ABSTRACT
Plasmodium vivax predominates in South-East Asia and the American continent, causes significant morbidity and inflicts a huge socioeconomic burden. Sequencing completion of the Plasmodium vivax genome and transcriptome provides the chance to identify antigens. Enolase is the eighth enzyme in the glycolytic pathway, which, apart from its glycolytic function, also possess antigenic properties and is present on the cell wall of many invasive organisms, such as Candida albicans. In order to assess whether enolase of Plasmodium vivax is also antigenic, in this study, we first reported the expression and purification of recombinant Plasmodium vivax enolase (r-Pven) in Escherichia coli, using prokaryotic expression vector. The r-Pven was expressed in soluble form in E. coli, and the expression was verified by SDS-PAGE and western blotting analysis. The r-Pven was purified to 90% purity by nickel–nitrilotriacetic acid (Ni2+–NTA) resin chromatography. For reactivity with r-Pven, compared with the average values of the reactivity of control serum samples, the average values of the reactivity of 99 individual serums from vivax malaria patients appeared higher, and there was significant difference between them (p=0.0117<0.05). Mice anti-r-Pven antibodies inhibited the growth of in vitro cultures of P. falciparum. Mice immunized with r-Pven showed protection against a challenge with the mouse malarial parasite Plasmodium berghei. The antibodies raised against r-Pven were specific for Plasmodium and did not react to the host tissues. These observations established Plasmodium vivax enolase to be a potential protective antigen.
Introduction
The malaria, continues an important public health problem in the world, which affects mainly developing countries, including Africa, Asia and Latin America. By the World Health Organization, it was estimated that there were 214 million cases of malaria worldwide in 2015.Citation1 Among these cases, there were 13.8 million cases caused by Plasmodium vivax, which predominated in South-East Asia and the American continent where there were more than 50% of malaria cases.Citation1 Despite intensive efforts to control the malaria epidemic, the proportion of cases caused by P. vivax is increasing, and current prophylaxis and drug treatments still proved insufficient.Citation2
Sequencing completion of the Plasmodium vivax genome and transcriptome provides the chance to identify antigens. So far, several proteins, including merozoite surface protein 1 (MSP1), and apical membrane antigen 1 (AMA1), have shown antigens and/or potential vaccine candidates, but developing an effective malaria vaccine has remained an incredibly difficult challenge.Citation3
Enolase, as a major immunostimulatory protein of visceral leishmaniasis,Citation4 is present on the cell surface of many organisms,Citation5 could play a protective role in Candida albicans infection,Citation6 and has recently been reported as a potential vaccine candidate against Chlamydia pneumoniae.Citation7 Therefore, for investigating whether Plasmodium vivax Enolase (r-Pven) could play a protective role against Plasmodium vivax infection, we decided to produce Plasmodium vivax Enolase in Escherichia coli and examine the immunogenic and protective properties of Pven.
In this study, we had successfully expressed P. vivax Enolase in Escherichia coli, examined the immunogenic properties of Pven in sera of P. vivax malaria patients in regions of China, where malaria is endemic, and demonstrated the growth-inhibitory properties of anti-enolase antibodies, and the protective capacity of recombinant Pven in mice challenged with the mouse malaria parasite Plasmodium berghei.
Results
Plasmid Construction of plasmodium vivax enolase (r-Pven)
The construct for Enolase expression, containing a His-tag for affinity purification, was depicted in . The recombinant plasmid pET28α- Pven protein sequence was verified by DNA sequencing (Nanjing Genscript Bio. Co. Ltd). The correct construct was transformed into the expression host E. coli BL21 (DE3). As shown in , there was an obvious protein band after IPTG induction, which could be detected using the western blotting method with anti-6×His tag antibody. The apparent molecular weight of the r-Pven protein was about 50 kDa.
Figure 1. Schematic illustration of Plasmid Construction of Plasmodium vivax Enolase (r-Pven) in pET28α prokaryotic expression vector with kanamycin resistant gene. (A) For the cDNA encoding Pven protein, the method of overlapping polymerase chain reaction (overlap PCR) was used, with 3 primers P1, P2, and P3; (B) Schematic representation of the expression vector pET28α- r-Pven.
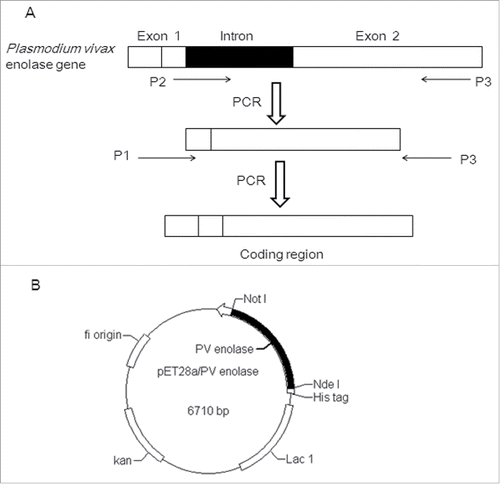
Figure 2. The expression analysis of recombinant Plasmodium vivax Enolase (r-Pven) expressed in E. coli BL21. The expression analysis using 12% SDS-PAGE and Western blotting. Marker: Protein molecular weight marker; lane 1: Crude cells extracts of uninduced E. coli BL21 containing pET28α- r-Pven; lane 2: Crude cells extracts of induced E. coli BL21 containing pET28α- r-Pven; lane 3: Sonicated supernatant of induced E. coli BL21 containing pET28α- r-Pven; lane 4: Sonicated precipitation of induced E. coli BL21 containing pET28α- r-Pven; lane 5: Purified supernatant on Ni2+- IDA His-bind resin; lane 6: Purified supernatant examined using the western blotting.
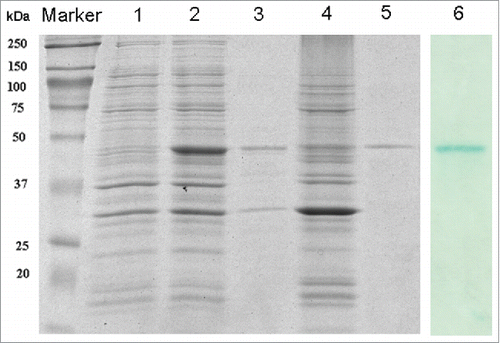
Expression, purification and identification of r-Pven
As described above, after IPTG induction, Ni–NTA resin was used for r-Pven protein purification. Most of the proteins without 6×His tags were removed from the Ni–NTA resin using washing buffer containing 20 mM imidazole, and the 6×His-tagged r-Pven was eluted with more than 90% purity using elution buffer containing 250 mM imidazole (). The purity was estimated through SDS-PAGE gels stained using Coomassie Blue, and the western blotting method was used to further confirm the expression of r-Pven.
Reactivity of r-Pven with seras from plasmodium vivax malaria patients
The well-type amine array was carried out with r-Pven as the substrate, using serum from Plasmodium vivax malaria patients, along with control serum samples, who had not been exposed to malaria. The individual reactivity of serum samples from patients was shown in . Compared with the average values of the reactivity of control serum samples, the average values of the reactivity of serum from vivax malaria patients appeared higher, and there was significant difference between them (p=0.0117<0.05). These results suggested that anti-Pv enolase antibodies were prevalent among Plasmodium vivax malaria patients in China.
ELISA, immunoblotting and growth inhibition assays
In order to examine the antibody titer of the antibodies raised in mice against r-Pven, an ELISA was carried out. As shown in , anti-r-Pven antibody titers were greater than 1:300,000, and even at 1: 500,000, as shown in , the reactivity color of anti-r-Pven antibody could be examined.
Figure 4. Antibody titer was determined for the prepared antisera according to the indirect ELISA method. Test results about anti-r-Pven antibody titers (A) and the corresponding reactivity color of anti-r-Pven antibody (B).
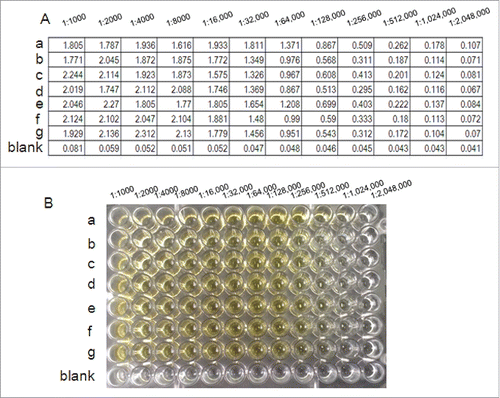
In order to test whether antibodies against r-Pven could inhibit parasite growth, the specificity of the antibodies raised in mice against r-Pven was examined. Whole-cell extracts prepared from P. falciparum and from human erythrocyte were subjected to SDS-PAGE and blotted on a membrane, which was treated with anti-r-Pven antibodies (). The antibodies raised in mice against r-Pven did not react with human erythrocyte proteins. The immunoblotting data showed the specificity of the anti-r-Pven antibodies toward Pfen.
Figure 5. Inhibition of anti-r-Pven antibody against P. falciparum FCC1/HN. (A)Whole-cell extracts prepared from P. falciparum and from human were subjected to SDS-PAGE and blotted on a membrane, and the blot was treated with anti-r-Pven antibodies; (B) Anti-plasmodial assay of anti-r-Pven antibody.
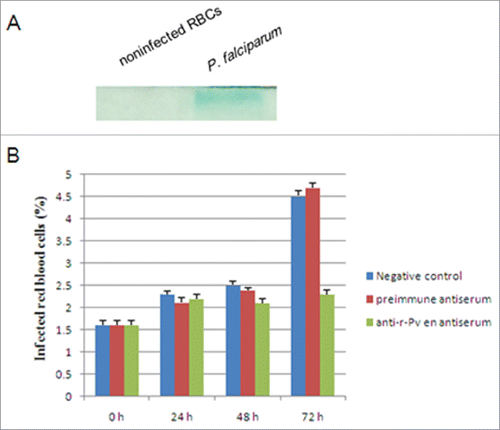
Anti-plasmodial assay was then performed using P. falciparum asexual stages cultures of Plasmodium falciparum, which were exposed to anti-r-Pven antibodies. As shown in , the anti-r-Pven antibodies could reduce the parasite density over the time course of the experiment in relation to controls. At 72 hours post-treatment, the maximum inhibitory effect was observed. These results suggested that the antibodies against Pven could inhibit the growth of the parasites.
Vaccination studies with r-Pven in mice. Next, we tried to evaluate the ability of the Pven to protect mice against a challenge with mouse malaria parasites. In order to demonstrate that the antibodies raised in mice against r-Pven could react with Plasmodium berghei enolase, whole-cell extracts prepared from P. berghei and from mice were subjected to SDS-PAGE and blotted on a membrane, which was treated with anti-r-Pven antibodies (). The antibodies raised in mice against r-Pven did not react with mice erythrocyte proteins. The immunoblotting data confirmed the specificity of the anti-r-Pven antibodies toward P. berghei enolase.
Figure 6. Vaccination study using r-Pfen as an immunogen. (A) Whole-cell extracts prepared from Plasmodium berghei and from mice were subjected to SDS-PAGE and blotted on a membrane, and the blot was treated with anti-r-Pven antibodies; (B) The average parasitemia for each group of mice were shown; (C) The survival pattern for each group of mice were shown.
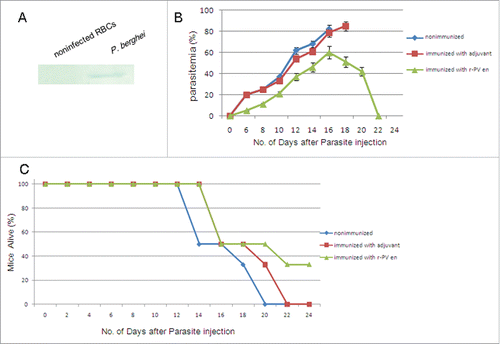
Then, we examined the effects of immunization of mice with r-Pven, followed by a challenge with mouse malaria parasites P. berghei. As shown in , the reduction in parasitemia correlated with the antibody titer present in each mouse. showed the survival profile of the mice. The immunized mice also had a significantly longer survival period. These data suggested that the protection was specific for r-Pven.
Discussion
In this study, we have shown that the Plasmodium vivax enolase protein was immunogenic and that anti-Pv enolase antibodies could protect against the malarial parasite. Protective anti-r-Pven antibodies raised in animals were specific for Plasmodium. These reacted with the enolase from both human and murine Plasmodium species but did not cross-react with the host proteins. So, Pven possesses the properties suitable for a potential candidate malaria vaccine antigen.
In rodent malaria challenge studies, 2 leading vaccine candidates, MSP-119 and AMA-1 (apical membrane antigen 1), showed protection only from homologous, but not heterologous challenge.Citation8,9 In several other studies, it has been reported that protection acrossed a limited number of strains.Citation9,10 A chimeric antigens vaccine including multiple epitopes from different Plasmodium antigens is considered to be an ideal way to address these difficulties. r-Pven was maybe one such candidate. Although it was shown that the Plasmodium enolase protein had considerable homology with the human ortholog, it had certain novel plant-like features,Citation11 and distinct structural differences.Citation12 In this study, the lack of cross-reactivity of anti-r-Pven with host enolases had been shown by methods of immunoblotting.
Enolase was identified as a multifunctional protein, involved in the pathogenicity in several Streptococcus by binding the plasminogen of an infected host,Citation13,14 including the bacterial migration across the mammalian extracellular matrix,Citation15 and an important antigen using immunoproteomics analysis of extracellular and cell-wall associated proteins of SS2.Citation16,17 So far, several reports have shown that the enolase of several types of G+ bacteria could activate plasminogen and then the surface-bond plasmin could degrade extracellular matrix and laminin, and facilitate penetration of the organism through reconstituted basement membrane,Citation18 suggesting that anti-Enolase antibodies could be important as an adjunctive vaccine.Citation19 However, the potential of enolase developed as novel vaccine antigen remained to be convinced.
Conclusion
In this study, we had shown that a housekeeping enolase protein of Plasmodium vivax could protect mice against malaria. An effective malaria vaccine has been shown to be difficult to achieve for several reasons, predominant among which are antigenic variation and diversity. An additional problem is the emerging resistance of the parasite to most antimalarial drugs. Thus, it would be worthwhile to evaluate enolase, a protein critical to the survival of the parasite, for the immunoprophylactic and immunotherapeutic control of malaria. Enolases, which have been well characterized for their catalytic function in glucose metabolism, are no longer considered to be the house-keeping enzymes only. Some of the more interesting and challenging issues, regarding enolase multifunction that need to be addressed are (i) the mechanism of its export to the cell surface, (ii) the role of posttranslational modifications of enolase and implications on its subcellular distribution and function. Investigations of these subjects would provide insights into its important role and it would make this protein an interesting drug target.
Materials and methods
Human serum samples Human serum samples were collected in areas of Anhui province, China. 99 individual serums from Plasmodium vivax malaria patients were selected and tested for reactivity with r- Pven protein. The samples were collected with appropriate ethical clearance after obtaining subject consent. Approximately 0.1 to 1.0 ml of blood was collected using heparinized capillaries or tubes. The geographical distribution, sampling time, and parasitemia of Plasmodium vivax malaria patients were shown in Table S1.
Construction of pET28α- pven protein expressing vector
To obtain the cDNA encoding Pven protein, the method of overlapping polymerase chain reaction (overlap PCR) was used. Three primers for PCR were designed as follows based on the Pven sequence:
P1 -(sense): 5′-cggcCATATGgcccacgtaattactcgtatttctgcccgtgaaattttgg
(box = NdeI site)
P2 -(sense): 5′-gtatttctgcccgtgaaattttggactcccgaggaaacccaaccg
P3-(anti-sense): 5′-agcaGCGGCCGCtcaacttaattggtgcctaaatttctc
(box = NotI site)
The primers P1 and P2 had the overlapping sequence (underlined), and the primers P2 and P3 were used to amplify the part of Exon 1 and Exon 2 from genomic DNA of Plasmodium vivax, which was extracted from whole blood, using QIAamp DNA Blood Mini Kit (QIAGEN, Germany). After the first round of PCR, a second round of PCR was performed using the PCR products of the first round as template with the primers P1 and P3, which resulted in the cDNA encoding Pven protein. The PCR product was then digested by NdeI and NotI and was inserted in frame into the pET28α expression vector (Novagen) (). The recombinant vector was transformed into the competent DH5α cells for propagation of the recombinant plasmid. The recombinant plasmid pET28α-Pven was confirmed, using restriction endonucleases digestion and sequencing.
Expression and purification of plasmodium vivax enolase (r-Pven)
The constructed recombinant plasmid pET28α-Pven was transformed into competent E. coli BL21. One colony of E. coli BL21 harboring the plasmid pET28α-Pven was grown overnight at 37°C in 10 ml of LB medium supplemented with kanamycin (30 μg/ml). The whole culture was inoculated into l L fresh LB medium and grown to an OD600 of 0.6 at 37°C. Fusion protein expression was initiated with the addition of IPTG to 1 mM. After 6 h induction, cells were collected using centrifugation at 7,000 g for 10 min at 4°C, resuspended in binding buffer (20 mM Tris/HCl, 0.5 M NaCl, and 5 mM imidazole pH 7.9), and lysed on ice using sonication at 400 W for 100 cycles (5 s working, 5 s free). The recombinant Plasmodium vivax Enolase in the supernatant of the cell lysate was then purified using Ni-NTA affinity chromatography, according to our previously published method.Citation20
SDS-PAGE and western blotting analyses
The SDS-PAGE analysis was performed, according to Laemmli, using 12% polyacrylamide gels.Citation20 The total expression protein samples from cell lysates after induction were mixed with equivalent sample buffer (125 mM Tris–HCl, pH 6.8, 20% glycerol, 4% SDS, 0.005% bromophenol blue, and 10% 2-mercaptoethanol). Gels were stained with Coomassie brilliant blue R-250.
For western blotting analysis, the same protein sample was separated on a 12% polyacrylamide gel under reducing conditions and then transferred to a polyvinylidene difluoride (PVDF) membrane (Roche Applied Science). The western blotting was performed as described.Citation20 The rabbit IgG secondary antibody was used against His-tag primary antibody (Novagen). The blots were developed using TMB immunoblotting system.
Reactivity of r-Pven with seras from plasmodium vivax malaria patients
99 individual sera from vivax malaria patients were used in well-type amine arrays. The procedure was performed as described in a previous report,Citation9 and the fluorescence intensities of array spots were quantified by the fixed-circle method using ScanArray Express software (version 4.0; PerkinElmer, Boston, MA, USA).Citation21,22 The cut-off value was calculated as the mean fluorescence intensity (MFI) value of reactivity of each patient's serum with r-Pven.
Vaccination studies with r-Pven in mice. Experiments were performed on 3 groups of 8-week-old male Kunming mice (5 mice per group), which were purchased from Beijing Animal Experimental Center, China. The r-Pven was rapidly mixed with an equal volume of Quick Antibody (Kangbiquan Inc., China), a novel water-solubility immunoadjuvant without emulsification to distinguish from the Freund's adjuvant. The resulting mixture was transferred to a 1 mL-syringe and was then injected into the hind leg muscle of a mouse. After 3 weeks, the antigen was dissolved in Quick Antibody adjuvant to conduct a boosting injection in the same (the first injection was 100 μg of r-Pven, followed by 100 μg for the one booster). In one control group, mice were injected in parallel with the adjuvant. The other control group received no injections. After three immunizations, the antibody titers against r-Pven were monitored. Mice having anti-r-Pven antibody titers greater than 1:300,000 were then challenged with Plasmodium berghei (106 parasites per mouse), and parasitemia was monitored daily. Results of the parasitemia profiles were statistically analyzed using one-way analysis of variance (GraphPad InStat, San Diego, CA). Laboratory animal handling and experimental procedures were performed in accordance with the requirements of Provisions and General Recommendation of Chinese Experimental Animals Administration Legislation.
ELISA
Antibody titer was determined for the prepared antisera according to the indirect ELISA method.Citation23 To each well of a 96 well microplate, 100 μL of the pure SUMO-MT solution (10μg/mL) dissolved in the coating buffer (0.5 M Na2CO3/NaHCO3, pH 9.6) was added and the microplate was set still at 4°C overnight. The antiserum was diluted in 1,000–2,048,000 folds with 1×pH 7.4 PBS. The incubation with the secondary antibody was carried out with 100 μL of a 5,000 fold diluted horseradish peroxidase-conjugated goat antibody (GeneTex, USA) diluted with PBSTB (1 9 pH 7.4 PBS, 1 % BSA, 0.1 % Tween). Then added 100 μL substrate mixture of o-phenylenediamine (OPD) (Amresco, USA) per well and incubated for 30 min at room temperature. Finally, 100 μL of 2 M H2SO4 was added to stop the reaction and quantification was performed with a microplate reader (TECAN Infinite M200, Switzerland) at 492 nm.
Anti-plasmodial assay
The P. falciparum FCC1/HN strain was used for the assay. The FCC1/HN line was isolated from Hainan Island, China.Citation24 The strain was cultured in human erythrocytes type A+ at 5 % hematocrit. The strain was cultured in RPMI (Gibco) medium supplemented with HEPES 25 mM, glutamine 2 mM, glucose 2 g/l, NaHCO3 2 g/l, hypoxanthine 29.25 mg/l, gentamicin 60 mg/l and Albumax 1.6 % at pH 7.4. Cultures were kept in 96% nitrogen, 3 % CO2 and 1% oxygen atmosphere at 37°C, and fresh medium was added every 24 h.Citation25
Cultured human erythrocytes at 1.5 % of parasitemia (P. falciparum asexual stages) were cultured in the presence of anti- r-Pven antibodies for 24, 48 and 72 h. Every 24 h, the medium was discharged, and fresh medium with the appropriate anti- r-Pven antibodies concentration was added. The percentage of infected red blood cells was determined by microscopic examination of thin blood films stained with Giemsa. Controls with preimmune antiserum and nontreated infected erythrocytes were included.
Statistical analysis
Results were expressed as mean+SD from at least 3 independent experiments. Statistical analysis was performed according to Student's t-test by one-way analysis of variance. Significant difference was taken as P < 0.05.
Disclosure of potential conflicts of interest
The authors declare that there are no conflicts of interest.
Author contributions
Conceived and designed the experiments: Chao Zhang, Jun Cao, Qi Gao. Performed the experiments: Chao Zhang, Yaping Gu, Jianxia Tang, Feng Lu Yuanyuan Cao. Analyzed the data: Chao Zhang, Huayun Zhou, Guoding Zhu, Jun Cao, Qi Gao. Wrote the paper: Chao Zhang, Jun Cao, Qi Gao.
Supplemental Files
Download MS Word (112 KB)Funding
This work was supported by grants from National Nature Science Foundation of China (81101276 and 81271870), Nature Science Foundation of Jiangsu Province (BK2011163 and BK20150001), Jiangsu Science and Technology Department (BM2015024), and Jiangsu Health Department (X201127).
References
- Arévalo-Herrera M, Lopez-Perez M, Dotsey E, Jain A, Rubiano K, Felgner PL, Davies DH, Herrera S. Antibody Profiling in Naïve and Semi-immune Individuals Experimentally Challenged with Plasmodium vivax Sporozoites. PLoS Negl Trop Dis 2016; 10(3):e0004563; http://dx.doi.org/10.1371/journal.pntd.0004563
- Mueller I, Galinski MR, Baird JK, Carlton JM, Kochar DK, Alonso PL, del Portillo HA. Key gaps in the knowledge of Plasmodium vivax, a neglected human malaria parasite. Lancet Infect Dis 2009; 9:555-66; PMID:19695492; http://dx.doi.org/10.1016/S1473-3099(09)70177-X
- Girard MP, Reed ZH, Friede M, Kieny MP. A review of human vaccine research and development: malaria. Vaccine 2007; 25:1567-80; PMID:17045367; http://dx.doi.org/10.1016/j.vaccine.2006.09.074
- Gupta SK, Sisodia BS, Sinha S, Hajela K, Naik S, Shasany AK, Dube A. Proteomic approach for identification and characterization of novel immunostimulatory proteins from soluble antigens of Leishmania donovani promastigotes. Proteomics 2007; 7:816-23; PMID:17295358; http://dx.doi.org/10.1002/pmic.200600725
- A´ngels DR, Anna RB, Ana GM, Roser LA. α-Enolase, a Multifunctional Protein: Its Role on Pathophysiological Situations. J Biomed Biotechnol 2012; 2012:156795; PMID:23118496
- Montagnoli C, Sandini S, Bacci A, Romani L, La Valle R. Immunogenicity and protective effect of recombinant enolase of Candida albicans in a murine model of systemic candidiasis. Med Mycol 2004; 42:319-24; PMID:15473356; http://dx.doi.org/10.1080/13693780310001644653
- Finco O, Bonci A, Agnusdei M, Scarselli M, Petracca R, Norais N, Ferrari G, Garaguso I, Donati M, Sambri V, et al. Identification of new potential vaccine candidates against Chlamydia pneumoniae by multiple screenings. Vaccine 2005; 23:1178-88; PMID:15629361; http://dx.doi.org/10.1016/j.vaccine.2004.07.045
- Renia L, Ling IT, Marussig M, Miltgen F, Holder AA, Mazier D. Immunization with a recombinant C-terminal fragment of Plasmodium yoelii merozoite surface protein 1 protects mice against homologous but not heterologous P. yoelii sporozoite challenge. Infect Immun 1997; 65:4419-23; PMID:9353014
- Crewther PE, Matthew ML, Flegg RH, Anders RF. Protective immune responses to apical membrane antigen 1 of Plasmodium chabaudi involve recognition of strain-specific epitopes. Infect Immun 1996; 64:3310-7; PMID:8757869
- Majarian WR, Daly TM, Weidanz WP, Long CA. Passive immunization against murine malaria with an IgG3 monoclonal antibody. J Immunol 1984; 132:3131-7; PMID:6725950
- Narum DL, Ogun SA, Batchelor AH, Holder AA. Passive immunization with a multicomponent vaccine against conserved domains of apical membrane antigen 1 and 235-kgdalton rhoptry proteins protects mice against Plasmodium yoelii blood-stage challenge infection. Infect Immun 2006; 74:5529-36; PMID:16988228; http://dx.doi.org/10.1128/IAI.00573-06
- Sato K, Kano S, Matsumoto Y, Glanarongran R, Krudsood S, Looareesuwan S, Aikawa M, Suzuki M. Application of yeast enolase as antigen for immunodiagnosis of malaria. Southeast Asian J Trop Med Public Health 2000; 31:79-84; PMID:11414465
- Jones MN, Holt RG. Cloning and characterization of an α-enolase of the oral pathogen Streptococcus mutans that binds human plasminogen. Biochem Biophys Res Commun 2007; 364(4):924-9; PMID:17964283; http://dx.doi.org/10.1016/j.bbrc.2007.10.098
- Veiga-Malta I, Duarte M, Dinis M, Tavares D, Videira A, Ferreira P. Enolase from Streptococcus sobrinus is an immunosuppressive protein. Cell Microbiol 2004; 6(1):79-88; PMID:14678332; http://dx.doi.org/10.1046/j.1462-5822.2003.00344.x
- Pancholi V. Multifunctional α-enolase: its role in diseases. Cell Mol Life Sci 2001; 58(7):902-20; PMID:11497239; http://dx.doi.org/10.1007/PL00000910
- Jing HB, Yuan J, Wang J, Yuan Y, Zhu L, Liu XK, Zheng YL, Wei KH, Zhang XM, Geng HR, et al. Proteome analysis of Streptococcus suis serotype 2. Proteomics 2008; 8(2):333-49; PMID:18081191; http://dx.doi.org/10.1002/pmic.200600930
- Zhang A, Xie C, Chen H, Jin M. Identification of immunogenic cell wall-associated proteins of Streptococcus suis serotype 2. Proteomics 2008; 8(17):3506-15; PMID:18686301; http://dx.doi.org/10.1002/pmic.200800007
- Eberhard T, Kronvall G, Ullberg M. Surface bound plasmin promotes migration of Streptococcus pneumoniae through reconstituted basementmembranes. Microb Pathog 1999; 26(3):175-81; PMID:10089158; http://dx.doi.org/10.1006/mpat.1998.0262
- Whiting GC, Evans JT, Patel S, Gillespie SH. Purification of native α-enolase from Streptococcus pneumoniae that binds plasminogen and is immunogenic. J Med Microbiol 2002; 51(10):837-43; PMID:12435062; http://dx.doi.org/10.1099/0022-1317-51-10-837
- Zhang C, He X, Gu Y, Zhou H, Cao J, Gao Q. Recombinant Scorpine Produced Using SUMO Fusion Partner in Escherichia coli Has the Activities against Clinically Isolated Bacteria and Inhibits the Plasmodium falciparum Parasitemia In Vitro. PLoS ONE 2014; 9(7):e103456; PMID:25068263; http://dx.doi.org/10.1371/journal.pone.0103456
- Chen JH, Jung JW, Wang Y, Ha KS, Lu F, Lim CS, Takeo S, Tsuboi T, Han ET. Immunoproteomics profiling of blood stage Plasmodium vivax infection by high-throughput screening assays. J Proteome Res 2010; 9:6479-89; PMID:20949973; http://dx.doi.org/10.1021/pr100705g
- Lu F, Li J, Wang B, Cheng Y, Kong DH, Cui L, Ha KS, Sattabongkot J, Tsuboi T, Han ET. Profiling the humoral immune responses to Plasmodium vivax infection and identification ofcandidate immunogenic rhoptry-associated membrane antigen (RAMA). J Proteomics 2014; 102:66-82; PMID:24607491; http://dx.doi.org/10.1016/j.jprot.2014.02.029
- He Y, Ma W, Li Y, Liu J, Jing W, Wang L. Expression of metallothionein of freshwater crab (Sinopotamon henanense) in Escherichia colienhances tolerance and accumulation of zinc, copper and cadmium. Ecotoxicology 2014; 23(1):56-64; PMID:24276409; http://dx.doi.org/10.1007/s10646-013-1151-0
- Pan WQ, Huang DQ, Zhang QF, Li Qu, Zhang DM, Xue X, Qian F. Fusion of Two Malaria Vaccine Candidate Antigens Enhances Product Yield, Immunogenicity, and Antibody-Mediated Inhibition of Parasite Growth In Vitro. J Immunol 2004; 172:6167-74; PMID:15128804; http://dx.doi.org/10.4049/jimmunol.172.10.6167
- Trager W, Jensen JB. Human malaria parasites in continuous culture. Science 1976; 193:673-5; PMID:781840; http://dx.doi.org/10.1126/science.781840