ABSTRACT
In attempts to develop recombinant subunit vaccines against rotavirus disease, it was previously shown that the N-terminal truncated VP8* protein, VP8-1 (aa26-231), is a good vaccine candidate when used for immunization in combination with Freund's adjuvant. However, this protein stimulated only weak immune response when aluminum hydroxide was used as an adjuvant. In this study, the nontoxic B subunit of cholera toxin (CTB) was employed as intra-molecular adjuvant to improve the immunogenicity of VP8-1. Both, the N-terminal and C-terminal fusion proteins, were purified to homogeneity, at which stage they formed pentamers, and showed significantly higher immunogenicity and protective efficacy than a VP8-1/aluminum hydroxide mixture in a mouse model. Compared to VP8-1-CTB, CTB-VP8-1 showed higher binding activity to both, GM1 and the conformation sensitive neutralizing monoclonal antibodies specific to VP8. More importantly, CTB-VP8-1 elicited higher titers of neutralizing antibodies and conferred higher protective efficacy than VP8-1-CTB. Therefore, the protein CTB-VP8-1, with enhanced immunogenicity and immunoprotectivity, could be considered as a viable candidate for further development of an alternative, replication-incompetent, parenterally administered vaccine against rotavirus disease.
Introduction
Rotavirus is one of the major causes of severe diarrhea worldwide, resulting in high morbidity and mortality in children under 5 y old.Citation1,2 As there is no specific medicine to treat people with rotavirus illness, vaccination is considered as the most powerful strategy to prevent rotavirus infection and reduce disease burden. Currently, 2 live attenuated rotavirus vaccines, Rotarix® (GlaxoSmithKline) and RotaTeq® (Merck), have been approved by FDA and are widely used in most countries.Citation3,4 Recently, a third rotavirus vaccine was licensed in India.Citation5,6 However, the protective efficiency of these vaccines is lower in certain low-income countries in Africa, Asia and Central America,Citation7-10 where vaccines are most needed to save lives. In addition, there have been safety concerns about these 2 vaccines, such as increased risk of intussusception Citation11,12 and the re-assortment of vaccine strains with infected rotavirus strains.Citation13-17
Compared to live attenuated rotavirus, recombinant subunit vaccine is safer due to the absence of viral replication-competent genetic materials. In the past 20 years, a number of recombinant antigens derived from rotavirus structural proteins (VP4, VP6 and VP7) and the non-structural protein NSP4 as well as different types of virus-like particles have been explored as candidates of rotavirus vaccines, and they have been shown to be effective in small animal models.Citation18-23
Among these proteins, VP4 and VP7 can induce neutralizing antibodies independently.Citation24 The spike protein VP4 plays an important role in the penetration, internalization and virulence of rotavirus, and can be cleaved into VP8* (∼28 kDa) and VP5* (∼60 kDa) in the presence of trypsin.Citation25,26 The VP8* protein can bind to cell surface receptors and mediate the attachment of rotavirus, and antibodies against VP8 can block the first step in rotavirus infection. Wen et al. expressed the core of VP8 (ΔVP8*, aa65-aa223) in Escherichia coli (E. coli) and proved it to be immunogenic in a guinea pig model; however, it was less immunogenic in a mouse model.Citation18 Later, the tetanus toxoid CD4 T cell epitope P2 was added to the N-terminus of ΔVP8* to improve its immunogenicity,Citation27 but the fusion protein was not able to induce sufficient neutralizing antibody response (with response rate of about 60%) in adults after a 3-dose immunization regimen.Citation28 Meanwhile, we found that a novel truncated VP8 protein with a prolonged N terminus, VP8-1 (aa26-231), could stimulate higher levels of neutralizing antibodies than ΔVP8* in mice when the proteins were used for immunization in combination with Freund's adjuvant, and protected the pups from rotavirus-induced diarrhea effectively in a maternal antibody model.Citation29 However, when aluminum hydroxide was used as an adjuvant, VP8-1 induced only a weak antibody response. Therefore, the immunogenicity of VP8-1 in combination with aluminum hydroxide as adjuvant should be enhanced before VP8-1 can be further investigated as a human vaccine candidate. Different aluminum-based adjuvants and immunization doses of immunization have been tried to improve the performance of VP8-1; however, no significant improvement in immunogenicity has been observed (data not published).
Bacterial toxins are often used as carrier proteins or adjuvants to improve the immunogenicity of target haptens or antigens. The success of conjugate pneumonia vaccine (Prevnar®13) is an excellent example of a conjugate vaccine, in which a non-toxic mutant of diphtheria toxin (CRM197) was included as an intra-molecular adjuvant.Citation30 In addition to CRM197, other bacterial toxins and toxin mutants have also been shown to be effective for improving the immunogenicity of a given antigen, including cholera toxin,Citation31 the nontoxic B subunit of cholera toxin (CTB),Citation32 E.coli heat labile toxin,Citation33 tetanus toxin Citation27 and others. Among these toxins and toxin mutants, CTB has been extensively studied and was shown to enhance both of the humoral immunity and mucosal immunity in different formulations with a given antigen (coupled or uncoupled) or through different immunization routes (oral, intranasal or intramuscular).Citation32 However, in the case of VP8-1, uncoupled CTB did not improve the immunogenicity of the target protein (data not shown).
In this study, CTB was fused to either the N- or C-terminus of VP8-1, producing CTB-VP8-1 or VP8-1-CTB, respectively, to improve the immunogenicity of VP8-1. Both of the fusion proteins were homogeneous pentamers after purification and refolding, and they maintained the monosialoganglioside 1 (GM1) binding property of CTB and the binding capacity of VP8-1 to neutralizing antibodies. When aluminum hydroxide was used as adjuvant, the fusion proteins, especially CTB-VP8-1, manifested significantly higher immunogenicity and protective efficacy in a mouse model compared to VP8-1. These results suggested that CTB-VP8-1 should be considered as a viable candidate for further development of an alternative, replication-incompetent, parenterally administrated vaccine against rotavirus disease.
Results
Expression, purification and polymerization analysis of the recombinant proteins
VP8-1 was expressed in soluble form and purified from the supernatant of the cell lysate to homogeneity as described previously.Citation29 The other 3 recombinant proteins (CTB-VP8-1, VP8-1-CTB and CTB) were expressed in insoluble forms (inclusion bodies). After purification, the purity of all the target proteins was greater than 90%, as analyzed by SDS-PAGE and immunoblot when the samples were boiled in loading buffer with reducing reagent ().
Figure 1. Expression, purification and characterization of the recombinant proteins. (A) Schematic diagram of the recombinant proteins. (B) 12% SDS-PAGE and immunoblotting analysis of the purified recombinant proteins. The antibody in immunoblotting was anti-VP8 mAb 1E1. Lane M: protein molecular weight markers; lane 1, CTB; lane 2, VP8-1; lane 3, CTB-VP8-1; lane 4, VP8-1-CTB. (C) HPSEC (G3000PWXL) analysis of purified proteins. The retention time (the deduced molecular weight) for a given protein was labeled with the corresponding color of its curve: green for CTB-VP8-1, red for VP8-1-CTB, blue for VP8-1 and purple for CTB. (D) The AUC analysis of VP8-1 and the fusion proteins. (E) The binding activity of the recombinant proteins to GM1 detected using anti-VP8 mAb 1E1. (F) The binding activity of the recombinant proteins to GM1 detected using anti-serum against CTB. In both (E) and (F), each assay was repeated in 3 wells, and the error bar represents the SD.
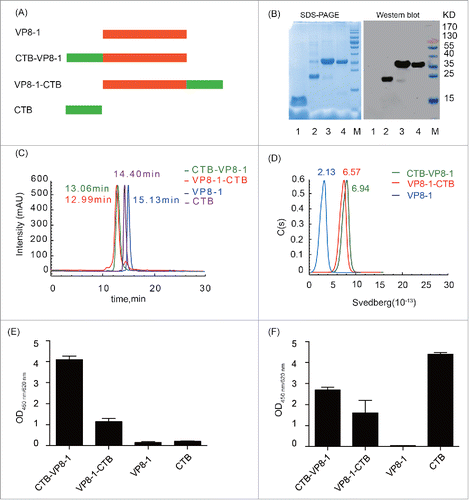
To further investigate the aggregation status and the homogeneity of the recombinant proteins in solution, HPSEC analyses were conducted. The proteins CTB-VP8-1, VP8-1-CTB, VP8-1 and CTB were eluted with a single peak at 13.06 min, 12.99 min, 15.13 min and 14.40 min, respectively, and their deduced molecular weight was approximately 150 kDa, 150 kDa, 50 kDa and 60 kDa (). Compared with their theoretical molecular weights (34 kDa, 34 kDa, 22 kDa and 12 kDa for CTB-VP8-1, VP8-1-CTB, VP8-1 and CTB, respectively), CTB and both fusion proteins existed as homogeneous pentamers, while VP8-1 was dimer in solution. These findings were further supported by analytical ultracentrifugation (AUC) analysis ().
GM1 binding activity and antigenicity of the recombinant proteins
As binding to its receptor, GM1, is essential for the adjuvant activity of CTB,Citation34 the binding capacities of the fusion proteins were first assessed using a modified ELISA with GM1-coated microplates. The results indicated that both fusion proteins could bind to GM1 obviously () although their binding activities were slightly lower than that of CTB (). Meanwhile, CTB fused to the N-terminus of VP8-1 (CTB-VP8-1) retained more receptor binding capacity than CTB fused to the C-terminus of VP8-1 (VP8-1-CTB) ().
To determine the influence of CTB on the conformation of VP8-1 in the fusion proteins, the antigenicity of these recombinant proteins were tested using 3 conformation-sensitive neutralizing mAbs (B1, B3 and B5) and an anti-serum sample collected from mice immunized with inactivated rotavirus. Compared with VP8-1, the reactivity of the fusion proteins with mAbs and anti-serum was not decreased (). Specially, for mAb B1 and anti-serum, the reactivity of CTB-VP8-1 and VP8-1-CTB was similar and both were slightly higher than that of VP8-1, while for mAb B3 and B5, the reactivity of CTB-VP8-1 was higher than that of VP8-1-CTB.
Figure 2. The reactivity of the recombinant proteins with conformation-sensitive VP8-specific neutralizing mAbs and mouse anti-serum elicited by inactivated rotavirus. (A) mAb B1 (IC50 = 100 ng/ml); (B) mAb B3 (IC50 = 80 ng/ml); (C) mAb B5 (IC50 = 55 ng/ml); (D) Pooled serum samples from inactivated rotavirus immunized mice. Each assay was repeated in 3 wells, and the error bar represents the SD.

Immunogenicity of the recombinant proteins in aluminum adjuvant
To evaluate the immunogenicity of the recombinant proteins in aluminum adjuvant, groups of mice were immunized with 3 doses of 10 μg of each individual protein. Fourteen days after the last dose, blood samples were collected to determine anti-VP8 antibody titers and neutralizing antibody titers. As shown in , VP8-1 in aluminum adjuvant elicited very low levels of anti-VP8 antibody and neutralizing antibody, which were not significantly different from those induced by negative controls, or the CTB group. When VP8-1 was fused to CTB either at its N- or C- terminus (CTB-VP8-1 or VP8-1-CTB), it could induce significantly higher titers of anti-VP8 antibodies and neutralizing antibodies than VP8-1 alone. Meanwhile, the anti-VP8 antibody titers induced by CTB-VP8-1 reached approximately 1×104, but those induced by VP8-1-CTB were less than 1×103 (). Furthermore, the geometric mean titers (GMTs) of neutralizing antibodies elicited by CTB-VP8-1 and VP8-1-CTB were 5,026 and 3,411, respectively (). The former was slightly higher than the latter, but the difference was not significant (P = 0.2073). Weak neutralizing activity was observed for the CTB antibody, but there was no significant difference between the CTB and PBS immunized groups (P = 0.0793) ().
Figure 3. The immunogenicity of the recombinant proteins. Groups of mice were immunized 3 times at an interval of 14 d with 10 μg of CTB-VP8-1, VP8-1-CTB, VP8-1 or CTB in aluminum hydroxide adjuvant, and the serum samples collected on day 14 after the final immunization were tested. (A) Anti-VP8 antibody titers, which are presented on a log10 scale; (B) neutralizing antibody titers, which are presented on a log2 scale. The error bars indicate the standard error of each group (n = 7). Different numbers of stars (*) indicate significant difference between the anti-VP8 antibody titers or neutralizing antibody titers elicited by the fusion proteins and those elicited by VP8-1 (****, P < 0.0001, **, P < 0.01).
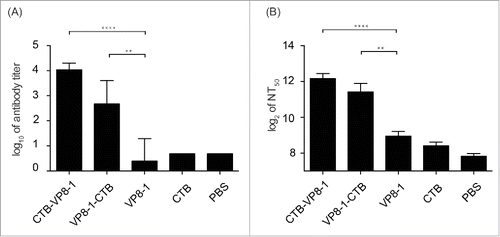
Protective efficacy of the recombinant proteins in a Balb/c mouse maternal antibody model
In previous studies, maternal antibodies were found to protect offspring from rotavirus infection and decrease the severity of rotavirus-induced diarrhea.Citation29 In this study, the pups delivered by dams immunized with recombinant proteins in aluminum adjuvant were challenged with live rotavirus (strain LLR). Results for both diarrhea and the pathological changes in the ileum of challenged pups indicated that the fusion proteins, especially CTB-VP8-1, manifested significantly higher protective efficacy than VP8-1.
After oral challenge with rotavirus strain LLR, the percentages of pups developing diarrhea were 100%, 45.45%, 76.47%, 100% and 100% in the VP8-1, CTB-VP8-1, VP8-1-CTB, CTB and PBS groups, respectively ( and ). While almost all of the pups in the VP8-1, CTB and PBS groups developed severe diarrhea, pups with severe diarrhea constituted 9.09% (1/11) in the CTB-VP8-1group and 58.82% (10/17) in the VP8-1-CTB group of ( and ). Compared with VP8-1, immunization with CTB-VP8-1 and VP8-1-CTB provided more effective protection against rotavirus-induced diarrhea with delayed onset, shortened duration and lower severity ( and ). Among all 3 VP8-derived recombinant proteins, CTB-VP8-1 showed the highest protective efficacy in pups delivered by vaccinated dams. The CTB-VP8-1 group had lower percentage of pups that developed diarrhea (5/11 vs 13/17, P = 0.047) or severe diarrhea (1/11 vs 10/17, P = 0.004) and lower cumulative diarrhea scores (8.1 ± 1.5 vs 11 ± 3.1, P = 0.08) than VP8-1-CTB group ( and ).
Figure 4. The course of diarrhea in pups after rotavirus challenge in a mouse maternal antibody protection model. The pups in the 4 immunized groups (immunized with CTB-VP8-1, VP8-1-CTB, VP8-1, CTB and PBS) were challenged with rotavirus strain LLR on days 7 of life, and they were monitored daily for diarrhea for 7days post infection. (A) The percentage of pups with diarrhea from 1 to 7 d post infection. (B) The percentage of pups with severe diarrhea from 1 to 7 d post infection. Different numbers of * indicate significant differences between the percentage of pups with diarrhea or severe diarrhea in a given immunized group and those in the CTB group (**, P < 0.01, ***, P < 0.001 and ****, P < 0.0001).
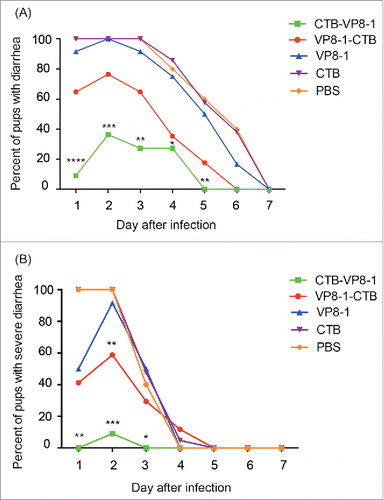
Table 1. The onset, duration and severity of rotavirus induced diarrhea for pups in a given immunized group in a maternal antibody model.
Additionally, the protective efficacy of these recombinant proteins against rotavirus-induced pathological intestinal changes were also evaluated. Typical pathological changes were observed in the ileum of pups in the CTB, VP8-1 and PBS groups, including: hydropic degeneration and diffuse necrosis of the villi, vacuolar degeneration, shedding of the villus tips, and inflammatory cells in the villous stroma (). Less severe tissue damage in the ileum was observed in the VP8-1-CTB group (), while there was no obvious pathological change observed in CTB-VP8-1 group ().
Figure 5. H&E staining images of ileum tissues after rotavirus challenge in a mouse maternal antibody model. The ileums of the pups were collected on day 2 post infection, fixed and sliced. Then, the sections were stained with hematoxylin and eosin (H&E) and observed under a microscope at an original magnification of 20×. Arrows indicate different histological changes such as vacuolar degeneration and necrosis of enterocytes (→), villus shortening (→), shedding of villus tips (→) and inflammatory cells in the villus stroma (→).
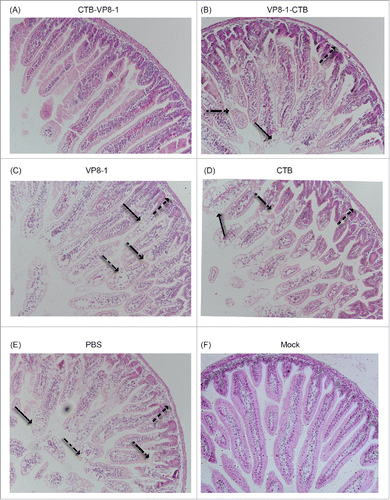
Discussion
In this study, the immunogenicity and immunoprotectivity of the truncated VP8 protein VP8-1 (aa16-231) Citation29 was successfully enhanced through expression and a fusion protein with CTB, either at its N- or C- terminus. Compared with VP8-1, the resulting fusion proteins, especially CTV-VP8-1, elicited significantly higher levels of rotavirus neutralizing antibodies and conferred better protection against rotavirus induced diseases when aluminum hydroxide was used as an adjuvant.
VP8-1 (aa26-231), with a prolonged N-terminus, induced notably higher titers of neutralizing antibodies and conferred dramatically higher protective efficacy against rotavirus challenge than ΔVP8* (aa65-223) when emulsified in Freund's adjuvant.Citation29 However, when aluminum hydroxide adjuvant was used, the protein only stimulated very weak antibody responses and provided nearly no protection against rotavirus infection as demonstrated in this study. Therefore, among future studies exploring the feasibility of VP8-1 as a parenteral rotavirus vaccine in humans, its immunogenicity in aluminum hydroxide adjuvant should first be improved. Different aluminum hydroxide adjuvants (including different P/Al concentration and ratio, pH values and salt concentrations) and higher doses (50 μg per dose), were tested;, however, the immune responses in mice were all weak, and no significant differences were observed (data not shown).
It was beyond our expectation that the immunogenicity of VP8-1 in aluminum hydroxide adjuvant was significantly weaker than that in Freund's adjuvant, and the reason for this phenomenon is unclear. In previously published studies, it has also been reported that the immunogenicity of some antigens in different adjuvants varies greatly.Citation35,36 When QS-21 (included in the AS-02 adjuvant) was used as an adjuvant, Ciarlet et al. found that antibody levels elicited by rotavirus 2/4/6/7 VLPs were comparable to those in animals immunized with Freund's adjuvant.Citation35 However, QS-21 was unavailable to us.
Non-toxic mutants or subunits of bacterial toxins are often used to improve the immunogenicity of a target antigen as an intra-molecular adjuvant through conjugation or fusion expression.Citation37 For example, in a commercial pneumonia vaccine (Prevnar®13), CRM197, a non-toxic mutant of diphtheria toxin, was successfully employed as an intra-molecular adjuvant and carrier protein.Citation38 CTB, the non-toxic B subunit of cholera toxin, is another widely used toxin-derived protein with adjuvant activity.Citation37 Although originally considered as an effective mucosal adjuvant for nasal or oral vaccines,Citation39 it was recently shown to enhance the immune response against its coupled target antigens when vaccinated intramuscularly.Citation40 In addition, it has been reported that repeated exposure to conjugates based on CRM197 or tetanus toxin could interfere with the anti-polysaccharide response.Citation41 CTB is not as widely used as CRM197 and tetanus toxin, and thus the anti-VP8 responses could not be significantly altered by pre-existing immune responses when CTB was used as carrier protein. Both of the fusion proteins (CTB-VP8-1 and VP8-1-CTB) manifested significantly higher immunogenicity and immunoprotectivity than the VP8-1 protein ( and ).
Comparison of the 2 fusion proteins of VP8-1 and CTB showed that the CTB-VP8-1 protein, induced higher anti-VP8 antibody and neutralizing antibody titers in mice and showed higher protective efficacy in a mouse maternal antibody protection model. These differences may be attributable to 2 reasons. On one hand, CTB-VP8-1 can be more effectively presented by antigen-presenting cells (APCs) than VP8-1-CTB. Through receptor-mediated uptake, antigens coupled to CTB can be effectively internalized and presented by APCs, resulting in increased immunogenicity in the organism.Citation34,42 The receptor (GM1) binding activity of CTB-VP8-1 was significantly higher than that of VP8-1-CTB (). Thus, the CTB-VP8-1 protein would be more effectively presented by APCs and be of higher immunogenicity than VP8-1-CTB. On the other hand, the conformation of VP8-1 in the CTB-VP8-1 protein may have been better maintained than that in the VP8-1-CTB protein. The polypeptides of residues 65-224 of VP8 form a lectin-like globular domain, while the fragment of residues 26-64 extends from the globular domain in a more flexible form.Citation25 As for VP8-1, it is believed that its N-terminus is more flexible than its C-terminus. Thus, the conformation of VP8-1 may be more intensively disturbed by CTB when CTB is fused at its C-terminus than at its N-terminus. Notably, although there was no difference in the reactivity of the 2 fusion proteins with anti-serum elicited by rotavirus, the CTB-VP8-1 protein had higher reactivity with 2 out of 3 conformation-sensitive neutralizing mAbs than the VP8-1-CTB protein ().
It is exciting that the immunogenicity of VP8-1 in aluminum hydroxide adjuvant was successfully enhanced by fusion with CTB, but there is still a great deal of work that should be done in the future before CTB-VP8-1 can be used as a candidate vaccine in humans. First, the corresponding CTB-VP8-1 proteins of P[4], P[6] and P[8], the 3 predominant P genotypes in humans,Citation43 should be expressed to cover most of the human rotavirus P genotypes, because the protection of the VP8 protein against heterotypic rotavirus infection can be significantly lower than VP8 protection against homotypic virus infection.Citation18 Second, the protective efficacy of CTB-VP8-1 should be evaluated in an active immunity model instead of the passive mouse maternal antibody protection model used in this study. Third, different immunization routes and doses should be tested to improve the performance of CTB-VP8-1. Last, large animals such as pigs and monkeys should be used to investigate the immunogenicity and immunoprotectivity of CTB-VP8-1, as it has been found that some rotavirus antigens that induced high protective immunity in small animals were not effective in large animals.Citation44,45
Materials and methods
Virus, cell culture, monoclonal antibodies and serum
Virus stock
The lamb rotavirus strain LLR (G10P[12]) was kindly supplied by Beijing Wantai Biological Pharmacy Enterprise Co., Ltd. (Beijing, China) and propagated in MA-104 cells (ATCC®CRL2378.1) as described previously.Citation46 The infectious titer of rotavirus was determined by an enzyme-linked immune-spot (Elispot) assay.Citation47 When used as antigen for immunization, the virus was inactivated by 1% formalin treatment at 37°C for 72 h, and then the excess formalin was neutralized with 0.05% sodium bisulfite for 30 min followed by dialysis against PBS; the inactivation was verified by a TCID50 assay to demonstrate the lack of a cytopathic effect.
Monoclonal antibodies (mAbs)
Specific mouse anti-VP8 mAbs were prepared using standard hybridoma technology.Citation48 Balb/c mice were immunized through 3 injections (0, 2, 4 weeks) of lamb rotavirus LLR. These mAbs were produced in mouse ascites fluid and affinity-purified using a Protein A column. Then, the purified mAbs were diluted to 1.0 mg/ml in PBS and stored in PBS at −20°C. In this study, one mAb (1E1), which recognize a linear epitope was used for immunoblot analysis, and 3 conformation-sensitive neutralizing mAbs (B1, B3 and B5) were used for antigenicity analysis of recombinant proteins in enzyme-linked immunosorbent assays (ELISA).
Serum samples
The anti-rotavirus and anti-CTB serum used in the ELISA assays were collected from the mice after 3 immunization doses with inactivated rotavirus LLR or recombinant CTB, respectively.
Construction of the expression plasmids
The recombinant expression plasmid containing the VP8-1 gene (PTO-T7-VP8-1) was obtained as described previously.Citation29 The gene encoding CTB (GenBank accession number: KT278773) was synthesized by Sangon Biotech (Shanghai, China) with the restriction endonuclease sites Bgl II and Nde I at the 5′-terminus, and BamH I and Hind III at the 3′-terminus for subsequent cloning. A stop codon was introduced into the CTB gene between BamH I and Hind III. The CTB gene was cloned into the PTO-T7 vector between Nde I and Hind III multiple cloning sites (PTO-T7-CTB).Citation49 The gene encoding VP8-1 was amplified and cloned into PTO-T7-CTB at BamH I and Hind III sites (PTO-T7-CTB-VP8-1). The gene encoding CTB was digested with Bgl II and Hind III and cloned into PTO-T7-VP8-1 between the BamH I and Hind III site located at the 3′-terminus of VP8-1-encoding gene (PTO-T7-VP8-1-CTB). Schematic diagrams of the clones are shown in .
Expression and purification of the recombinant proteins
The plasmids PTO-T7-CTB, PTO-T7-CTB-VP8-1 and PTO-T7-VP8-1-CTB were transformed into competent cells of the E. coli expression strain ER2566 (NEB, Bad Schwalbach, Germany) for expression. The E.coli strains carrying the recombinant plasmids were incubated at 37°C with shaking (180 r.p.m.), and protein expression was induced by the addition of 0.2 mM isopropyl-β-d-thiogalactoside (IPTG) at 25 °C for another 6 h. Most of the recombinant proteins formed inclusion bodies, and were purified from the cellular debris by extensive washing with buffer (20 mM Tris-HCl, pH7.4, 5 mM EDTA, 100 mM NaCl, and 2% Triton X-100). Then, the inclusion bodies were dissolved in 4 M urea. The solubilized fusion proteins, CTB-VP8-1 and VP8-1-CTB, and the CTB were re-natured by removal of urea by dialysis against 50 mM Tris-HCl, pH 8.0 (TB 8.0), and phosphate buffer, pH 6.0 (PB 6.0) at room temperature. Subsequently, the refolded fusion proteins were further purified using a Q Sepharose High Performance column (GE, Sweden) with elution by 200 mM NaCl. The CTB protein was purified through an SP Sepharose FF column (GE, Sweden) with elution by 1M NaCl. The VP8-1 protein was expressed and purified as described previously.Citation29 Protein concentrations were determined using a BCA assay (Thermo Fisher Scientific, Rockford, USA) according to the manufacturer's instructions, and all purified proteins were examined using sodium dodecyl sulfate polyacrylamide gel electrophoresis (SDS-PAGE), followed by immunoblotting. For immunoblotting, the proteins were transferred to Immobilon-P polyvinylidene difluoride membranes (Millipore, Massachusetts, USA) after separation, and the free binding sites on the membrane were blocked with 5% skim milk. After incubation with VP8-specific mAb (1E1) diluted in 5% skim milk for 1 h, the membrane was washed with 50 mM Tris-HCl (pH8.0) containing 0.05% Tween 20. Then, binding antibodies were detected with horseradish peroxide-conjugated goat anti-mouse antibodies diluted at 1:5000 (Abcam, Cambridge, UK)) and visualized by enhanced chemiluminescence (Immobilon Western HRP substrate, Thermo). The protein bands on the blots were detected using an ImageQuant LAS 4000 biomolecular imager (GE, Fairfield, USA).
High-performance size-exclusion chromatography (HPSEC)
The homogeneities and the molecular weights of the purified recombinant proteins, VP8-1, VP8-1-CTB, CTB-VP8-1 and CTB were evaluated using an Agilent 1200 HPLC system (Agilent Technologies, Santa Clara, CA) with an analytical TSK Gel G3000 PWXL 7.8 mm × 300 mm column (TOSOH, Tokyo, Japan). The flow rate was maintained at 0.5 ml/min with PBS as the elution buffer, and the absorbance at 280 nm was monitored for the detection of the protein in the eluent.
Analytical ultracentrifugation (AUC)
The homogeneity of the purified recombinant proteins (VP8-1, VP8-1-CTB and CTB-VP8-1) in solution was further evaluated in an AUC velocity assay using a Beckman XL-A analytical ultracentrifuge (Beckman Coulter, Fullerton, CA) as previously described by Li et al.Citation19 For the SV analyses, the sedimentation coefficient and f/f0 were obtained by the c(s) method using Sedfit software. The data were fitted with a single ideal species model.
Enzyme-linked immuno-sorbent assay (ELISA)
CTB receptor binding assay
To determine the receptor binding activities of CTB and its fusion proteins, GM1 (Sigma, Saint Louis, MO, USA), the receptor of CTB, was coated on 96-well microplates and the plates were blocked. The microplates were incubated with each recombinant protein (50 ng/well) at 37°C for 30 min and then incubated with anti-VP8 mAb 1E1 or mouse anti-CTB serum. The reaction was detected with HRP-conjugated goat anti-mouse IgG (GAM-HRP) (Abcam, Cambridge, UK) diluted at 1:5000. Tetramethylbenzidine (TMB) substrate was added, and the reaction was stopped with 2M H2SO4 after 15 min of incubation at 37°C; then the absorbance at 450 nm was determined using an automated ELISA reader (TEACAN, Männedorf, Switzerland,) with a reference wavelength of 620 nm.
Antigenicity analysis
To assess the antigenicity of the recombinant proteins, 96-well microplates were coated with 100 μL of recombinant VP8-1, VP8-1-CTB, CTB-VP8-1 or CTB at a concentration of 500 ng/ml. Three conformation-sensitive neutralizing mAbs (B1, B3 and B5) and a serum sample obtained from mice immunized with 3 doses of inactivated rotavirus (anti-RV serum) were 2-fold serially diluted, and added into the microplates. After incubation at 37°C for 30 min, the reactivity of each well was detected by GAM-HRP.
Anti-VP8 antibody titer determination in immunized mice
To evaluate the immunogenicity of the recombinant proteins, 96-well microplates were coated with VP8-1 in TB 8.0 (50 ng/well). The serial twofold diluted anti-sera obtained from mice immunized with VP8-1, VP8-1-CTB, CTB-VP8-1, CTB or PBS were added to the plates and incubated at 37°C for 30 min, then detected by GAM-HRP. The end point dilution yielding an OD450/620 above 0.2 was considered as its titer.
Animal immunization and virus challenge
All of the experiments were conducted according to the guidelines approved by the experimental animal ethics committee of Xiamen University. Five- to 6-week-old naive female Balb/c mice were divided into groups of 7 and maintained under animal biosafety level-2 conditions in isolator cages for the entire duration of the experiment. The mice were immunized intramuscularly with 10 μg of recombinant VP8-1, VP8-1-CTB, CTB-VP8-1 and CTB with aluminum hydroxide adjuvant or aluminum hydroxide adjuvant only (PBS) for 3 doses with 2-week intervals between each dose. The antigens (0.2 mg/ml) were mixed with 2× aluminum hydroxide adjuvant (31 mmol/L Aluminum and 4.7 mmol/L Phosphate, pH7.4) at 1:1 in volume. After at least 4 hours of settling, the antigen-adjuvant mixtures were mixed again and mice were immunized with 100 μl of the mixtures. Blood samples were collected prior to each immunization as well as 14 d after the third immunization.
After the third immunization, the female mice were mated with male mice and kept in individual cages prior to delivery. On days 6∼8 of life, the pups were challenged with 5 ×106 TCID50 LLR rotavirus (equivalent to 20 DD50 [diarrhea dose 50 percent]; unpublished data) by intragastric inoculation. The diarrhea of each mouse was monitored daily and the diarrhea scores were recorded daily for 7 d after virus challenge to assess the severity of diarrhea as described.Citation19 A score of 1 was considered as normal, while a score of 2 or 3 was considered as diarrhea or severe diarrhea, respectively.
Micro-neutralization assay
The neutralizing activities of the sera from mice immunized with recombinant proteins were determined by Elispot assay as previously described.Citation47 Briefly, 100 μl aliquots of 2-fold serially diluted serum were mixed with equal volumes of trypsin-activated rotavirus LLR; after neutralization, 100 μl of each serum-rotavirus mixture was added to MA104 cells in 96-well microplates. After 14 h of incubation, reductions in rotavirus infectivity were measured by Elispot. The inhibition was calculated by the following formula: 100 × [1- (average number of spots in experiment wells/average number of spots in control wells)]. The reciprocal of the serum dilution with a 50% reduction in the spot number compared to the control was considered as the neutralizing titer.
Histopathology
The small intestines of pups randomly selected from any given group were collected on day 2 post rotavirus infection, the time point at which the most severe tissue damage occurred in the ileum.Citation50 The collected intestine samples were fixed and mounted on slides, and then ileum sections were stained with hematoxylin and eosin using a standard procedure.Citation51
Statistical analysis
Statistical comparisons between the groups were performed with SPSS16.0 (IBM, New York, CA). A Chi-square test was used when comparing serum antibody levels and neutralizing antibody levels after 3 doses of immunization. The Wilcoxon signed-rank test, ANOVA, and Chi-square test were used when comparing the protection against LLR challenge in the offspring. A difference was considered significant when a P-value of <0.05 was obtained.
Disclosure of potential conflicts of interest
No potential conflicts of interest were disclosed.
Funding
Financial support was provided by the National Natural Science Foundation of China (81501741) and Fujian Province Science and Technology Innovation Platform (2014Y2004).
References
- Tate JE, Burton AH, Boschi-Pinto C, Steele AD, Duque J, Parashar UD. 2008 estimate of worldwide rotavirus-associated mortality in children younger than 5 years before the introduction of universal rotavirus vaccination programmes: a systematic review and meta-analysis. Lancet Infect Dis 2012; 12:136-41; PMID:22030330; http://dx.doi.org/10.1016/S1473-3099(11)70253-5
- Parashar UD, Hummelman EG, Bresee JS, Miller MA, Glass RI. Global illness and deaths caused by rotavirus disease in children. Emerg Infect Dis 2003; 9:565-72; PMID:12737740; http://dx.doi.org/10.3201/eid0905.020562
- Ruiz-Palacios GM, Pérez-Schael I, Velázquez FR, Abate H, Breuer T, Clemens SAC, Cheuvart B, Espinoza F, Gillard P, Innis BL, et al. Safety and efficacy of an attenuated vaccine against severe rotavirus gastroenteritis. N Engl J Med 2006; 354:11-22; PMID:16394298; http://dx.doi.org/10.1056/NEJMoa052434
- Vesikari T, Matson DO, Dennehy P, Van Damme P, Santosham M, Rodriguez Z, Dallas MJ, Heyse JF, Goveia MG, Black SB, et al. Safety and efficacy of a pentavalent human-bovine (WC3) reassortant rotavirus vaccine. N Engl J Med 2006; 354:23-33; PMID:16394299; http://dx.doi.org/10.1056/NEJMoa052664
- Bhandari N, Rongsen-Chandola T, Bavdekar A, John J, Antony K, Taneja S, Goyal N, Kawade A, Kang G, Rathore SS, et al. Efficacy of a monovalent human-bovine (116E) rotavirus vaccine in Indian infants: a randomised, double-blind, placebo-controlled trial. Lancet 2014; 383:2136-43; PMID:24629994; http://dx.doi.org/10.1016/S0140-6736(13)62630-6
- Tate JE, Arora R, Kang G, Parashar UD. Rotavirus vaccines at the threshold of implementation in India. Natl Med J India 2014; 27:245-8; PMID:26037421
- Sow SO, Tapia M, Haidara FC, Ciarlet M, Diallo F, Kodio M, Doumbia M, Dembélé RD, Traoré O, Onwuchekwa UU, et al. Efficacy of the oral pentavalent rotavirus vaccine in Mali. Vaccine 2012; 30 Suppl 1:A71-8; PMID:22520140; http://dx.doi.org/10.1016/j.vaccine.2011.11.094
- Cunliffe NA, Witte D, Ngwira BM, Todd S, Bostock NJ, Turner AM, Chimpeni P, Victor JC, Steele AD, Bouckenooghe A, et al. Efficacy of human rotavirus vaccine against severe gastroenteritis in Malawian children in the first two years of life: a randomized, double-blind, placebo controlled trial. Vaccine 2012; 30 Suppl 1:A36-43; PMID:22520135; http://dx.doi.org/10.1016/j.vaccine.2011.09.120
- Zaman K, Anh DD, Victor JC, Shin S, Yunus M, Dallas MJ, Podder G, Thiem VD, Mai LTP, Luby SP. Efficacy of pentavalent rotavirus vaccine against severe rotavirus gastroenteritis in infants in developing countries in Asia: a randomised, double-blind, placebo-controlled trial. Lancet 2010; 376:568-70; http://dx.doi.org/10.1016/S0140-6736(10)60755-6
- Armah GE, Sow SO, Breiman RF, Dallas MJ, Tapia MD, Feikin DR, Binka FN, Steele AD, Laserson KF, Ansah NA, et al. Efficacy of pentavalent rotavirus vaccine against severe rotavirus gastroenteritis in infants in developing countries in sub-Saharan Africa: a randomised, double-blind, placebo-controlled trial. Lancet 2010; 376:606-14; PMID:20692030; http://dx.doi.org/10.1016/S0140-6736(10)60889-6
- Greenberg HB. Rotavirus vaccination and intussusception–act two. N Engl J Med 2011; 364:2354-5; PMID:21675894; http://dx.doi.org/10.1056/NEJMe1105302
- Weintraub ES, Baggs J, Duffy J, Vellozzi C, Belongia EA, Irving S, Klein NP, Glanz JM, Jacobsen SJ, Naleway A, et al. Risk of intussusception after monovalent rotavirus vaccination. N Engl J Med 2014; 370:513-9; PMID:24422678; http://dx.doi.org/10.1056/NEJMoa1311738
- Rose TL, Marques da Silva MF, Gomez MM, Resque HR, Ichihara MY, Volotao Ede M, Leite JP. Evidence of vaccine-related reassortment of rotavirus, Brazil, 2008-2010. Emerg Infect Dis 2013; 19:1843-6; PMID:24188273; http://dx.doi.org/10.3201/eid1911.121407
- Donato CM, Ch'ng LS, Boniface KF, Crawford NW, Buttery JP, Lyon M, Bishop RF, Kirkwood CD. Identification of strains of RotaTeq rotavirus vaccine in infants with gastroenteritis following routine vaccination. J Infect Dis 2012; 206:377-83; PMID:22615314; http://dx.doi.org/10.1093/infdis/jis361
- Bucardo F, Rippinger CM, Svensson L, Patton JT. Vaccine-derived NSP2 segment in rotaviruses from vaccinated children with gastroenteritis in Nicaragua. Infect Genet Evol 2012; 12:1282-94; PMID:22487061; http://dx.doi.org/10.1016/j.meegid.2012.03.007
- Boom JA, Sahni LC, Payne DC, Gautam R, Lyde F, Mijatovic-Rustempasic S, Bowen MD, Tate JE, Rench MA, Gentsch JR, et al. Symptomatic infection and detection of vaccine and vaccine-reassortant rotavirus strains in 5 children: a case series. J Infect Dis 2012; 206:1275-9; PMID:22872730; http://dx.doi.org/10.1093/infdis/jis490
- Hemming M, Vesikari T. Detection of rotateq vaccine-derived, double-reassortant rotavirus in a 7-year-old child with acute gastroenteritis. Pediatr Infect Dis J 2014; 33:655-6; PMID:24326415; http://dx.doi.org/10.1097/INF.0000000000000221
- Wen X, Cao D, Jones RW, Li J, Szu S, Hoshino Y. Construction and characterization of human rotavirus recombinant VP8* subunit parenteral vaccine candidates. Vaccine 2012; 30:6121-6; PMID:22885016; http://dx.doi.org/10.1016/j.vaccine.2012.07.078
- Li T, Lin H, Zhang Y, Li M, Wang D, Che Y, Zhu Y, Li S, Zhang J, Ge S, et al. Improved characteristics and protective efficacy in an animal model of E. coli-derived recombinant double-layered rotavirus virus-like particles. Vaccine 2014; 32:1921-31; PMID:24530406; http://dx.doi.org/10.1016/j.vaccine.2014.01.093
- Ward RL, McNeal MM. VP6: A candidate rotavirus vaccine. J Infect Dis 2010; 202 Suppl:S101-7; PMID:20684688; http://dx.doi.org/10.1086/653556
- Khodabandehloo M, Shahrabadi MS, Keyvani H, Bambai B, Sadigh Z. Recombinant outer capsid glycoprotein (VP7) of rotavirus expressed in insect cells induces neutralizing antibodies in rabbits. Iran J Public Health 2012; 41:73-84; PMID:23113180
- Xie L, Yan M, Wang X, Ye J, Mi K, Yan S, Niu X, Li H, Sun M. Immunogenicity and efficacy in mice of an adenovirus-based bicistronic rotavirus vaccine expressing NSP4 and VP7. Virus Res 2015; 210:298-307; PMID:26368053; http://dx.doi.org/10.1016/j.virusres.2015.09.010
- Mackow ER, Vo PT, Broome R, Bass D, Greenberg HB. Immunization with baculovirus-expressed VP4 protein passively protects against simian and murine rotavirus challenge. J Virol 1990; 64:1698-703; PMID:2157052
- Offit PA. Rotaviruses: immunological determinants of protection against infection and disease. Adv Virus Res 1994; 44:161-202; PMID:7817873; http://dx.doi.org/10.1016/S0065-3527(08)60329-2
- Settembre EC, Chen JZ, Dormitzer PR, Grigorieff N, Harrison SC. Atomic model of an infectious rotavirus particle. EMBO J 2011; 30:408-16; PMID:21157433; http://dx.doi.org/10.1038/emboj.2010.322
- References S, Estes M, Graham D, Mason B. Proteolytic enhancement of rotavirus infectivity: molecular mechanisms. J Virol 1981; 39:879-88; PMID:6270356
- Wen X, Wen K, Cao D, Li G, Jones RW, Li J, Szu S, Hoshino Y, Yuan L. Inclusion of a universal tetanus toxoid CD4 T cell epitope P2 significantly enhanced the immunogenicity of recombinant rotavirus DeltaVP8* subunit parenteral vaccines. Vaccine 2014; 32:4420-7; PMID:24962749; http://dx.doi.org/10.1016/j.vaccine.2014.06.060
- Fix AD, Harro C, McNeal M, Dally L, Flores J, Robertson G, Boslego JW, Cryz S. Safety and immunogenicity of a parenterally administered rotavirus VP8 subunit vaccine in healthy adults. Vaccine 2015; 33:3766-72; PMID:26065919; http://dx.doi.org/10.1016/j.vaccine.2015.05.024
- Xue M, Yu L, Che Y, Lin H, Zeng Y, Fang M, Li T, Ge S, Xia N. Characterization and protective efficacy in an animal model of a novel truncated rotavirus VP8 subunit parenteral vaccine candidate. Vaccine 2015; 33:2606-13; PMID:25882173; http://dx.doi.org/10.1016/j.vaccine.2015.03.068
- Jackson LA, Gurtman A, van Cleeff M, Jansen KU, Jayawardene D, Devlin C, Scott DA, Emini EA, Gruber WC, Schmoele-Thoma B, et al. Immunogenicity and safety of a 13-valent pneumococcal conjugate vaccine compared to a 23-valent pneumococcal polysaccharide vaccine in pneumococcal vaccine-naive adults. Vaccine 2013; 31:3577-84; PMID:23688526; http://dx.doi.org/10.1016/j.vaccine.2013.04.085
- Lycke N, Holmgren J. Strong adjuvant properties of cholera toxin on gut mucosal immune responses to orally presented antigens. Immunology 1986; 59:301-8; PMID:3021614
- Stratmann T. Cholera toxin subunit B as adjuvant–an accelerator in protective immunity and a break in autoimmunity. Vaccines (Basel) 2015; 3:579-96; PMID:26350596; http://dx.doi.org/10.3390/vaccines3030579
- Marchioro SB, Fisch A, Gomes CK, Jorge S, Galli V, Haesebrouck F, Maes D, Dellagostin O, Conceição FR. Local and systemic immune responses induced by a recombinant chimeric protein containing Mycoplasma hyopneumoniae antigens fused to the B subunit of Escherichia coli heat-labile enterotoxin LTB. Vet Microbiol 2014; 173:166-71; PMID:25091529; http://dx.doi.org/10.1016/j.vetmic.2014.07.009
- Bharati K, Ganguly NK. Cholera toxin: a paradigm of a multifunctional protein. Indian J Med Res 2011; 133:179-87; PMID:21415492
- Ciarlet M, Crawford SE, Barone C, Bertolotti-Ciarlet A, Ramig RF, Estes MK, Conner ME. Subunit rotavirus vaccine administered parenterally to rabbits induces active protective immunity. J Virol 1998; 72:9233-46; PMID:9765471
- Pan M, Jiang H Q, Zhou Y, Zheng J. Comparison of the role of Freund's adjuvant and aluminum hydroxide hydrate in inducing mouse adaptive immune response. Current Immunology 2006; 18:2082-93.
- Pizza M, Giuliani MM, Fontana MR, Monaci E, Douce G, Dougan G, Mills KH, Rappuoli R, Del Giudice G. Mucosal vaccines: non toxic derivatives of LT and CT as mucosal adjuvants. Vaccine 2001; 19:2534-41; PMID:11257389; http://dx.doi.org/10.1016/S0264-410X(00)00553-3
- Gruber WC, Scott DA, Emini EA. Development and clinical evaluation of Prevnar 13, a 13-valent pneumocococcal CRM197 conjugate vaccine. Ann N Y Acad Sci 2012; 1263:15-26; PMID:22830997; http://dx.doi.org/10.1111/j.1749-6632.2012.06673.x
- Holmgren J, Adamsson J, Anjuere F, Clemens J, Czerkinsky C, Eriksson K, Flach CF, George-Chandy A, Harandi AM, Lebens M, et al. Mucosal adjuvants and anti-infection and anti-immunopathology vaccines based on cholera toxin, cholera toxin B subunit and CpG DNA. Immunol Lett 2005; 97:181-8; PMID:15752556; http://dx.doi.org/10.1016/j.imlet.2004.11.009
- Harakuni T, Sugawa H, Komesu A, Tadano M, Arakawa T. Heteropentameric cholera toxin B subunit chimeric molecules genetically fused to a vaccine antigen induce systemic and mucosal immune responses: a potential new strategy to target recombinant vaccine antigens to mucosal immune systems. Infect Immun 2005; 73:5654-65; PMID:16113283; http://dx.doi.org/10.1128/IAI.73.9.5654-5665.2005
- Pecetta S, Lo Surdo P, Toritini M, Proietti D, Zambonelli C, Bottomley MJ, Biagini M, Berti F, Costantino P, Romano MR, et al. Carrier priming with CRM197 or diphtheria toxoid has a different impact on the immunogenicity of the respective glycoconjugates: Biophysical and immunochemical interpretation. Vaccine 2015; 33:314-20; PMID:25448110; http://dx.doi.org/10.1016/j.vaccine.2014.11.026
- Baldauf KJ, Royal JM, Hamorsky KT, Matoba N. Cholera toxin B: one subunit with many pharmaceutical applications. Toxins (Basel) 2015; 7:974-96; PMID:25802972; http://dx.doi.org/10.3390/toxins7030974
- Santos N, Hoshino Y. Global distribution of rotavirus serotypes/genotypes and its implication for the development and implementation of an effective rotavirus vaccine. Rev Med Virol 2005; 15:29-56; PMID:15484186; http://dx.doi.org/10.1002/rmv.448
- Istrate C, Hinkula J, Charpilienne A, Poncet D, Cohen J, Svensson L, Johansen K. Parenteral administration of RF 8-2/6/7 rotavirus-like particles in a one-dose regimen induce protective immunity in mice. Vaccine 2008; 26:4594-601; PMID:18588935; http://dx.doi.org/10.1016/j.vaccine.2008.05.089
- El-Attar L, Oliver SL, Mackie A, Charpilienne A, Poncet D, Cohen J, Bridger JC. Comparison of the efficacy of rotavirus VLP vaccines to a live homologous rotavirus vaccine in a pig model of rotavirus disease. Vaccine 2009; 27:3201-8; PMID:19446192; http://dx.doi.org/10.1016/j.vaccine.2009.03.043
- Arnold M, Patton JT, McDonald SM. Culturing, storage, and quantification of rotaviruses. Curr Protoc Microbiol 2009; 15:C.3.1-C.3.24.
- Li T, Lin H, Yu L, Xue M, Ge S, Zhao Q, Zhang J, Xia N. Development of an enzyme-linked immunospot assay for determination of rotavirus infectivity. J Virol Methods 2014; 209:7-14; PMID:25172048; http://dx.doi.org/10.1016/j.jviromet.2014.08.012
- Zhang C. Hybridoma technology for the generation of monoclonal antibodies. In: Proetzel G, Ebersbach H, eds. Antibody Methods and Protocols. Berlin: Springer, 2012; 117-35.
- Luo WX, Zhang J, Yang HJ, Li SW, Xie XY, Pang SQ, Li SJ, Xia NS. Construction and application of an Escherichia coli high effective expression vector with an enhancer. Sheng Wu Gong Cheng Xue Bao 2000; 16:578-81; PMID:11191761
- Buragohain M, Dhale GS, Raut CG, Kang G, Chitambar SD. Analyses of clinical, pathological and virological features of human rotavirus strain, YO induced gastroenteritis in infant BALB/c mice. Microbes and Infection 2011; 13:331-8; PMID:21163362; http://dx.doi.org/10.1016/j.micinf.2010.12.001
- Lee GL. Munual of histological staining methods of the Armed Forces Institute of Pathology. New York: Blakiston Divison,McGraw-Hill; 1968; 258 p.