abstract
Millions of seasonal and pandemic influenza vaccine doses containing oil-in-water emulsion adjuvant have been administered in order to enhance and broaden immune responses and to facilitate antigen sparing. Despite the enactment of a Global Action Plan for Influenza Vaccines and a multi-fold increase in production capabilities over the past 10 years, worldwide capacity for pandemic influenza vaccine production is still limited. In developing countries, where routine influenza vaccination is not fully established, additional measures are needed to ensure adequate supply of pandemic influenza vaccines without dependence on the shipment of aid from other, potentially impacted first-world countries. Adaptation of influenza vaccine and adjuvant technologies by developing country influenza vaccine manufacturers may enable antigen sparing and corresponding increases in global influenza vaccine coverage capacity. Following on previously described work involving the technology transfer of oil-in-water emulsion adjuvant manufacturing to a Romanian vaccine manufacturing institute, we herein describe the preclinical evaluation of inactivated split virion H5N1 influenza vaccine with emulsion adjuvant, including immunogenicity, protection from virus challenge, antigen sparing capacity, and safety. In parallel with the evaluation of the bioactivity of the tech-transferred adjuvant, we also describe the impact of concurrent antigen manufacturing optimization activities. Depending on the vaccine antigen source and manufacturing process, inclusion of adjuvant was shown to enhance and broaden functional antibody titers in mouse and rabbit models, promote protection from homologous virus challenge in ferrets, and facilitate antigen sparing. Besides scientific findings, the operational lessons learned are delineated in order to facilitate adaptation of adjuvant technologies by other developing country institutes to enhance global pandemic influenza preparedness.
Introduction
Increasing global pandemic influenza vaccine manufacturing capacity is a key aim of the World Health Organization (WHO) and the Biomedical Advanced Research and Development Authority (BARDA) of the US Department of Human and Health Services (HHS). According to the WHO,Citation1 influenza vaccine manufacturing capacity has been enhanced from 2.6 B doses in 2009 to 4.5 B doses in 2014, representing a significant improvement. Moreover, 22 countries are now producing approved influenza vaccines compared to 19 countries in 2009. However, in order to immunize 70% of the world's population, with 2 doses of vaccines, ∼10.2 billion dose manufacturing capacity is needed. Therefore, antigen sparing strategies such as adjuvants represent a key technology for global pandemic influenza preparedness.
We previously reported the successful technology transfer of oil-in-water emulsion adjuvant manufacturing and quality control from the Infectious Disease Research Institute (IDRI) in Seattle, USA, to the Cantacuzino National Research Institute (designated CI hereafter) in Bucharest, Romania, including physicochemical compatibility testing with split virion inactivated H5N1 vaccine.Citation2 We now report the second phase of this program, the preclinical evaluation of influenza A/H5N1 vaccine with an oil-in-water emulsion adjuvant in mouse, rabbit, and ferret models to determine effects of the adjuvant on immunogenicity (humoral and cellular) and protective efficacy, with a focus on optimizing the antigen sparing capacity. We note here that to the best of our knowledge and based on ongoing freedom to operate analyses, the adjuvant and antigen technologies employed here are unencumbered from intellectual property infringements.
The practical challenges associated with the project are highlighted to facilitate the success of related technology transfer efforts in the future. In particular, technology transfer to developing country manufacturers, as opposed to developed country manufacturers, is more likely to be hampered by resource and facility constraints, underdeveloped regulatory agencies, and obsolete manufacturing practices. For instance, unforeseen antigen manufacturing developments related to seasonal influenza vaccine production at CI directly influenced the pandemic influenza vaccine program, necessitating modification of pandemic influenza antigen manufacturing processes at the same time that adjuvant technology was being implemented at CI. Thus, the present manuscript describes concurrent activities involving the 1) evaluation of the bioactivity of the tech-transferred adjuvant as well as 2) assessment of the bioactivity resulting from different vaccine antigen manufacturing processes. Despite this added complexity, the adjuvant manufactured by CI demonstrated enhanced functional immune responses and antigen sparing capacity in various animal models.
Results
Characterization results for adjuvant and antigen batches employed in the studies described here are listed in , respectively. Testing results passed all quality control specifications established previously. For all studies described below, antigen and adjuvant were stored separately at 2–8°C prior to use and mixed immediately before immunization.
Table 1. Adjuvant characterization results.
Table 2. Antigen characterization results.
The first mouse experiment was an exploratory approach to evaluate the effects of different influenza H5N1 (A/Vietnam/1194/04 [Clade 1]) antigen manufacturing processes () on immunogenicity, compared to an H1N1 antigen as a positive control, and to determine the changes in immune response magnitude, quality, and duration attributable to the adjuvant, employing a uniform dose of antigen (3 μg) for all groups. Groups of 15 female BALB/c mice were intramuscularly immunized twice, 3 weeks apart, with antigen alone or antigen mixed with adjuvant. The H1N1 vaccine serving as a positive control, induced 100% seroconversion and 100% seroprotection 3 weeks after one immunization (). The groups receiving H5N1 vaccine showed high seroconversion and seroprotection rates one week after the second immunization (73.3% and 66% for H5N1 Process Y [detergent split/β-propiolactone inactivation]; 100% and 73.3% for H5N1 Process X [ether split/formaldehyde inactivation]) and 3 weeks after the second immunization (100% and 83% for H5N1 Process Y; 100% and 100% for Process X). In general, immunogenicity of H5N1-X was similar to that of H5N1-Y except for the Day 28 time point, where H5N1-X elicited higher HI titers. The variation in HI response (but not the magnitude) among mice of the same group appears to decrease over time, suggesting that a limit of response magnitude was attained. Furthermore, significant enhancement in antigen-specific HI titers due to the inclusion of adjuvant was not evident even at 6 weeks post-boost, nor was HI titer to heterologous strains increased by adjuvant (A/turkey/Turkey/1/2005 [Clade 2.2] and A/Cambodia/R0405050/2007 [Clade 1], data not shown). Likewise, the adjuvant did not generally enhance or broaden microneutralization titers (except for the adjuvanted H1N1 group at 6 weeks post-boost, Fig. S1), cytokine-producing cells (IFNγ or IL-5) or long-lived plasma cells (data not shown). The body weight of immunized mice was monitored throughout the study but showed similar weight gain profile for control and immunized mice (data not shown).
Figure 1. Flow diagram of antigen manufacturing processes employed by CI with corresponding preclinical studies which employed the antigens, with changes highlighted in orange shading. The process used to manufacture the Sanofi Pasteur H5N1 vaccine, used in Mouse Exp 3 and Ferret Exp 1, was similar to Process Z according to Treanor et al. New Eng J Med 2006, 354:1343 as well as the package insert.
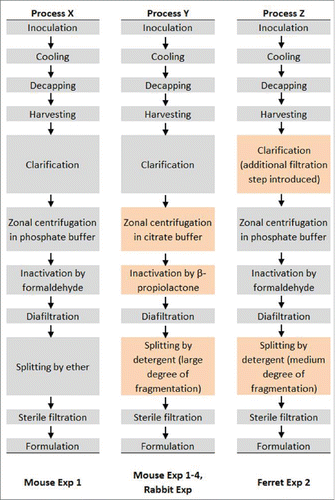
Figure 2. H5N1 antigens manufactured by different processes elicit comparable immunogenicity in mice, but effect of adjuvant is not apparent at antigen dose of 3 μg. H5N1 and H1N1 antigens were manufactured by Process X (H5N1-X, H1N1-X) or Process Y (H5N1-Y). Groups of 15 female BALB/c mice were immunized i.m. on Day 0 and Day 21 with PBS, antigen alone (3 μg), or antigen (3 μg) + SE. HI titers for 8–15 mice were measured on Day 20 (a), Day 28 (b), Day 42 (c), and Day 62 (d). Multiple comparisons between groups were analyzed by one-way ANOVA with Tukey's correction, #p<0.05 H5N1-X vs. H5N1-Y. The data are shown as box and whisker plots, with whiskers representing the minimum and maximum values.
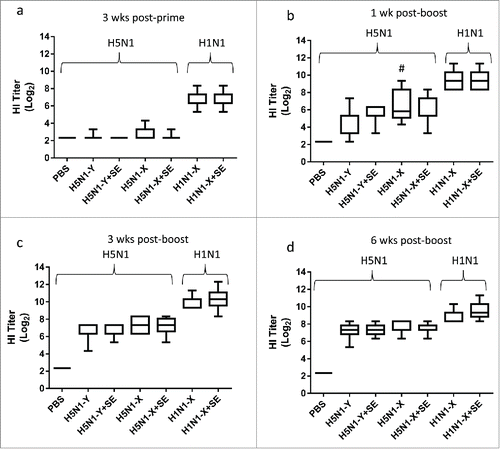
In order to determine whether the minimal adjuvant effect in the first mouse experiment was due to the high antigen dose of 3 μg, a second mouse experiment was designed to titrate the dose of antigen manufactured by Process Y over 2 orders of magnitude from 10 μg to 0.1 μg to achieve an antigen dose in the mouse where the effect of adjuvant could be more readily determined, as well as to evaluate antigen sparing potential. Group size, mouse strain, and immunization regimen remained identical to the first mouse experiment described above. In this case, the adjuvant significantly enhanced homologous HI and MN titers at all dose levels, even at the 3 μg antigen dose (). Moreover, HI and MN titers obtained at the 0.1 μg antigen dose with adjuvant were significantly higher than or equivalent to those elicited by 10 μg of antigen alone, demonstrating a significant antigen sparing effect. Seroconversion and seroprotection rates against the homologous strain (A/Vietnam/1194/04) measured 3 weeks after the boost immunization were >91% for all adjuvanted groups. Furthermore, adjuvanted vaccine broadened HI and/or MN titers against the heterologous A/turkey/Turkey/1/2005 and A/Cambodia/R0405050/2007 strains (). Assay sensitivity and the adjuvant-enhancing effect on HI responses were even more clearly apparent when horse RBCs were employed in the HI assay instead of turkey RBCs such that all adjuvanted groups elicited significantly higher HI titers than the 10 μg dose of antigen alone ( and Supplementary Information); thus, horse RBCs were employed for all subsequent HI assays described below. Consistent with the HI and MN titer data, significantly increased long-lived plasma cell counts and antigen-specific IgG, IgG1, and IgG2a titers due to adjuvant inclusion were evident (). Consistent with reports regarding MF59®,Citation3, 4 the SE adjuvant increased both IgG1 and IgG2a titers but did not appear to dramatically skew the quality (i.e. Th1/Th2 balance) of the immune response compared to the antigen alone, although there is a trend toward more of Th2 response as evidenced by . Likewise, changes in the ratio of antigen-stimulated IL-5 secreting cells to IFNγ secreting cells as determined by ELISPOT assay showed a trend toward increased response in the IL-5/IFNγ ratio due to adjuvant, but these assays involved only 4 mice per group and the differences were not statistically significant ().
Figure 3. SE facilitates enhanced HI, MN, IgG, and long-lived plasma cell responses and antigen dose sparing. H5N1 antigen was manufactured by Process Y (H5N1-Y). Groups of 15 female BALBc mice were immunized i.m. on Day 0 and Day 21 with PBS, antigen alone, SE alone, or antigen + SE at the antigen doses indicated. Except as noted, serum HI titers were measured by CI using turkey RBCs from 11--15 mice per group on Day 28 against the homologous strain (a), on Day 28 against the heterologous A/turkey/Turkey/1/2005 strain (b), on Day 42 against the homologous strain (c), on Day 42 against the homologous strain using horse RBCs at the Southern Research Institute (SRI) (d), and on Day 42 against the heterologous A/turkey/Turkey/1/2005 strain (e). MN titers were measured from 8--15 mice per group (except for the negative controls) on Day 28 against the homologous (f) and heterologous (g) strains or on Day 42 against the homologous (h) and heterologous (i) strains. Long-lived antibody-secreting plasma cells determined by ELISPOT (j), antibody titers determined by ELISA and interpolated from a standard curve for IgG (k), IgG1 (l), and IgG2a (m), including the calculated IgG2/aIgG1 ratio (n), and the ratio of IL-5-secreting cells:IFNγ-secreting cells determined by ELISPOT (o) were all measured on Day 42. ELISPOT assays employed 4 mice per group whereas antibody ELISA assays were for 9--13 mice with samples below detection assigned a value of zero. Multiple comparisons between groups were analyzed by one-way ANOVA with Tukey's correction, *p < 0.05 vs. H5N1-Y (10 μg), #p < 0.05 vs. same antigen dose without adjuvant. The data in (a-m, o) are shown as box and whisker plots, with whiskers representing the minimum and maximum values. The columns in (n) represent grouped average ratios.
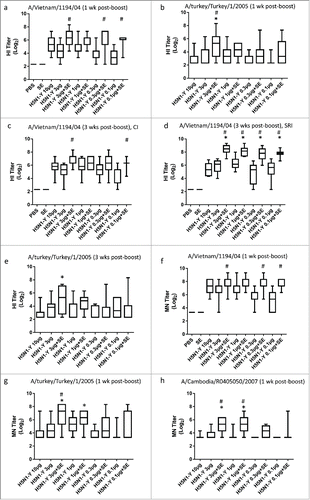
Based on the results from the second mouse experiment and in order to benchmark the antigen and adjuvant materials to established controls, a third mouse experiment was designed to compare the immunogenicity profile of antigen produced by CI (Process Y) to that induced by the SP antigen both in combination with SE adjuvant (produced by CI or IDRI), or EM022, an oil-in-water emulsion adjuvant designed to have similar composition as that reported for MF59®.Citation5 EM022 contains 2.5% v/v squalene emulsified by non-ionic surfactants whereas SE contains 2% v/v squalene emulsified by phospholipid and block copolymer.Citation2, 6 In this third mouse experiment, the mouse strain and immunization regimen remained identical to the previous 2 mouse experiments described above, although group size was reduced to 10 animals. Immunogenicity analyses were focused only on HI titers against homologous and heterologous strains (). Groups receiving antigen alone showed no significant difference in HI titers between CI-prepared antigen and SP-prepared antigen. Moreover, adjuvant inclusion significantly boosted homologous and heterologous HI titers after 2 immunizations, regardless of the adjuvant manufacturer (IDRI or CI) or adjuvant composition (SE or EM022). Seroprotection and seroconversion rates against the homologous strain (A/Vietnam/1194/04) measured one or 3 weeks after the boost immunization were 100% for all adjuvanted groups, and ≥80% against both heterologous strains (A/turkey/Turkey/1/2005 or A/Cambodia/R0405050/2007) at the same time points.
Figure 4. Adjuvanted H5N1 vaccines from different manufacturers elicit enhanced HI titers from immunized mice regardless of antigen or adjuvant manufacturer or composition. H5N1 antigen was manufactured by CI using Process Y (H5N1-Y) or by Sanofi Pasteur (H5N1-S) using a process similar to Process Z. Groups of 10 female BALB/c mice were immunized i.m. on Day 0 and Day 21 with PBS, antigen alone (1 or 0.1 μg), or antigen + emulsion adjuvant (SE manufactured by CI, SE manufactured by IDRI, or EM022 manufactured by IDRI). HI titers were measured by CI from all 10 mice from each group on Day 28 (a-c) or Day 42 (d-f) using horse RBCs. HI titers against the homologous strain (a,d) or heterologous strains (A/turkey/Turkey/1/2005[b,e] or A/Cambodia/R0405050/2007[c,f]) were measured. Multiple comparisons between groups were analyzed by one-way ANOVA with Tukey's correction, *p < 0.05 vs. H5N1-Y (1 μg and 0.1 μg) or H5N1-S (1 μg and 0.1 μg). The data are shown as box and whisker plots, with whiskers representing the minimum and maximum values.
![Figure 4. Adjuvanted H5N1 vaccines from different manufacturers elicit enhanced HI titers from immunized mice regardless of antigen or adjuvant manufacturer or composition. H5N1 antigen was manufactured by CI using Process Y (H5N1-Y) or by Sanofi Pasteur (H5N1-S) using a process similar to Process Z. Groups of 10 female BALB/c mice were immunized i.m. on Day 0 and Day 21 with PBS, antigen alone (1 or 0.1 μg), or antigen + emulsion adjuvant (SE manufactured by CI, SE manufactured by IDRI, or EM022 manufactured by IDRI). HI titers were measured by CI from all 10 mice from each group on Day 28 (a-c) or Day 42 (d-f) using horse RBCs. HI titers against the homologous strain (a,d) or heterologous strains (A/turkey/Turkey/1/2005[b,e] or A/Cambodia/R0405050/2007[c,f]) were measured. Multiple comparisons between groups were analyzed by one-way ANOVA with Tukey's correction, *p < 0.05 vs. H5N1-Y (1 μg and 0.1 μg) or H5N1-S (1 μg and 0.1 μg). The data are shown as box and whisker plots, with whiskers representing the minimum and maximum values.](/cms/asset/ec2eb7bf-6f0b-4a5f-a60b-dbb2659f30b3/khvi_a_1111495_f0004_b.gif)
Following completion of the third mouse study, an immunogenicity experiment (5 animals/group) was designed to evaluate different antigen doses (ranging from 3.75 to 15 μg) in another animal model: New Zealand white rabbits. In addition, another mouse experiment was conducted in parallel employing the same antigen and adjuvant materials (antigen doses ranging from 0.75 to 3 μg). In the rabbits, a dose of 7.5 μg antigen with adjuvant induced significantly higher HI titers 3 and 6 weeks after the boost immunization compared to the 15 μg dose of antigen alone (). In the parallel mouse experiment, all adjuvanted groups elicited significantly higher HI titers than 3 μg antigen alone at one, 3, or 6 weeks after the boost immunization (Fig. S2).
Figure 5. H5N1 vaccine with SE adjuvant induces enhanced HI titers and antigen dose sparing in immunized rabbits. H5N1 antigen was manufactured by CI using Process Y (H5N1-Y). Groups of 5 male New Zealand white rabbits were immunized i.m. on Day 0 and Day 21 with PBS, antigen alone (15 μg), or antigen (15, 7.5, or 3.75 μg) + emulsion adjuvant. HI titers against the homologous strain were measured using horse RBCs from all 5 rabbits from each group on Day 21 (a), Day 28 (b), Day 42 (c), or Day 63 (d). Multiple comparisons between groups were analyzed by one-way ANOVA with Tukey's correction, *p < 0.05 vs. H5N1-Y (15 μg). The data are shown as box and whisker plots, with whiskers representing the minimum and maximum values.
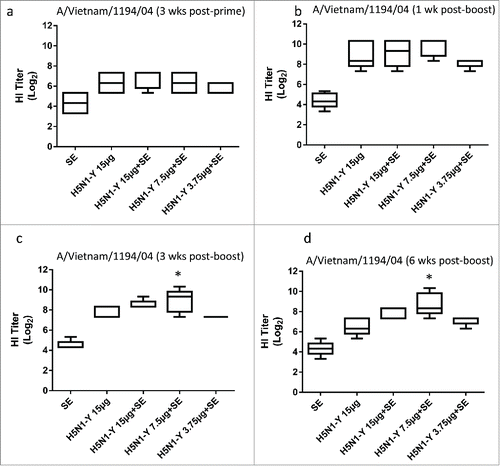
The preclinical results described above positioned the development program to advance into ferret studies to establish the antigen sparing potential of the adjuvant in a pandemic influenza challenge model. In contrast to the mouse model, H5 influenza viruses infect the ferret without the requirement for prior host adaptation, and manifest clinical signs of disease akin to human influenza.Citation7 There are 2 main lines of evidence indicating that the respiratory tract of ferrets closely resembles that of humans; first, ferrets possess a predominance of α-(2–6)-linked sialic acids on the upper airway epithelia and second, avian H5N1 and human H3N2 influenza viruses exhibit similar patterns of virus attachment to tissues from both species.Citation8-12 The primary goal of the ferret studies described below was to evaluate the antigen sparing capacity of the adjuvant; in this regard and consistent with clinical study designs addressing the same question,Citation13 the most efficient use of animals called for an experimental design with a high dose antigen alone group compared to lower dose antigen groups with or without adjuvant.
However, it was during this period that further antigen manufacturing process changes were determined to be necessary at CI due to potential stability concerns with some seasonal influenza vaccine strains related to the manufacturing procedure in Process Y. Therefore, while antigen manufacturing Process Z was under development at CI, an initial ferret study was designed to establish the ability of the SE adjuvant (produced by CI) combined with the SP antigen to facilitate antigen sparing in terms of protection from challenge as well as functional antibody titers. Groups of 7 male ferrets each received 2 intramuscular immunizations, 3 weeks apart, of SP antigen alone (3.75 or 15 μg) or in combination with SE adjuvant (3.75 μg antigen). Fourteen days following the second immunization, the ferrets were challenged intranasally with A/Vietnam/1203/04 H5N1 and followed for clinical signs of morbidity and nasal viral titers. Following death or euthanasia, tissues were analyzed for virus titers and histopathology. Serology was measured in samples collected 3 weeks after each immunization.
All ferrets that were administered only saline (negative control) required euthanasia by day 9 post-challenge (). Ferrets receiving antigen alone (15 or 3.75 μg) demonstrated 71% and 57% survival, respectively, 14 d post-challenge. Notably, all ferrets receiving 3.75 μg of antigen with adjuvant survived challenge. After comparing all group pairs using the Log-rank (Mantel-Cox) test and correcting for multiple comparisons by the Bonferroni method, only the adjuvanted group showed significantly enhanced survival compared to the negative control group. The average clinical scores of the challenged ferrets were consistent with the survival data, with the adjuvanted group showing the lowest average scores (). Likewise, reduction in average body weight loss and reduction in temperature increase was most apparent for the adjuvanted group (). Nasal viral titers were significantly reduced by all groups containing antigen compared to the saline control when measured at Day 1 and Day 5 post-challenge, but not Day 3 (). Both HI and MN titers were negative for all animals when measured 3 weeks after the prime or boost immunizations. However, antigen-specific IgG titers measured 3 weeks after the boost immunization were significantly elevated in the adjuvanted group compared to all groups without adjuvant (). No significant differences in histopathology scores were apparent between groups (Fig. S3).
Figure 6. Ferrets immunized with adjuvanted H5N1 manufactured by Sanofi (H5N1-S) demonstrate enhanced protection from homologous challenge. (a) Percent survival following challenge. (b) Average clinical score for each group of ferrets following challenge: 0 = normal; 1 = mild lethargy; 2 = reduced activity; 3 = not playful or active, lethargic; 4 = severe illness, moribund (euthanized); 5 = found dead. No ferrets were found dead in this experiment, but all ferrets with score of 4 were euthanized and scored as 5 on the days following euthanization. (c) Average loss of body weight when compared to Day 0 post-challenge. Dead animals were assigned an arbitrary weight loss of 25%. (d) Average ferret body temperature when compared to the day before challenge. Dead animals were assigned an arbitrary temperature increase of 5%. (e) Virus titers in nasal flushes collected 1, 3, and 5 d post-challenge (animals with undetectable virus titers were given an arbitrary value of 10 (i.e., 1 log)). (f) Antigen-specific IgG titers for immunized ferrets measured 3 weeks after the boost immunization (immediately prior to challenge). For the survival data, all group pairs were compared using the Log-rank (Mantel-Cox) test and corrected for multiple comparisons by the Bonferroni method. For viral titer and IgG data, multiple comparisons between groups were analyzed by one-way ANOVA with Tukey's correction, ‡p<0.05 vs. PBS, #p < 0.05 vs. H5N1-S (3.75 μg), *p < 0.05 vs. H5N1-S (15 μg). The data in (e-f) are shown as box and whisker plots, with whiskers representing the minimum and maximum values.
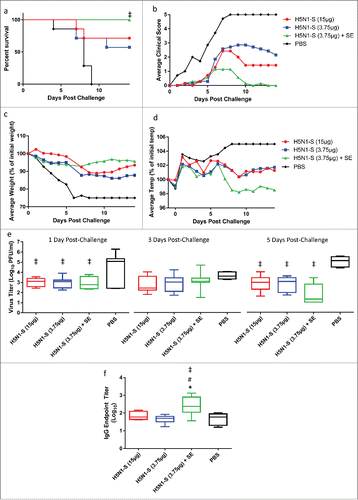
Since the adjuvant facilitated protection from challenge and antigen sparing in ferrets immunized with the SP antigen, a second ferret challenge experiment was conducted in order to evaluate the performance of the adjuvant in combination with the H5N1 vaccine (A/Vietnam/1194/04) manufactured by CI using Process Z (H5N1-Z). Experimental design was similar to the first ferret experiment described above, with the exception that a greater antigen dose range was employed in order to determine if detectable HI and MN titers could be generated. Groups of 6 male ferrets each received 2 intramuscular immunizations, 3 weeks apart, of H5N1-Z antigen alone (45 or 15 μg) or in combination with SE adjuvant (15, 3.75, or 1 μg antigen). Fourteen days following the second immunization, the ferrets were challenged intranasally with A/Vietnam/1203/04 H5N1 and followed for clinical signs of morbidity and nasal viral titers. Following death or euthanasia, tissues were analyzed for virus titers and histopathology. Serology was measured in samples collected 3 weeks after each immunization.
Five of 6 ferrets that were administered only saline (negative control) required euthanasia by Day 8 post-challenge, with one ferret surviving to the end of the study (Day 14 post-challenge, ). Ferrets receiving antigen alone (45 or 15 μg) demonstrated 83% and 100% survival, respectively, 14 d post-challenge. Likewise, all ferrets receiving 15 μg with adjuvant survived challenge. However, ferrets receiving 3.75 or 1 μg antigen with adjuvant demonstrated 33% and 50% survival, respectively. After comparing all group pairs using the Log-rank (Mantel-Cox) test and correcting for multiple comparisons by the Bonferroni method, no significant differences between groups existed. The average clinical scores, reduction in average body weight, and reduction in temperature increase of the challenged ferrets were consistent with the survival data (). Nasal viral titers were significantly reduced 5 d post-challenge by the 15 μg antigen groups (with or without adjuvant) and the 3.75 μg antigen group (with adjuvant), but not the 45 μg antigen group (without adjuvant) or the 1 μg antigen group (with adjuvant, ). As with the first ferret experiment, both HI and MN titers were negative for all animals when measured 3 weeks after the prime or boost immunizations, although antigen-specific IgG titers measured 3 weeks after the boost immunization were significantly elevated in all groups compared to the saline control (). The lack of detectable serological responses is not attributable to assay error, since positive controls responded properly and sera were sent to a second laboratory (CI), where the results were confirmed. No significant differences in histopathology scores were apparent between groups (Fig. S4).
Figure 7. Ferrets immunized with adjuvanted H5N1 manufactured by CI (H5N1-Z) demonstrate similar clinical response compared to animals immunized without adjuvant. (a) Percent survival following challenge. (b) Average clinical score for each group of ferrets following challenge: 0 = normal; 1 = mild lethargy; 2 = reduced activity; 3 = not playful or active, lethargic; 4 = severe illness, moribund (euthanized); 5 = found dead. Ferrets with score of 4 were euthanized and scored as 5 on the days following euthanization. (c) Average loss of body weight when compared to Day 0 post-challenge. Dead animals were assigned an arbitrary weight loss of 25%. (d) Average ferret body temperature when compared to the day before challenge. Dead animals were assigned an arbitrary temperature increase of 5%. (e) Virus titers in nasal flushes collected 1, 3, and 5 d post-challenge (animals with undetectable virus titers were given an arbitrary value of 10 (i.e. 1 log)). (f) Antigen-specific IgG titers for immunized ferrets measured 3 weeks after the boost immunization (immediately prior to challenge). For the survival data, all group pairs were compared using the Log-rank (Mantel-Cox) test and corrected for multiple comparisons by the Bonferroni method. For viral titer and IgG data, multiple comparisons between groups were analyzed by one-way ANOVA with Tukey's correction, ‡p < 0.05 vs. PBS. The data in (e-f) are shown as box and whisker plots, with whiskers representing the minimum and maximum values.
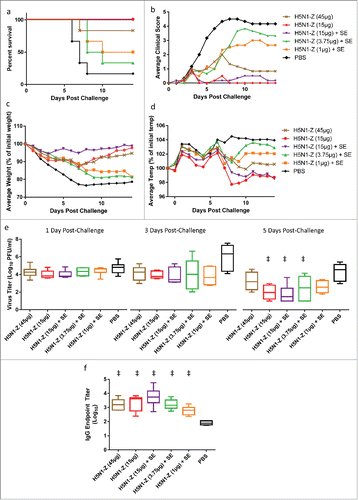
In summary, the enhancement in protective immune response to the SP antigen (H5N1-S in ) vaccine attributable to the adjuvant was clearly evident in the first ferret experiment, demonstrating at least a 4-fold antigen sparing effect. However, in the second ferret experiment to evaluate the H5N1-Z vaccine, no enhancement in protective immune response attributable to the adjuvant was apparent at the antigen doses evaluated. This finding did not appear to be related to an antigen-adjuvant compatibility issue, since no loss in vaccine potency, as determined by SRID, was apparent one month after mixing with antigen (data not shown). In order to determine whether the H5N1-Z vaccine contained unique immunostimulatory activity attributable to viral pattern recognition receptor ligands or bacterial contamination, the human monocytic cell line THP-1 was stimulated with different vaccine preparations manufactured by CI. Upregulation of the proinflammatory cytokine TNF-α was evident in cells stimulated by H5N1-Z but not antigens manufactured by Process Y or Process X (Fig. S5). Moreover, the TNF-α upregulation was not affected by anti-TLR4 antibody, but was inhibited by anti-TLR2 antibody, suggesting potential microbial contamination involving TLR2 ligand.
Discussion
The work described in the present manuscript directly follows from our previous publication focusing on the adjuvant manufacturing aspects of technology transfer wherein CI successfully demonstrated the ability to independently manufacture SE adjuvant that passed predetermined physicochemical specifications.Citation2 The next phase of the project was to evaluate the preclinical bioactivity of pandemic influenza vaccine containing the adjuvant, including its capacity to facilitate antigen sparing. The primary difficulty of this phase of the project was that the timeline coincided with both seasonal and pandemic influenza vaccine manufacturing optimization efforts at CI (conducted under the guidance of WHO and other tech transfer partners). Thus, it was not feasible to have a finalized vaccine antigen preparation from the start of the preclinical evaluation studies described here. Unfortunately this complicated the design and interpretation of the ensuing preclinical studies. Nevertheless, in order to present a real-world case study of the actual challenges encountered in a complex technology transfer project to a developing country, we have presented both the progress and setbacks to provide insight into the practical difficulties associated with such an effort, thus providing a distinct approach compared to more typical reporting of preclinical adjuvanted influenza vaccine studies. This is important since there are very few vaccine technology transfer efforts reported in the literature, even though such efforts are considered a critical necessity to enhance global health.Citation14-16
Overall, the preclinical studies demonstrated the antigen sparing capacity of the adjuvant manufactured by CI (tech transfer recipient) and its comparability to adjuvant manufactured by IDRI (tech transfer partner) and thus validated that the technology transfer of adjuvant manufacturing succeeded in bioactivity as well as physicochemical expectations. However, this success was tempered by variability in vaccine bioactivity apparently attributable to different antigen preparations and/or HI assay reagents which prevented a linear preclinical development process. We discuss these findings in detail below.
It is evident from the preclinical findings reported here that the importance of contrasting dosing across species is key to elucidating the potential antigen sparing effects of adjuvants when added to influenza vaccines. For instance, 3 μg antigen employed in the first mouse experiment did not indicate significant adjuvant enhancement (), whereas the lower antigen doses employed in the second and third mouse experiments showed a clear enhancement and antigen sparing effect in functional responses, attributable to the adjuvant (). Nevertheless, even at a 3 μg antigen dose, adjuvant elicited significantly higher responses in later mouse experiments ( and Fig. S2), in contrast to the first mouse experiment, indicating some other factor could be responsible for the discrepancy. At least in the case of the HI assay, a potential contributing factor is the source of RBCs employed in the assay. HI assays conducted using horse-derived RBCs appeared to result in a more sensitive assay compared to turkey-derived RBCs, in agreement with previously published work based on differences between species in sialic acid receptor specificity,Citation17 and thus more clearly show the influence of adjuvant inclusion on enhancing homologous and especially heterologous HI responses (see and Supplementary Information Table S1 and Fig. S6). As for antigen dosing in rabbits and ferrets, a 15 μg antigen dose (without adjuvant) was immunogenic in rabbits and protective in ferrets, even though no detectable HI or MN titers were evident in ferrets. Thus, in order to determine the potential benefits of adjuvant inclusion in these species, lower antigen doses may need to be employed when administered with adjuvant.
Another potential contributing factor for discrepancies between experiments is the manufacturing process and source of the antigen and the adjuvant. Regarding this point, a mouse experiment evaluating antigen and adjuvant from different manufacturers showed equivalent responses (). Unfortunately, the antigen manufacturing Process Y employed to produce the antigen used in all of the preclinical mouse and rabbit studies was not the same process recommended for final production of the antigen (Process Z) for the ferret study due to regulatory, manufacturability, and antigen stability concerns related to seasonal strains (data not shown). The SE adjuvant performed well with the SP antigen in the initial ferret challenge experiment (), demonstrating comparable protective efficacy and antigen sparing ability as that reported for AS03 in a study employing a similar dose range.Citation18 Nevertheless, the same batch of SE adjuvant failed to enhance immune responses in a follow-up ferret challenge experiment employing the H5N1-Z antigen from CI (). Although similar processes were employed to manufacture the antigens from CI and Sanofi, it is notable that the 15 μg of H5N1-Z (without adjuvant) resulted in a trend of improved protection and clinical scores in challenged ferrets compared to the same dose of SP antigen (H5N1-S in ) employed in the previous ferret experiment (). Nevertheless, the 3.75 μg dose of H5N1-Z (with adjuvant) showed a trend of decreased protection and other clinical readouts compared to the same dose of H5N1-S with adjuvant, indicating that some difference in the composition (such as microbial TLR ligand, see Fig. S5) or structure of the H5N1-Z did not facilitate further enhanced biological activity when combined with adjuvant. In vitro tests, such as the one described to evaluate TLR ligands, can be in this case suitable for additional antigen characterization and evaluation of adjuvant contribution.
While H5N1 is generally considered a weak antigen, requiring high antigen amounts to generate sufficient serological response,Citation19 it is interesting that no HI or MN titers were detected in either ferret experiment even after the boost immunization, regardless of whether vaccines included adjuvant. Previous reports on H5N1 vaccine studies in ferrets have indicated at least some HI titer response when adjuvant is present.Citation18,20,21 Since 100% protection was achieved in several of the groups in the current study, alternative immunological mechanisms may function in protection in ferrets, such as CD4+ T-cell responsesCitation22 or antibody (non-HI antibody) dependent cytotoxicity (ADCC) responses.Citation23 In ferrets, influenza virus infection of the central nervous system (CNS) is particularly common with highly pathogenic A influenza H5N1 viruses. The neurovirulence may correlate with the strain, dose and intranasal administration which allow spread to the olfactory bulb and by cranial nerves into the brain.Citation10,24,25 However, the vaccines studied here did not significantly reduce histopathology scores in the brain or other tissues of the ferrets (Figs. S3 and S4).
Obviously, it is preferred to have an optimized final antigen manufacturing process before embarking on an adjuvant development program. However, economic and political realities may preclude manufacturers from focusing only on one development aspect at a time, and antigen-adjuvant compatibility considerations may even help determine an optimal antigen manufacturing process. Nevertheless, we recommend that developing country influenza vaccine manufacturers that are considering employing adjuvant in their programs should ensure that their antigen manufacturing processes are optimized as much as possible such that adjuvant development efforts can focus on evaluating the antigen sparing capabilities of the adjuvant through systematic studies in the appropriate animal models. Furthermore, initial antigen dose ranging studies in each animal model will help reduce the number of studies required since antigen doses too high or too low may reduce the ability to show significant benefit from adjuvant inclusion. In this respect, it is hoped that the data reported here highlight the importance of systematic experimental design.
In contrast to developed country manufacturers, developing country manufacturers may have a higher likelihood of encountering challenges related to out-dated facilities or manufacturing processes, resource or personnel constraints, and availability of reagents. Thus, the difficulties described in the present work were related to efforts to modernize both seasonal and pandemic antigen manufacturing processes and equipment while simultaneously acquiring novel adjuvant manufacturing technology. Moreover, a significant portion of the work was being performed under timeline and resource pressure related to the local political and economic situation. Such complications significantly increased the difficulty of the project, and the potential for similar challenges in other developing country technology transfer efforts should not be underestimated.
Together, these recommendations mean that vaccine developers and technology transfer partners should take into account the timelines and sequence of events necessary to ensure the most efficient effort. Moreover, it must not be assumed that seemingly minor antigen process modifications will not affect the capacity of the adjuvant for antigen sparing. Despite the challenges described above, this manuscript, together with our previous report,Citation2 demonstrates the technology transfer and subsequent manufacture of a clinical adjuvant with antigen sparing capacity by a developing country manufacturer. The access of adjuvant technology by developing countries is a critical factor in global pandemic influenza preparedness.
Conclusion
The preclinical evaluation of inactivated split virion H5N1 influenza vaccine adjuvanted with locally manufactured adjuvant enhanced vaccine immunogenicity in mice and rabbits and protective efficacy in ferrets, resulting in antigen sparing capacity. However, such adjuvant-related benefits were dependent on antigen source and manufacturing process, which was being developed in parallel with the preclinical activities. The lessons learned from the practical difficulties encountered may be useful to inform other technology transfer projects involving developing country manufacturers.
Materials and methods
Adjuvant
Manufacture and quality control of stable oil-in-water emulsion (SE) was performed as described previously,Citation2 or with slight modification, namely the addition of a small amount of antioxidant to the oil phase (volume ratio of 1:189 α-tocopherol:squalene) to alleviate oxidative instability apparent in initial adjuvant batches. The mouse and rabbit studies were completed with adjuvant not containing antioxidant whereas the ferret studies employed adjuvant containing antioxidant. As used in vaccines, the primary differences between MF59®, a squalene-based emulsion used in approved influenza vaccines in many countries, and SE are that the latter is 2% v/v squalene primarily emulsified with phospholipid whereas MF59® is 2.3% v/v squalene and employs non-ionic surfactant emulsifiers. Briefly, SE manufacture consisted of mixing squalene oil (SAFC) with or without α-tocopherol (Spectrum Chemical), and egg phosphatidylcholine (Avanti Polar Lipids) in a water bath sonicator. The oil phase was mixed under high shear with an aqueous phase consisting of poloxamer 188 (Sigma-Aldrich or Spectrum Chemical), glycerol (Sigma-Aldrich or Spectrum Chemical), and ammonium phosphate buffer (Sigma-Aldrich or J.T. Baker). The crude emulsion was then processed by high pressure homogenization and filtered through a 0.2-μm filter. Characterization of the adjuvant consisted of dynamic light scattering for particle size, visual appearance, gas chromatography for squalene content, pH measurement, and osmolality measurement, as described previously.Citation2 EM022 is a squalene emulsion with polysorbate 80 (NOF Corp.) and sorbitan trioleate (Sigma-Aldrich) emulsifiers in a 10 mM citrate buffer (J.T. Baker) manufactured by high pressure homogenization as described earlier.Citation6
Antigen
Split virion inactivated influenza H5N1 antigen was produced by Sanofi Pasteur (designated SP hereafter) obtained from the National Pre-Pandemic Influenza Vaccine Stockpile (NPIVS) through HHS or by CI. For antigen produced by CI, the manufacturing process and quality control tests followed the same process described in detail previously (2) or with modification as described below and illustrated schematically in . Briefly, virus reassortant NIBRG-14 (A/Vietnam/1194/04) supplied by NIBSC (UK) was propagated in SPF hen eggs for the seed batch. For production batches, reassortant vaccine virus was propagated in conventional hen eggs followed by harvesting of allantoic fluid that is then prefiltered, clarified, and purified by zonal centrifugation in phosphate buffer (processes X and Z) or citrate buffer (process Y). Inactivation was achieved by formaldehyde (processes X and Z) or by β-propiolactone (process Y). Following removal of sucrose, the splitting process is achieved by treatment with ether (process X) or Triton™ X-100 detergent (processes Y and Z). The inactivated split virion vaccine is then sequentially filtered through 0.45 and 0.22 μm membranes. H1N1 (A/California/7/2009) was also produced by CI using process X. The basic production process of the vaccine from SP is similar to process Z based on a previous report (19) as well as the package insert; this antigen is designated hereafter as SP antigen or H5N1-S.
Preclinical animal experiments
All protocols on mice and rabbits were approved by the Internal Ethics Committee of CI. All animal care was in accordance with standards set forth in the Council Directive 86/609/EEC. The ferret studies were approved by the Institutional Animal Care and Use Committee (IACUC) at Colorado State University and carried out according to National Institute of Health (NIH) guidelines. All work with this virus isolate was approved by appropriate institutional offices and federal agencies (CDC/USDA). In all immunization designs, inactivated split virion was combined 1:1 on the day of immunization with SE adjuvant (final 2% oil-in-water stable emulsion) or phosphate buffered saline (PBS), according to the immunization design.
Mice
BALB/c female mice (6–10 animals/group), 6–8 weeks of age, were used from CI's SPF animal facility, with free access to standard chow and tap water. Immunizations consisted of two 0.1 ml intramuscular inoculations in 2 sites (quadriceps), at 3 week intervals according to established protocols of antigen doses and formulation. The sera were collected by retro-orbital bleeding before the study and after immunization at day 21, 28, 42 and 63 as indicated. For ELISPOT tests on day 28, mice were sacrificed to harvest spleen and femurs (bone marrow).
Rabbits
New-Zealand white rabbit males 4–6 months of age (5 animals/group) were used from the breeding colony of the CI animal facility. Rabbits were immunized twice, 3 weeks apart, with 0.5 ml intramuscular injection in the caudal muscle of the hind leg. Sera were obtained from the auricular vein on day 0, 21 and 28.
Serological evaluation
Serum samples were assessed by microneutralization (MN) and hemagglutination inhibition (HI) assays. For both techniques animal serum samples were treated with receptor-destroying enzyme (RDE) and heat inactivated for 30 min at 56°C. The influenza viruses were high growth reassortants provided by NIBSC: NIBRG-14 (A/Vietnam/1194/04), NIBRG-23 (A/turkey/Turkey/1/2005), NIBRG-88 A/Cambodia/R0405050/2007, and NYMC X179A (A/California/7/2009). Virus stocks used as antigens were propagated in the allantoic cavities using standard procedures.
Microneutralization (MN) assay
The 50% cell culture infectious dose (CCID50) of each virus was determined by titration on Madin-Darby canine kidney (MDCK) cells and calculated by the method of Reed and Muench (Citation26). Duplicates of serial diluted sera were preincubated with a standardized amount of influenza virus (100 TCID50) before the addition to the monolayer of MDCK cells. After ˜40 h incubation, cells were fixed and the presence of influenza A virus nucleoprotein in infected cells was detected by ELISA. Four positive virus controls and 4 negative cell controls were included in each plate. The absorbance was measured at 460 nm with an automated plate spectrophotometer. The average A460 was determined for virus-infected (VC) and -uninfected (CC) control wells, and a neutralizing endpoint was determined by using a 50% specific signal calculation. The endpoint titer was expressed as the reciprocal of the highest dilution of serum with A460 value less than X, where X = [(average A460 of VC wells) − (average A460 of CC wells)]/2 + (average A460 of CC wells). Sera which tested negative at a dilution of 1/20 were assigned a titer of 10.
Hemagglutination inhibition (HI) assay
Triplicates of RDE treated serial diluted sera were assessed in U- or V-bottom 96-well microtiter plates with 0.5% turkey or 1.0% v/v horse erythrocytes. Two-fold serial dilutions of sera (25-μL system) were mixed with an equal volume of diluent containing each influenza virus reassortant at 4 HA units/25 μL. Four control wells of virus plus diluent or diluent alone were included on each plate. Plates were covered and incubated for 120–140 min at room temperature (15–30°C). Fifty (50) μL of 1.0% horse erythrocytes were then added to every well on all plates and incubated for 60–75 minutes at room temperature (15–30°C). Plates were then tilted and read for agglutination. Sera which tested negative at a dilution of 1/10 were assigned a titer of 5. Seroprotection was defined as HI titer ≥1:40 and seroconversion was defined as ≥4-fold increase in HI titer post-vaccination compared to pre-vaccination.
Antibody ELISA
Flat bottom 96-well Nunc MediSorp plates were coated overnight at 4°C with 50 μL antigen diluted to 5 μg/mL HA in PBS, washed 3 times with PBS containing 0.05% Tween 20, blocked with 150 μL of 1% BSA in PBS for 2h at room temperature and washed 3 times with PBS containing 0.05% Tween 20. Serum samples, serially diluted 4-fold (range 1:400 to 1:409600) in PBS containing 1% BSA, were added to the antigen coated plates and incubated 3 h at room temperature. The wells were washed 5 times (PBS, 0.05% Tween 20), before being incubated for 1 h with 50 μL/well of horseradish peroxidase conjugated goat anti-mouse IgG (R&D Systems), rat anti-mouse IgG1 or rat anti-mouse IgG2a (BD Biosciences), 1000-fold diluted in 1% BSA in PBS. After an additional 5 washes, 100 μL per well of TMB substrate (R&D Systems) was added and incubated for 20 min at room temperature. Reactions were stopped with 50 μL 2N H2SO4 and absorbance was measured at 450 nm on a Tecan Infinite M1000 microplate reader. To allow normalization across plates, an internal standard was produced from a random pool of 200 immune sera from the same experiment. The internal standard was tested on each plate in 6 serial dilutions (4 fold - 1:2000 to 1:2048000) and a 4 parameter logistic regression model with power of the mean error estimation was fitted to the measured values. Sample dilutions falling within standard range and not showing an estimated error greater than 30% were averaged by arithmetic mean and samples below detection were assigned a value of zero. A calculated value of 1 indicates antibody reactivity equal to that of the pooled sera. All calculations were performed in R version 3.1.1(Citation27) using the calibFit 2.1.0 package (Citation28).
Antibody-secreting plasma cell enumeration
Detection of antibody-secreting plasma cells was performed using Mouse IgM ELISPOT kit from Mabtech as per manufacturer's instructions. Briefly, ELISPOT plates (96 wells) were coated with antigen (10 μg/mL HA concentration), or total IgG capture antibody (15 μg/mL), or BSA (0.1%) overnight at 4°C. Plates were washed 5 times with sterile PBS and blocked with 1% BSA in PBS for 2 h at room temperature. Freshly isolated bone marrow cells (femurs) from immunized mice were added in concentrations of 106 or 105 per well, in triplicate, and incubated for 16 h at 37°C, 5% CO2, in RPMI-1640 media containing 10% FCS and 2-mercaptoethanol. Cells were removed and the wells were washed 5 times before addition of biotinylated anti-mouse IgG detection antibody (1 μg/mL) followed by incubation for 2 h at room temperature. Steptavidin-HRP (diluted 1000-fold) was added and incubated 1 h at room temperature and the wells were washed 5 times with PBS. Colorimetric detection was performed with filtered BCIP/NBT-plus solution and the reaction was allowed to continue until visible spots were formed and subsequently stopped by extensive washing in tap water. Images were captured on an AID iSpot FluoroSpot Reader under white LED illumination and image processing and automated spot detection and counting were performed in ImageJ(Citation29) using a macro written in-house.
Cytokine-producing cell enumeration
Detection of antigen-specific IFN-γ and IL-5 secreting cells was performed using Mouse IFN-γ/IL-5 FluoroSpot kit from Mabtech as per manufacturer instructions. Briefly, plates were coated with 100 μL of 15 μg/mL anti-IFN-γ and anti-IL-5 capture antibodies overnight at 4°C, washed 5 times in sterile PBS and blocked with 1% BSA in PBS for 2 h. Splenocytes isolated from immunized mice were incubated at 106 and 105 cells per well, in triplicate, stimulated with either antigen (10 μg/mL HA), ConA (5 μg/mL) or PBS and incubated for 36 h at 37°C, 5% CO2, in RPMI-1640 media containing 10% FCS and 2-mercaptoethanol. Following incubation, cells were removed by 5 PBS washes and 100 μL/well of detection antibody solution (1:200 diluted anti-IFN-γ-FITC and 1:500 diluted anti-IL-5-biotin in PBS with 0.1% BSA) were added, and incubated for 2 h at room temperature. Following 5 PBS wash steps, detection was performed by incubation with anti-FITC-green (1:100) and Streptavidin-red (1:200) secondaries for 1 h at room temperature in the dark. The wells were washed 5 more times, followed by 15 min incubation with Fluorescence enhancer-II and drying in the dark. Fluorescence photographs, using the appropriate filters were acquired on a AID iSpot FluoroSpot Reader and spot detection and counting was performed using the AID EliSpot software Version 7.0.
Ferret immunization and viral challenge
Split virion inactivated influenza H5N1 antigen was combined on the day of immunization with SE adjuvant (final 2% oil-in-water stable emulsion) or phosphate buffered saline (PBS), according to the immunization design. Ferrets, purchased from Triple F Farms (Sayer, PA), were injected with 500 μL vaccine in the right quadriceps muscle at Day 0 and 500 μL vaccine in the left quadriceps muscle at Day 21. Experiments using the highly pathogenic H5N1 influenza virus, A/Vietnam/1203/04, were performed under the guidance of the U.S. National Select Agent Program in negative pressure HEPA-filtered BSL-3+ laboratories at Colorado State University. Virus challenge was carried out on Day 42 using intranasal inoculation with virus diluted in sterile PBS. Ferret inoculations were carried out in a total volume of 500 μL containing 9.4 × 105 pfu, with 250 μL inoculated into each nare. Following virus challenge, animals were monitored daily for 14 d for weight loss, body temperature (IPTT-300 transponders, BioMedic Data Systems, Seaford DE), and general health using a 5 point clinical score (0 = normal to 4 = poorly responsive or moribund). Nasal flushing samples were collected on days 1, 3, 5, 7 and 9 post-challenge, and stored frozen (-70°C) until samples were analyzed for virus quantitation. Animals were euthanized humanely if body weight loss reached 25% or clinical appearance was scored as 4; surviving ferrets were euthanized and necropsied 14 d following challenge. Virus titrations from sera and tissues, microneutralization serologic assays and histopathologic analyses were performed as described previously (Citation30). Tissues collected at necropsy were fixed in 10% neutral-buffered formalin, embedded in paraffin and sections stained with hematoxylin and eosin using standard procedures. Lesions were scored on a scale of 0 (normal) to 6 (severe and affecting greater than 50% of organ) based on degree of inflammation and, in the case of lung, pneumocyte hypertrophy by a pathologist blinded to the groupings.
In vitro TNF-α release from human cells
TNF-α release was performed on the differentiated human monocytic cell line THP-1, with cells purchased from the Health Protection Agency Culture Collections. THP-1 were grown in RPMI-1640 medium supplemented with 10% fetal bovine serum (FBS), 1 mM L-glutamine, 100 U/ml penicillin and 100 mg/ml streptomycin (complete culture medium) in a humidified incubator at 37°C with 5% CO2. Cell density was kept at <5 × 105 cells/mL by diluting with fresh complete culture medium every 2 d. Differentiation of THP-1 cells into macrophages was induced by treatment with 30 ng/ml phorbol-12-myristate-13 acetate (PMA) in complete culture medium for 72 h. After 72 h, the PMA-containing medium was removed and replaced by fresh complete culture medium. Cells were maintained in culture with complete medium only for an additional 24 h for recovery after PMA treatment, and were then collected for use in the experiments. PMA-differentiated THP-1 cells were stimulated for 24 h with 100 ng/mL of ultra pure lipopolysaccharide (LPS) from E. coli 0111:B4 strain (EcLPS) or probes. Unstimulated cells were used as a negative control. Cell-free supernatants were harvested after 24 h of stimulation and kept at −20°C until the cytokine measurements. The release of TNF-α in culture supernatants was determined by a sandwich ELISA technique using the DuoSet ELISA Development Systems (R&D Systems Inc., Minneapolis, USA) according to the manufacturer's instructions. In order to verify the specificity, anti-TLR2 and anti-TLR4 antibodies or MYD88 and TRIF inhibitory peptides were used.
Disclosure of potential conflicts of interest
No potential conflicts of interest were disclosed.
2015HV0342R1-s02.doc
Download MS Word (487 KB)Acknowledgments
We acknowledge the contributions from the technology transfer teams from IDRI (Tony Phan, Patricia Hon, Tara Evers) and CI (Francisc Czobor, Mariana Saulea, Aliana Mihalcea, Leontina Banica, Doina Proteasa, Adina Iancu, Daniela Florescu, Chirita Daniel, Dorel Lucian Radu, Maria Elena Mihai, Elena Enache, Denisa Mihai and the QC laboratory), BARDA project officers and advisers for helpful discussions (Louis Mocca, Mario Barro, Sheng Li, Rick Bright), and excellent technical and project assistance at Southern Research Institute (Donna Bowen, Michelle Bell, Logan Haller, Shixiong Li, Candi Looney, Valerie Johnson, Barbara Taggart). BARDA and Sanofi Pasteur are gratefully acknowledged for providing access to the Sanofi Pasteur A/Vietnam H5N1 split-virus inactivated vaccine.
Funding
This work reported in this publication was supported by a Biomedical Advanced Research and Development Authority (BARDA) cooperative agreement (IDSEP100008) in the Office of the Assistant Secretary for Preparedness and Response (ASPR), United States Department of Health and Human Services (HHS). The findings and conclusions in this report are solely those of the authors and do not represent the views of the United States Department of Health and Human Services or its components.
References
- Kieny MP. Global action plan for influenza vaccines: what still needs to be done? Seventh Meeting with International Partners on Prospects for Influenza Vaccine Technology Transfer to Developing Country Vaccine Manufacturers; Dubai, UAE, 25 March 2014, www.who.int/phi/DAY1_01_Kieny_PM_Dubai2014.pdf
- Fox CB, Huynh C, O'Hara MK, Onu A. Technology transfer of oil-in-water emulsion adjuvant manufacturing for pandemic influenza vaccine production in Romania. Vaccine 2013; 31:1633-40; PMID:23103197; http://dx.doi.org/10.1016/j.vaccine.2012.10.048
- Baudner BC, Ronconi V, Casini D, Tortoli M, Kazzaz J, Singh M, Hawkins LD, Wack A, O'Hagan DT. MF59 emulsion is an effective delivery system for a synthetic TLR4 agonist (E6020). Pharm Res 2009; 26:1477-85; PMID:19255727; http://dx.doi.org/10.1007/s11095-009-9859-5
- O'Hagan DT, Ott GS, Van Nest G, Rappuoli R, Giudice GD. The history of MF59 adjuvant: a phoenix that arose from the ashes. Exp Rev Vaccines 2013; 12:13-30; PMID:23256736; http://dx.doi.org/10.1586/erv.12.140
- O'Hagan DT, Singh M. MF59: a safe and potent oil-in-water emulsion adjuvant. In: Singh M, editor. Vaccine Adjuvants and Delivery Systems. Hoboken, NJ: John Wiley & Sons; 2007. p. 115-29.
- Fox CB, Barnes VL, Evers T, Chesko JD, Vedvick TS, Coler RN, Reed SG, Baldwin SL. Adjuvanted pandemic influenza vaccine: variation of emulsion components affects stability, antigen structure, and vaccine efficacy. Influenza Other Respi Viruses 2013; 7:815-26; PMID:23122325; http://dx.doi.org/10.1111/irv.12031
- Smith H, Sweet C. Lessons for human influenza from pathogenicity studies with ferrets. Rev Infect Dis 1988; 10:56-75; PMID:3281223; http://dx.doi.org/10.1093/clinids/10.1.56
- Ibricevic A, Pekosz A, Walter MJ, Newby C, Battaile JT, Brown EG, Holtzman MJ, Brody SL. Influenza virus receptor specificity and cell tropism in mouse and human airway epithelial cells. J Virol 2006; 80:7469-80; PMID:16840327; http://dx.doi.org/10.1128/JVI.02677-05
- Maher JA, DeStefano J. The ferret: an animal model to study influenza virus. Lab Anim 2004; 33:50-3; PMID:15457202; http://dx.doi.org/10.1038/laban1004-50
- Tanaka H, Park CH, Ninomiya A, Ozaki H, Takada A, Umemura T, Kida H. Neurotropism of the Hong Kong H5N1 influenza virus in mice. Vet Microbiol 1997; 95:1-13; PMID:12860072; http://dx.doi.org/10.1016/S0378-1135(03)00132-9
- van Riel D, Munster VJ, de Wit E, Rimmelzwaan GF, Fouchier RAM, Osterhaus AD, Kuiken T. Virus attachment to lower respiratory tract. Science 2006; 312:399; PMID:16556800; http://dx.doi.org/10.1126/science.1125548
- van Riel D, den Bakker MA, Leijten LME, Chutinimitkul S, Munster VJ, de Wit E, Rimmelzwaan GF, Fouchier RAM, Osterhaus ADME, Kuiken T. Seasonal and pandemic human influenza viruses attach better to human upper respiratory tract epithelium than avian influenza viruses. Am J Pathol 176(4):1614-8; PMID:20167867; http://dx.doi.org/10.2353/ajpath.2010.090949
- Jackson LA, Campbell JD, Frey SE, Edwards KM, Keitel WA, Kotloff KL, Berry AA, Graham I, Atmar RL, Creech CB, et al. Effect of varying doses of a monovalent h7n9 influenza vaccine with and without as03 and mf59 adjuvants on immune response: A randomized clinical trial. JAMA 2015; 314(3):237-46; PMID:26197184; http://dx.doi.org/10.1001/jama.2015.7916
- Hamidi A, Boog C, Jadhav S, Kreeftenberg H. Lessons learned during the development and transfer of technology related to a new Hib conjugate vaccine to emerging vaccine manufacturers. Vaccine 2014; 32(33):4124-30; PMID:24923635; http://dx.doi.org/10.1016/j.vaccine.2014.05.023
- Ventura R, Brunner L, Heriyanto B, de Boer O, O'Hara M, Huynh C, Suhardono M, Collin N. Technology transfer of an oil-in-water vaccine-adjuvant for strengthening pandemic influenza preparedness in Indonesia. Vaccine 2013; 31(12):1641-5; PMID:22884665; http://dx.doi.org/10.1016/j.vaccine.2012.07.074
- Crager SE. Improving global access to new vaccines: intellectual property, technology transfer, and regulatory pathways. Am J Public Health 2014; 104(11):e85-e91; PMID:25211753; http://dx.doi.org/10.2105/AJPH.2014.302236
- Stephenson I, Wood JM, Nicholson KG, Zambon MC. Sialic acid receptor specificity on erythrocytes affects detection of antibody to avian influenza haemagglutinin. J Med Virol 2003; 70(3):391-8; PMID:12767002; http://dx.doi.org/10.1002/jmv.10408
- Leroux-Roels G. Prepandemic H5N1 influenza vaccine adjuvanted with AS03: a review of the pre-clinical and clinical data. Expert Opin Biol Ther 2009; 9(8):1057-71; PMID:19555313; http://dx.doi.org/10.1517/14712590903066695
- Treanor JJ, Campbell JD, Zangwill KM, Rowe T, Wolff M. Safety and immunogenicity of an inactivated subvirion influenza A (H5N1) vaccine. New Eng J Med 2006; 354:1343-51; PMID:16571878; http://dx.doi.org/10.1056/NEJMoa055778
- Layton RC, Petrovsky N, Gigliotti AP, Pollock Z, Knight J, Donart N, Pyles J, Harrod KS, Gao P, Koster F. Delta inulin polysaccharide adjuvant enhances the ability of split-virion H5N1 vaccine to protect against lethal challenge in ferrets. Vaccine 2011; 29(37):6242-51; PMID:21736913; http://dx.doi.org/10.1016/j.vaccine.2011.06.078
- Middleton D, Rockman S, Pearse M, Barr I, Lowther S, Klippel J, Ryan D, Brown L. Evaluation of vaccines for H5N1 influenza virus in ferrets reveals the potential for protective single-shot immunization. J Virol 2009; 83:7770-8; PMID:19457991; http://dx.doi.org/10.1128/JVI.00241-09
- Cox R. Correlates of protection to influenza virus, where do we go from here? Hum Vaccines Immunother 2013; 9(2):405-8; PMID:23291930
- DiLillo DJ, Tan GS, Palese P, Ravetch JV. Broadly neutralizing hemagglutinin stalk-specific antibodies require Fc[gamma]R interactions for protection against influenza virus in vivo. Nat Med 2014; 20(2):143-51; PMID:24412922; http://dx.doi.org/10.1038/nm.3443
- Bodewes R, Kreijtz JH, Van Amerongen G, Fouchier RA, Osterhaus AD, Rimmelzwaan G, Kuiken T. Pathogenesis of influenza A/H5N1 virus infection in ferrets differs between intransal and intratracheal routes of inoculation. Am J Path 2011; 179:30-6; PMID:21640972; http://dx.doi.org/10.1016/j.ajpath.2011.03.026
- Park CH, Ishinaka M, Takada A, Kida H, Kimura T, Ochiai K, Umemura T. The invasion routes of neurovirulent A/Hong Kong/483/97 (H5N1) influenza virus into the central nervous system after respiratory infection in mice. Arch Virol 2002; 147:1425-36; PMID:12111416; http://dx.doi.org/10.1007/s00705-001-0750-x
- Reed LJ, Muench H. A simple method of estimating fifty per cent endpoints. Am J Epidemiol 1938; 27(3):493-7
- R Core Team. R: a language and environment for statistical computing. Vienna, Austria: R Foundation for Statistical Computing; 2014 [updated 2014; cited]; Available from: https://www.R-project.org.
- Haaland P, Samarov D, McVey E. calibFit: Statistical models and tools for assay calibration. R package version 2.1.0. 2011 https://CRAN.R-project.org/package=calibFit.
- Schneider CA, Rasband WS, Eliceiri KW. NIH Image to ImageJ: 25 years of image analysis. Nat Methods 2012; 9:671-5; PMID:22930834; http://dx.doi.org/10.1038/nmeth.2089
- Clegg CH, Roque R, Perrone LA, Rininger JA, Bowen R, Reed SG. GLA-AF, an emulsion-free vaccine adjuvant for pandemic influenza. PLoS One 2014; 9(2):e88979; PMID:24551202; http://dx.doi.org/10.1371/journal.pone.0088979