ABSTRACT
Generation of protective immunity at mucosal surfaces can greatly assist the host defense against pathogens which either cause disease at the mucosal epithelial barriers or enter the host through these surfaces. Although mucosal routes of immunization, such as intranasal and oral, are being intensely explored and appear promising for eliciting protective mucosal immunity in mammals, their application in clinical practice has been limited due to technical and safety related challenges. Most of the currently approved human vaccines are administered via systemic (such as intramuscular and subcutaneous) routes. Whereas these routes are acknowledged as being capable to elicit antigen-specific systemic humoral and cell-mediated immune responses, they are generally perceived as incapable of generating IgA responses or protective mucosal immunity. Nevertheless, currently licensed systemic vaccines do provide effective protection against mucosal pathogens such as influenza viruses and Streptococcus pneumoniae. However, whether systemic immunization induces protective mucosal immunity remains a controversial topic. Here we reviewed the current literature and discussed the potential of systemic routes of immunization for the induction of mucosal immunity.
Introduction
The vast mucosal surfaces covering the gastrointestinal, urogenital and respiratory tracts, as well as the conjunctiva, inner ear and ducts of the exocrine glands, are endowed with powerful mechanical and physicochemical mechanisms that either prevent the entry of foreign bodies (including microorganisms) or facilitate their degradation.Citation1,2 Highly specialized innate and adaptive mucosal immune responses at these surfaces are of major importance to modulate the colonization of commensal and pathogenic microorganisms, and to defend the host against the extravasation of the pathogens through the epithelium to cause diseases at other tissues.Citation3 Extensive research has demonstrated that secretory IgA is the main immunoglobulin isotype mediating humoral immunity at mucosal surfaces, but some studies have shown that locally produced IgM and IgG also contribute to the mucosal immune defense.Citation4-10 Therefore, vaccines that generate protective antibody (and cell-mediated) responses at mucosal sites would greatly advance the field of vaccinology.
Mucosal route of immunization elicits immune responses at the local and distal mucosal sites, as well as systemic immune responses. Therefore, most current efforts attempting to elicit protective mucosal immunity have focused on the mucosal (such as oral and intranasal) routes of vaccination. Although live, attenuated oral vaccines are generally immunogenic and induce excellent protective immunity against the targeted pathogen, the production of such vaccines are complex and need to grow large amounts of the pathogen prior to their attenuation. The use of nonpathogenic mutants is relatively safer, but suffers from the potential risk of reversion to virulence. In contrast, non-replicating mucosal vaccines, based on subunit or acellular antigens, would be preferable from safety perspectives. However, subunit oral vaccines require administration of relatively large amounts of antigens to compensate for antigen degradation in the gastrointestinal tract, the co-administration of potent adjuvants and/or delivery system to facilitate antigen uptake by the antigen presenting cells (APC), and the need for neutralization of stomach acids prior to vaccine administration.Citation11 In contrast, the intranasal (i.n.) route of immunization requires lesser amounts of antigens than the oral administration, but the safety and efficacy of i.n. vaccines remain to be established.Citation12-14 For example, the currently licensed influenza vaccine, FluMist, is not recommended for children aged <2 yr or children aged <5 yr with a history of recurrent wheezing, or for asthmatic children and adults of any age.Citation15 Although great advances have been made toward the development of safe and effective subunit mucosal vaccines, there has been a renewed interest in investigating the potential of systemic immunization for eliciting mucosal immunity.
Systemic immunization has generally been considered as incapable of generating protective mucosal immune responses for a longtime now, however, cumulative data from recent studies suggest that some systemically administered vaccines are capable of eliciting mucosal immune responses, including secreting IgA antibodies. Such systemic vaccines may offer potential manufacturing and regulatory advantages over the mucosal vaccines. Here, we reviewed the current literature and discussed the potential of systemic routes of immunization with non-replicating vaccines for inducing mucosal immunity in mammalian hosts Citation(Tables 1–3). We have also included some references to transcutaneous immunization (TCI) in this review, since many of these studies involve the use of micro-needles or other means to penetrate past the intact skin surface to deliver the vaccine to the epidermis.
Intraperitoneal immunization
Intraperitoneal (i.p.) administration of vaccines has long been used and studied as an experimental immunization route for the induction of systemic immunity in animal models of vaccination. However, the i.p. route, in certain antigen-adjuvant combinations, has also been reported to induce mucosal immune responses, particularly gastrointestinal IgA responses. For example, i.p. immunization of an inactivated poliovirus vaccine with 1,25-Dihydroxyvitamin D3 as an adjuvant significantly promoted not only serum IgG but also salivary IgA responses in mice.Citation16 Robust antigen-specific serum IgG and pulmonary IgA responses were generated in pigs upon i.p. immunization with a Mycoplasma hyopneumoniae antigen co-administered with an oil emulsion.Citation17 The i.p. administration of Bacillus thuringiensis Cry1Ac protoxin in mice generated high levels of IgG and IgM, and low but detectable levels of IgA in sera and the lavage fluids from various mucosal sites (vagina, respiratory tract, small and large intestine).Citation18 The magnitude of individual Ig isotype responses induced appears to be depended on the mucosal site analyzed, with IgA being the highest in small intestine and both IgG and IgM being the strongest in respiratory tract. Although the protective efficacies of the induced mucosal immune responses were not evaluated in this study, a subsequent study by this group has shown that the mucosal immune responses elicited by i.p. immunization with the Cry1Ac protoxin and amoebal lysates enhances the protection against lethal intranasal challenges with Naegleria fowleri in mice.Citation19 Similarly, i.p. administration of an inactivated SARS Coronavirus (SARS-CoV) vaccine adjuvanted with a Poly (I:C) derivative induced antigen-specific IgG and IgA responses at multiple mucosal sites in mice, with the highest levels in the intestine and less significant but robust responses in vaginal washes and lowest responses in the mouth/saliva, while only strong IgG but no IgA responses were observed in sera and lungs.Citation20 Moreover, those systemic and mucosal antibodies were effective in virus neutralization activity.Citation20 In contrast, i.p. immunization of mice with mycobacterium PstS-1 antigen failed to induce any specific IgA responses in bronchoalveolar lavage (BAL) or saliva, nor did it induce cytokine responses (e.g., IL-4, IL-5 and IFN-γ) in the lungs, although strong serum IgG responses were observed.Citation21 In another study, little protection was observed against pulmonary infection in mice after i.p. vaccination with a cholera toxin (CT)-adjuvanted Mycoplasma pulmonisvaccine.Citation22 In a clinical study, i.p. immunization of patients on continuous ambulatory peritoneal dialysis with tetanus toxoid elicited significant specific IgG and IgA responses in sera and peritoneal fluids, and salivary IgG but failed to induce secretory IgA responses.Citation23 The inefficiency of i.p. immunization in generating mucosal immune responses was also observed in several other studies.Citation24,25
However, virus-like particles (VLPs) delivered by i.p. route have shown good potential in generating both systemic and mucosal immune responses. For example, i.p. administration of mice with CpG-adjuvanted SARS-CoV VLPs increased antigen-specific IFN-γ and IL-4 producing cell populations in the spleen, and IgA antibodies in lungs, intestine, feces, and vaginal washes.Citation26 Interestingly, i.p. immunization of mice with rotavirus 2/6 VLPs was shown to be more effective than oral immunization in the induction of mucosal IgG and IgA in the feces and uterine fluids, and serum IgG responses.Citation27 Furthermore, it is interesting to note that i.p. immunization with HIV-1 VLPs could induce significant cross-clad neutralizing antibodies against both autologous and heterologous primary isolates in sera and vaginal washes, and elicit stronger cytotoxic T lymphocyte (CTL) responsesthani.n.immunization.Citation28
Intramuscular immunization
Intramuscular (i.m.) administration is the most predominant vaccine delivery method for humans, and it enables relatively larger volumes to be injected.Citation29 In addition, i.m. immunization has been widely used in the immunogenicity and efficacy studies of experimental DNA vaccines. Those studies have demonstrated that i.m. vaccination can promote both systemic and mucosal immune responses, and protect against mucosal pathogen challenge.Citation30-34 For example, i.m. immunization with anti-caries DNA vaccine encoding S. mutans antigens fused to cytotoxic T lymphocyte antigen-4 (CTLA-4) elicited strong serum IgG and salivary IgA responses in both rabbits and monkeys.Citation35 Moreover, i.m. immunization of 2-week-old calves with a bovine respiratory syncytial virus (BRSV) DNA vaccine induced antigen-specific IgG and IgA responses in sera and BAL fluids, and accorded protection against i.n.BRSV challenges.Citation36 More importantly, i.m. immunization of a bovine rotavirus VP6 DNA vaccine effectively protected mice against oral challenges with a murine rotavirus strain by reducing virus shedding in feces, suggesting that heterologous protection can be obtained by i.m. immunization of VP6 DNA vaccine.Citation37 Heterologous protection was also observed against i.n. H5N1 challenge in ferrets i.m. immunized with H1N1 VLPs.Citation38 However, in mice only homologous protection was observed. In a human trial involving 6 healthy female volunteers, i.m. immunization with an alum-adjuvanted human papilloma virus (HPV) vaccine increased the numbers of circulating IgG- and IgA-secreting cells (ASCs) and generated HPV-specific IgG and neutralizing antibodies in sera, and cervical and vaginal wash fluids,Citation39 in consistence with the previous work where women i.m, immunized with HPV16 VLPs in menstrual cycle developed antigen-specific IgG in cervical secretions.Citation40 Furthermore, it was found that i.m. vaccination with an inactivated influenza virus elicited wide dispersion of IgG memory B cells to secondary lymphoid tissues including Peyer's patches (PP) and the nasal-associated lymphoid tissues, which would ensure prompt activation in the event of influenza infection.Citation41 In addition, i.m. vaccination of humans with the licensed inactivated hepatitis A and B vaccines induced high levels of specific antibody responses in sera and protection against hepatitis A and B infection,Citation42-45 Moreover, a recent meta-analysis of clinical studies indicate that i.m. immunization of >10-wk-old infants with 2 full or 1/5 doses of inactivated poliovirus vaccine resulted in >80% seroconversion and is likely to protect >80% of vaccinees against poliomyelitis.Citation46
In addition to promoting robust antibody responses, i.m. immunization has been shown to induce cell-mediated immune (CMI) responses at mucosal sites. For instance, i.m. immunization of mice with a DNA vaccine co-delivered with CCL25 chemokine enhanced antigen-specific IFN-γ secretion by CD3+CD8+ and CD3+CD4+ T cells in mesenteric lymph nodes (MLNs), and conferred complete protection against a lethal i.n. influenza challenge.Citation47 Similarly, i.m. administration of retinoic acid to mice immunized with a replication-defective adenovirus vector increased both effector and memory T cell numbers in the intestinal mucosal tissue and protected mice from an intravaginal vaccinia virus challenge.Citation48 Moreover, i.m. immunization of 7-day-old pigs with an inactivated M. hyopneumoniae vaccine significantly increased the number of IL-12 and IL-10 secreting cells in the lungs and bronchial lymph nodes, and generated antigen-specific IgG, IgM and IgA antibodies in BAL fluids as well.Citation49 In Indian rhesus macaques, a plasmid DNA vaccine expressing several SIV antigens delivered by i.m. electroporation increased antigen-specific IFN-γ-secreting, but not IL-2-secreting, T cells in blood and BAL fluids, with a greater proportion of specific CD8+ T cells in BAL fluids than that in the blood.Citation50 Furthermore, a fourth i.m. immunization administered 90 weeks after the third one, rapidly boosted antigen-specific humoral and cellular responses with higher population of specific IFN-γ+ memory T cells in the BAL fluid than in the blood. On the other hand, some vaccines administered by i.m. route were less effective or inefficient in inducing mucosal immune responses.Citation51,52 For example, in the herpes simplex virus type 2 (HSV-2) vaccine trials, i.m. vaccination of subunit vaccines such as glycoprotein B in oil-in-water adjuvant and glycoprotein D in alum and 3-O-deacylated monophosphoryl lipid A, failed to protect against genital HSV-2 infection despite the good immunogenicity.Citation53,54 Moreover, i.m. vaccination of the nursing home residents (aged 60–82 years) with an inactivated commercial influenza vaccine failed to elicit IgA responses in nasal washes, although strong haemagglutination inhibition (HI) titers were detected in the sera.Citation55 It is possible that the age or sex of the vaccinees or the type of vaccine administered was a contributing factor to these observations.
Subcutaneous immunization
Subcutaneous (s.c.) route of immunization is another conventional vaccination route widely used for various human vaccines and experimental vaccines in animal models. Recent studies suggest that s.c. immunization of non-replicating vaccines could induce both systemic and mucosal antigen-specific antibody responses, and protect the vaccinated animals against infectious challenge.Citation56-58 In a macaque study, s.c. immunization with HIV gp140 with recombinant macaque major histocompatibility complex (MHC) class I and II elicited serum and mucosal (recta and vagina) antigen-specific IgG and IgA responses to both HIV gp120 and MHC class I alleles, and conferred significant reduction in the plasma viral load after a rectal challenge with simian HIV.Citation59 In addition to mucosal humoral immune responses, s.c. vaccination can potentially enhance mucosal CMI responses. In this regard, s.c. immunization of 3- to 8-week-old calves with a BRSV immunostimulating complex (BRSV-ISCOM) vaccine induced potent lymphocyte proliferation responses concomitant with high levels of IFN-γ and IL-4 production in PBMCs as well as higher antigen-specific IgA and IgG in sera, nasal passages, and BAL fluids.Citation60 More significantly, in spite of the presence of variable levels of BRSV-specific maternally derived antibodies, the immunized calves were significantly protected against an aerosol BRSV challenge with significant reduction in virus titers in the upper and lower respiratory tracts.Citation60
Hammerschmidt et al.Citation61 demonstrated that s.c. administration of retinoic acid to mice upregulated gut-homing molecules on activated CD4+ and CD8+ T cells, and triggered the generation of gut-tropic IgA+ ASCs in the skin-draining inguinal lymph nodes. Furthermore, s.c. immunization with retinoic acid plus CT or inactivated Salmonella typhimurium elicited robust antigen-specific anti-CT and anti-Salmonella mucosal immune responses in the small intestine, and protected mice from cholera-related diarrhea and oral Salmonella challenge. It is important to note that some vaccines, such as inactivated influenza H5N1 vaccine, administered by s.c. route successfully protected mice against heterosubtypic challenge with potent cross-reactive antibody responses in sera and mucosal sites (such as vagina).Citation62,63 Interestingly, although i.m. immunization of mice with the B subunits of Shiga toxin type 1 and 2as a fusion protein failed to induce any fecal antibody responses, the vaccination efficiently reduced fecal bacterial shedding after oral challenge with E. coli O157:H7.Citation64 On the other hand, s.c. immunization with Tir proteins and type III secreted proteins IpaB and IpaD from E. coli O157:H7 failed to elicit protective mucosal immunity against subsequent pathogen challenges, although strong systemic immune responses were detected.Citation65-67 By using a combined s.c. and i.m. immunization strategy, rhesus macaques vaccinated with a vaccine comprising of Chlamydia trachomatis serover F native major outer membrane protein (MOMP) with CpG-2395 and Montanide ISA 720 VG as adjuvants developed potent systemic and mucosal humoral and CMI responses with high levels of antigen-specific IgG and IgA in plasma and mucosal secretions (vaginal washes, tears, saliva and stools), as well as enhanced lymphocyte proliferation responses and IFN-γ, TNF-α and IL-6 production by PBMCs.Citation68
Intradermal immunization
Intradermal (i.d.) vaccination, developed for the original smallpox vaccine and referred as scarification at the time, was reported to induce both systemic and mucosal immune responses.Citation69,70 In pigs, i.d. immunization with a commercial inactivated M. hyopneumoniae whole-cell vaccine elicited robust M. hyopneumoniae-specific serum IgG and pulmonary IgA responses, significantly increased level of IL-10, but not IL-6, TNF-α or IFN-γ, in the BAL fluids, although the number of antigen-specific IFN-γ producing cells in PBMCs was significantly higher in the i.d. immunized pigs.Citation71 Mice intradermally immunized with a HPV DNA vaccine together with a CTB plasmid vector generated high antigen-specific IgA and IgG titers in cervical secretions and feces, and showed enhanced CTL activity and Th1 (IL-2 and IFN-γ) cytokine expression in spleen.Citation72 Interestingly, i.d. administration of a sperm-DNA vaccine to female mice elicited mainly IgG responses in sera and largely IgM and IgA responses in the vaginal wash fluid.Citation73 Pigs i.d. immunized with a DNA vaccine showed significant reduction of gross pathological lesions and bacterial shedding in urogenital tract after a vaginal C. Trachomatis challenge.Citation74 It has been recently shown that i.d. vaccination of mice with inactivated influenza virus using microneedles induced more robust serum and lung IgG responses, increased expression of IL-4 and IFN-γ in spleen and IL-12 in lung, and provided better protection against i.n. viral challenge than i.m. vaccination.Citation75 Moreover, i.d. immunization (using microneedles) of mice with IpaB and IpaD adjuvanted with double mutant E. coli heat labile toxin (dmLT) resulted in the local recruitment of APCs (macrophages, CD11c+ dendritic cells and Langerhans cells), serum IgG responses, and secretion of various cytokines from T cells. The vaccinated mice were protected against lethal pulmonary challenges with S. flexneri (70% survival) or S. sonnei (50% survival) although little mucosal immune responses(mucosal IgA or mucosal and systemic IgA-ASCs) were detected.Citation76 However, some vaccines (such as a β-galactosidase and a rotavirus DNA vaccine) administered by i.d. route failed to induce sufficient mucosal antibodies or to protect against mucosal challenge.Citation77-79 The observation of adverse local reactions caused by i.d. injection or scarification in some studies should be considered in the future applications of i.d. vaccination to protect against mucosal pathogens.Citation69
Transcutaneous immunization
Transcutaneous immunization (TCI) is an approach of delivering the vaccine through the skin layer. Since this method requires some physical/chemical means to breach the intact skin so as to deliver the antigen/adjuvant into the epidermal layer, it is discussed in this review in the context of potential to elicit mucosal immunity although it is debatable whether TCI is truely a systemic immunization or not. Vaccination by TCI would be more acceptable by the patients as opposed to by traditional i.m., i.d., or s.c. methods, and TCI has been demonstrated to induce robust systemic and mucosal immune responses that protect the host against mucosal infection.Citation80-84 For example, CT- or CpG-adjuvanted chlamydial MOMP applied to the shaved skin on the back region of mice enhanced MOMP-specific IgG and IgA responses in sera, vaginal and uterine lavage fluids, and increased IFN-γ (but not IL-4) mRNA expression in the mononuclear cells from the reproductive tract-draining caudal and lumbar lymph nodes, and protected the mice against an intravaginal C. muridarum challenge.Citation85 Moreover, an adjuvant-free, powdered, inactivated influenza vaccine placed on the shaved abdominal skin of mice elicited specific IgG and IgA responses in serum and at several mucosal sites (e.g, small intestine, saliva, vagina, and nasal passages), and effectively increased the survival rate of mice against an i.n. challenge with the influenza virus.Citation86 Furthermore, based on the presence of antigen-specific IgA secreting ASCs in lamina propria of small intestine and the secretion of specific IgA from in vitro cultured tracheal and small intestinal samples, it was suggested that the antigen-specific antibodies were locally produced at the relevant mucosal sites, rather than diffusing from sera.Citation86 Specific IgG and IgA to both tetanus toxoid (TT) and CT were detected in sera, saliva, vaginal lavages and fecal extracts of mice transcutaneously immunized with TT admixed with CT, with comparatively higher titers in sera, saliva and vaginal lavage as compared to in fecal pellets.Citation87 In another study, it was shown that TCI with CT or its B subunit (CTB)elicited more potent anti-CTB serum IgG responses and comparable specific IgA responses in serum, feces and bile, when compared to oral immunization with live vaccine strain of Vibrio cholerae expressing CTB.Citation88
The immune responses elicited by s.c., i.d. and TCI immunization of an HIV nanoparticle vaccine were compared in mice.Citation89 The population of antigen-specific cytokine (IL-2 or IFN-γ or TNF-α) producing CD4+ T cells in the spleen from i.d. or s.c. immunized mice were significantly higher than those from TCI mice. However, the population of poly functional T cells which produce all 3 cytokines (IL-2, IFN-γ and TNF-α) was highest in TCI group, and lowest in i.d. immunized group. Significantly increased antigen-specific CD8+ T cells were found in blood after i.d. and TCI immunization while absent after s.c. immunization, consistent with higher population of CD3+CD8+ T cells in vaginal mucosa of TCI and i.d. vaccination when compared to s.c. vaccination. These results suggest that TCI and i.d. immunization redirected homing of antigen-specific effector/memory CD8+ T cells to the vaginal mucosa. Interestingly, TCI of mice at different anatomic skin sites (back, abdomen, and ear) induce different magnitude of systemic (spleen) and mucosal (PPs) CTL responses, with the strongest CTL responses in both mucosal and systemic sites elicited by TCI on the back.Citation87 In contrast, TCI immunization of mice with a synthetic hexasaccharide-protein conjugate vaccine failed to induce detectable mucosal immune responses or provide any protection against oral V. cholera challenges despite the presence of robust serum IgG and IgA responses.Citation90 In a double-blind, placebo-controlled clinical trial wherein 59 randomized adults were transcutaneously immunized with either the LT from enterotoxigenic E. coli (ETEC) or placebo, higher serum IgG and IgA as well as fecal IgA responses were detected in vaccinees compared to the placebo controls.Citation91 However, the vaccination only mitigated, but did not prevent, the infection after an oral challenge with a virulent ETEC strain.
Potential mechanisms of systemic vaccination-induced mucosal immunity
Although systemic immunization (s.c., i.m., i.d., i.p. and TCI) can induce mucosal immune responses under certain antigen and adjuvant combinations, the mechanism of this induction remains poorly understood, So far, several mechanisms have been proposed to explain the induction of mucosal antibodies after systemic immunizationCitation (Figure 1). Based on the relatively low number of APCs in some of the systemic tissues, it was hypothesized that an antigen first diffuses from a s.c., i.m. or i.p. immunization site to the regional draining lymph nodes, and from there is taken up by the local APCs (such as DCs, B cells, and macrophages). These APC cells then migrate to the mucosa-associated lymphoid tissue (MALT), such as PPs and nasopharynx-associated lymphoid tissue (NALT), where they activate CD4+ T cells and B cells.Citation92,93 On the other hand, antigen administered by i.d. or TCI can activate APCs, mainly the Langerhans cells and DCs, in the epidermis and dermis of the skin. These cells migrate to MALT and present the antigen to naïve T cells for the generation of antigen-specific T cells, including Th1, Th2, Th17, and cytotoxic T cells.Citation94,95 Alternatively, soluble or phagocytosed antigens may migrate to the MALT directly.Citation3
The immunostimulatory molecules (such as those provided by adjuvants) in the vaccines increase the local recruitment, antigen processing and presentation efficiency of the APCs at the site of vaccination, promote the proliferation of antigen-specific T cells and antibody-secreting B cells, which then migrate to the distant effector sites, such as lamina propria (LP) of the gut and salivary glandsCitation96,95,97 Under the influence of the specialized mucosal homing and imprinting mechanisms, antibody-secreting B cells finally differentiate into plasma cells and produce specific antibodies whereas a subpopulation of the antigen-activated T cells expressed different adhesion molecules, depending on the anatomic location of the lymph nodes and differentiated as tissue-resident memory T cells (TRM). Recent studies indicate that these TRM cells persist in the tissue long after vaccination or the clearance of the infection for maximal and efficient control of locally invaded pathogens.Citation98,99,100 In addition, mucosal antibody responses can also be induced through exudation, transcytosis, or production by the local plasma cells.
Conclusion
Based on the published literature to date, it is well recognized that the protective efficacy of a vaccine delivered by varying routes of immunization is affected by the choice of the antigen, the antigen carrier/delivery vehicle, and the adjuvant, among many other factors. It is also generally acknowledged that immunization via mucosal routes, using subunit antigens, can elicit robust mucosal (and systemic) immune responses that accord protection against specific mucosal pathogens. Increasing evidence from experimental vaccine and animal model studies suggest that under some circumstances (antigen, adjuvant, delivery vehicle) systemic routes of immunization have the potential to induce immune responses in both the systemic and multiple mucosal compartmentsCitation (Tables 1–3). However, it is currently unknown as to under what specific circumstances would a systemic immunization elicit a protective mucosal immune response in an animal model, or if the observations in animal models would be translated to human subjects? However, it appears that i.p. immunization generally induces non-protective mucosal (particularly the gastrointestinal) IgA responses while i.m. immunization with DNA-based vaccines is likely to induce a protective mucosal immune response including CMI. In addition, TCI appears to be another encouraging route of systemic immunization to induce protective mucosal immunity.
The results from many studies on the potential of systemic immunization to elicit protective mucosal immunity in animal models are often difficult to interpret because there is the failure to evaluate whether the protection (if seen) was due to the mucosal immune responses elicited or could have been as a result of strong systemic responses per se? Part of the challenge may be that there are no appropriate animal models of disease, wherein it is clearly known that only a strong mucosal immune response would protect the vaccinated host against the specific, mucosal pathogen challenge. Moreover, observations on the presence of mucosal immune responses (such as serum and salivary IgA) in human subjects that have been immunized with a systemic vaccine were often complicated by the prior exposure to the antigens or pathogens. In spite of these limitations, the ongoing studies to date do indicate that there is the potential to develop systemic vaccination strategies that may offer an alternative approach to mucosal immunization for the elicitation of both mucosal and systemic immune responses.
Table 1. Levels of antigen-specific IgA antibody responses at systemic and mucosal sites.
Table 2. Levels of antigen-specific IgG antibody responses at systemic and mucosal sites.
Table 3. Levels of antigen-specific IgM antibody responses at systemic and mucosal sites.
Figure 1. Potential mechanisms of systemic vaccination-induced mucosal antibody responses. Intradermal (i.d.) or transcutaneous (TC) immunization activates Langerhans cells and dermal dendritic cells in the epidermis and dermis of skin, which then migrate to the mucosa-associated lymphoid tissue (MALT) where they present the antigen to CD4+ T cells and B cells. An antigen delivered by i.m. or s.c. route mainly diffuses to the draining peripheral lymph nodes (DPLN) where it activates APCs, such as B cells, dendritic cells and macrophages. Mucosal antibody responses are triggered when they reach to the MALT and present the antigen to CD4+ T cells and B cells. A free antigen may migrate to MALT directly.
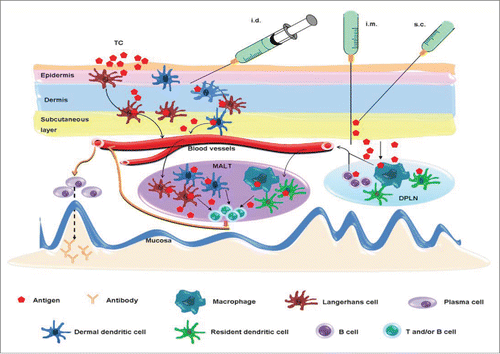
Disclosure of potential conflicts of interest
No potential conflicts of interest were disclosed.
Funding
Fei Su is a visiting researcher from Zhejiang University, China through a scholarship from Chinese Scholarship Council under a Memorandum of Understanding between NRC and Chinese Ministry of Education. The mucosal adjuvant and vaccine research in the authors' laboratory was partially supported by the NRC Vaccine Program. The views expressed in this paper are the sole responsibility of the authors and do not necessarily represent the official views of the NRC or the Zhejiang University.
References
- Brandtzaeg P, Pabst R. Let's go mucosal: communication on slippery ground. Trends Immunol 2004; 25:570-7; PMID:15489184; http://dx.doi.org/10.1016/j.it.2004.09.005
- Czerkinsky C, Holmgren J. Mucosal delivery routes for optimal immunization: targeting immunity to the right tissues. Curr Top Microbiol Immunol 2012; 354:1-18; PMID:21053117
- Bouvet JP, Decroix N, Pamonsinlapatham P. Stimulation of local antibody production: parenteral or mucosal vaccination? Trends Immunol 2002; 23:209-13; PMID:11923116; http://dx.doi.org/10.1016/S1471-4906(02)02186-5
- Holmgren J, Czerkinsky C. Mucosal immunity and vaccines. Nat Med 2005; 11:S45-53; PMID:15812489; http://dx.doi.org/10.1038/nm1213
- Parr EL, Parr MB. Immunoglobulin G is the main protective antibody in mouse vaginal secretions after vaginal immunization with attenuated herpes simplex virus type 2. J Virol 1997; 71:8109-15; PMID:9343160
- Brandtzaeg P. Mucosal immunity: induction, dissemination, and effector functions. Scand J Immunol 2009; 70:505-15; PMID:19906191; http://dx.doi.org/10.1111/j.1365-3083.2009.02319.x
- Brandtzaeg P. Induction of secretory immunity and memory at mucosal surfaces. Vaccine 2007; 25:5467-84; PMID:17227687; http://dx.doi.org/10.1016/j.vaccine.2006.12.001
- Berneman A, Belec L, Fischetti VA, Bouvet JP. The specificity patterns of human immunoglobulin G antibodies in serum differ from those in autologous secretions. Infect Immun 1998; 66:4163-8; PMID:9712763
- Bouvet JP, Fischetti VA. Diversity of antibody-mediated immunity at the mucosal barrier. Infect Immun 1999; 67:2687-91; PMID:10338470
- Mbawuike IN, Pacheco S, Acuna CL, Switzer KC, Zhang Y, Harriman GR. Mucosal immunity to influenza without IgA: an IgA knockout mouse model. J Immunol 1999; 162:2530-7; PMID:10072492
- Lambert PH, Laurent PE. Intradermal vaccine delivery: will new delivery systems transform vaccine administration? Vaccine 2008; 26:3197-208; PMID:18486285; http://dx.doi.org/10.1016/j.vaccine.2008.03.095
- Nochi T, Yuki Y, Takahashi H, Sawada S, Mejima M, Kohda T, Harada N, Kong IG, Sato A, Kataoka N, et al. Nanogel antigenic protein-delivery system for adjuvant-free intranasal vaccines. Nat Mater 2010; 9:572-8; PMID:20562880; http://dx.doi.org/10.1038/nmat2784
- Mutsch M, Zhou W, Rhodes P, Bopp M, Chen RT, Linder T, Spyr C, Steffen R. Use of the inactivated intranasal influenza vaccine and the risk of Bell's palsy in Switzerland. N Engl J Med 2004; 350:896-903; PMID:14985487; http://dx.doi.org/10.1056/NEJMoa030595
- Yuki Y, Kiyono H. New generation of mucosal adjuvants for the induction of protective immunity. Rev Med Virol 2003; 13:293-310; PMID:12931340; http://dx.doi.org/10.1002/rmv.398
- Esposito S, Montinaro V, Groppali E, Tenconi R, Semino M, Principi N. Live attenuated intranasal influenza vaccine. Hum Vaccin Immunother 2012; 8:76-80; PMID:22251995; http://dx.doi.org/10.4161/hv.8.1.18809
- Ivanov AP, Dragunsky EM, Chumakov KM. 1,25-dihydroxyvitamin d3 enhances systemic and mucosal immune responses to inactivated poliovirus vaccine in mice. J Infect Dis 2006; 193:598-600; PMID:16425140; http://dx.doi.org/10.1086/499970
- Sheldrake RF, Romalis LF, Saunders MM. Serum and mucosal antibody responses against Mycoplasma hyopneumoniae following intraperitoneal vaccination and challenge of pigs with M hyopneumoniae. Res Vet Sci 1993; 55:371-6; PMID:8284503; http://dx.doi.org/10.1016/0034-5288(93)90110-2
- Moreno-Fierros L, Garcia N, Gutierrez R, Lopez-Revilla R, Vazquez-Padron RI. Intranasal, rectal and intraperitoneal immunization with protoxin Cry1Ac from Bacillus thuringiensis induces compartmentalized serum, intestinal, vaginal and pulmonary immune responses in Balb/c mice. Microbes Infect 2000; 2:885-90; PMID:10962271; http://dx.doi.org/10.1016/S1286-4579(00)00398-1
- Rojas-Hernandez S, Rodriguez-Monroy MA, Lopez-Revilla R, Resendiz-Albor AA, Moreno-Fierros L. Intranasal coadministration of the Cry1Ac protoxin with amoebal lysates increases protection against Naegleria fowleri meningoencephalitis. Infect Immun 2004; 72:4368-75; PMID:15271892; http://dx.doi.org/10.1128/IAI.72.8.4368-4375.2004
- Gai WW, Zhang Y, Zhou DH, Chen YQ, Yang JY, Yan HM. PIKA provides an adjuvant effect to induce strong mucosal and systemic humoral immunity against SARS-CoV. Virol Sin 2011; 26:81-94; PMID:21468931; http://dx.doi.org/10.1007/s12250-011-3183-z
- Rodriguez A, Troye-Blomberg M, Lindroth K, Ivanyi J, Singh M, Fernandez C. B- and T-cell responses to the mycobacterium surface antigen PstS-1 in the respiratory tract and adjacent tissues. Role of adjuvants and routes of immunization. Vaccine 2003; 21:458-67; PMID:12531644; http://dx.doi.org/10.1016/S0264-410X(02)00478-4
- Hodge LM, Simecka JW. Role of upper and lower respiratory tract immunity in resistance to Mycoplasma respiratory disease. J Infect Dis 2002; 186:290-4; PMID:12134269; http://dx.doi.org/10.1086/341280
- Lue C, van den Wall BA, Prince SJ, Julian BA, Tseng ML, Radl J, Elson CO, Mestecky J. Intraperitoneal immunization of human subjects with tetanus toxoid induces specific antibody-secreting cells in the peritoneal cavity and in the circulation, but fails to elicit a secretory IgA response. Clin Exp Immunol 1994; 96:356-63; PMID:8187345; http://dx.doi.org/10.1111/j.1365-2249.1994.tb06567.x
- Rodriguez A, Rottenberg M, Tjarnlund A, Fernandez C. Immunoglobulin A and CD8 T-cell mucosal immune defenses protect against intranasal infection with Chlamydia pneumoniae. Scand J Immunol 2006; 63:177-83; PMID:16499570; http://dx.doi.org/10.1111/j.1365-3083.2006.01725.x
- Heurtault B, Gentine P, Thomann JS, Baehr C, Frisch B, Pons F. Design of a liposomal candidate vaccine against Pseudomonas aeruginosa and its evaluation in triggering systemic and lung mucosal immunity. Pharm Res 2009; 26:276-85; PMID:18781377; http://dx.doi.org/10.1007/s11095-008-9724-y
- Lu B, Huang Y, Huang L, Li B, Zheng Z, Chen Z, Chen J, Hu Q, Wang H. Effect of mucosal and systemic immunization with virus-like particles of severe acute respiratory syndrome coronavirus in mice. Immunology 2010; 130:254-61; PMID:20406307; http://dx.doi.org/10.1111/j.1365-2567.2010.03231.x
- Shuttleworth G, Eckery DC, Awram P. Oral and intraperitoneal immunization with rotavirus 2/6 virus-like particles stimulates a systemic and mucosal immune response in mice. Arch Virol 2005; 150:341-9; PMID:15578236; http://dx.doi.org/10.1007/s00705-004-0447-z
- Buonaguro L, Visciano ML, Tornesello ML, Tagliamonte M, Biryahwaho B, Buonaguro FM. Induction of systemic and mucosal cross-clade neutralizing antibodies in BALB/c mice immunized with human immunodeficiency virus type 1 clade A virus-like particles administered by different routes of inoculation. J Virol 2005; 79:7059-67s; PMID:15890945; http://dx.doi.org/10.1128/JVI.79.11.7059-7067.2005
- Nicolas JF, Guy B. Intradermal, epidermal and transcutaneous vaccination: from immunology to clinical practice. Expert Rev Vaccines 2008; 7:1201-14; PMID:18844594; http://dx.doi.org/10.1586/14760584.7.8.1201
- Jones T, Adamovicz JJ, Cyr SL, Bolt CR, Bellerose N, Pitt LM, Lowell GH, Burt DS. Intranasal Protollin/F1-V vaccine elicits respiratory and serum antibody responses and protects mice against lethal aerosolized plague infection. Vaccine 2006; 24:1625-32; PMID:16243411; http://dx.doi.org/10.1016/j.vaccine.2005.09.052
- Santillan DA, Andracki ME, Hunter SK. Protective immunization in mice against group B streptococci using encapsulated C5a peptidase. Am J Obstet Gynecol 2008; 198:111-4.
- EL-Malky MA, Al-Harthi SA, Mohamed RT, EL BM, Saudy NS. Vaccination with Toxoplasma lysate antigen and CpG oligodeoxynucleotides: comparison of immune responses in intranasal versus intramuscular administrations. Parasitol Res 2014; 113:2277-84; PMID:24728558; http://dx.doi.org/10.1007/s00436-014-3882-0
- Vilte DA, Larzabal M, Garbaccio S, Gammella M, Rabinovitz BC, Elizondo AM, Cantet RJ, Delgado F, Meikle V, Cataldi A, et al. Reduced faecal shedding of Escherichia coli O157:H7 in cattle following systemic vaccination with gamma-intimin C(2)(8)(0) and EspB proteins. Vaccine 2011; 29:3962-8; PMID:21477674; http://dx.doi.org/10.1016/j.vaccine.2011.03.079
- Haan L, Verweij WR, Holtrop M, Brands R, van Scharrenburg GJ, Palache AM, Agsteribbe E, Wilschut J. Nasal or intramuscular immunization of mice with influenza subunit antigen and the B subunit of Escherichia coli heat-labile toxin induces IgA- or IgG-mediated protective mucosal immunity. Vaccine 2001; 19:2898-907; PMID:11282201; http://dx.doi.org/10.1016/S0264-410X(00)00556-9
- Jia R, Guo JH, Fan MW, Bian Z, Chen Z, Fan B, Yu F, Xu QA. Immunogenicity of CTLA4 fusion anti-caries DNA vaccine in rabbits and monkeys. Vaccine 2006; 24:5192-200; PMID:16675075; http://dx.doi.org/10.1016/j.vaccine.2006.03.090
- Taylor G, Bruce C, Barbet AF, Wyld SG, Thomas LH. DNA vaccination against respiratory syncytial virus in young calves. Vaccine 2005; 23:1242-50; PMID:15652666; http://dx.doi.org/10.1016/j.vaccine.2004.09.005
- Yang K, Wang S, Chang KO, Lu S, Saif LJ, Greenberg HB, Brinker JP, Herrmann JE. Immune responses and protection obtained with rotavirus VP6 DNA vaccines given by intramuscular injection. Vaccine 2001; 19:3285-91; PMID:11312027; http://dx.doi.org/10.1016/S0264-410X(00)00543-0
- Perrone LA, Ahmad A, Veguilla V, Lu X, Smith G, Katz JM, Pushko P, Tumpey TM. Intranasal vaccination with 1918 influenza virus-like particles protects mice and ferrets from lethal 1918 and H5N1 influenza virus challenge. J Virol 2009; 83:5726-34; PMID:19321609; http://dx.doi.org/10.1128/JVI.00207-09
- Huo Z, Bissett SL, Giemza R, Beddows S, Oeser C, Lewis DJ. Systemic and mucosal immune responses to sublingual or intramuscular human papilloma virus antigens in healthy female volunteers. Plos One 2012; 7:e33736; PMID:22438987; http://dx.doi.org/10.1371/journal.pone.0033736
- Nardelli-Haefliger D, Wirthner D, Schiller JT, Lowy DR, Hildesheim A, Ponci F, De Grandi P. Specific antibody levels at the cervix during the menstrual cycle of women vaccinated with human papillomavirus 16 virus-like particles. J Natl Cancer Inst 2003; 95:1128-37; PMID:12902442; http://dx.doi.org/10.1093/jnci/djg018
- Joo HM, He Y, Sundararajan A, Huan L, Sangster MY. Quantitative analysis of influenza virus-specific B cell memory generated by different routes of inactivated virus vaccination. Vaccine 2010; 28:2186-94; PMID:20056191; http://dx.doi.org/10.1016/j.vaccine.2009.12.058
- Van Herck K, Crasta PD, Messier M, Hardt K, Van Damme P. Seventeen-year antibody persistence in adults primed with two doses of an inactivated hepatitis A vaccine. Hum Vaccin Immunother 2012; 8:323-7; PMID:22327499; http://dx.doi.org/10.4161/hv.18617
- Andre F, Van Damme P, Safary A, Banatvala J. Inactivated hepatitis A vaccine: immunogenicity, efficacy, safety and review of official recommendations for use. Expert Rev Vaccines 2002; 1:9-23; PMID:12908508; http://dx.doi.org/10.1586/14760584.1.1.9
- Lee WM. Hepatitis B virus infection. N Engl J Med 1997; 337:1733-45; PMID:9392700; http://dx.doi.org/10.1056/NEJM199712113372406
- Michel ML, Tiollais P. Hepatitis B vaccines: protective efficacy and therapeutic potential. Pathol Biol (Paris) 2010; 58:288-95; PMID:20382485; http://dx.doi.org/10.1016/j.patbio.2010.01.006
- Grassly NC. Immunogenicity and effectiveness of routine immunization with 1 or 2 doses of inactivated poliovirus vaccine: systematic review and meta-analysis. J Infect Dis 2014; 210 Suppl 1:S439-46; PMID:24634499; http://dx.doi.org/10.1093/infdis/jit601
- Kathuria N, Kraynyak KA, Carnathan D, Betts M, Weiner DB, Kutzler MA. Generation of antigen-specific immunity following systemic immunization with DNA vaccine encoding CCL25 chemokine immunoadjuvant. Hum Vaccin Immunother 2012; 8:1607-19; PMID:23151454; http://dx.doi.org/10.4161/hv.22574
- Tan X, Sande JL, Pufnock JS, Blattman JN, Greenberg PD. Retinoic acid as a vaccine adjuvant enhances CD8+ T cell response and mucosal protection from viral challenge. J Virol 2011; 85:8316-27; PMID:21653670; http://dx.doi.org/10.1128/JVI.00781-11
- Marchioro SB, Maes D, Flahou B, Pasmans F, Del PSR, Vranckx K, Melkebeek V, Cox E, Wuyts N, Haesebrouck F. Local and systemic immune responses in pigs intramuscularly injected with an inactivated Mycoplasma hyopneumoniae vaccine. Vaccine 2013; 31:1305-11; PMID:23306368; http://dx.doi.org/10.1016/j.vaccine.2012.12.068
- Patel V, Valentin A, Kulkarni V, Rosati M, Bergamaschi C, Jalah R, Alicea C, Minang JT, Trivett MT, Ohlen C, et al. Long-lasting humoral and cellular immune responses and mucosal dissemination after intramuscular DNA immunization. Vaccine 2010; 28:4827-36; PMID:20451642; http://dx.doi.org/10.1016/j.vaccine.2010.04.064
- Schreckenberger C, Sethupathi P, Kanjanahaluethai A, Muller M, Zhou J, Gissmann L, Qiao L. Induction of an HPV 6bL1-specific mucosal IgA response by DNA immunization. Vaccine 2000; 19:227-33; PMID:10930677; http://dx.doi.org/10.1016/S0264-410X(00)00173-0
- Hu MC, Jones T, Kenney RT, Barnard DL, Burt DS, Lowell GH. Intranasal Protollin-formulated recombinant SARS S-protein elicits respiratory and serum neutralizing antibodies and protection in mice. Vaccine 2007; 25:6334-40; PMID:17640780; http://dx.doi.org/10.1016/j.vaccine.2007.06.017
- Corey L, Langenberg AG, Ashley R, Sekulovich RE, Izu AE, Douglas JJ, Handsfield HH, Warren T, Marr L, Tyring S, et al. Recombinant glycoprotein vaccine for the prevention of genital HSV-2 infection: two randomized controlled trials. Chiron HSV Vaccine Study Group. JAMA 1999; 282:331-40; PMID:10432030; http://dx.doi.org/10.1001/jama.282.4.331
- Belshe RB, Leone PA, Bernstein DI, Wald A, Levin MJ, Stapleton JT, Gorfinkel I, Morrow RL, Ewell MG, Stokes-Riner A, et al. Efficacy results of a trial of a herpes simplex vaccine. N Engl J Med 2012; 366:34-43; PMID:22216840; http://dx.doi.org/10.1056/NEJMoa1103151
- Muszkat M, Greenbaum E, Ben-Yehuda A, Oster M, Yeu'L E, Heimann S, Levy R, Friedman G, Zakay-Rones Z. Local and systemic immune response in nursing-home elderly following intranasal or intramuscular immunization with inactivated influenza vaccine. Vaccine 2003; 21:1180-6; PMID:12559796; http://dx.doi.org/10.1016/S0264-410X(02)00481-4
- Lima FA, Miyaji EN, Quintilio W, Raw I, Ho PL, Oliveira ML. Pneumococcal Surface Protein A does not affect the immune responses to a combined diphtheria tetanus and pertussis vaccine in mice. Vaccine 2013; 31:2465-70; PMID:23541622; http://dx.doi.org/10.1016/j.vaccine.2013.03.026
- Rioux G, Mathieu C, Russell A, Bolduc M, Laliberte-Gagne ME, Savard P, Leclerc D. PapMV nanoparticles improve mucosal immune responses to the trivalent inactivated flu vaccine. J Nanobiotechnology 2014; 12:19; PMID:24885884; http://dx.doi.org/10.1186/1477-3155-12-19
- Jang SI, Lillehoj HS, Lee SH, Lee KW, Lillehoj EP, Bertrand F, Dupuis L, Deville S. Montanide IMS 1313 N VG PR nanoparticle adjuvant enhances antigen-specific immune responses to profilin following mucosal vaccination against Eimeria acervulina. Vet Parasitol 2011; 182:163-70; PMID:21700391; http://dx.doi.org/10.1016/j.vetpar.2011.05.019
- Yang GB, Wang Y, Babaahmady K, Scholler J, Rahman D, Bunnik E, Spallek R, Zong CM, Duan JZ, Qin C, et al. Immunization with recombinant macaque major histocompatibility complex class I and II and human immunodeficiency virus gp140 inhibits simian-human immunodeficiency virus infection in macaques. J Gen Virol 2012; 93:1506-18; PMID:22492918; http://dx.doi.org/10.1099/vir.0.041061-0
- Hagglund S, Hu K, Vargmar K, Pore L, Olofson AS, Blodorn K, Anderson J, Ahooghalandari P, Pringle J, Taylor G, et al. Bovine respiratory syncytial virus ISCOMs-Immunity, protection and safety in young conventional calves. Vaccine 2011; 29:8719-30; PMID:21864616; http://dx.doi.org/10.1016/j.vaccine.2011.07.146
- Hammerschmidt SI, Friedrichsen M, Boelter J, Lyszkiewicz M, Kremmer E, Pabst O, Forster R. Retinoic acid induces homing of protective T and B cells to the gut after subcutaneous immunization in mice. J Clin Invest 2011; 121:3051-61; PMID:21737878; http://dx.doi.org/10.1172/JCI44262
- Budimir N, de Haan A, Meijerhof T, Gostick E, Price DA, Huckriede A, Wilschut J. Heterosubtypic cross-protection induced by whole inactivated influenza virus vaccine in mice: influence of the route of vaccine administration. Influenza Other Respir Viruses 2013; 7:1202-9; PMID:24102979; http://dx.doi.org/10.1111/irv.12142
- Wolf AI, Mozdzanowska K, Williams KL, Singer D, Richter M, Hoffmann R, Caton AJ, Otvos L, Erikson J. Vaccination with M2e-based multiple antigenic peptides: characterization of the B cell response and protection efficacy in inbred and outbred mice. Plos One 2011; 6:e28445;
- Zhang XH, He KW, Zhang SX, Lu WC, Zhao PD, Luan XT, Ye Q, Wen LB, Li B, Guo RL, et al. Subcutaneous and intranasal immunization with Stx2B-Tir-Stx1B-Zot reduces colonization and shedding of Escherichia coli O157:H7 in mice. Vaccine 2011; 29:3923-9; PMID:21338683; http://dx.doi.org/10.1016/j.vaccine.2011.02.007
- Babiuk S, Asper DJ, Rogan D, Mutwiri GK, Potter AA. Subcutaneous and intranasal immunization with type III secreted proteins can prevent colonization and shedding of Escherichia coli O157:H7 in mice. Microb Pathog 2008; 45:7-11; PMID:18487034; http://dx.doi.org/10.1016/j.micpath.2008.01.005
- Fan HY, Wang L, Luo J, Long BG. Protection against Escherichia coli O157:H7 challenge by immunization of mice with purified Tir proteins. Mol Biol Rep 2012; 39:989-97; PMID:21567195; http://dx.doi.org/10.1007/s11033-011-0824-0
- Herve PL, Raliou M, Bourdieu C, Dubuquoy C, Petit-Camurdan A, Bertho N, Eleouet JF, Chevalier C, Riffault S. A novel subnucleocapsid nanoplatform for mucosal vaccination against influenza virus that targets the ectodomain of matrix protein 2. J Virol 2014; 88:325-38; PMID:24155388; http://dx.doi.org/10.1128/JVI.01141-13
- Cheng C, Pal S, Bettahi I, Oxford KL, Barry PA, de la Maza LM. Immunogenicity of a vaccine formulated with the Chlamydia trachomatis serovar F, native major outer membrane protein in a nonhuman primate model. Vaccine 2011; 29:3456-64; PMID:21376796; http://dx.doi.org/10.1016/j.vaccine.2011.02.057
- Krejci J, Nechvatalova K, Kudlackova H, Leva L, Bernardy J, Toman M, Faldyna M. Effects of adjuvants on the immune response of pigs after intradermal administration of antigen. Res Vet Sci 2013; 94:73-6; PMID:22929313; http://dx.doi.org/10.1016/j.rvsc.2012.07.021
- Locher CP, Witt SA, Ashlock BM, Levy JA. Evaluation of genetic immunization adjuvants to improve the effectiveness of a human immunodeficiency virus type 2 (HIV-2) envelope DNA vaccine. DNA Cell Biol 2004; 23:107-10; PMID:15000750; http://dx.doi.org/10.1089/104454904322759911
- Martelli P, Saleri R, Cavalli V, De Angelis E, Ferrari L, Benetti M, Ferrarini G, Merialdi G, Borghetti P. Systemic and local immune response in pigs intradermally and intramuscularly injected with inactivated Mycoplasma hyopneumoniae vaccines. Vet Microbiol 2014; 168:357-64; PMID:24332702; http://dx.doi.org/10.1016/j.vetmic.2013.11.025
- Sanchez AE, Aquino G, Ostoa-Saloma P, Laclette JP, Rocha-Zavaleta L. Cholera toxin B-subunit gene enhances mucosal immunoglobulin A, Th1-type, and CD8+ cytotoxic responses when coadministered intradermally with a DNA vaccine. Clin Diagn Lab Immunol 2004; 11:711-9; PMID:15242946
- Naz RK. Effect of sperm DNA vaccine on fertility of female mice. Mol Reprod Dev 2006; 73:918-28; PMID:16596628; http://dx.doi.org/10.1002/mrd.20487
- Schautteet K, De Clercq E, Jonsson Y, Lagae S, Chiers K, Cox E, Vanrompay D. Protection of pigs against genital Chlamydia trachomatis challenge by parenteral or mucosal DNA immunization. Vaccine 2012; 30:2869-81; PMID:22387629; http://dx.doi.org/10.1016/j.vaccine.2012.02.044
- Sullivan SP, Koutsonanos DG, Del PMM, Lee JW, Zarnitsyn V, Choi SO, Murthy N, Compans RW, Skountzou I, Prausnitz MR. Dissolving polymer microneedle patches for influenza vaccination. Nat Med 2010; 16:915-20; PMID:20639891; http://dx.doi.org/10.1038/nm.2182
- Heine SJ, Diaz-McNair J, Andar AU, Drachenberg CB, van de Verg L, Walker R, Picking WL, Pasetti MF. Intradermal delivery of Shigella IpaB and IpaD type III secretion proteins: kinetics of cell recruitment and antigen uptake, mucosal and systemic immunity, and protection across serotypes. J Immunol 2014; 192:1630-40; PMID:24453241; http://dx.doi.org/10.4049/jimmunol.1302743
- Horner AA, Ronaghy A, Cheng PM, Nguyen MD, Cho HJ, Broide D, Raz E. Immunostimulatory DNA is a potent mucosal adjuvant. Cell Immunol 1998; 190:77-82; PMID:9826449; http://dx.doi.org/10.1006/cimm.1998.1400
- Choi AH, Knowlton DR, McNeal MM, Ward RL. Particle bombardment-mediated DNA vaccination with rotavirus VP6 induces high levels of serum rotavirus IgG but fails to protect mice against challenge. Virology 1997; 232:129-38; PMID:9185596; http://dx.doi.org/10.1006/viro.1997.8552
- Bal SM, Slutter B, Verheul R, Bouwstra JA, Jiskoot W. Adjuvanted, antigen loaded N-trimethyl chitosan nanoparticles for nasal and intradermal vaccination: adjuvant- and site-dependent immunogenicity in mice. Eur J Pharm Sci 2012; 45:475-81; PMID:22009113; http://dx.doi.org/10.1016/j.ejps.2011.10.003
- Glenn GM, Scharton-Kersten T, Vassell R, Mallett CP, Hale TL, Alving CR. Transcutaneous immunization with cholera toxin protects mice against lethal mucosal toxin challenge. J Immunol 1998; 161:3211-4; PMID:9759833
- Hickey DK, Aldwell FE, Tan ZY, Bao S, Beagley KW. Transcutaneous immunization with novel lipid-based adjuvants induces protection against gastric Helicobacter pylori infection. Vaccine 2009; 27:6983-90; PMID:19800441; http://dx.doi.org/10.1016/j.vaccine.2009.09.078
- Heckert RA, Elankumaran S, Oshop GL, Vakharia VN. A novel transcutaneous plasmid-dimethylsulfoxide delivery technique for avian nucleic acid immunization. Vet Immunol Immunopathol 2002; 89:67-81; PMID:12208052; http://dx.doi.org/10.1016/S0165-2427(02)00186-1
- Martin MP, Seth S, Koutsonanos DG, Jacob J, Compans RW, Skountzou I. Adjuvanted influenza vaccine administered intradermally elicits robust long-term immune responses that confer protection from lethal challenge. Plos One 2010; 5:e10897; PMID:20531947; http://dx.doi.org/10.1371/journal.pone.0010897
- Rollenhagen JE, Kalsy A, Cerda F, John M, Harris JB, Larocque RC, Qadri F, Calderwood SB, Taylor RK, Ryan ET. Transcutaneous immunization with toxin-coregulated pilin A induces protective immunity against Vibrio cholerae O1 El Tor challenge in mice. Infect Immun 2006; 74:5834-9; PMID:16988262; http://dx.doi.org/10.1128/IAI.00438-06
- Berry LJ, Hickey DK, Skelding KA, Bao S, Rendina AM, Hansbro PM, Gockel CM, Beagley KW. Transcutaneous immunization with combined cholera toxin and CpG adjuvant protects against Chlamydia muridarum genital tract infection. Infect Immun 2004; 72:1019-28; PMID:14742549; http://dx.doi.org/10.1128/IAI.72.2.1019-1028.2004
- Chen D, Periwal SB, Larrivee K, Zuleger C, Erickson CA, Endres RL, Payne LG. Serum and mucosal immune responses to an inactivated influenza virus vaccine induced by epidermal powder immunization. J Virol 2001; 75:7956-65; PMID:11483740; http://dx.doi.org/10.1128/JVI.75.17.7956-7965.2001
- Gockel CM, Bao S, Beagley KW. Transcutaneous immunization induces mucosal and systemic immunity: a potent method for targeting immunity to the female reproductive tract. Mol Immunol 2000; 37:537-44; PMID:11163403; http://dx.doi.org/10.1016/S0161-5890(00)00074-2
- John M, Bridges EA, Miller AO, Calderwood SB, Ryan ET. Comparison of mucosal and systemic humoral immune responses after transcutaneous and oral immunization strategies. Vaccine 2002; 20:2720-6; PMID:12034098; http://dx.doi.org/10.1016/S0264-410X(02)00208-6
- Liard C, Munier S, Arias M, Joulin-Giet A, Bonduelle O, Duffy D, Shattock RJ, Verrier B, Combadiere B. Targeting of HIV-p24 particle-based vaccine into differential skin layers induces distinct arms of the immune responses. Vaccine 2011; 29:6379-91; PMID:21554912; http://dx.doi.org/10.1016/j.vaccine.2011.04.080
- Rollenhagen JE, Kalsy A, Saksena R, Sheikh A, Alam MM, Qadri F, Calderwood SB, Kovac P, Ryan ET. Transcutaneous immunization with a synthetic hexasaccharide-protein conjugate induces anti-Vibrio cholerae lipopolysaccharide responses in mice. Vaccine 2009; 27:4917-22; PMID:19563890; http://dx.doi.org/10.1016/j.vaccine.2009.06.040
- McKenzie R, Bourgeois AL, Frech SA, Flyer DC, Bloom A, Kazempour K, Glenn GM. Transcutaneous immunization with the heat-labile toxin (LT) of enterotoxigenic Escherichia coli (ETEC): protective efficacy in a double-blind, placebo-controlled challenge study. Vaccine 2007; 25:3684-91; PMID:17313998; http://dx.doi.org/10.1016/j.vaccine.2007.01.043
- Hansen S, Lehr CM. Nanoparticles for transcutaneous vaccination. Microb Biotechnol 2012; 5:156-67; PMID:21854553; http://dx.doi.org/10.1111/j.1751-7915.2011.00284.x
- Suh H, Shin J, Kim YC. Microneedle patches for vaccine delivery. Clin Exp Vaccine Res 2014; 3:42-9; PMID:24427762; http://dx.doi.org/10.7774/cevr.2014.3.1.42
- Kim YC, Park JH, Prausnitz MR. Microneedles for drug and vaccine delivery. Adv Drug Deliv Rev 2012; 64:1547-68; PMID:22575858; http://dx.doi.org/10.1016/j.addr.2012.04.005
- Chang SY, Cha HR, Igarashi O, Rennert PD, Kissenpfennig A, Malissen B, Nanno M, Kiyono H, Kweon MN. Cutting edge: Langerin+ dendritic cells in the mesenteric lymph node set the stage for skin and gut immune system cross-talk. J Immunol 2008; 180:4361-5; PMID:18354155; http://dx.doi.org/10.4049/jimmunol.180.7.4361
- Enioutina EY, Visic D, Daynes RA. The induction of systemic and mucosal immune responses to antigen-adjuvant compositions administered into the skin: alterations in the migratory properties of dendritic cells appears to be important for stimulating mucosal immunity. Vaccine 2000; 18:2753-67; PMID:10781863; http://dx.doi.org/10.1016/S0264-410X(00)00059-1
- Belyakov IM, Hammond SA, Ahlers JD, Glenn GM, Berzofsky JA. Transcutaneous immunization induces mucosal CTLs and protective immunity by migration of primed skin dendritic cells. J Clin Invest 2004; 113:998-1007; PMID:15057306; http://dx.doi.org/10.1172/JCI20261
- Beagley KW, Eldridge JH, Lee F, Kiyono H, Everson MP, Koopman WJ, Hirano T, Kishimoto T, McGhee JR. Interleukins and IgA synthesis. Human and murine interleukin 6 induce high rate IgA secretion in IgA-committed B cells. J Exp Med 1989; 169:2133-48; PMID:2786548; http://dx.doi.org/10.1084/jem.169.6.2133
- Corthesy B. Role of secretory IgA in infection and maintenance of homeostasis. Autoimmun Rev 2013; 12:661-5; PMID:23201924; http://dx.doi.org/10.1016/j.autrev.2012.10.012
- Park CO, Kupper TS. The emerging role of resident memory T cells in protective immunity and inflammatory disease. Nat Med 2015; 21:688-97; PMID:26121195; http://dx.doi.org/10.1038/nm.3883