGA for GI.3 VLP (D) binding. Serum from mice receiving PBS was used as a negative control (Ctrl). Results are shown as the mean % blocking with standard errors of 2 – 3 independent experiments. A dashed line indicates 50% cut-off.Citation47
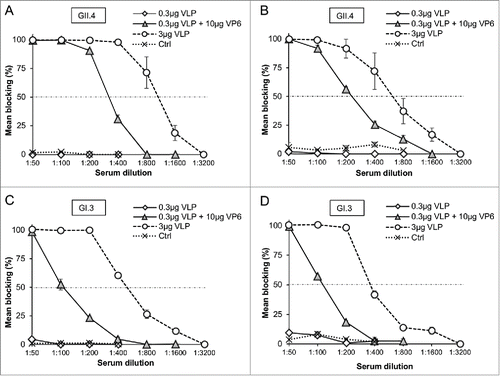
RV VP6 induces high levels of IL-4 and IFN-γ cytokine production
Splenocytes of the mice immunized with VP6 in combination with GII.4 () or GI.3 VLPs () produced considerable quantities of interleukin (IL) −4 when stimulated in vitro with RV Wa cell culture antigen or recombinant VP6 but not the mock cell culture MA104 nor the culture medium (CM). Mice receiving NoV VLPs alone or control mice did not produce any VP6-specific IL-4 (). Furthermore, RV-specific interferon (IFN) -γ production was tested from the cells of mice immunized with suboptimal dose of GII.4 VLPs in the presence or absence of VP6. Strong RV-specific IFN-γ responses were observed only in mice that received VP6 ().
Figure 6. RV VP6-specific IL-4 and IFN-γ production by T-cells. RV Wa cell culture antigen and recombinant VP6 protein were used to stimulate IL-4 ((A)and B) and IFN-γ (C) production from the splenocytes of mice immunized with 0.3 μg of GII.4 or GI.3 VLPs alone or in a combination with 10 μg of RV VP6. Control (Ctrl) mice received carrier only (PBS). Results are expressed as the mean spot forming cells (SFC)/106 cells of the duplicate wells with standard errors. The experiments were repeated 2 or more times with similar results. A dashed line indicates the cut-off limit >50 SFC/106 splenocytes.
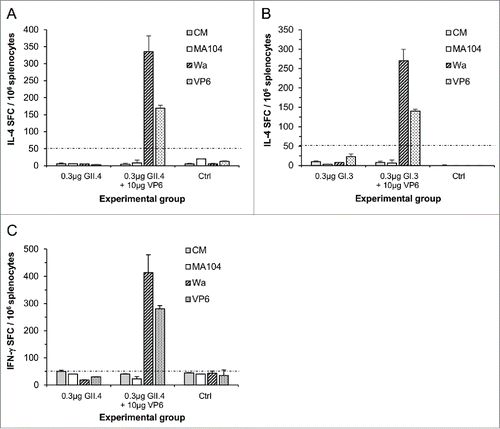
Discussion
RV VP6 protein forms polymeric structures including nanotubes and nanospheres, when produced in BV or E. coli recombinant expression systems.Citation7-9,38 We and othersCitation15,16,28,38 have recently shown that these structures are extremely potent inducers of B and T cell immune responses in animal models. Moreover, among different nanostructures VP6 tubes were shown to be the most immunogenic.Citation7 Our group has been working on the development of a combination vaccine candidate consisting of NoV VLPs and RV VP6 against acute childhood gastroenteritis.Citation28,29 The vaccine candidate induced strong NoV- and RV-specific B and T cell immune responses and protected mice against RV challenge validating the combination vaccine concept.Citation28,29,33 The earlier studies additionally suggested an adjuvant effect of RV VP6 on NoV-specific cross-reactive antibody responses.Citation28 In here, we hypothesized that in contrast to the current NoV VLP vaccine candidates in clinical trials, which contain external adjuvants,Citation39-41 in our vaccine candidate external adjuvants might be substituted by extremely immunogenic VP6 protein. The experimental design using suboptimal doses of highly purified NoV VLPs enabled us to evidently address the adjuvant effect of VP6.
We first determined high purity of VP6 as well as NoV VLPs used for immunization of animals, to exclude an adjuvant effect of impurities related to the BV expression system such as DNA, live BV, gp64 and endotoxin.Citation42,43 Additionally, EM images showed that protein assemblies similar to those of sucrose gradient purified products previously described by our groupCitation29 were obtained, and that polymeric nature of these proteins was not affected by the rigorous purification process.
It was recently shown that NoV uses HBGAs, complex carbohydrates present on red blood cells and epithelial cell surface and as free antigens in body secretions, as cellular attachment factors or receptors.Citation44-46 NoV-specific antibodies, which block binding of NoV VLPs to the HBGAs, are considered surrogate for neutralizing antibodies and the only correlate of protection from NoV infection identified so far.Citation44,47-49 Therefore, in the present study we primarily investigated in vivo adjuvant effect of RV VP6 on NoV-specific antibody responses. Due to the lack of cross-reactive immune responses between NoV GI and GII, it is thought that NoV vaccine should contain at least one representative of each genogroup.Citation29,50,51 In addition to the type-specific antibodies, induction of cross-reactive antibodies within the genogroup is considered important. When highly purified VP6 was administered to mice in a combination with suboptimal doses (0.3 μg respectively) of NoV GII.4 or GI.3 VLPs, it exerted an adjuvant effect by inducing significant NoV type-specific and cross-reactive antibody responses, as well as blocking antibodies.
As the adjuvant effect of RV VP6 was observed on both NoV VLP-specific IgG1 and IgG2a antibody responses, we next investigated if RV VP6 immunization induced IL-4 and IFN-γ cytokine production, hallmarks of CD4+ Th2 and Th1 cells immunity.Citation37,52,53 The ELISPOT assay showed that when the cells of immunized mice were stimulated in vitro with the RV Wa cell culture antigen or theVP6 protein they produced remarkable amount of both cytokines. Strong induction of VP6-specific CD4+ Th2 cells is of significance, as soluble cytokine IL-4 produced by these cells may help antibody generation by promoting proliferation and differentiation of NoV antigen primed B lymphocytes into antibody secreting plasma cells and memory B cells. The concept of intermolecular help for RV neutralizing antibody generation by VP6-specific Th2 cells has been suggested by Esquivel et al. in a mouse RV challenge study.Citation54 However, to prove the above hypothesis, in vivo cell depletion or CD4+ T cell transfer experiments should be performed.
Future studies to identify the molecular mechanisms of VP6 adjuvant action are required. When bluetongue virus (BTV) nanotubes of nonstructural protein NS1 or RV VP6 nanospheres were used as an antigen carrier in chimeric delivery platforms, the humoral response to the foreign antigen was improved,Citation34,35,55 suggesting an adjuvant role for the 2 proteins. The tubular structures of the BTV, which are similar in size and morphology to the VP6 tubes, appear favorable for APC uptake via macropinocytosis and endocytosis.Citation4 Recent findings by Rodriguez et al.Citation56 showed that RV VP6 nanotubes were also internalized by macrophages. The uptake process might activate and mature professional APCs, which play a central role in activating adaptive immune responses.Citation11,57 Our preliminary findings (unpublished observation) indicate that RV VP6 is internalized by macrophages and immature dendritic cells, and induces activation and maturation of these APCs by upregulating expression of cell surface antigen presenting and costimulatory molecules and production of pro-inflammatory cytokines (IL-6 and TNF-α). Pro-inflammatory cytokines are important for APC migration and recruitment to the injection site,Citation58 thereby RV VP6 may create a milieu for adequate antigen presentation of co-delivered antigens at the site.
The results in this study show that RV VP6 protein, in addition to being a subunit RV vaccine candidate, has in vivo adjuvant properties providing dose-sparing advantages for induction of antibody responses against NoV, when co-administered with NoV VLPs. These findings support the use of VP6 protein as a part of the NoV-RV combination vaccine, especially when the use of external adjuvants is not desirable, such as in the vaccines for pediatric population.
Materials and Methods
Recombinant proteins production and purification
NoV GI.1 (Reference strain accession no. AY502016), GI.3 (AF414403), GII.4–1999 (AF080551), called GII.4, GII.4–2009 New Orleans (NO) (GU445325), and GII.4–2012 Sydney (AFV08795.1) VLPs as well as human RV VP6 protein (GQ477131) used in analytical methods were produced in a BV-insect cell expression system (Invitrogen, Cat. 10359–016) and purified on sucrose gradients as described in details elsewhere.Citation28,29,38,59
Highly purified GII.4 VLPs, GI.3 VLPs and VP6 proteins used for immunization of animals were generated using multistep chromatography purification process (UMN Pharma Inc., Japan; unpublished data and Patent application no. 2013–146240). The purity, identity and morphology of the protein preparations were confirmed as previously described.Citation28,60 In brief, the total protein, dsDNA concentration and purity were determined with the Pierce BCA protein assay (Thermo Scientific, Cat. 23227), Quant-it dsDNA Broad-Range Assay Kit (Invitrogen, Q33120) and Sodium Dodecyl Sulfate Polyacrylamide Gel Electrophoresis (SDS-PAGE) followed by densitometric analysis after silver staining (PageSilver™ silver staining kit, Fermentas, Cat. K0681). EM after negative staining with 3% uranyl acetate pH 4.6 was used to identify protein morphology. Infectious BV and endotoxin level were determined with BacPAK™ RapidTiter Kit (Clontech laboratories, Cat. 631406) and Limulus Amebocyte Lysate assay (Lonza, Cat. N184–25). To verify the absence of BV proteins, the products were further analyzed with SDS-PAGE followed by immunoblotting with anti-BV gp64 antibody (Santa Cruz Biotechnology, Cat. sc-65499).
Immunization of mice
shows experimental and control immunization groups of mice. Two doses (0.3 and 3 μg) of NoV GII.4 and GI.3 VLPs in sterile phosphate-buffered saline (PBS) were administered to female 7-week-old BALB/c mice (Harlan Laboratories, Horst, Netherlands) intramuscularly. The 0.3 μg dose was administered either alone or in a mixture with RV VP6 protein. The 10 μg dose of the VP6 protein was previously identified to be optimal for induction of RV VP6-specific immune responses in mice.Citation20,29 Mice were immunized twice, at day 0 and day 21, and terminated at day 35. Control mice received carrier (sterile PBS) only. Animals were anesthetized prior to immunization and euthanasia with a formulation of medetomidine (Dorbene® vet, Laboratorios SYVA S.A., Cat. 067632) and ketamine (Ketaminol® vet, Intervet International B.V., Cat. 511485). Blood samples and spleens were collected at the time of termination as previously described.Citation61 All the procedures were approved by the Finnish Animal Experiment Board.
NoV- and RV VP6-specific serum IgG ELISA
Induction of anti-NoV and anti-VP6 antibody responses was determined measuring NoV type-specific (GII.4 and GI.3, respectively) and cross-reactive (GII.4 NO, GII.4 Sydney and GI.1) as well as RV VP6-specific IgG antibody levels in serum samples of experimental and control mice. Serially diluted (two-fold dilution series starting at 1:100) sera of each animal were analyzed by enzyme-linked immunosorbent assay (ELISA) according to the previously published procedures.Citation28,29,38,62 Briefly, Costar High Binding half area plates (Corning Inc., Cat. 3690) were coated with 0.4 to 1.0 μg/ml of GI.1, GI.3, GII.4, GII.4 NO, GII.4 Sydney VLPs or VP6 protein in PBS. The bound antibodies were detected with horseradish peroxidase (HRP)-conjugated goat anti-mouse IgG (Sigma-Aldrich, Cat. A4416), IgG1 (Invitrogen, Cat. A10551) or IgG2a (Invitrogen, Cat. A10685), followed by a reaction with the SIGMAFAST o-phenylenediamine dihydrochloride (OPD) substrate (Sigma-Aldrich, Cat. P9187). Optical density at 490 nm (OD490) was measured with VictorCitation2 1420 microplate reader (Wallac, Perkin Elmer, Waltham, MA). The wells of control mouse sera were used to determine the cut-off value (mean OD490 + 3 standard deviations). Specimens with a net OD490 above the set cut-off value and >0.1 were considered positive. The end-point antibody titers of sera were defined as the highest sample dilution with an OD490 above the set cut-off value.
Blocking assay of NoV VLP binding to HBGA
Blocking assay measuring NoV-specific serum antibodies which block binding of NoV VLPs to the HBGAs is considered a surrogate neutralization assay.Citation44,47 The method has been described in details elsewhere.Citation28,61-63 In brief, serial two-fold dilutions starting at 1:50 were prepared from serum samples of experimental and control mice. For the blocking assay using synthetic HBGAs, sera were pre-incubated with 0.4 μg/ml of NoV GII.4 or GI.3 VLPs prior to plating on biotin-conjugated synthetic HBGA (all from GlycoTech Corporation)-coated NeutrAvidin plates (Pierce, Cat. 15508). Synthetic H type 1 (Led (H type 1)-PAA-Biotin, Cat. 01–037) HBGAs were used for binding of GII.4 VLPs, whereas GI.3 VLPs were tested on Lewisa (Lea-PAA-Biotin, Cat. 01–035) HBGAs. For the saliva blocking assay, Costar half-area plates were coated with secretor-positive human type A saliva prior to adding the mixtures of serum with 0.1 μg/ml of GI.3 or GII.4 VLPs. The HBGAs used in the present study were chosen based on the binding profiles of these VLPs published by our group.Citation63 Maximum binding of VLPs to the HBGAs was determined in wells with VLPs in the absence of serum sample. Bound VLPs were detected with human NoV antiserum followed by anti-human IgG-HRP antibody (Invitrogen, Cat. 62–7120) and OPD substrate. The blocking % was calculated as follows: 100% - [(OD490 of wells with VLP and serum/OD490 of wells with VLP without serum) ×100%].
RV VP6-specific ELISPOT IL-4 and IFN-γ assay
T cell responses specific for RV were analyzed using previously published enzyme-linked immunospot (ELISPOT) IFN-γ assay.Citation29,38 Multiscreen 96-well HTS-IP filter plates (Millipore, Cat. MSIPN4W50) were coated either with 5 μg/ml of anti-mouse IL-4 monoclonal antibody 11B11 (Mabtech AB, Cat. 3311–3) or monoclonal anti-mouse IFN-γ antibody AN18 (Mabtech AB, Cat 3321–3). Splenocytes (0.4 × 106 cells/well for IL-4, 0.1 × 106 cells/well for IFN-γ) from experimental and control mice were stimulated in duplicate wells with human RV Wa cell culture antigen (containing VP6 protein at 0.5 μg/ml) or with VP6 protein (5 μg/ml). MA104 mock cell culture was used as a negative control. Splenocytes incubated in culture media (CM) alone served as a background control and splenocytes stimulated with 10 μg/ml of concanavalin A (Sigma-Aldrich, Cat. C5275) as a cell viability control. Following incubation of 45 h for IL-4 or 20 h for IFN-γ at 37°C, cytokine secretion was detected with 0.5 μg/ml of biotin-conjugated anti-mouse IL-4 monoclonal antibody BVD6–24G2 (Mabtech AB, Cat. 3311–6) or biotinylated anti-mouse IFN-γ monoclonal antibody (Mabtech AB, Cat. 3321–6) and 1:1000 diluted streptavidin-alkaline phosphatase (Mabtech AB, Cat. 3310–10). After development with BCIP/NBT substrate (Mabtech AB, Cat. 3650–10) the spots were counted with ImmunoSpot® automatic CTL analyzer (CTL-Europe GmbH, Bonn, Germany). The results were expressed as mean spot forming cells (SFC)/106 splenocytes of duplicate wells.
Statistics
A nonparametric Mann-Whitney U-test and Fisher's exact test were employed to assess the statistical differences between non-parametric observations of 2 independent groups. Data was analyzed with IBM SPSS Statistics -software (SPSS Inc., Chicago, IL) version 22.0 and a statistically significant difference was defined as p ≤ 0.05.
Abbreviations
RV | = | rotavirus |
NoV | = | norovirus |
VLPs | = | virus-like particles |
BV | = | baculovirus |
APC | = | antigen presenting cells |
GI | = | genogroup I |
GII | = | genogroup II |
EM | = | electron microscopy |
GMT | = | geometric mean titer |
GII.4 NO | = | GII.4 New Orleans |
Le | = | Lewis |
HBGA | = | histo-blood group antigen |
IL | = | interleukin |
IFN-γ | = | interferon-gamma |
CM | = | culture medium |
BTV | = | bluetongue virus |
TNF-α | = | tumor necrosis factor-alpha |
SDS-PAGE | = | sodium dodecyl sulfate polyacrylamide gel electrophoresis |
PBS | = | phosphate-buffered saline |
ELISA | = | enzyme-linked immunosorbent assay |
HRP | = | horseradish peroxidase |
OPD | = | o-phenylenediamine dihydrochloride |
OD | = | optical density |
ELISPOT | = | enzyme-linked immunospot |
Disclosure of potential conflicts of interest
The authors report no conflicts of interest.
Acknowledgments
Technical support of K. Tamminen, H. Uusi-Kerttula, M. Karlsberg, E. Jokela, S. Kaven and N. Koivisto is greatly acknowledged. We gratefully acknowledge the technical assistance of personnel at UMN Pharma Inc., Japan.
References
- Roldao A, Mellado MC, Castilho LR, Carrondo MJ, Alves PM. Virus-like particles in vaccine development. Expert Rev Vaccines 2010; 9:1149-76; PMID:20923267; http://dx.doi.org/10.1586/erv.10.115
- Pushko P, Pumpens P, Grens E. Development of virus-like particle technology from small highly symmetric to large complex virus-like particle structures. Intervirology 2013; 56:141-65; PMID:23594863; http://dx.doi.org/10.1159/000346773
- Lavelle L, Gingery M, Phillips M, Gelbart WM, Knobler CM, Cadena-Nava RD, Vega-Acosta JR, Pinedo-Torres LA, Ruiz-Garcia J. Phase diagram of self-assembled viral capsid protein polymorphs. J Phys Chem B 2009; 113:3813-9PMID:19673134; http://dx.doi.org/10.1021/jp8079765
- Ghosh MK, Deriaud E, Saron MF, Lo-Man R, Henry T, Jiao X, Roy P, Leclerc C. Induction of protective antiviral cytotoxic T cells by a tubular structure capable of carrying large foreign sequences. Vaccine 2002; 20:1369-77; PMID:11818155; http://dx.doi.org/10.1016/S0264-410X(01)00467-4
- Prasad BV, Wang GJ, Clerx JP, Chiu W. Three-dimensional structure of rotavirus. J Mol Biol 1988; 199:269-75; http://dx.doi.org/10.1016/0022-2836(88)90313-0
- Estes MK, Crawford SE, Penaranda ME, Petrie BL, Burns JW, Chan WK, Ericson B, Smith GE, Summers MD. Synthesis and immunogenicity of the rotavirus major capsid antigen using a baculovirus expression system. J Virol 1987; 61:1488-94; PMID:3033276
- Bugli F, Caprettini V, Cacaci M, Martini C, Paroni Sterbini F, Torelli R, Della Longa S, Papi M, Palmieri V, Giardina B, et al. Synthesis and characterization of different immunogenic viral nanoconstructs from rotavirus VP6 inner capsid protein. Int J Nanomedicine 2014; 9:2727-39; PMID:24936129
- Li T, Lin H, Zhang Y, Li M, Wang D, Che Y, Zhu Y, Li S, Zhang J, Ge S, et al. Improved characteristics and protective efficacy in an animal model of E. coli-derived recombinant double-layered rotavirus virus-like particles. Vaccine 2014; 32:1921-31; PMID:24530406; http://dx.doi.org/10.1016/j.vaccine.2014.01.093
- Lepault J, Petitpas I, Erk I, Navaza J, Bigot D, Dona M, Vachette P, Cohen J, Rey FA. Structural polymorphism of the major capsid protein of rotavirus. EMBO J 2001; 20:1498-507; PMID:11285214; http://dx.doi.org/10.1093/emboj/20.7.1498
- Manolova V, Flace A, Bauer M, Schwarz K, Saudan P, Bachmann MF. Nanoparticles target distinct dendritic cell populations according to their size. Eur J Immunol 2008; 38:1404-13; PMID:18389478; http://dx.doi.org/10.1002/eji.200737984
- Xiang SD, Scholzen A, Minigo G, David C, Apostolopoulos V, Mottram PL, Plebanski M. Pathogen recognition and development of particulate vaccines: Does size matter? Methods 2006; 40:1-9; http://dx.doi.org/10.1016/j.ymeth.2006.05.016
- Hubbell JA, Thomas SN, Swartz MA. Materials engineering for immunomodulation. Nature 2009; 462:449-60; PMID:19940915; http://dx.doi.org/10.1038/nature08604
- Cubas R, Zhang S, Kwon S, Sevick-Muraca EM, Li M, Chen C, Yao Q. Virus-like particle (VLP) lymphatic trafficking and immune response generation after immunization by different routes. J Immunother 2009; 32:118-28; PMID:19238010; http://dx.doi.org/10.1097/CJI.0b013e31818f13c4
- Svensson L, Sheshberadaran H, Vene S, Norrby E, Grandien M, Wadell G. Serum antibody responses to individual viral polypeptides in human rotavirus infections. J Gen Virol 1987; 68 (Pt 3):643-51; PMID:3029295; http://dx.doi.org/10.1099/0022-1317-68-3-643
- Ward RL, McNeal MM. VP6: A candidate rotavirus vaccine. J Infect Dis 2010; 202 Suppl:S101-7; PMID:20684688; http://dx.doi.org/10.1086/653556
- Ishida S, Feng N, Tang B, Gilbert JM, Greenberg HB. Quantification of systemic and local immune responses to individual rotavirus proteins during rotavirus infection in mice. J Clin Microbiol 1996; 34:1694-700; PMID:8784572
- Corthesy B, Benureau Y, Perrier C, Fourgeux C, Parez N, Greenberg H, Schwartz-Cornil I. Rotavirus anti-VP6 secretory immunoglobulin A contributes to protection via intracellular neutralization but not via immune exclusion. J Virol 2006; 80:10692-9; http://dx.doi.org/10.1128/JVI.00927-06
- Garaicoechea L, Olichon A, Marcoppido G, Wigdorovitz A, Mozgovoj M, Saif L, Surrey T, Parreno V. Llama-derived single-chain antibody fragments directed to rotavirus VP6 protein possess broad neutralizing activity in vitro and confer protection against diarrhea in mice. J Virol 2008; 82:9753-64; http://dx.doi.org/10.1128/JVI.00436-08
- Aiyegbo MS, Sapparapu G, Spiller BW, Eli IM, Williams DR, Kim R, Lee DE, Liu T, Li S, Woods VL, Jr, et al. Human rotavirus VP6-specific antibodies mediate intracellular neutralization by binding to a quaternary structure in the transcriptional pore. PLoS One 2013; 8:e61101; http://dx.doi.org/10.1371/journal.pone.0061101
- Lappalainen S, Pastor AR, Tamminen K, Lopez-Guerrero V, Esquivel-Guadarrama F, Palomares LA, Vesikari T, Blazevic V. Immune responses elicited against rotavirus middle layer protein VP6 inhibit viral replication in vitro and in vivo. Hum Vaccin Immunother 2014; 10:2039-47; PMID:25424814; http://dx.doi.org/10.4161/hv.28858
- Burns JW, Siadat-Pajouh M, Krishnaney AA, Greenberg HB. Protective effect of rotavirus VP6-specific IgA monoclonal antibodies that lack neutralizing activity. Science 1996; 272:104-7; PMID:8600516; http://dx.doi.org/10.1126/science.272.5258.104
- Feng N, Lawton JA, Gilbert J, Kuklin N, Vo P, Prasad BV, Greenberg HB. Inhibition of rotavirus replication by a non-neutralizing, rotavirus VP6-specific IgA mAb. J Clin Invest 2002; 109:1203-13; PMID:11994409; http://dx.doi.org/10.1172/JCI14397
- Patel NC, Hertel PM, Estes MK, de la Morena M, Petru AM, Noroski LM, Revell PA, Hanson IC, Paul ME, Rosenblatt HM, et al. Vaccine-acquired rotavirus in infants with severe combined immunodeficiency. N Engl J Med 2010; 362:314-9; PMID:20107217; http://dx.doi.org/10.1056/NEJMoa0904485
- Weintraub ES, Baggs J, Duffy J, Vellozzi C, Belongia EA, Irving S, Klein NP, Glanz JM, Jacobsen SJ, Naleway A, et al. Risk of intussusception after monovalent rotavirus vaccination. N Engl J Med 2014; 370:513-9; http://dx.doi.org/10.1056/NEJMoa1311738
- Hemming M, Vesikari T. Vaccine-derived human-bovine double reassortant rotavirus in infants with acute gastroenteritis. Pediatr Infect Dis J 2012; 31:992-4; http://dx.doi.org/10.1097/INF.0b013e31825d611e
- Vesikari T, Matson DO, Dennehy P, Van Damme P, Santosham M, Rodriguez Z, Dallas MJ, Heyse JF, Goveia MG, Black SB, et al. Safety and efficacy of a pentavalent human-bovine (WC3) reassortant rotavirus vaccine. N Engl J Med 2006; 354:23-33; PMID:16394299; http://dx.doi.org/10.1056/NEJMoa052664
- Murphy TV, Gargiullo PM, Massoudi MS, Nelson DB, Jumaan AO, Okoro CA, Zanardi LR, Setia S, Fair E, LeBaron CW, et al. Intussusception among infants given an oral rotavirus vaccine. N Engl J Med 2001; 344:564-72; PMID:11207352; http://dx.doi.org/10.1056/NEJM200102223440804
- Blazevic V, Lappalainen S, Nurminen K, Huhti L, Vesikari T. Norovirus VLPs and rotavirus VP6 protein as combined vaccine for childhood gastroenteritis. Vaccine 2011; 29:8126-33; PMID:21854823; http://dx.doi.org/10.1016/j.vaccine.2011.08.026
- Tamminen K, Lappalainen S, Huhti L, Vesikari T, Blazevic V. Trivalent combination vaccine induces broad heterologous immune responses to norovirus and rotavirus in mice. PLoS One 2013; 8:e70409; http://dx.doi.org/10.1371/journal.pone.0070409
- Glass RI, Parashar UD, Estes MK. Norovirus gastroenteritis. N Engl J Med 2009; 361:1776-85; PMID:19864676; http://dx.doi.org/10.1056/NEJMra0804575
- Kroneman A, Vega E, Vennema H, Vinje J, White PA, Hansman G, Green K, Martella V, Katayama K, Koopmans M. Proposal for a unified norovirus nomenclature and genotyping Arch Virol 2013; 158:2059-68; PMID:23615870; http://dx.doi.org/10.1007/s00705-013-1708-5
- Vega E, Barclay L, Gregoricus N, Shirley SH, Lee D, Vinje J. Genotypic and epidemiologic trends of norovirus outbreaks in the united states, 2009 to 2013. J Clin Microbiol 2014; 52:147-55; http://dx.doi.org/10.1128/JCM.02680-13
- Lappalainen S, Pastor AR, Malm M, Lopez-Guerrero V, Esquivel-Guadarrama F, Palomares LA, Vesikari T, Blazevic V. Protection against live rotavirus challenge in mice induced by parenteral and mucosal delivery of VP6 subunit rotavirus vaccine. Arch Virol 2015; 160:2075-8; PMID:26016444.
- Redmond MJ, Ohmann HB, Hughes HP, Sabara M, Frenchick PJ, Poku SK, Ijaz MK, Parker MD, Laarveld B, Babiuk LA. Rotavirus particles function as immunological carriers for the delivery of peptides from infectious agents and endogenous proteins. Mol Immunol 1991; 28:269-78; PMID:1850113; http://dx.doi.org/10.1016/0161-5890(91)90073-S
- Frenchick PJ, Sabara MI, Ready KF, Babiuk LA. Biochemical and immunological characterization of a novel peptide carrier system using rotavirus VP6 particles. Vaccine 1992; 10:783-91; http://dx.doi.org/10.1016/0264-410X(92)90514-K
- Peralta A, Molinari P, Taboga O. Chimeric recombinant rotavirus-like particles as a vehicle for the display of heterologous epitopes. Virol J 2009; 6:192,422X-6-192; PMID:19891790; http://dx.doi.org/10.1186/1743-422X-6-192
- Chen Q, He F, Kwang J, Chan JK, Chen J. GM-CSF and IL-4 stimulate antibody responses in humanized mice by promoting T, B, and dendritic cell maturation. J Immunol 2012; 189:5223-9; PMID:23089398; http://dx.doi.org/10.4049/jimmunol.1201789
- Lappalainen S, Tamminen K, Vesikari T, Blazevic V. Comparative immunogenicity in mice of rotavirus VP6 tubular structures and virus-like particles. Hum Vaccin Immunother 2013; 9:1991-2001; PMID:23777748; http://dx.doi.org/10.4161/hv.25249
- Treanor JJ, Atmar RL, Frey SE, Gormley R, Chen WH, Ferreira J, Goodwin R, Borkowski A, Clemens R, Mendelman PM. A novel intramuscular bivalent norovirus virus-like particle vaccine candidate-reactogenicity, safety, and immunogenicity in a phase 1 trial in healthy adults. J Infect Dis 2014; 210:1763-71.
- Bernstein DI, Atmar RL, Lyon GM, Treanor JJ, Chen WH, Jiang X, Vinje J, Gregoricus N, Frenck RW, Jr, Moe CL, et al. Norovirus vaccine against experimental human GII.4 virus illness: A challenge study in healthy adults. J Infect Dis 2014; 211:870-8; PMID:25210140
- Atmar RL, Bernstein DI, Harro CD, Al-Ibrahim MS, Chen WH, Ferreira J, Estes MK, Graham DY, Opekun AR, Richardson C, et al. Norovirus vaccine against experimental human norwalk virus illness. N Engl J Med 2011; 365:2178-87; PMID:22150036; http://dx.doi.org/10.1056/NEJMoa1101245
- Abe T, Takahashi H, Hamazaki H, Miyano-Kurosaki N, Matsuura Y, Takaku H. Baculovirus induces an innate immune response and confers protection from lethal influenza virus infection in mice. J Immunol 2003; 171:1133-9; PMID:12874198; http://dx.doi.org/10.4049/jimmunol.171.3.1133
- Hervas-Stubbs S, Rueda P, Lopez L, Leclerc C. Insect baculoviruses strongly potentiate adaptive immune responses by inducing type I IFN. J Immunol 2007; 178:2361-9; PMID:17277142; http://dx.doi.org/10.4049/jimmunol.178.4.2361
- Harrington PR, Lindesmith L, Yount B, Moe CL, Baric RS. Binding of norwalk virus-like particles to ABH histo-blood group antigens is blocked by antisera from infected human volunteers or experimentally vaccinated mice. J Virol 2002; 76:12335-43; PMID:12414974; http://dx.doi.org/10.1128/JVI.76.23.12335-12343.2002
- Marionneau S, Cailleau-Thomas A, Rocher J, Le Moullac-Vaidye B, Ruvoen N, Clement M, Le Pendu J. ABH and lewis histo-blood group antigens, a model for the meaning of oligosaccharide diversity in the face of a changing world. Biochimie 2001; 83:565-73; http://dx.doi.org/10.1016/S0300-9084(01)01321-9
- Hutson AM, Atmar RL, Graham DY, Estes MK. Norwalk virus infection and disease is associated with ABO histo-blood group type. J Infect Dis 2002; 185:1335-7; http://dx.doi.org/10.1086/339883
- Reeck A, Kavanagh O, Estes MK, Opekun AR, Gilger MA, Graham DY, Atmar RL. Serological correlate of protection against norovirus-induced gastroenteritis. J Infect Dis 2010; 202:1212-8; PMID:20815703; http://dx.doi.org/10.1086/656364
- Nurminen K, Blazevic V, Huhti L, Rasanen S, Koho T, Hytonen VP, Vesikari T. Prevalence of norovirus GII-4 antibodies in finnish children. J Med Virol 2011; 83:525-31; PMID:21264875; http://dx.doi.org/10.1002/jmv.21990
- Malm M, Uusi-Kerttula H, Vesikari T, Blazevic V. High serum levels of norovirus genotype-specific blocking antibodies correlate with protection from infection in children. J Infect Dis 2014; 210:1755-62; PMID:24970849; http://dx.doi.org/10.1093/infdis/jiu361
- Debbink K, Lindesmith LC, Baric RS. The state of norovirus vaccines. Clin Infect Dis 2014; 58:1746-52; PMID:24585561; http://dx.doi.org/10.1093/cid/ciu120
- Atmar RL, Estes MK. Norovirus vaccine development: Next steps. Expert Rev Vaccines 2012; 11:1023-5; http://dx.doi.org/10.1586/erv.12.78
- Choi P, Reiser H. IL-4: Role in disease and regulation of production. Clin Exp Immunol 1998; 113:317-9; PMID:9737656; http://dx.doi.org/10.1046/j.1365-2249.1998.00690.x
- Geginat J, Paroni M, Maglie S, Alfen JS, Kastirr I, Gruarin P, De Simone M, Pagani M, Abrignani S. Plasticity of human CD4 T cell subsets. Front Immunol 2014; 5:630; PMID:25566245; http://dx.doi.org/10.3389/fimmu.2014.00630
- Esquivel FR, Lopez S, Guitierrez-X L, Arias C. The internal rotavirus protein VP6 primes for an enhanced neutralizing antibody response. Arch Virol 2000; 145:813-25; PMID:10893159; http://dx.doi.org/10.1007/s007050050674
- Ghosh MK, Borca MV, Roy P. Virus-derived tubular structure displaying foreign sequences on the surface elicit CD4+ th cell and protective humoral responses. Virology 2002; 302:383-92; http://dx.doi.org/10.1006/viro.2002.1648
- Rodriguez M, Wood C, Sanchez-Lopez R, Castro-Acosta RM, Ramirez OT, Palomares LA. Understanding internalization of rotavirus VP6 nanotubes by cells: Towards a recombinant vaccine. Arch Virol 2014; 159:1005-15; PMID:24232915; http://dx.doi.org/10.1007/s00705-013-1916-z
- Fifis T, Gamvrellis A, Crimeen-Irwin B, Pietersz GA, Li J, Mottram PL, McKenzie IF, Plebanski M. Size-dependent immunogenicity: Therapeutic and protective properties of nano-vaccines against tumors. J Immunol 2004; 173:3148-54; PMID:15322175; http://dx.doi.org/10.4049/jimmunol.173.5.3148
- Hoebe K, Janssen E, Beutler B. The interface between innate and adaptive immunity. Nat Immunol 2004; 5:971-4; PMID:15454919; http://dx.doi.org/10.1038/ni1004-971
- Huhti L, Blazevic V, Nurminen K, Koho T, Hytonen VP, Vesikari T. A comparison of methods for purification and concentration of norovirus GII-4 capsid virus-like particles. Arch Virol 2010; 155:1855-8; PMID:20721592; http://dx.doi.org/10.1007/s00705-010-0768-z
- Huhti L, Tamminen K, Vesikari T, Blazevic V. Characterization and immunogenicity of norovirus capsid-derived virus-like particles purified by anion exchange chromatography. Arch Virol 2013; 158:933-42; PMID:23229011; http://dx.doi.org/10.1007/s00705-012-1565-7
- Tamminen K, Huhti L, Koho T, Lappalainen S, Hytonen VP, Vesikari T, Blazevic V. A comparison of immunogenicity of norovirus GII-4 virus-like particles and P-particles. Immunology 2012; 135:89-99; PMID:22044070; http://dx.doi.org/10.1111/j.1365-2567.2011.03516.x
- Malm M, Tamminen K, Lappalainen S, Uusi-Kerttula H, Vesikari T, Blazevic V. Genotype considerations for virus-like particle-based bivalent norovirus vaccine composition. Clin Vaccine Immunol 2015; 22:656-63; PMID:25903355; http://dx.doi.org/10.1128/CVI.00015-15
- Uusi-Kerttula H, Tamminen K, Malm M, Vesikari T, Blazevic V. Comparison of human saliva and synthetic histo-blood group antigens usage as ligands in norovirus-like particle binding and blocking assays. Microbes Infect 2014; 16:472-80; PMID:24631874; http://dx.doi.org/10.1016/j.micinf.2014.02.010